DOI:
10.1039/D3QI01537H
(Research Article)
Inorg. Chem. Front., 2023,
10, 6401-6406
Manipulating pre-equilibria in olefin polymerization catalysis: backbone-stiffening converts a living into a highly active salan-type catalyst†
Received
4th August 2023
, Accepted 21st September 2023
First published on 22nd September 2023
Abstract
Stiffening of the catalyst backbone of salan-type catalyst 1via ring closure yields indanosalan 3 and increases activity and molar mass capability by two orders of magnitude. In propene polymerization, catalyst 3 is highly isotactic selective and nearly as active as one of the most productive known salan-catalysts today (2), showing much higher molar mass capability. NMR studies provide evidence of the identity of the active metal-polymeryl species for the catalyst pair 1/3, explaining their vast activity differences: the traditional salan catalyst 1 is trapped in the inactive mer–mer configuration, while indanosalan 3 prefers the active fac–fac isomer.
Introduction
Pre-equilibria in catalysis can “make1–3 or break4–7” a catalyst. In the specific case of olefin polymerization, e.g., formation of mixed-metallic species between the active catalyst and other components of the catalytic pool can slow the catalyst down.4,8–13 Another prominent example is the formation of more stable but catalytically inactive isomers.5,6,14 Post-metallocene catalysts of the bis(phenoxy-amine) family (“salan-type”), first introduced by Kol,15–18 offer a striking example in this respect. The inherent flexibility of the tetradentate [ONNO] ligand (L) allows it to wrap around the metal in various ways,14 leading to multiple energetically accessible isomers (fac–fac, fac–mer, mer–mer, named after the fac or mer geometries of the two [ONN] fragments, see Fig. 1).
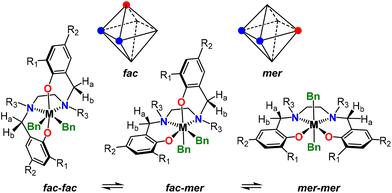 |
| Fig. 1 Illustration of the fac and mer isomerism for octahedral compounds (top) and of the possible isomers for salan precatalyst (bottom). | |
The C2-symmetric fac–fac isomer is generally favored in neutral precatalysts LMR2 of group 4 metals (R = Bn, Me2CHO).15,19 Contrarily, the mer–mer isomer is often the most stable isomer for pentacoordinated active cations adopting a distorted square pyramidal geometry as demonstrated by solution NMR spectroscopy and DFT.5,6,20
Since after activation the coordination vacancy is trans to the R group in the cationic mer–mer isomer, these species are polymerization inactive. For catalysts preferring the mer–mer isomer, isomerization to the fac–fac isomer is required prior to olefin insertion (ΔGiso > 0) and passes through the fac–mer isomer.5,6 This pre-equilibrium is integral part of the propagation barrier (Fig. 2) and responsible for the often at best sluggish activity of salan catalysts with α-olefins.15,19
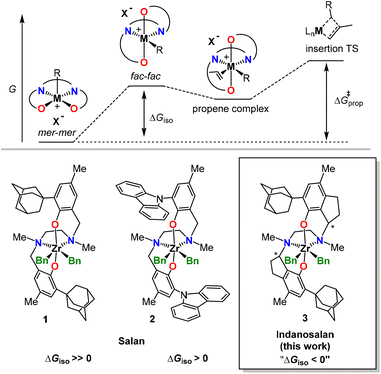 |
| Fig. 2 Top: Idealized energetic profile of propene insertion, starting from the mer–mer isomer (ΔGiso = free energy difference between fac–fac and mer–mer isomers; ΔG‡prop = propagation barrier). Bottom: salan complexes 1 (slow catalyst) and 2 (fast catalyst) with flexible catalyst backbone and novel indanosalan 3 with a rigid skeleton. | |
Phenolate ring substituents (Fig. 1) strongly influence catalyst performance in propene polymerization: (a) both R1 and R2 can affect activity and regioselectivity through electronic effects,19 (b) R1 influences stereo- and regioselectivity via steric effects,15,19 and (c) R1 substituents can also alter the fac–fac/mer–mer equilibrium via sterics.6 The non-obvious coupling of the latter two effects complicates rational tuning. On the other hand, we have recently shown that limiting catalyst flexibility can dramatically increase catalyst performance,21 even in systems as well studied as ansa-metallocenes.22,23
Employing a conceptually similar approach, we reasoned that locking salan-type catalysts in the polymerization active fac–fac isomer through stiffening of the catalyst backbone should improve their activity. In particular, the interconversion of the mer–mer into the fac–fac isomer or vice versa requires a rotation around the carbon–carbon bond between the methylene spacer and the phenyl ring (Fig. 1 and ESI†), which can be “easily” blocked via ring-closure.
As proof of principle, we designed and synthesized a novel indanosalan catalyst bearing an ortho-1-adamantyl substituent in position R1 (3, Fig. 2). We chose the ortho-1-adamantyl substituted salan,19 because catalyst 1 in Fig. 2 is highly isotactic selective in propene polymerization showing low activity, to the point that was used to synthesize well-defined iPP-block-PE copolymers.24
Results and discussion
Indanosalan 3 has four stereocenters (two chiral N and two chiral C atoms). DFT calculations indicate that for a given rac-isomer with respect to the two chiral carbon atoms, mer–mer and fac–fac isomers of 3 differ in the chirality of the N-bridge atoms; direct isomerization with retention of chirality at N is impossible, as ring closure blocks rotations and we could not locate such an isomer employing Grimme's conformer–rotamer ensemble sampling tool (CREST)25 followed by subsequent full DFT optimization of identified conformers at the TPSSh-Dzero(PCM)/TZ//TPSSh/DZ level of theory.26–31 Instead, isomerization requires N-decoordination and umbrella inversion at both nitrogen atoms. As a consequence, 3 should be kinetically trapped in the fac–fac isomer, at least on the timescale of a typical polymerization.
The key step in the assembly of the indanosalan ligand 3d (Scheme 1) was alkylation of N,N′-dimethylethylenediamine with indanol 3c. Due to the presence of two stereocenters in 3d a mixture of rac- and meso-isomers of the ligand was expected. Surprisingly, a single compound precipitated from the reaction mixture in 72% yield. 1H and 13C NMR spectra agreed with the structure of the ligand but did not allow positive identification of the diastereomer. Reaction of the isolated ligand with ZrBn4 cleanly afforded a single, C1-symmetric, ZrBn2 complex. Activation with Ph3C+B(C6F5)4−/tri-iso-butyl aluminum (TTB/TiBA) in the presence of 1-hexene yielded no polymer, strongly indicating that the isolated ligand was meso-3d.
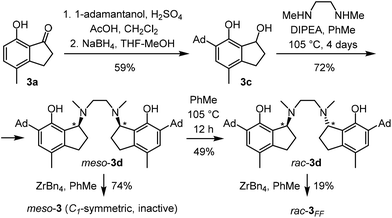 |
| Scheme 1 Synthesis of meso-3 and rac-3FF from 3a.32 | |
Selective formation of one diastereomer points to a reversible mechanism33 involving an o-quinone methide intermediate.34 Consequently, under the reaction conditions, the two diastereomers of the ligand should be in equilibrium, which is shifted by precipitation of meso-3d. Taking advantage of this reversibility, a ∼1
:
1 rac/meso-3d mixture was prepared from pure meso-3d through stirring a hot dilute solution of meso-3d in toluene overnight followed by quick cooling to room temperature to “freeze” the equilibrium. Subsequently, selective precipitation of meso-3d yielded a solution of rac-3d pure enough for metalation. Reaction of rac-3d with ZrBn4 in toluene yielded a mixture of C2- (fac–fac, rac-3FF) and C1- (presumably fac–mer, rac-3FM) isomeric complexes in 61% yield from which rac-3FF was isolated through crystallization in 19% yield. Unlike meso-3, rac-3FF polymerizes 1-hexene when activated with TTB/TiBA.
The X-ray crystal structure of rac-3FF is shown in Fig. 3. The complex adopts a slightly distorted C2-symmetric octahedral geometry. The additional ring closures introduce obvious changes in the bond angles and torsions around the two spacer carbons C3 and C23; nonetheless, those changes do not translate into significantly different parameters of the coordinational polyhedron in comparison with those in adamantyl-substituted salan complexes known in the literature,35,36 as the O–N distances remain nearly the same.
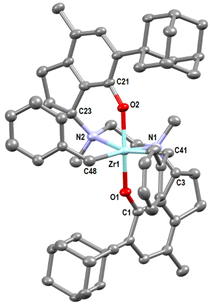 |
| Fig. 3 X-ray crystal structure of rac-3FF. Hydrogen atoms omitted for clarity; ellipsoids drawn at 50% probability level. The left Zr-benzyl moiety is disordered, one of the two local minima is shown. Selected bond lengths (Å): Zr1–C41 2.285(4), Zr1–C48 2.306(4), Zr1–O1 2.000(2), Zr1–O2 2.002(2), Zr1–N1 2.552(3), Zr1–N2 2.485(3). Selected angles (°): C41–Zr1–C48 106.20(14), N1–Zr1–N1 72.06(10), C1–O1–Zr1 146.2(2), C21–O2–Zr1 145.4(2). | |
Low-temperature NMR studies provide insight into the reaction of 1-hexene with 1-Bn+ and 3-Bn+, generated in C6D5Cl by adding one equivalent of TTB to 1 and 3.
At 233 K, 1-Bn+ is predominantly present in the form of the fac–fac isomer, as indicated by the characteristic 13C NMR chemical shift of the NMe groups (>40 ppm for both, Fig. 4a).37 Addition of ∼15 equiv. of 1-hexene resulted in the slow consumption of both 1-hexene and 1-Bn+ along with the formation of a new, relatively stable, organometallic species. Given the known living nature of this catalyst, we formulate it as 1-Pn+. The majority of 1-Pn+ accumulates in the form of the inactive mer–mer isomer as evidenced by 13C NMR chemical shifts of the NMe groups at 37.6 and 42.6 ppm (Fig. 4b).37
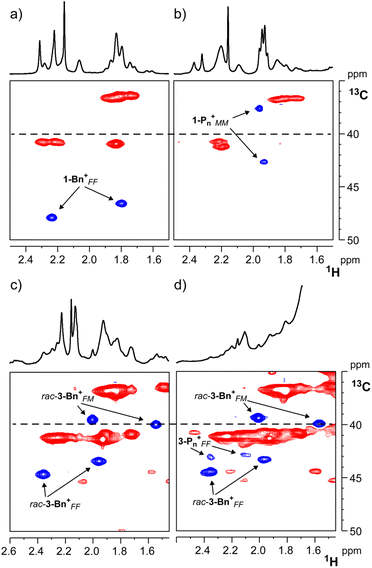 |
| Fig. 4 Sections of four multiplicity-edited 1H,13C HSQC NMR experiments (blue cross peaks = CH or CH3 moieties) relative to the NMe region and showing: (a) the fac–fac isomer of 1-Bn+; (b) formation of the mer–mer isomer of 1-Pn+ after the addition of 15 equiv. of 1-hexene to 1-Bn+; (c) the mixture of fac–fac and fac–mer isomers of 3-Bn+ derived from a 3 : 2 mixture of rac-3FM/rac-3FF; (d) formation of the fac–fac isomer of 3-Pn+ after the addition of 460 equiv. of 1-hexene to 3-Bn+. All experiments were carried out in C6D5Cl at 233 K. | |
The possibility to prepare the rac-3 metal complex as a mixture of both fac–fac and fac–mer isomers offered the unique opportunity to study their chemical equilibria. Upon activation of a 3
:
2 mixture of rac-3FM/rac-3FF, the 13C NMR spectrum shows a 2
:
1 mixture of the fac–fac and fac–mer isomers of 3-Bn+ (δC = 43.4 and 44.6 ppm for the fac–fac, 39.5 and 39.9 ppm for the fac–mer, respectively; Fig. 4c). Chemical exchange between fac–fac and fac–mer isomers of 3-Bn+ is slow on the NMR time scale, indicating substantial barriers for isomerization as pointed out by DFT calculations (vide supra). The rate of site epimerization at the fac–fac isomer is not dissimilar to that of 1-Bn+ under similar conditions: kSE = 0.6 s−1 at 223 K and 0.4 s−1 at 228 K, for 3FF-Bn+ and 1-Bn+, respectively. Addition of ∼15 equiv. of 1-hexene to the mixture leads to immediate formation of poly-1-hexene, without apparent consumption of either 3-Bn+ species. However, addition of a larger excess of 1-hexene (∼460 equiv.) resulted in a substantial consumption of 3FF-Bn+ but not 3FM-Bn+, indicating the latter is inactive (or much less active). In turn, 3FF-Bn+ displays the typical behavior of a very active olefin polymerization catalyst, i.e., the first olefin insertion is much slower than subsequent insertions.38
A prolonged 1H,13C HSQC NMR experiment (Fig. 4d), lasting several hours from the addition of 1-hexene, allowed detection of two new 13C NMR resonances in the region of the NMe groups, tentatively assigned to a 3-Pn+FF species. While complete identification of this species is hampered by the poly-1-hexene signals, the NMe chemical shifts (δC > 40 ppm) clearly indicate that the active species, differently from 1-Pn+, retains fac–fac geometry.
The novel indanosalan catalyst rac-3FF, the parent salan 1 and the ortho-N-carbazolyl salan 2, which is one of the most active salan [ONNO]Zr propene polymerization catalysts presently known and mildly isotactic selective,6,39 have been tested in propene polymerization at 60 °C using MAO/BHT8,40–44 as activator (Table 1) in a high-throughput experimentation platform (Freeslate parallel pressure reactor, PPR).45–49 The polymerization procedure is reported in the ESI† and described in more detail in ref. 22, 23 and 50.
Table 1 Propene polymerization results (averaged on at least triplicate experiments) using 1, 2 and rac-3FF at Tp 60 °C and ppropene = 6.6 bar
Precatalyst |
R
p a |
σ
|
[2,1] % |
M
n, kDa |
M
w, kDa |
PDI |
In kgPP mmolZr−1 h−1.
Probability to select the preferred monomer enantioface at an active site of given chirality.
|
1
|
0.19 |
0.998 |
0.52 |
7.6 |
14 |
1.8 |
2
|
17 |
0.82 |
0.80 |
1.3 |
2.0 |
1.5 |
rac
-
3
FF
|
38 |
0.994 |
0.53 |
113 |
248 |
2.2 |
Inspection of Table 1 reveals that the ligand modification, leading to the novel precatalyst rac-3FF, yields a dramatic enhancement of catalyst productivity (Rp), exceeding its homologue (1) by over two orders of magnitude and even surpassing the performance of 2. Strikingly, the catalyst derived from rac-3FF also shows a much higher polymer molar mass capability compared with both 1 and 2 (Table 1). This indicates that the 3-Pn+FF active species (Pn = growing polymeryl) features a higher ratio between the apparent rates of chain propagation and chain transfer than both 1-Pn+ and 2-Pn+. Neither stereo- nor regioselectivity are affected by the ligand modification and no indications for the loss of C2-symmetry under polymerization conditions are observed, indicating that the catalyst is stable under polymerization conditions.
Conclusions
rac-3FF precatalyst has been designed and synthetized ad hoc to hamper, during propene polymerization, the fac–fac/mer–mer isomerization, responsible for the poor activity of most salan-type catalysts. Gratifyingly, rac-3FF, upon activation with MAO/BHT, polymerizes propene with a productivity that exceeds one of the most active salan-type catalysts (2) and with almost two orders of magnitude higher molecular mass capability.
Data availability
Crystallographic data has been deposited at the CCDC with number 2283479.† Synthetic procedures and characterization, detailed NMR spectra, polymerization procedures, polymer characterization procedures, and polymer analytical characterizations, computational details for conformer sampling are reported in the ESI.†
Author contributions
The manuscript was written through contributions of all authors. All authors have given approval to the final version of the manuscript.
Conflicts of interest
There are no conflicts to declare.
Acknowledgements
The work of ADA, CZ, AV, CE, RC, AM and VB forms part of the research programme of DPI, project #835. The authors wish to express their gratitude to P. H. M. Budzelaar for helpful discussions. AM and CZ also thank the European Union – NextGenerationEU under the Italian Ministry of University and Research (MUR) National Innovation Ecosystem grant ECS00000041 – VITALITY for supporting this work. AM and CZ also acknowledge Università degli Studi di Perugia and MUR for support within the project Vitality. DVU, MIS, GPG, KML, and AZV thank Ministry of Science and Higher Education for support of their work (project #121021000105-7) and Lomonosov Moscow State University Program of Development for opportunity to use NMR, X-ray, and chromatographic equipment. DVU and GPG also thank Konstantin A. Lyssenko for help with the X-ray measurements and refinements.
References
- C. Ehm, J. Krüger and D. Lentz, How a Thermally Unstable Metal Hydrido Complex Can Yield High Catalytic Activity Even at Elevated Temperatures, Chem. – Eur. J., 2016, 22, 9305–9310 CrossRef CAS PubMed.
- T. Louis-Goff, H. V. Trinh, E. Chen, A. L. Rheingold, C. Ehm and J. Hyvl, Stabilizing Effect of Pre-equilibria: A Trifluoromethyl Complex as a CF2 Reservoir in Catalytic Olefin Difluorocarbenation, ACS Catal., 2022, 12, 3719–3730 CrossRef CAS.
- D. Y. Curtin and M. C. Crew, Rearrangement with Nitrous Acid of the Diastereoisomeric 1-p-Anisyl-1-phenyl-2-aminopropanols. The cis Effect of Methyl and Phenyl Groups1, J. Am. Chem. Soc., 1955, 77, 354–357 CrossRef CAS.
- V. Busico, R. Cipullo, R. Pellecchia, G. Talarico and A. Razavi, Hafnocenes and MAO: Beware of Trimethylaluminum!, Macromolecules, 2009, 42, 1789–1791 CrossRef CAS.
- G. Ciancaleoni, N. Fraldi, P. H. M. Budzelaar, V. Busico and A. Macchioni, Structure and Dynamics in Solution of Bis(phenoxy-amine)Zirconium Catalysts for Olefin Polymerization, Organometallics, 2011, 30, 3096–3105 CrossRef CAS.
- G. Ciancaleoni, N. Fraldi, R. Cipullo, V. Busico, A. Macchioni and P. H. M. Budzelaar, Structure/Properties Relationship for Bis(phenoxyamine)Zr(IV)-Based Olefin Polymerization Catalysts: A Simple DFT Model To Predict Catalytic Activity, Macromolecules, 2012, 45, 4046–4053 CrossRef CAS.
- L. Rocchigiani, V. Busico, A. Pastore and A. Macchioni, Probing the interactions between all components of the catalytic pool for homogeneous olefin polymerisation by diffusion NMR spectroscopy, Dalton Trans., 2013, 42, 9104–9111 RSC.
- V. Busico, R. Cipullo, F. Cutillo, N. Friederichs, S. Ronca and B. Wang, Improving the Performance of Methylalumoxane: A Facile and Efficient Method to Trap “Free” Trimethylaluminum, J. Am. Chem. Soc., 2003, 125, 12402–12403 CrossRef CAS PubMed.
- L. Rocchigiani, V. Busico, A. Pastore, G. Talarico and A. Macchioni, Unusual Hafnium–Pyridylamido/ERn Heterobimetallic Adducts (ERn=ZnR2 or AlR3), Angew. Chem., Int. Ed., 2014, 53, 2157–2161 CrossRef CAS PubMed.
- C. Ehm, R. Cipullo, P. H. M. Budzelaar and V. Busico, Role(s) of TMA in polymerization, Dalton Trans., 2016, 45, 6847–6855 RSC.
- M. Bochmann, The Chemistry of Catalyst Activation: The Case of Group 4 Polymerization Catalysts, Organometallics, 2010, 29, 4711–4740 CrossRef CAS.
- F. Ghiotto, C. Pateraki, J. R. Severn, N. Friederichs and M. Bochmann, Rapid evaluation of catalysts and MAO activators by kinetics: what controls polymer molecular weight and activity in metallocene/MAO catalysts?, Dalton Trans., 2013, 42, 9040–9048 RSC.
- K. P. Bryliakov, E. P. Talsi, A. Z. Voskoboynikov, S. J. Lancaster and M. Bochmann, Formation and Structures of Hafnocene Complexes in MAO- and AlBui3/CPh3[B(C6F5)4]-Activated Systems, Organometallics, 2008, 27, 6333–6342 CrossRef CAS.
- G. Ciancaleoni, N. Fraldi, P. H. M. Budzelaar, V. Busico and A. Macchioni, Activation of a bis(phenoxy-amine) precatalyst for olefin polymerisation: first evidence for an outer sphere ion pair with the methylborate counterion, Dalton Trans., 2009, 8824–8827, 10.1039/B908805A.
- E. Y. Tshuva, I. Goldberg and M. Kol, Isospecific Living Polymerization of 1-Hexene by a Readily Available NonmetalloceneC2-Symmetrical Zirconium Catalyst, J. Am. Chem. Soc., 2000, 122, 10706–10707 CrossRef CAS.
- A. Cohen, J. Kopilov, M. Lamberti, V. Venditto and M. Kol, Same Ligand, Different Metals: Diiodo−Salan Complexes of the Group 4 Triad in Isospecific Polymerization of 1-Hexene and Propylene, Macromolecules, 2010, 43, 1689–1691 CrossRef CAS.
- S. Segal, I. Goldberg and M. Kol, Zirconium and Titanium Diamine Bis(phenolate) Catalysts for α-Olefin Polymerization: From Atactic Oligo(1-hexene) to Ultrahigh-Molecular-Weight Isotactic Poly(1-hexene), Organometallics, 2005, 24, 200–202 CrossRef CAS.
- A. Yeori, S. Groysman, I. Goldberg and M. Kol, Diastereoisomerically Selective Enantiomerically Pure Titanium Complexes of Salan Ligands: Synthesis, Structure, and Preliminary Activity Studies, Inorg. Chem., 2005, 44, 4466–4468 CrossRef CAS PubMed.
- V. Busico, R. Cipullo, R. Pellecchia, S. Ronca, G. Roviello and G. Talarico, Design of stereoselective Ziegler–Natta propene polymerization catalysts, Proc. Natl. Acad. Sci. U. S. A., 2006, 103, 15321–15326 CrossRef CAS PubMed.
- C. Zuccaccia, V. Busico, R. Cipullo, G. Talarico, R. D. J. Froese, P. C. Vosejpka, P. D. Hustad and A. Macchioni, On the First Insertion of α-Olefins in Hafnium Pyridyl-Amido Polymerization Catalysts, Organometallics, 2009, 28, 5445–5458 CrossRef CAS.
- G. P. Goryunov, M. I. Sharikov, A. N. Iashin, J. A. M. Canich, S. J. Mattler, J. R. Hagadorn, D. V. Uborsky and A. Z. Voskoboynikov, Rigid Postmetallocene Catalysts for Propylene Polymerization: Ligand Design Prevents the Temperature-Dependent Loss of Stereo- and Regioselectivities, ACS Catal., 2021, 11, 8079–8086 CrossRef CAS.
- C. Ehm, A. Vittoria, G. P. Goryunov, V. V. Izmer, D. S. Kononovich, P. S. Kulyabin, R. Di Girolamo, P. H. M. Budzelaar, A. Z. Voskoboynikov, V. Busico, D. V. Uborsky and R. Cipullo, A Systematic Study of the Temperature-Induced Performance Decline of ansa-Metallocenes for iPP, Macromolecules, 2020, 53, 9325–9336 CrossRef CAS.
- P. S. Kulyabin, G. P. Goryunov, M. I. Sharikov, V. V. Izmer, A. Vittoria, P. H. M. Budzelaar, V. Busico, A. Z. Voskoboynikov, C. Ehm, R. Cipullo and D. V. Uborsky, ansa-Zirconocene Catalysts for Isotactic-Selective Propene Polymerization at High Temperature: A Long Story Finds a Happy Ending, J. Am. Chem. Soc., 2021, 143, 7641–7647 CrossRef CAS PubMed.
- V. Busico, R. Cipullo, N. Friederichs, S. Ronca, G. Talarico, M. Togrou and B. Wang, Block Copolymers of Highly Isotactic Polypropylene via Controlled Ziegler−Natta Polymerization, Macromolecules, 2004, 37, 8201–8203 CrossRef CAS.
- P. Pracht, F. Bohle and S. Grimme, Automated exploration of the low-energy chemical space with fast quantum chemical methods, Phys. Chem. Chem. Phys., 2020, 22, 7169–7192 RSC.
-
M. J. Frisch, G. W. Trucks, H. B. Schlegel, G. E. Scuseria, M. A. Robb, J. R. Cheeseman, G. Scalmani, V. Barone, G. A. Petersson, H. Nakatsuji, X. Li, M. Caricato, A. V. Marenich, J. Bloino, B. G. Janesko, R. Gomperts, B. Mennucci, H. P. Hratchian, J. V. Ortiz, A. F. Izmaylov, J. L. Sonnenberg, D. Williams-Young, F. Ding, F. Lipparini, F. Egidi, J. Goings, B. Peng, A. Petrone, T. Henderson, D. Ranasinghe, V. G. Zakrzewski, J. Gao, N. Rega, G. Zheng, W. Liang, M. Hada, M. Ehara, K. Toyota, R. Fukuda, J. Hasegawa, M. Ishida, T. Nakajima, Y. Honda, O. Kitao, H. Nakai, T. Vreven, K. Throssell, J. A. Montgomery, Jr., J. E. Peralta, F. Ogliaro, M. J. Bearpark, J. J. Heyd, E. N. Brothers, K. N. Kudin, V. N. Staroverov, T. A. Keith, R. Kobayashi, J. Normand, K. Raghavachari, A. P. Rendell, J. C. Burant, S. S. Iyengar, J. Tomasi, M. Cossi, J. M. Millam, M. Klene, C. Adamo, R. Cammi, J. W. Ochterski, R. L. Martin, K. Morokuma, O. Farkas, J. B. Foresman and D. J. Fox, Gaussian 16, revision A.03, Gaussian, Inc., Wallingford, CT, 2016 Search PubMed.
- J. M. Tao, J. P. Perdew, V. N. Staroverov and G. E. Scuseria, Climbing the density functional ladder: Nonempirical meta-generalized gradient approximation designed for molecules and solids, Phys. Rev. Lett., 2003, 91, 146401 CrossRef PubMed.
- B. P. Pritchard, D. Altarawy, B. Didier, T. D. Gibson and T. L. Windus, New Basis Set Exchange: An Open, Up-to-Date Resource for the Molecular Sciences Community, J. Chem. Inf. Model., 2019, 59, 4814–4820 CrossRef CAS PubMed.
- P. Schwerdtfeger, The Pseudopotential Approximation in Electronic Structure Theory, ChemPhysChem, 2011, 12, 3143–3155 CrossRef CAS PubMed.
- J. Tomasi, B. Mennucci and R. Cammi, Quantum Mechanical Continuum Solvation Models, Chem. Rev., 2005, 105, 2999–3094 CrossRef CAS PubMed.
- K. A. Peterson, D. Figgen, M. Dolg and H. Stoll, Energy-consistent relativistic pseudopotentials and correlation consistent basis sets for the 4d elements Y–Pd, J. Chem. Phys., 2007, 126, 124101 CrossRef PubMed.
- Y.-S. Huang, J.-Q. Liu, L.-J. Zhang and H.-L. Lu, Synthesis of 1-Indanones from Benzoic Acids, Ind. Eng. Chem. Res., 2012, 51, 1105–1109 CrossRef CAS.
- P. C. B. Page, H. Heaney, M. J. McGrath, E. P. Sampler and R. F. Wilkins, Retro-Mannich reactions of 3-alkyl-3,4-dihydro-2H-1,3-benz[e]oxazines and the synthesis of axially chiral resorcinarenes, Tetrahedron Lett., 2003, 44, 2965–2970 CrossRef CAS.
- R. W. Van De Water and T. R. R. Pettus, o-Quinone methides: intermediates underdeveloped and underutilized in organic synthesis, Tetrahedron, 2002, 58, 5367–5405 CrossRef CAS.
- A. Yeori, I. Goldberg, M. Shuster and M. Kol, Diastereomerically-Specific Zirconium Complexes of Chiral Salan Ligands: Isospecific Polymerization of 1-Hexene and 4-Methyl-1-pentene and Cyclopolymerization of 1,5-Hexadiene, J. Am. Chem. Soc., 2006, 128, 13062–13063 CrossRef CAS PubMed.
- A. Yeori, I. Goldberg and M. Kol, Cyclopolymerization of 1,5-Hexadiene by Enantiomerically-Pure Zirconium Salan Complexes. Polymer Optical Activity Reveals α-Olefin Face Preference, Macromolecules, 2007, 40, 8521–8523 CrossRef CAS.
- A. Dall’Anese, P. S. Kulyabin, D. V. Uborsky, A. Vittoria, C. Ehm, R. Cipullo, P. H. M. Budzelaar, A. Z. Voskoboynikov, V. Busico, L. Tensi, A. Macchioni and C. Zuccaccia, Inorg. Chem., 2023 DOI:10.1021/acs.inorgchem.3c02153.
- Z. Liu, E. Somsook, C. B. White, K. A. Rosaaen and C. R. Landis, Kinetics of Initiation, Propagation, and Termination for the [rac-(C2H4(1-indenyl)2)ZrMe][MeB(C6F5)3]-Catalyzed Polymerization of 1-Hexene, J. Am. Chem. Soc., 2001, 123, 11193–11207 CrossRef CAS PubMed.
- G. Antinucci, B. Dereli, A. Vittoria, P. H. M. Budzelaar, R. Cipullo, G. P. Goryunov, P. S. Kulyabin, D. V. Uborsky, L. Cavallo, C. Ehm, A. Z. Voskoboynikov and V. Busico, Selection of Low-Dimensional 3-D Geometric Descriptors for Accurate Enantioselectivity Prediction, ACS Catal., 2022, 12, 6934–6945 CrossRef CAS.
- F. Zaccaria, C. Zuccaccia, R. Cipullo, P. H. M. Budzelaar, A. Macchioni, V. Busico and C. Ehm, BHT-Modified MAO: Cage Size Estimation, Chemical Counting of Strongly Acidic Al Sites, and Activation of a Ti-Phosphinimide Precatalyst, ACS Catal., 2019, 9, 2996–3010 CrossRef CAS.
- F. Zaccaria, P. H. M. Budzelaar, R. Cipullo, C. Zuccaccia, A. Macchioni, V. Busico and C. Ehm, Reactivity Trends of Lewis Acidic Sites in Methylaluminoxane and Some of Its Modifications, Inorg. Chem., 2020, 59, 5751–5759 CrossRef CAS PubMed.
- F. Zaccaria, C. Zuccaccia, R. Cipullo, P. H. M. Budzelaar, A. Macchioni, V. Busico and C. Ehm, On the Nature of the Lewis Acidic Sites in “TMA-Free” Phenol-Modified Methylaluminoxane, Eur. J. Inorg. Chem., 2020, 2020, 1088–1095 CrossRef CAS.
- J.-N. Pédeutour, K. Radhakrishnan, H. Cramail and A. Deffieux, Use of “TMA-depleted” MAO for the activation of zirconocenes in olefin polymerization, J. Mol. Catal. A: Chem., 2002, 185, 119–125 CrossRef.
- R. A. Stapleton, B. R. Galan, S. Collins, R. S. Simons, J. C. Garrison and W. J. Youngs, Bulky Aluminum Alkyl Scavengers in Olefin Polymerization with Group 4 Catalysts, J. Am. Chem. Soc., 2003, 125, 9246–9247 CrossRef CAS PubMed.
- V. Murphy, X. Bei, T. R. Boussie, O. Brümmer, G. M. Diamond, C. Goh, K. A. Hall, A. M. Lapointe, M. Leclerc, J. M. Longmire, J. A. W. Shoemaker, H. Turner and W. H. Weinberg, High-Throughput Approaches for the Discovery and Optimization of New Olefin Polymerization Catalysts, Chem. Rec., 2002, 2, 278–289 CrossRef CAS PubMed.
- T. R. Boussie, G. M. Diamond, C. Goh, K. A. Hall, A. M. LaPointe, M. Leclerc, C. Lund, V. Murphy, J. A. W. Shoemaker, U. Tracht, H. Turner, J. Zhang, T. Uno, R. K. Rosen and J. C. Stevens, A Fully Integrated High-Throughput Screening Methodology for the Discovery of New Polyolefin Catalysts: Discovery of a New Class of High Temperature Single-Site Group (IV) Copolymerization Catalysts, J. Am. Chem. Soc., 2003, 125, 4306–4317 CrossRef CAS PubMed.
- Y. L. Dar, High-Throughput Experimentation: A Powerful Enabling Technology for the Chemicals and Materials Industry, Macromol. Rapid Commun., 2004, 25, 34–47 CrossRef CAS.
- T. R. Boussie, G. M. Diamond, C. Goh, K. A. Hall, A. M. LaPointe, M. K. Leclerc, V. Murphy, J. A. W. Shoemaker, H. Turner, R. K. Rosen, J. C. Stevens, F. Alfano, V. Busico, R. Cipullo and G. Talarico, Nonconventional Catalysts for Isotactic Propene Polymerization in Solution Developed by Using High-Throughput-Screening Technologies, Angew. Chem., Int. Ed., 2006, 45, 3278–3283 CrossRef CAS PubMed.
- V. Busico, R. Pellecchia, F. Cutillo and R. Cipullo, High-Throughput Screening in Olefin-Polymerization Catalysis: From Serendipitous Discovery Towards Rational Understanding, Macromol. Rapid Commun., 2009, 30, 1697–1708 CrossRef CAS PubMed.
- C. Ehm, A. Mingione, A. Vittoria, F. Zaccaria, R. Cipullo and V. Busico, High-Throughput Experimentation in Olefin Polymerization Catalysis: Facing the Challenges of Miniaturization, Ind. Eng. Chem. Res., 2020, 59, 13940–13947 CrossRef CAS.
Footnote |
† Electronic supplementary information (ESI) available: Synthetic procedures and characterization, detailed NMR spectra, polymerization procedures, polymer characterization procedures, and polymer analytical characterizations, computational details for conformer sampling (PDF). Coordinates for DFT structures (XYZ). CCDC 2283479. For ESI and crystallographic data in CIF or other electronic format see DOI: https://doi.org/10.1039/d3qi01537h |
|
This journal is © the Partner Organisations 2023 |
Click here to see how this site uses Cookies. View our privacy policy here.