DOI:
10.1039/D3QO00255A
(Research Article)
Org. Chem. Front., 2023,
10, 2680-2687
A Ru(II)-catalyzed C–H activation and annulation cascade for the construction of highly coumarin-fused benzo[a]quinolizin-4-ones and pyridin-2-ones†
Received
22nd February 2023
, Accepted 14th April 2023
First published on 17th April 2023
Abstract
A reaction involving a Ru(II)-catalyzed C–H activation and annulation cascade was successfully established for constructing coumarin-fused benzo[a]quinolizin-4-ones and pyridin-2-ones. In these constructions, an intriguing 6-6-6-6-6 pentacyclic coumarin-fused scaffold was formed as a result of two successive C–H bond activation/[4 + 2] annulation cascade in a one-pot operation with moderate to good yields, and a broad range of substrates were amenable to the reaction. More importantly, this work was the first time, to the best of our knowledge, that such complex 6-6-6-6-6 pentacyclic coumarin scaffolds were constructed using a successive Ru(II)-catalyzed C–H activation and annulation cascade. The resulting products were further transformed into two new interesting skeletons, and biological evaluations showed the highly fused products having good affinity for the ENL YEATS domain.
Introduction
Fused-coumarins represent an important class of privileged structural scaffolds and are extensively and frequently found in natural products, pharmaceutical molecules and functional materials.1 Some representative examples of such natural and artificial products include defucogilvocarin (M, E and V),2 lamellarin D, the synthesized derivatives isolamellarins3 and compounds I–III.4 These products show extensive biological activities such as HIV-1 integrase inhibition,5 anticancer,6 antibacterial,7,8 and anti-inflammatory activities8 (Fig. 1). Furthermore, some of these derivatives also show excellent photophysical properties and are widely used as fluorescent labeling probes.9
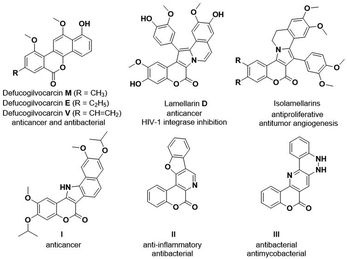 |
| Fig. 1 Examples of coumarin-fused heterocyclic derivatives. | |
These potential benefits have for a long time attracted the interest of chemists, specifically for developing effective synthetic strategies to construct diverse coumarin-based compounds for the purpose of expanding the chemical space of drug discovery or other applications. Recently, a few classic protocols have been used to prepare these intriguing coumarin-based derivatives. For instance, multiple research groups have employed coumarins bearing an electron-withdrawing group (EWG) at the C3 position as the starting point to build cyclopropa[c]coumarins with different single-carbon coupling partners by performing classic Lewis base (LB)-catalyzed cyclopropanations (Scheme 1a, left).10 The Ye,11a Lu11b and Lin11c groups independently introduced coupling partners each containing two reactive sites (with examples of these partners including ketenes, allenes, azomethine ylides, etc.) to construct tricyclic benzopyrano[3,4-c]pyrrolidines via classic cycloaddition reaction pathways (Scheme 1a, right).11 However, in contrast to the above achievements, the construction of highly fused polycyclic coumarin scaffolds has still been full of challenges and lack developed synthetic strategies, with only a few examples having been reported. The Gryko and Tang groups did report a cyclocondensation reaction involving the treatment of coumarin 3-carboxylate with 1,8-diazabicyclo[5.4.0]undec-8-ene (DBU) to synthesize pentacyclic coumarin derivatives displaying fluorescence characteristics, but the substrate scope was very limited.12,13 The Bu group constructed a 6-6-5-5-6 pentacyclic core by carrying out a Friedel–Crafts alkylation/cyclization/isomerization sequence between 3-benzoyl coumarins and 3-substituted indoles (Scheme 1b).14 Similarly, dihydroisoquinoline with classic cycloaddition strategies was used to build polycyclic coumarin-derived 8-oxoprotoberbines.15 The substrates for these constructions were relatively complex and needed to be prepared using multiple reaction steps. It has so far been rare and difficult to build highly coumarin-fused derivatives by using simple starting materials via a one-pot multi-step reaction strategy involving C–H activation and cyclization cascade steps. As a continuation of our interest and efforts in investigating new strategies for constructing diverse drug-like polycyclic scaffolds,16 we set out in the current work to construct highly coumarin-fused benzo[a]quinolizin-4-ones and pyridin-2-ones using a Ru(II)-catalyzed cascade reaction of 3-carboxamide coumarin and diphenylethyne; this strategy offered high efficiency, a wide substrate scope and potential versatility (Scheme 1c).
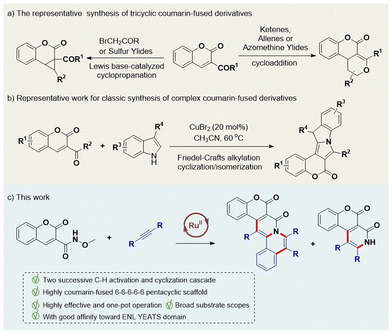 |
| Scheme 1 Various methods for synthesizing fused coumarins. | |
Results and discussion
We started our investigation by using N-methoxy-coumarin-3-carboxamide (1a, 0.4 mmol) and biphenylacetylene (2a, 0.4 mmol) as the model substrates, which were treated with [Cp*RhCl2]2 (4 mol%), AgSbF6 (16 mol%), Cu(OAc)2·H2O (2 equiv.) in DCE at 90 °C for 12 h (Table 1, entry 1). Encouragingly, an obvious new product was found, and the corresponding NMR and mass spectroscopy analyses indicated that it may have been a highly fused coumarin derivative with a 6-6-6-6-6 pentacyclic scaffold (3aa) (Table 1, entry 1). We speculated that the coumarin substrate underwent two successive C–H bond activation/annulation cascade to give this highly fused coumarin derivative, and its structure was unambiguously confirmed from the results of an X-ray crystallographic analysis. In view of this intriguing polycyclic coumarin-fused scaffold, we decided to optimize the reaction conditions to most efficiently construct such skeletons.
Table 1 Optimization of reaction conditionsa,b
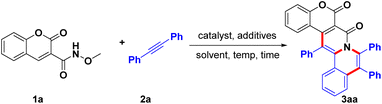
|
Entry |
Catalyst |
Ag salt |
Additive |
Solvent |
Yieldb (%) |
Reaction conditions: 1a (0.4 mmol), 2a (1.2 mmol), [Cp*RhCI2]2 (4 mol%), Ag salt (16 mol%), additive (2 equiv.) in DCE at 90 °C for 12 h.
Isolated yield.
Zn(OAc)2 (0.5 equiv.).
Zn(OAc)2 (3 equiv.).
Trace.
70 °C.
110 °C.
|
1 |
[Cp*RhCl2]2 |
AgSbF6 |
Cu(OAc)2·H2O |
DCE |
26 |
2 |
[Ru(p-cymene)Cl2]2 |
AgSbF6 |
Cu(OAc)2·H2O |
DCE |
40 |
3 |
[Ir*CpCl2]2 |
AgSbF6 |
Cu(OAc)2·H2O |
DCE |
35 |
4 |
[Co*CpCl2]2 |
AgSbF6 |
Cu(OAc)2·H2O |
DCE |
NR |
5 |
[Ru(p-cymene)Cl2]2 |
AgSbF6 |
Cu(OAc)2 |
DCE |
42 |
6 |
[Ru(p-cymene)Cl2]2 |
AgSbF6 |
AgOAc |
DCE |
30 |
7 |
[Ru(p-cymene)Cl2]2 |
AgSbF6 |
CsOAc |
DCE |
NR |
8 |
[Ru(p-cymene)Cl2]2 |
AgSbF6 |
K2CO3 |
DCE |
NR |
9 |
[Ru(p-cymene)Cl2]2 |
AgSbF6 |
HOAc |
DCE |
25 |
10
|
[Ru(p-cymene)Cl
2
]
2
|
AgSbF
6
|
Zn(OAc)
2
|
DCE
|
70
|
11c |
[Ru(p-cymene)Cl2]2 |
AgSbF6 |
Zn(OAc)2 |
DCE |
60 |
12d |
[Ru(p-cymene)Cl2]2 |
AgSbF6 |
Zn(OAc)2 |
DCE |
65 |
13 |
[Ru(p-cymene)Cl2]2 |
CF3PO2Ag |
Zn(OAc)2 |
DCE |
41 |
14 |
[Ru(p-cymene)Cl2]2 |
AgNTF2 |
Zn(OAc)2 |
DCE |
32 |
15 |
[Ru(p-cymene)Cl2]2 |
AgPF6 |
Zn(OAc)2 |
DCE |
36 |
16 |
[Ru(p-cymene)Cl2]2 |
|
Zn(OAc)2 |
DCE |
—e |
17 |
[Ru(p-cymene)Cl2]2 |
AgSbF6 |
Zn(OAc)2 |
MeCN |
18 |
18 |
[Ru(p-cymene)Cl2]2 |
AgSbF6 |
Zn(OAc)2 |
Toluene |
37 |
19 |
[Ru(p-cymene)Cl2]2 |
AgSbF6 |
Zn(OAc)2 |
Dioxane |
20 |
20 |
[Ru(p-cymene)Cl2]2 |
AgSbF6 |
Zn(OAc)2 |
TFE |
46 |
21f |
[Ru(p-cymene)Cl2]2 |
AgSbF6 |
Zn(OAc)2 |
DCE |
60 |
22g |
[Ru(p-cymene)Cl2]2 |
AgSbF6 |
Zn(OAc)2 |
DCE |
70 |
First, we investigated the effects of different catalysts (Table 1, entries 1–4). Of the catalysts tested, [Ru(p-cymene)Cl2]2 showed the best catalytic effect, with the yield of 3aa reaching 40% (Table 1, entry 2). The screening of reaction additives was subsequently carried out (Table 1, entries 5–10). When using Zn(OAc)2 as the additive, the yield of 3aa was 70% (Table 1, entry 10). To further improve the effect of additives, we changed the number of equivalents of the additive from 2 to 0.5 in one test and to 3 in another (Table 1, entries 11 and 12), but both tests showed reduced yields of 3aa. In a screening of silver salts, AgSbF6 provided the highest yield and the removal of silver salts resulted in no target product (Table 1, entries 13–16). Subsequently, we kept the optimal catalytic system to investigate reaction solvents. The originally used DCE remained the best reaction solvent for this transformation (Table 1, entries 17–20). Finally, the reaction temperature was investigated. We found a lower yield of 3aa when lowering the reaction temperature from 90 °C to 70 °C (Table 1, entries 21 and 22), and the most suitable temperature was hence determined to be 90 °C. Based on these screenings, the optimal conditions for this reaction were determined to involve stirring [Ru(p-cymene)Cl2]2 (4 mol%), AgSbF6 (16 mol%), Zn(OAc)2 (2 equiv.) in DCE at 90 °C for 12 h (Table 1, entry 10).
Under the optimal reaction conditions, we set out to assess the scopes of the two substrates (Table 2). First, we investigated the influences of different substituents of the biphenylacetylene on this reaction (2a–2g). We tested groups with different electron properties introduced into the para-position of phenyl group of biphenylacetylene 2a. Substituting in electron-donating groups and halogen atoms had almost no effect on the target product yield (3ab–3af), but including a trifluoromethyl group resulted in a large decrease in the yield (3ag), indicating that the electron-withdrawing groups may be detrimental for this transformation. We next evaluated the influences of the substituents of the coumarin ring on the yields of target pentacyclic coumarin derivatives. For instance, we introduced independently methyl, methoxy, fluoro, chloro and trifluoro groups into the benzene ring of the coumarin scaffold, mainly involving 6-position and 7-position substitutions, and the results demonstrated that all these substrates could be easily transformed to corresponding products with good yields (3ba–3ge), indicating a good substrate tolerance for this strategy.
Table 2 Scope of the two substratesa,b
Reaction conditions: 1 (0.4 mmol) and 2 (1.2 mmol), [Ru(p-cymene)Cl2]2 (4 mol%), AgSbF6 (16 mol%), Zn(OAc)2 (2 equiv.) in DCE at 90 °C, for 12 h.
Isolated yields.
|
|
In order to further investigate the reaction scope of this strategy, we introduced examples of symmetric aliphatic alkynes instead of arylalkynes for this transformation (Table 3). As we expected, the coumarin-fused pyridin-2-one scaffold could also be formed via a single C–H bond activation and annulation cascade under Ru(II) catalysis. First, 3-carboxamide coumarins (1a, 1b, 1d and 1e) were investigated to couple alkyl alkynes (2h and 2i) under the standard reaction conditions, respectively, and the corresponding coumarin-fused pyridin-2-one products were obtained with good yields (3ah–3eh). We tried to react diethyl acetylenedicarboxylate with N-methoxy-2-oxo-2H-chromene-3-carboxamide, but target product 3ak was obtained in only 15% yield. When replacing symmetric alkynes with asymmetric alkynes, such as 1-phenyl-1-propyne, the reaction afforded three products, but only 3aj as a major product was isolated; its structure was confirmed from the results of an X-ray crystallographic analysis.
Table 3 Aliphatic alkyne substrate scopea,b
Reaction conditions: 1 (0.4 mmol), 2 (1.2 mmol), [Ru(p-cymene)Cl2]2 (4 mol%), Ag salt (16 mol%), Zn(OAc)2 (2 equiv.) in DCE at 90 °C, for 12 h.
Isolated yields.
|
|
To assess the efficiency of these methods and their potential for being utilized, a gram-scale experiment for model substrates 1a and 2a was performed, and target product 3aa was obtained with an isolated yield of 65%; and when substrate 2h was used, product 3ah with an isolated yield of 34% was obtained (Scheme 2a). More interestingly, products 3aa and 3ga were further converted into biaryl compound 4a and 4b in DMF–POCl3 mixed solvent under heated conditions. Here, this transformation may have each undergone amide bond breakage and subsequent intramolecular cyclization (Scheme 2b). The structure of 4a was verified by carrying out an X-ray crystallographic analysis. Additionally, product 3ah was easily converted into pyridine-fused coumarin derivative 5 in a rapid reduction reaction with borane-methyl sulfide complex; this derivative may have some potential pharmacological activities and fluorescence properties (Scheme 2c).17
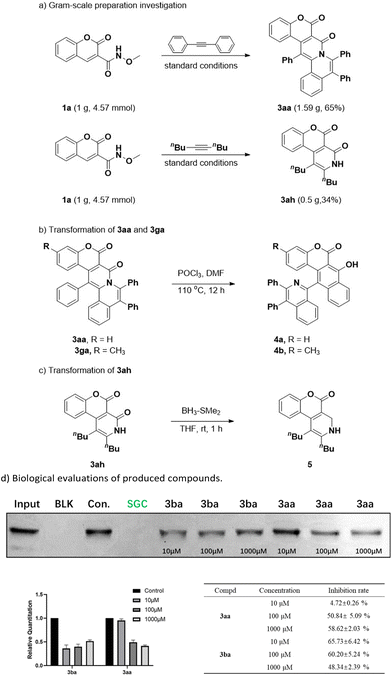 |
| Scheme 2 Synthesis application and biological evaluation (the YEATS domain inhibitor SGC as a positive control).18b | |
In order to investigate the potential biological value of these highly fused coumarin scaffolds, we evaluated their affinities for the ENL YEATS domain. Histone acylation modification and gene transcriptional activity are closely related to the recognition of acetylation modification (Kac) or croton acylation modification (Kcr) on lysine residues by YEATS domain. The dysregulation of the YEATS (YAF9, ENL, AF9, TAF14 and SAS5)-domain-containing proteins correlates with the onset and progression of cancers. In particular, interest in the ENL YEATS domain has been very high due to the reported significant involvement of this domain in acute leukemia pathophysiology.18a Therefore, the development of small-molecule inhibitors targeting the ENL YEATS domain may provide a new strategy for the treatment of ENL-dependent leukemia. In order to investigate the potential biological value of these highly coumarin-fused scaffolds, pull-down experiments were carried out to verify the binding inhibitory activities of these compounds against the ENL YEATS domain and H3K9cr (which has a high affinity for the ENL YEATS domain), and the results indicated good inhibitory activities displayed by compounds 3aa and 3ba (Scheme 2d), which laid a foundation for subsequent studies.
Then, we performed mechanism studies. First, a competition experiment between the electron-withdrawing substituted substrate 1d and electron-donating substituted substrate 1c with diphenylalkyne 2a was performed. The results showed that the electron-withdrawing substrate reacted faster than did the electron-donating substrate with a ratio of 1.35
:
1; a similar result was also observed when reacting substrates 1d and 1b with dec-5-yne (2h), and a ratio of 3.15
:
1 was measured (Scheme 3a). These results indicated that the C–H activation might proceed through a concerted metalation–deprotonation (CMD) mechanism.19 Also, by decreasing the number of equivalents of biphenylacetylene and shortening the reaction time, we trapped intermediate VI (Scheme 3b). In addition, we performed an H/D exchange experiment to attempt to gain insights into the preliminary mechanism of this reaction. First, when treating the model substrate 1a without substrate 2a under standard reaction conditions with D2O for 12 h, we found that in about 10% of the product molecules, the 4-position of the 3-carboxamide coumarin scaffold was exchanged with a deuterium atom (Scheme 3c), indicating that this C–H bond activation was reversible.
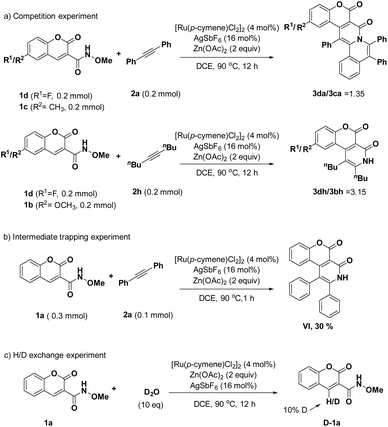 |
| Scheme 3 Preliminary mechanism studies. | |
Based on literature reports20 and the H/D exchange experiment, we derived a speculative yet plausible mechanism for this transformation (Scheme 4). According to this mechanism, the first step was the activation of the ruthenium catalyst under the effect of AgSbF6 and Zn(OAc)2, inducing then a C4 metalation of coumarin 1a to form a 5-membered ring I with the activated catalyst. Diphenylalkyne complexed with I, followed by the coordination of alkyne to the cyclometalation complex II and insertion to give a seven-membered Ru-metallacycle III, with III becoming converted into IV through an internal oxidation, and with reductive elimination of IV forming V. When R was an alkyl group, the catalytic cycle afforded coumarin-fused pyridin-2-one scaffold VI. When R was an aryl group, another C–H bond activation catalytic cycle occurred to construct complex 6-6-6-6-6 pentacyclic coumarin scaffold 3aa.
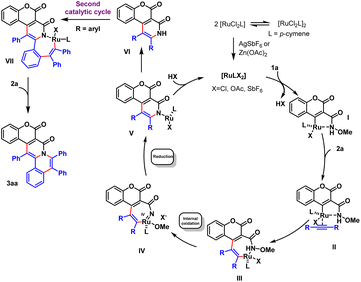 |
| Scheme 4 Proposed reaction mechanism. | |
Conclusions
In conclusion, we have successfully established a novel and effective strategy to construct two kinds of highly fused coumarin scaffolds with a benzo[a]quinolizin-4-one core or pyridin-2-one core by carrying out a Ru(II)-catalyzed C–H activation and annulation cascade, in which the construction of a 6-6-6-6-6 pentacyclic coumarin scaffold underwent two successive C–H bond activation/[4 + 2] annulation cascade in a one-pot operation with good yields. In this reaction, the Ru(II)-catalyzed C–H activation and annulation cascade strategy was for the first time performed, to the best of our knowledge, to construct such complex 6-6-6-6-6 pentacyclic coumarin scaffolds. Additionally, this transformation was shown to tolerate a broad range of substrates, and provide moderate to good yields, with the resulting polycyclic compounds containing an important drug-privileged scaffold, and perhaps having potential biological applications for further drug discovery.
Conflicts of interest
There are no conflicts to declare.
Acknowledgements
We thank the National Natural Science Foundation of China (82173656, 21977106, 82151219), Shandong Laboratory of Yantai Drug Discovery and Bohai Rim Advanced Research Institute for Drug Discovery for financial support.
Notes and references
-
(a) A. M. Vijesh, A. M. Isloor, V. Prabhu, S. Ahmad and S. Malladi, Synthesis, characterization and anti-microbial studies of some novel 2,4-disubstituted thiazoles, Eur. J. Med. Chem., 2010, 45, 5460–5464 CrossRef CAS;
(b) A. Stefanachi, F. Leonetti, L. Pisani, M. Catto and A. Carotti, Coumarin: A Natural, Privileged and Versatile Scaffold for Bioactive Compounds, Molecules, 2018, 23, 250 CrossRef PubMed;
(c) D. Srikrishna, C. Godugu and K. P. Dubey, A Review on Pharmacological Properties of Coumarins, Mini-Rev. Med. Chem., 2018, 18, 113–141 CrossRef CAS PubMed;
(d) S. Sandhu, Y. Bansal, O. Silakari and G. Bansal, Coumarin hybrids as novel therapeutic agents, Bioorg. Med. Chem., 2014, 22, 3806–3814 CrossRef CAS PubMed;
(e) J. Nawrot-Modranka, E. Nawrot and J. Graczyk, In vivo antitumor, in vitro antibacterial activity and alkylating properties of phosphorohydrazine derivatives of coumarin and chromone, Eur. J. Med. Chem., 2006, 41, 1301–1309 CrossRef CAS PubMed;
(f) C. A. Kontogiorgis and D. J. Hadjipavlou-Litina, Synthesis and Antiinflammatory Activity of Coumarin Derivatives, J. Med. Chem., 2005, 48, 6400–6408 CrossRef CAS PubMed;
(g) K. Kasperkiewicz, M. B. Ponczek, J. Owczarek, P. Guga and E. Budzisz, Antagonists of Vitamin K-Popular Coumarin Drugs and New Synthetic and Natural Coumarin Derivatives, Molecules, 2020, 25, 1465 CrossRef CAS PubMed;
(h) X.-L. Hu, C. Gao, Z. Xu, M.-L. Liu, L.-S. Feng and G.-D. Zhang, Recent Development of Coumarin Derivatives as Potential Antiplasmodial and Antimalarial Agents, Curr. Top. Med. Chem., 2018, 18, 114–123 CrossRef CAS PubMed;
(i) Y. Dong, K. Nakagawa-Goto, C.-Y. Lai, S. L. Morris-Natschke, K. F. Bastow and K.-H. Lee, Antitumor agents 278. 4-Amino-2H-benzo[h]chromen-2-one (ABO) analogs as potent in vitro anti-cancer agents, Bioorg. Med. Chem. Lett., 2010, 20, 4085–4087 CrossRef CAS PubMed;
(j) D. Cao, Z. Liu, P. Verwilst, S. Koo, P. Jangjili, J. S. Kim and W. Lin, Coumarin-Based Small-Molecule Fluorescent Chemosensors, Chem. Rev., 2019, 119, 10403–10519 CrossRef CAS.
- X. Huang, T. Zhu, Z. Huang, Y. Zhang, Z. Jin, G. Zanoni and Y. R. Chi, Carbene-Catalyzed Formal [5 + 5] Reaction for Coumarin Construction and Total Synthesis of Defucogilvocarcins, Org. Lett., 2017, 19, 6188–6191 CrossRef CAS.
-
(a) T. Balalas, A. Abdul-Sada, D. Hadjipavlou-Litina and K. Litinas, Pd-Catalyzed Efficient Synthesis of Azacoumestans Via Intramolecular Cross Coupling of 4-(Arylamino)coumarins in the Presence of Copper Acetate under Microwaves, Synthesis, 2017, 2575–2583 CAS;
(b) N. Thasana, R. Worayuthakarn, P. Kradanrat, E. Hohn, L. Young and S. Ruchirawat, Copper(I)-Mediated and Microwave-Assisted Caryl–Ocarboxylic Coupling: Synthesis of Benzopyranones and Isolamellarin Alkaloids, J. Org. Chem., 2007, 72, 9379–9382 CrossRef CAS PubMed;
(c) T. Khan, V. Kumar and O. Das, An Improved Synthesis of Natural Product Inspired Chromenopyrrolizines and Chromenoindolizines Scaffolds: Rapid Access to the Diverse Pyrrolizine Analogs of Aza-Medicarpin and Tetracyclic Isolamellarin Core through a General Base and Metal Free Strategy, Bull. Chem. Soc. Jpn., 2016, 89, 1331–1340 CrossRef CAS.
-
(a)
M. Iwao, F. Ishibashi, T. Fukuda and H. Hasegawa, Patent WO 99129, 2012;
(b) L. V. Frolova, I. Malik, P. Y. Uglinskii, S. Rogelj, A. Kornienko and I. V. Magedov, Multicomponent Synthesis of 2,3-Dihydrochromeno[4,3-d]pyrazolo[3,4-b]pyridine-1,6-diones: A Novel Heterocyclic Scaffold with Antibacterial Activity, Tetrahedron Lett., 2011, 52, 6643–6645 CrossRef CAS PubMed.
- M. V. R. Reddy, M. R. Rao, D. Rhodes, M. S. T. Hansen, K. Rubins, F. D. Bushman, Y. Venkateswarlu and D. J, Faulkner Lamellarin α 20-Sulfate, an Inhibitor of HIV-1 Integrase Active against HIV-1 Virus in Cell Culture, J. Med. Chem., 1999, 42, 1901–1907 CrossRef CAS.
-
(a) C. Bailly, Anticancer properties of lamellarins, Mar. Drugs, 2015, 13, 1105–1123 CrossRef CAS PubMed;
(b) P. Pahari, M. K. Kharel, M. D. Shepherd, S. G. van Lanen and J. Rohr, Enzymatic total synthesis of defucogilvocarcin M and its implications for gilvocarcin biosynthesis, Angew. Chem., Int. Ed., 2012, 51, 1216–1220 CrossRef CAS.
- M. Renuka, T. Harold and P. Ramesh, Defucogilvocarcin V, a new antibiotic from Streptomyces arenae, 2064: isolation, characterization, partial synthesis and biological activity, J. Antibiot., 1985, 38, 1280–1283 CrossRef.
- I. A. Khan, M. V. Kulkarni, M. Gopal, M. S. Shahabuddin and C. M. Sun, Synthesis and biological evaluation of novel angularly fused polycyclic coumarins, Bioorg. Med. Chem. Lett., 2005, 15, 3584–3587 CrossRef CAS.
-
(a) K.-S. Lee, H.-J. Kim, G.-H. Kim, I. Shin and J.-I. Hong, Fluorescent Chemodosimeter for Selective Detection of Cyanide in Water, Org. Lett., 2008, 10, 49–51 CrossRef CAS PubMed;
(b) Y. Zhao, Q. Zheng, K. Dakin, K. Xu, M. L. Martinez and W.-H. Li, New Caged Coumarin Fluorophores with Extraordinary Uncaging Cross Sections Suitable for Biological Imaging Applications, J. Am. Chem. Soc., 2004, 126, 4653–4663 CrossRef CAS PubMed.
-
(a) H. Tsuchida, M. Tamura and E. Hasegawa, Cyclization and Ring-Expansion Processes Involving Samarium Diiodide Promoted Reductive Formation and Subsequent Oxidative Ring Opening of Cyclopropanol Derivatives, J. Org. Chem., 2009, 74, 2467–2475 CrossRef CAS;
(b) J.-C. Sun, J.-L. Li, C.-B. Ji, Y.-Y. Peng and X.-P. Zeng, Construction of Cyclopropa[c]coumarins via cascade Michael-alkylation process of 3-cyanocoumarin with 2-bromomalonate, Tetrahedron, 2020, 76, 130852 CrossRef CAS;
(c) J. C. Sun, X. H. Wang, C. B. Ji, Y. Y. Peng and X. P. Zeng, Enantioselective Construction of Chiral Cyclopropa[c]coumarins via Lewis Base-Catalyzed Cyclopropanation, J. Org. Chem., 2020, 85, 14963–14970 CrossRef CAS PubMed;
(d) V. V. Shchepin, M. M. Kalyuzhnyi, P. S. Silaichev, N. Y. Russkikh, R. V. Shchepin, M. A. Ezhikova and M. I. Kodess, Reactions of zinc enolates derived from 1-aryl-2,2-di-bromoalkanones with 2-acyl-3H-benzo[f]chromen-3-ones, 6-bromo-2-oxochromene-3-carboxamides, and 3-oxo-3H-benzo-[f]chromene-2-carboxamides, Russ. J. Org. Chem., 2004, 40, 1353–1358 CrossRef CAS;
(e) X.-S. Meng, S. Jiang, X.-Y. Xu, Q.-X. Wu, Y.-C. Gu and D.-Q. Shi, Stabilized Sulfur Ylide Mediated Cyclopropanations and Formal [4+1] Cycloadditions of 3-Acyl-2H-chromenones and Their Imines, Eur. J. Org. Chem., 2016, 4778–4781 CrossRef CAS;
(f) S. K. Jonnalagadda, B. I. Huwaimel, S. Jonnalagadda, J. C. Garrison and P. C. Trippier, Access to Highly Strained Tricyclic Ketals Derived from Coumarins, J. Org. Chem., 2022, 87, 4476–4482 CrossRef CAS PubMed;
(g) A. Bojilova, A. Trendafilova, C. Ivanov and N. A. Rodios, Cyclopropanation reaction of 3-Acyl-2H-1-benzopyran-2-ones with phenacylbromide in phase transfer systems, Tetrahedron, 1993, 49, 2275–2286 CrossRef CAS.
-
(a) T. Y. Jian, X. Y. Chen, L. H. Sun and S. Ye, N-heterocyclic carbene-catalyzed [4 + 2] cycloaddition of ketenes and 3-aroylcoumarins: highly enantioselective synthesis of dihydrocoumarin-fused dihydropyranones, Org. Biomol. Chem., 2013, 11, 158–163 RSC;
(b) X. Han, H. Ni, W. L. Chan, X. Gai, Y. Wang and Y. Lu, Highly enantioselective synthesis of dihydrocoumarin-fused dihydropyrans via the phosphine-catalyzed [4 + 2] annulation of allenones with 3-aroylcoumarins, Org. Biomol. Chem., 2016, 14, 5059–5064 RSC;
(c) G. H. Chang, C. Y. Wang, G. Madhusudhan Reddy, Y. L. Tsai and W. Lin, Enantioselective Synthesis of Polysubstituted
Benzopyrano[3,4-c]pyrrolidine Frameworks via [3 + 2] Cycloaddition of Azomethine Ylides and Coumarin Derivatives, J. Org. Chem., 2016, 81, 10071–10080 CrossRef CAS PubMed;
(d) Y. Wang, Z. H. Yu, H. F. Zheng and D. Q. Shi, DABCO and Bu3P catalyzed [4 + 2] and [3 + 2] cycloadditions of 3-acyl-2H-chromen-ones and ethyl 2,3-butadienoate, Org. Biomol. Chem., 2012, 10, 7739–7746 RSC;
(e) M. Potowski, C. Golz, C. Strohmann, A. P. Antonchick and H. Waldmann, Biology-oriented synthesis of benzopyrano[3,4-c]pyrrolidines, Bioorg. Med. Chem., 2015, 23, 2895–2903 CrossRef CAS PubMed;
(f) L.-P. Fan, W.-J. Yang, D.-C. Xu, X.-S. Li and J.-W. Xie, Efficient Methods for the Synthesis of Benzopyrano[3,4-c]pyrrolidines by Catalyzed 1,3-Dipolar Cycloaddition of Azomethine Ylides with 3-Substituted Coumarins, Synth. Commun., 2011, 41, 3376–3384 CrossRef CAS.
- Y. M. Poronik and D. T. Gryko, Pentacyclic coumarin-based blue emitters - the case of bifunctional nucleophilic behavior of amidines, Chem. Commun., 2014, 50, 5688–5690 RSC.
- F. Bu, R. Duan, Y. Xie, Y. Yi, Q. Peng, R. Hu, A. Qin, Z. Zhao and B. Z. Tang, Unusual Aggregation-Induced Emission of a Coumarin Derivative as a Result of the Restriction of an Intramolecular Twisting Motion, Angew. Chem., Int. Ed., 2015, 54, 14492–14497 CrossRef CAS PubMed.
- S. J. Jin, J. M. Guo, Y. S. Zhu, Q. L. Wang and Z. W. Bu, A copper-catalyzed tandem reaction for the construction of coumarin fused 9H-pyrrolo[1,2-a]indoles, Org. Biomol. Chem., 2017, 15, 8729–8737 RSC.
- S. Chithanna and D. Y. Yang, Construction of 2-pyridones via oxidative cyclization of enamides: access to Pechmann dye derivatives, Org. Biomol. Chem., 2021, 19, 1565–1574 RSC.
-
(a) Y. Li, J. Li, X. Wu, Y. Zhou and H. Liu, Rh(III)-Catalyzed C–H Cyclization of Arylnitrones with Diazo Compounds: Access to 3-Carboxylate Substituted N-Hydroxyindoles, J. Org. Chem., 2017, 82, 8984–8994 CrossRef CAS PubMed;
(b) F. Fang, C. Zhang, C. Zhou, Y. Li, Y. Zhou and H. Liu, Rh(III)-Catalyzed C–H Activation of Benzoylacetonitriles and Tandem Cyclization with Diazo Compounds to Substituted Benzo[de]chromenes, Org. Lett., 2018, 20, 1720–1724 CrossRef CAS PubMed;
(c) Z. Shu, J. Zhou, J. Li, Y. Cheng, H. Liu, D. Wang and Y. Zhou, Rh(III)-Catalyzed Dual C–H Functionalization/Cyclization Cascade by a Removable Directing Group: A Method for Synthesis of Polycyclic Fused Pyrano[de]Isochromenes, J. Org. Chem., 2020, 85, 12097–12107 CrossRef CAS;
(d) F. Fang, S. Hu, C. Li, Q. Wang, R. Wang, X. Han, Y. Zhou and H. Liu, Catalytic System-Controlled Divergent Reaction Strategies for the Construction of Diversified Spiropyrazolone Skeletons from Pyrazolidinones and Diazopyrazolones, Angew. Chem., Int. Ed., 2021, 60, 21327–21333 CrossRef CAS;
(e) Z. Jiang, J. Zhou, H. Zhu, H. Liu and Y. Zhou, Rh(III)-Catalyzed [5 + 1] Annulation of Indole-enaminones with Diazo Compounds To Form Highly Functionalized Carbazoles, Org. Lett., 2021, 23, 4406–4410 CrossRef CAS PubMed;
(f) X. Han, F. Gao, C. Li, D. Fang, X. Xie, Y. Zhou and H. Liu, Synthesis of Highly Fused Pyrano[2,3-b]pyridines via Rh(III)-Catalyzed C-H Activation and Intramolecular Cascade Annulation under Room Temperature, J. Org. Chem., 2020, 85, 6281–6294 CrossRef CAS PubMed;
(g) J. Zhou, J. Li, Y. Li, C. Wu, G. He, Q. Yang, Y. Zhou and H. Liu, Direct Synthesis of 3-Acylindoles through Rhodium(III)-Catalyzed Annulation of N-Phenylamidines with α-Cl Ketones, Org. Lett., 2018, 20, 7645–7649 CrossRef CAS PubMed;
(h) X. Wu, B. Wang, Y. Zhou and H. Liu, Propargyl Alcohols as One-Carbon Synthons: Redox-Neutral Rhodium(III)-Catalyzed C–H Bond Activation for the Synthesis of Isoindolinones Bearing a Quaternary Carbon, Org. Lett., 2017, 19, 1294–1297 CrossRef CAS;
(i) C. Zhou, F. Fang, Y. Cheng, Y. Li, H. Liu and Y. Zhou, Rhodium(III)-Catalyzed C-H Activation of Benzoylacetonitriles and Cyclization with Sulfoxonium Ylides to Naphthols, Adv. Synth. Catal., 2018, 360, 2546–2551 CrossRef CAS;
(j) Q. Yang, C. Wu, J. Zhou, G. He, H. Liu and Y. Zhou, Highly selective C–H bond activation of N-arylbenzimidamide and divergent couplings with diazophosphonate compounds: a catalyst-controlled selective synthetic strategy for 3-phosphorylindoles and 4-phosphorylisoquinolines, Org. Chem. Front., 2019, 6, 393–398 RSC.
- L. J. Nuñez-Vergara, V. Pardo-Jiménez, C. Barrientos, C. A. Olea-Azar, P. A. Navarrete-Encina and J. A. Squella, Dihydropyridine-fused and pyridine-fused coumarins: Reduction on a glassy carbon electrode in dimethylformamide, Electrochim. Acta, 2012, 85, 336–344 CrossRef.
-
(a) L. Wan, H. Wen, Y. Li, J. Lyu, Y. Xi, T. Hoshii, J. K. Joseph, X. Wang, Y.-H. E. Loh and M. A. Erb, ENL links histone acetylation to oncogenic gene expression in acute myeloid leukaemia, Nature, 2017, 543, 265–269 CrossRef CAS;
(b) M. Moustakim, T. Christott, O. P. Monteiro, J. Bennett, C. Giroud, J. Ward, C. M. Rogers, P. Smith, I. Panagakou, L. Díaz-Sáez, S. L. Felce, V. Gamble, C. Gileadi, N. Halidi, D. Heidenreich, A. Chaikuad, S. Knapp, K. V. M. Huber, G. Farnie, J. Heer, N. Manevski, G. Poda, R. Al-Awar, D. J. Dixon, P. E. Brennan and O. Fedorov, Discovery of an MLLT1/3 YEATS Domain Chemical Probe, Angew. Chem., Int. Ed., 2018, 57, 16302–16307 CrossRef CAS.
- D. Lapointe and K. Fagnou, Overview of the Mechanistic Work on the Concerted Metallation–Deprotonation Pathway, Chem. Lett., 2010, 39, 1118–1126 CrossRef.
-
(a) X. Tan, B. Liu, X. Li, B. Li, S. Xu, H. Song and B. Wang, Rhodium-catalyzed cascade oxidative annulation leading to substituted naphtho[1,8-bc]pyrans by sequential cleavage of C(sp2)-H/C(sp3)-H and C(sp2)-H/O-H bonds, J. Am. Chem. Soc., 2012, 134, 16163–16166 CrossRef CAS PubMed;
(b) Y. J. Wang, T. T. Wang, L. Yao, Q. L. Wang and L. M. Zhao, Access to 4-Alkenylated Coumarins via Ruthenium-Catalyzed Olefinic C-H Alkenylation of Coumarins with Modifiable and Removable Directing Groups, J. Org. Chem., 2020, 85, 9514–9524 CrossRef CAS PubMed;
(c) M. Shankar, K. Ghosh, K. Mukherjee, R. K. Rit and A. K. Sahoo, One-Pot Unsymmetrical {[4 + 2] and [4 + 2]} Double Annulations of o/o’-C-H Bonds of Arenes: Access to Unusual Pyranoisoquinolines, Org. Lett., 2018, 20, 5144–5148 CrossRef CAS PubMed;
(d) N. González-Gallardo, B. Saavedra, G. Guillena and D. Ramón, A jackpot C–H activation protocol using simple ruthenium catalyst in deep eutectic solvents, Green Chem., 2022, 24, 4941–4951 RSC.
|
This journal is © the Partner Organisations 2023 |
Click here to see how this site uses Cookies. View our privacy policy here.