Diyne-steered switchable regioselectivity in cobalt(II)-catalysed C(sp2)–H activation of amides with unsymmetrical 1,3-diynes†
Received
1st December 2022
, Accepted 17th January 2023
First published on 8th February 2023
Abstract
The regiochemical outcome of a cobalt(II) catalysed C–H activation reaction of aminoquinoline benzamides with unsymmetrical 1,3-diynes under relatively mild reaction conditions can be steered through the choice of diyne. The choice of diyne provides access to either 3- or 4-hydroxyalkyl isoquinolinones, paving the way for the synthesis of more highly elaborate isoquinolines.
Introduction
Transition metal catalysed C–H bond activation is an important method for the synthesis of a broad range of bioactive molecules and materials without the need for prefunctionalization, thus minimising waste and enhancing atom economy.1–5 Traditionally, despite their low abundance and toxicity, transition metals such as palladium,6 rhodium,7–9 ruthenium,10–12 and iridium13 have been the catalysts of choice for efficient C–H bond activation.14 However, the past decade has witnessed a shift towards more abundant and comparatively less toxic base metal catalysts.14 In this context, cobalt has been of significant interest due to its high relative abundance and the unique catalytic activities provided by this element's three oxidation states.15–18 Very recently, Niu and co-workers developed a Co(II)/organic oxidant catalytic system for the selective activation of 1°, 2°, and 3° C-(sp3)–H bonds for constructing C–N and C–O bonds.19 Knochel and co-workers synthesised organozinc pivalates using cobalt-catalysed cross-coupling reactions.20 Numerous elegant examples of cobalt-catalysed C–H activations have been reported by Daugulis,21–23 Glorius,24–27 Ackermann,26,27 and others.28–30
Although cobalt catalysts have made available a variety of new disconnections, the regioselective synthesis of heterocycles using an unsymmetrical coupling partner remains unrealised. In addition, the basis for regiochemical preferences associated with 1,3-diyne-based annulations has not yet been investigated.
Many isoquinolones are highly bioactive molecules and their synthesis through C–H activation has been realised with various alkynes.31–34 C–H activation strategies employing 1,3-diyne addition have become important methods to synthesise this important heterocyclic moiety. For example, Miura first achieved the synthesis of isoquinolones by C–H activation using Rh(III) catalysts.35 Glorius developed a novel route for the synthesis of conjugated isoquinolones and bis-heterocycles through double C–H activation.36 Later, we developed a cobalt catalysed C–H activation of benzamides with 1,3-diynes using aminoquinoline as a chelating group (Fig. 1a).37 After these initial developments, research teams have disclosed a range of interesting examples with symmetrical 1,3-diynes (Scheme 1a).38–42 Nonetheless, the use of unsymmetrical 1,3-diynes has been limited. This may be due to the inherent possibility of metalloalkynylation through two similar sites, leading to several possible regioisomers (Fig. 1b). For example, Jeganmohan and co-workers have reported that four different unsymmetrical 1,3-diynes when used in a cobalt(III) catalysed regioselective (4 + 2) annulation of sulfoxonium ylides provided two regioisomeric products.43,45 A Rh(III) catalysed (3 + 2) spirocyclisation of 2H-imidazoles with unsymmetrical 1,3-diynes was also reported by the Dong group.44 In this case, the regioselectivity was comparable to that of our cobalt system.37 Given the potential regiochemical promiscuity available when using unsymmetrical 1,3-diynes, we envisaged that the nature of the 1,3-diyne could have an impact on the preferred outcome of the reaction. Herein, we report our findings on the cobalt(II) catalysed C–H activation of aminoquinoline benzamides with unsymmetrical 1,3-diynes (Fig. 1c).
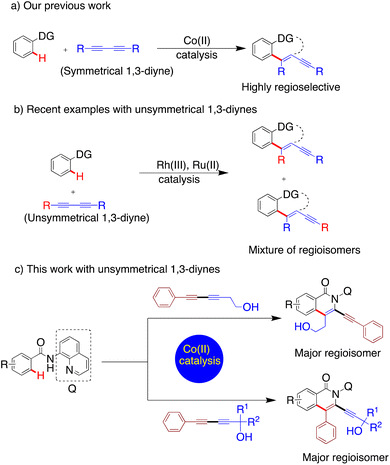 |
| Fig. 1 Regioselective C–H activation. | |
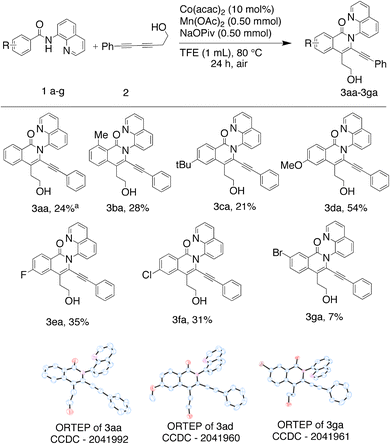 |
| Scheme 1 Substrate scope with 1,3-diyne 2 (ain situ yields were calculated by HPLC). | |
Results and discussion
Reactions were performed using 2.0 equiv. of benzamide (1a–g) and 1.0 equiv. of unsymmetrical 1,3-diyne (2–5) as the starting substrates for the C–H annulation reaction using 10 mol% of cobalt(II)acetylacetonate, 2.0 equiv. of manganese(II)acetate as the external oxidant, 2.0 equiv. of sodium trimethylacetate hydrate as the base and 2,2,2-trifluoroethanol (TFE) as the solvent. These conditions were selected to provide moderate yields of the major regioisomer, so that the eventual influence on yields due to variations in the amides and diynes used could be observed.
In the case of unsubstituted and o- and p-substituted amides, four modes of insertions are possible, leading to four possible regioisomeric isoquinolone products and eight possible products when the amide bears an m-substituent. The reactions were carried out under air, at 80 °C for 24 h. In each case, the major regioisomer was isolated and purified by flash column or preparative thin layer chromatography and 2D NMR elucidated structures. 2D NMR data were further confirmed by XRD, where possible. An HPLC-assay was developed and used to determine the in situ yields of the major regioisomers from each of the C–H activation reactions, i.e. the yield as determined from the reaction vessel prior to work-up and purification. The HPLC-assay also revealed the presence of unreacted starting materials and minor isomers, and the latter, in some cases, could be isolated and characterized (Fig. 2).
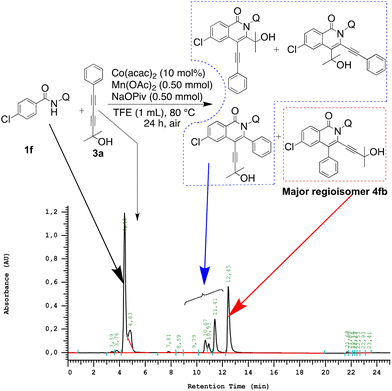 |
| Fig. 2 HPLC trace of the crude reaction mixture of 1f with 3, with unreacted starting materials, isolated major regioisomer and peaks arising from the expected non-isolated minor regioisomers marked. | |
Initial efforts employed a series of benzamides (1a–g) and an unsymmetrical diyne (2) with a polar hydroxyl functionality and less steric bulk than in the apolar symmetrical diynes used in previous studies. The corresponding products displayed the same preferred geometry of insertion of the unsymmetrical diyne as that reported in our earlier work using symmetrical 1,3-diynes,37 namely with the resulting phenyl bearing alkynyl substituent ortho to the isoquinolone nitrogen (Scheme 1), with the hydroxyalkyl bearing alkyne being the preferred insertion partner. The reaction was generally tolerant toward a range of benzamide substituents. Of note was the significantly better yield associated with the strongly electron donating p-methoxy (3da), relative to the unsubstituted case (3aa), and the low yield of the major regioisomer from the reaction performed using m-bromobenzamide (1g), where the low yield was attributed to a combination of the competition between eight insertion modes and the steric hindrance afforded by the bromo-substituent.
We then deployed an isopropyl alcohol-substituted diyne (3), a slightly bulkier and shorter diyne (containing one additional carbon and a quaternary carbon, as compared to 2), with the same series of benzamides (1a–g) (Scheme 2). Again, all the major regioisomers displayed the alkynyl substituent ortho to the isoquinolone nitrogen; however, and to our surprise, the orientation of insertion in all major products was reversed with respect to the orientation of the diyne-substituents obtained when using 2. Moreover, the yield of the unsubstituted benzamide product was notably higher compared to its reaction with diyne 2. Again, the strongly electron-donating p-methoxy substituent produced the highest yield, and even the yield of the reaction with m-bromobenzamide improved. The reactivity of two substantially more sterically demanding diynes (4 and 5) was then explored, with the major regioisomers again demonstrating the reversal of orientation of insertion, though with lower yields, possibly reflecting the impact of the increased bulk of the branched hydroxyl bearing substituents on the reaction energetics of metallocycle formation.
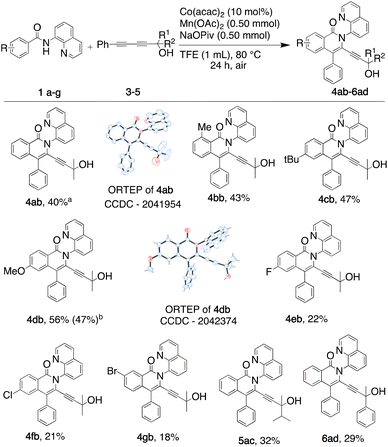 |
| Scheme 2 Substrate scope with 1,3-diynes 3–5 (ain situ yields were calculated by HPLC, bisolated yield). | |
When some reactions were performed using longer reaction times and higher temperatures (Scheme 3), the regiochemistries of the dominant regioisomers and their yields remained essentially the same, although the yields of three of the minor isomers increased to levels that permitted isolation and structural determination by 2D-NMR (7–9, Scheme 3a–c). Harsher conditions also led to the isolation of an acetylated by-product in some cases using the least sterically hindered diyne (2) (10, Scheme 3d).
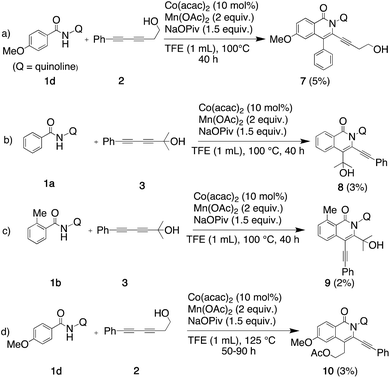 |
| Scheme 3 Isolated minor regioisomers and acetylated by-products (isolated yields). | |
Our rationalisation of the observed regioselectivity is based on our previous report39 and literature precedent.40–46 In our earlier work using symmetrical bulky diphenyl- and diphenoxy-1,3-diynes, the major regioisomers obtained each had the alkynyl substituent at the 3-position, ortho to the amide nitrogen and its pendent quinolinyl directing group. The steric bulk of the directing group makes the approach of the diyne for metallacycle formation easier with the bulky substituent furthest from the quinolinyl moiety. The same preference for insertion was observed for all reactions with the unsymmetrical diynes used in the present study, although with a substituent-dependent switch in the preferred orientation of insertion. The unsymmetrical diynes used had a bulky non-polar phenyl substituent and a hydroxyl-bearing alkyl chain. The switch was observed between reactions performed using the least sterically hindered hydroxyl diyne (2) and the diynes with more sterically demanding hydroxyl-bearing substituents (Fig. 3). We propose that the orientation of insertion of diyne 2 could be steered by the hydroxyl through a secondary interaction with the metal centre influencing the migratory insertion or its coordination to the metal centre and interaction, which is not feasible when the bulkier diynes are employed. Thus, the cobalt complex A reacts with diynes 2 and 3 to give complexes B and E with two diyne orientations possible for coordination. There is a selection of one cobalt-diyne complex (C and F) leading to D and G, and their corresponding products (3aa and 4ab) as the major regioisomers.
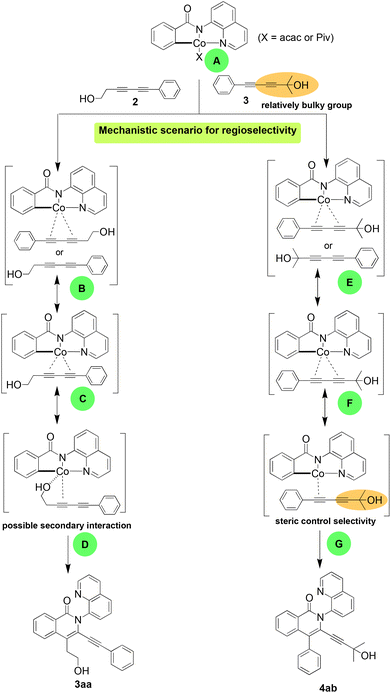 |
| Fig. 3 Proposed mechanistic scenario for the observed regioselectivity. | |
To test this hypothesis, we expanded our mechanistic studies to include diyne 11, with less bulk than diyne 2, and diyne 12. Importantly, the regioselectivity observed with 2 was maintained, resulting in the formation of the corresponding products 13 (51%) and 14 (56%) (Scheme 4). The less sterically hindered diynes thus appear to coordinate with the cobaltocycle through the substituent oxygen, facilitating the preferred regioselectivity.
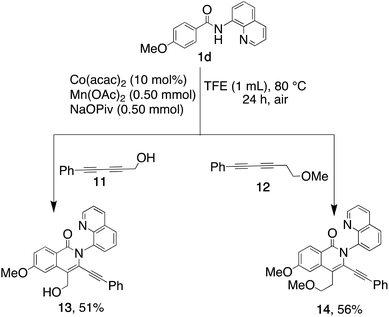 |
| Scheme 4 Mechanistic studies with diynes 11 and 12. | |
Conclusions
In summary, we report a regioselective cobalt catalysed C–H activation reaction of aminoquinoline benzamides with unsymmetrical 1,3-diynes that can be performed under relatively mild conditions. This reaction furnishes the corresponding 3- or 4-hydroxyalkyl substituted heterocycles with the regiochemistry of the dominant product determined by the nature of the substituents present on the 1,3-diyne. The ability to predetermine the orientation of insertion and subsequent location of functionality should be a valuable tool for the synthesis of isoquinolinone-derived materials or bioactive agents.
Experimental details
General procedure for cobalt catalyzed C–H activation with unsymmetrical diynes (Schemes 1 & 2)
In an oven dried reaction tube, charged with a magnetic stir-bar, unsymmetrical 1,3-diyne (0.25 mmol, 1.0 equiv.), Co(acac)2 (10 mol%), benzamide (0.50 mmol, 2.0 equiv.), Mn(OAc)2 (0.50 mmol, 2.0 equiv.), and NaOPiv (0.50 mmol, 2.0 equiv.) were added. Then, TFE (1 mL) was added to the system and the reaction was stirred at 80 °C for 24 h. The major regioisomer was isolated through flash chromatography in petroleum ether
:
acetone (3
:
2) and characterized by NMR, IR and HRMS.
General procedure for control experiments (Scheme 3)
In an oven dried reaction tube, charged with a magnetic stir-bar, unsymmetrical 1,3-diyne (0.58 mmol, 1.0 equiv.), Co(acac)2 (10 mol%), benzamide (0.86 mmol, 1.5 equiv.), Mn(OAc)2 (1.15 mmol, 2.0 equiv.), and NaOPiv (0.87 mmol, 1.5 equiv.) were added. Then, TFE (1 mL) was added to the system and the reaction was stirred at 100–125 °C for 40–90 h. The major and minor regioisomer and the acylated by-products were isolated through flash chromatography and preparative TLC in petroleum ether
:
acetone (3
:
2) and characterized by NMR, IR and HRMS.
HPLC-based yield determination
As the reaction resulted in complex mixtures due to the presence of minor isomers with similar chromatographic properties, an HPLC-based assay was proposed as a tool to directly determine the yield of the major isomer in situ, to avoid misinterpretation of reaction outcomes due to losses during purification. From the reaction mixture, 15 μL sample was taken and made up to 1 mL in 2-propanol with a dilution factor of 66.66. Pure samples of each of the major regioisomeric products with a stock solution of 3 mg mL−1 were used to construct calibration curves. The chromatograms were recorded using a D-7000 HSM software on a gradient method (petroleum ether/2-propanol) with a flow rate of 1 mL min−1, injection volume 10 μL, and acquisition wavelength ranging from 280–330 nm.
Experimental data
4-(2-Hydroxyethyl)-3-(2-phenylethynyl)-2-(quinolin-8-yl)-1,2-dihydroisoquinolin-1-one (3aa).
M.P.: 149–150 °C; Appearance: brown crystal; HPLC yield: 24%; 1H NMR (400 MHz, Methanol-d4, δ 3.31)δ 8.80 (dd, J = 4.3, 1.7 Hz, 1H), 8.52 (dd, J = 8.4, 1.7 Hz, 1H), 8.44 (ddd, J = 8.0, 1.4, 0.6 Hz, 1H), 8.20 (dd, J = 8.2, 1.5 Hz, 1H), 8.05 (ddd, J = 8.3, 1.1, 0.6 Hz, 1H), 7.94–7.85 (m, 2H), 7.83 (dd, J = 8.2, 7.3 Hz, 1H), 7.65 (ddd, J = 8.1, 7.1, 1.1 Hz, 1H), 7.59 (dd, J = 8.3, 4.3 Hz, 1H), 7.27–7.19 (m, 1H), 7.18–7.09 (m, 2H), 6.71–6.65 (m, 2H), 3.96–3.88 (m, 2H), 3.40 (dd, J = 7.7, 6.8 Hz, 2H).13C NMR (101 MHz, Methanol-d4, δ 49.00)δ 164.0, 152.1, 145.5, 138.7, 138.5, 138.1, 134.4, 131.9, 131.9, 130.9, 130.8, 130.2, 129.3, 129.2, 128.9, 127.8, 127.6, 126.9, 125.3, 123.2, 122.4, 120.8, 100.9, 83.6, 62.3, 33.7. FTIR (cm−1): 3412, 2140, 1651, 1599, 1328, 1033, 740. HRMS C28H20N2O2 [M + H+]: calculated: 417.1603, found: 417.1600.
4-(2-Hydroxyethyl)-8-methyl-3-(2-phenylethynyl)-2-(quinolin-8-yl)-1,2-dihydroisoquinolin-1-one (3ba).
M.P.: 188–190 °C; Appearance: brown crystal; HPLC yield: 28%; 1H NMR (400 MHz, DMSO-d6, δ 2.50)δ 8.84 (dd, J = 4.2, 1.7 Hz, 1H), 8.52 (dd, J = 8.4, 1.7 Hz, 1H), 8.32–8.10 (m, 1H), 7.91–7.87 (m, 1H), 7.84 (d, J = 8.3 Hz, 1H), 7.80 (t, J = 7.7 Hz, 1H), 7.73 (t, J = 7.7 Hz, 1H), 7.58 (dd, J = 8.3, 4.2 Hz, 1H), 7.39 (d, J = 7.4 Hz, 1H), 7.27 (d, J = 7.3 Hz, 1H), 7.21 (t, J = 7.3 Hz, 2H), 6.74–6.57 (m, 2H), 4.90 (s, 1H), 3.69 (t, J = 7.5 Hz, 2H), 3.22 (t, J = 7.5 Hz, 2H), 2.76 (s, 3H). 13C NMR (101 MHz, DMSO-d6, δ 39.52)δ 161.6, 150.9, 144.2, 141.5, 138.0, 137.8, 136.3, 132.2, 130.8, 130.5, 130.3, 129.2, 128.9, 128.7, 128.6, 126.3, 125.3, 124.5, 122.4, 121.9, 120.7, 117.7, 98.3, 83.1, 60.5, 33.3, 23.8. FTIR (cm−1): 3244.24, 3057.93, 2115.84, 1653, 1555.67, 1359.58, 1031.15, 750.40. HRMS C29H22N2O2 [M + H+]: calculated: 431.1760, found: 431.1755.
6-tert-Butyl-4-(2-hydroxyethyl)-3-(2-phenylethynyl)-2-(quinolin-8-yl)-1,2-dihydroisoquinolin-1-one (3ca).
M.P.: 145–146 °C; Appearance: off-white crystal; HPLC yield: 21%; 1H NMR (400 MHz, DMSO-d6) δ 8.81 (dd, J = 4.2, 1.7 Hz, 1H), 8.53 (dd, J = 8.4, 1.7 Hz, 1H), 8.23 (d, J = 5.5 Hz, 1H), 8.22–8.19 (m, 1H), 7.92 (d, J = 1.8 Hz, 1H), 7.91 (dd, J = 7.4, 1.5 Hz, 1H), 7.81 (t, J = 7.8 Hz, 1H), 7.72 (dd, J = 8.5, 1.8 Hz, 1H), 7.58 (dd, J = 8.3, 4.2 Hz, 1H), 7.31–7.25 (m, 1H), 7.22 (dd, J = 8.2, 6.5 Hz, 2H), 6.72–6.62 (m, 2H), 4.95 (t, J = 5.7 Hz, 1H), 3.73 (q, J = 6.9 Hz, 2H), 3.26 (dt, J = 7.3, 4.6 Hz, 2H), 1.43 (s, 9H). 13C NMR (101 MHz, DMSO-d6) δ 160.6, 155.8, 151.0, 144.1, 137.6, 136.3, 136.0, 130.5, 130.3, 129.2, 129.1, 128.6, 128.6, 127.6, 126.2, 125.5, 125.3, 124.0, 121.9, 120.7, 120.2, 118.4, 98.4, 83.2, 60.7, 35.2, 32.9, 30.9. FTIR (cm−1): 2960.92, 1649.05, 1595.09, 1360.57, 1020.16, 784.554. HRMS C32H28N2O2 [M + H+]: calculated: 473.2229, found: 473.2228.
4-(2-Hydroxyethyl)-6-methoxy-3-(2-phenylethynyl)-2-(quinolin-8-yl)-1,2-dihydroisoquinolin-1-one (3da).
M.P.: 190–191 °C; Appearance: white crystal; HPLC yield: 54%; 1H NMR (400 MHz, DMSO-d6, δ 2.50)δ 8.83 (dd, J = 4.2, 1.7 Hz, 1H), 8.52 (dd, J = 8.3, 1.7 Hz, 1H), 8.24–8.17 (m, 2H), 7.90 (d, J = 7.2 Hz, 1H), 7.80 (t, J = 7.7 Hz, 1H), 7.58 (dd, J = 8.3, 4.2 Hz, 1H), 7.36 (d, J = 2.4 Hz, 1H), 7.31–7.18 (m, 3H), 6.68–6.63 (m, 2H), 4.93 (t, J = 5.7 Hz, 1H), 3.98 (s, 3H), 3.71 (q, J = 6.8 Hz, 2H), 3.21 (t, J = 7.5 Hz, 2H). 13C NMR (101 MHz, DMSO-d6, δ 39.52)δ 162.8, 160.5, 151.0, 144.2, 138.3, 137.7, 136.4, 130.6, 130.4, 130.0, 129.4, 129.2, 128.7, 128.6, 126.3, 125.9, 122.0, 120.7, 119.9, 117.9, 116.1, 106.4, 98.5, 83.2, 60.6, 55.7, 33.0. FTIR (cm−1): 3370, 2963, 2175, 1599, 1567, 1486, 1377, 1328, 1226.478. HRMS C29H22N2O3 [M + H+]: calculated: 447.1709, found: 447.1699.
6-Fluoro-4-(2-hydroxyethyl)-3-(2-phenylethynyl)-2-(quinolin-8-yl)-1,2-dihydroisoquinolin-1-one (3ea).
M.P.: 110–113 °C; Appearance: off-white solid; HPLC yield: 35%; 1H NMR (400 MHz, CDCl3, δ 7.26)δ 8.85 (dd, J = 4.3, 1.7 Hz, 1H), 8.53 (dd, J = 8.9, 6.0 Hz, 1H), 8.27 (dd, J = 8.4, 1.7 Hz, 1H), 8.01 (dd, J = 8.3, 1.4 Hz, 1H), 7.84 (dd, J = 7.3, 1.4 Hz, 1H), 7.72 (t, J = 7.8 Hz, 1H), 7.46 (dd, J = 10.4, 2.5 Hz, 1H), 7.41 (dd, J = 8.3, 4.2 Hz, 1H), 7.23 (m, 1H), 7.19 (m, 1H), 7.11 (dd, J = 8.3, 6.8 Hz, 2H), 6.67 (m, 2H), 3.94 (t, J = 6.8 Hz, 2H), 3.27 (t, J = 6.7 Hz, 2H). 13C NMR (101 MHz, CDCl3, δ 77.16)δ 165.7 (d, J = 252.5 Hz), 161.5, 151.03, 139.3 (d, J = 9.9 Hz), 137.5, 136.6, 132.1 (d, J = 10.0 Hz), 131.0, 130.4, 129.2, 129.2, 129.0, 128.2, 127.3, 126.3, 123.3 (d, J = 2.0 Hz), 121.8, 121.3, 117.8 (d, J = 1.8 Hz), 115.8 (d, J = 23.3 Hz), 109.2 (d, J = 23.2 Hz), 100.0, 82.8, 61.9, 32.9. FTIR (cm−1): 3436.60, 2921.91, 2111.50, 1639.35, 1606.65, 1486.42, 1369.79, 1044.82, 752.49. HRMS C28H19FN2O2 [M + H+]: calculated: 435.1509, found: 435.1510.
6-Chloro-4-(2-hydroxyethyl)-3-(2-phenylethynyl)-2-(quinolin-8-yl)-1,2-dihydroisoquinolin-1-one (3fa).
M.P.: 166–168 °C; Appearance: light yellow solid; HPLC yield: 31%; 1H NMR (400 MHz, CDCl3, TMS)δ 8.78 (d, J = 4.1 Hz, 1H), 8.38 (d, J = 8.6 Hz, 1H), 8.20 (d, J = 8.4 Hz, 1H), 7.94 (d, J = 8.2 Hz, 1H), 7.76 (d, J = 7.3 Hz, 1H), 7.75 (s, 1H), 7.65 (t, J = 7.8 Hz, 1H), 7.40 (d, J = 8.6 Hz, 1H), 7.35 (dd, J = 8.3, 4.2 Hz, 1H), 7.12 (t, J = 7.6 Hz, 1H), 7.04 (t, J = 7.7 Hz, 2H), 6.60 (d, J = 7.6 Hz, 2H), 3.90 (t, J = 6.8 Hz, 2H), 3.23 (t, J = 6.8 Hz, 2H). 13C NMR (101 MHz, CDCl3, TMS)δ 160.5, 150.1, 143.4, 138.4, 137.1, 136.5, 135.4, 130.0, 129.6, 129.3, 128.2, 128.2, 128.0, 127.1, 126.8, 126.3, 125.3, 124.0, 122.3, 120.8, 120.2, 116.4, 99.1, 81.8, 60.9, 31.6. FTIR (cm−1): 3057.93, 2959.50, 1647.49, 1594.43, 1326.38, 1024.06, 776.919. HRMS C28H19ClN2O2 [M + H+]: calculated: 451.1213, found: 451.1207.
7-Bromo-4-(2-hydroxyethyl)-3-(2-phenylethynyl)-2-(quinolin-8-yl)-1,2-dihydroisoquinolin-1-one (3ga).
M.P.: 138–140 °C; Appearance: light yellow solid; HPLC yield: 7%; 1H NMR (400 MHz, Methanol-d4, δ 3.31)δ 8.81 (dd, J = 4.3, 1.7 Hz, 1H), 8.53 (td, J = 4.6, 4.0, 1.7 Hz, 2H), 8.22 (dd, J = 8.2, 1.5 Hz, 1H), 8.01 (dd, J = 8.8, 2.1 Hz, 1H), 7.97 (d, J = 8.8 Hz, 1H), 7.91 (dd, J = 7.4, 1.5 Hz, 1H), 7.84 (dd, J = 8.2, 7.4 Hz, 1H), 7.61 (dd, J = 8.3, 4.3 Hz, 1H), 7.26–7.21 (m, 1H), 7.17–7.12 (m, 2H), 6.70–6.66 (m, 2H), 3.91 (t, J = 7.2 Hz, 2H), 3.37 (t, J = 7.1 Hz, 2H). 13C NMR (101 MHz, Methanol-d4, δ 49.00)δ 162.8, 152.2, 145.4, 138.5, 138.4, 137.3, 137.1, 131.9, 131.8, 131.6, 131.0, 130.9, 130.4, 129.4, 129.0, 127.8, 127.5, 127.5, 123.3, 122.6, 122.3, 120.4, 101.4, 83.4, 62.2, 33.7. FTIR (cm−1): 3402.42, 2955.98, 2203.72, 1634.24, 1567.45, 1476.06, 1324.90, 1047.19, 785.95. HRMS C28H19BrN2O2 [M + H+]: calculated: 497.0688, found: 497.0688.
3-(3-Hydroxy-3-methylbut-1-yn-1-yl)-4-phenyl-2-(quinolin-8-yl)-1,2-dihydroisoquinolin-1-one (4ab).
M.P.: 218–220 °C; Appearance: light brown crystal; HPLC yield: 40%; NMR:1H NMR (400 MHz, DMSO-d6)δ 8.87 (dd, J = 4.2, 1.7 Hz, 1H), 8.50 (dd, J = 8.4, 1.7 Hz, 1H), 8.34–8.25 (m, 1H), 8.15 (dd, J = 8.3, 1.4 Hz, 1H), 7.88 (dd, J = 7.3, 1.4 Hz, 1H), 7.76 (ddd, J = 8.3, 7.3, 1.2 Hz, 2H), 7.66–7.55 (m, 2H), 7.55–7.39 (m, 5H), 7.31 (dt, J = 8.0, 1.0 Hz, 1H), 4.77 (s, 1H), 0.54 (s, 3H), 0.51 (s, 3H). 13C NMR: (101 MHz, DMSO-d6)δ 160.9, 150.8, 144.1, 137.3, 136.4, 136., 135.3, 132.9, 130.9, 130.8, 130.1, 129.0, 128.7, 128.3, 127.8, 127.6, 126.1, 125.7, 125.2, 125.2, 122.3, 121.8, 105.1, 75.0, 62.8, 30.0. FTIR (cm−1): 3068, 2973, 2145, 1627, 1497, 1578. HRMS C29H22N2O2 [M + H+]: calculated: 431.1760, found: 431.1758.
3-(3-Hydroxy-3-methylbut-1-yn-yl)-8-methyl-4-(phenyl)-2-(quinolin-8-yl)-1,2-dihydroisoquinolin-1-one (4bb).
M.P.: 208–210 °C; Appearance: brown crystal; HPLC yield: 43%; 1H NMR (400 MHz, Methanol-d4, δ 3.31)δ 8.84 (s, 1H), 8.47 (dd, J = 8.3, 2.8 Hz, 1H), 8.12 (dd, J = 8.2, 2.7 Hz, 1H), 7.84 (dd, J = 7.6, 2.5 Hz, 1H), 7.78 (dd, J = 7.9, 2.9 Hz, 1H), 7.64–7.56 (m, 1H), 7.52–7.44 (m, 4H), 7.45–7.39 (m, 2H), 7.34 (s, 1H), 7.17 (dd, J = 8.3, 2.8 Hz, 1H), 2.85 (s, 3H), 0.64 (s, 3H), 0.60 (s, 3H). 13C NMR (101 MHz, Methanol-d4, δ 49.00)δ 164.8, 151.9, 145.7, 143.3, 140.4, 138.9, 138.4, 137.9, 133.1, 132.2, 132.1, 131.9, 131., 130.55, 129.5, 129.4, 128.9, 127.8, 126.5, 126.0, 125.7, 125.4, 123.1, 105.5, 76.7, 64.9, 30.4, 24.4. FTIR (cm−1): 3440, 2980, 2120, 1675, 775. HRMS C30H24N2O2 [M + H+]: calculated: 445.1916, found: 445.1918.
6-tert-Butyl-3-(3-hydroxy-3-methylbut-1-yn-1-yl)-4-phenyl-2-(quinolin-8-yl)-1,2-dihydroisoquinolin-1-one (4cb).
M.P.: 136–138 °C; Appearance: white powder; HPLC yield: 47%; 1H NMR (400 MHz, Methanol-d4, δ 3.31)δ 8.83 (dd, J = 4.3, 1.7 Hz, 1H), 8.48 (dd, J = 8.4, 1.7 Hz, 1H), 8.36 (dd, J = 8.5, 0.5 Hz, 1H), 8.14 (dd, J = 8.2, 1.5 Hz, 1H), 7.86 (dd, J = 7.3, 1.5 Hz, 1H), 7.78 (dd, J = 8.2, 7.4 Hz, 1H), 7.73 (dd, J = 8.5, 1.9 Hz, 1H), 7.60 (dd, J = 8.3, 4.3 Hz, 1H), 7.55–7.43 (m, 5H), 7.41 (dd, J = 1.9, 0.6 Hz, 1H), 1.28 (s, 9H), 0.66 (s, 3H), 0.61 (s, 3H). 13C NMR (101 MHz, Methanol-d4, δ 49.00)δ 164.0, 157.9, 152.0, 145.6, 138.5, 138.5, 138.4, 137.2, 132.2, 132.0, 131.8, 130.9, 130.7, 129.4, 129.4, 129.1, 128.7, 127.7, 127.0, 126.9, 126.0, 125.0, 123.1, 123.1, 105.6, 76.8, 64.9, 36.2, 31.3, 30.3. FTIR (cm−1): 3412, 2966, 2120, 1634, 1606, 1567, 1497, 1321, 775. HRMS C33H30N2O2 [M + H+]: calculated: 487.2386, found: 487.2394.
3-(3-Hydroxy-3-methylbut-1-yn-1-yl)-6-methoxy-4-phenyl-2-(quinolin-8-yl)-1,2-dihydroisoquinolin-1-one (4db).
M.P.: 155–156 °C; Appearance: pale yellow crystal; HPLC yield: 56%; 1H NMR (400 MHz, Methanol-d4, δ 3.31)δ 8.84 (dd, J = 4.3, 1.7 Hz, 1H), 8.48 (dd, J = 8.3, 1.7 Hz, 1H), 8.35 (d, J = 8.9 Hz, 1H), 8.13 (dd, J = 8.2, 1.5 Hz, 1H), 7.85 (dd, J = 7.3, 1.5 Hz, 1H), 7.78 (dd, J = 8.2, 7.3 Hz, 1H), 7.60 (dd, J = 8.4, 4.3 Hz, 1H), 7.57–7.40 (m, 5H), 7.20 (dd, J = 8.9, 2.5 Hz, 1H), 6.75 (d, J = 2.5 Hz, 1H), 3.77 (s, 3H), 0.65 (s, 3H), 0.60 (s, 3H). 13C NMR (101 MHz, Methanol-d4, δ 49.00)δ 164.9, 163.9, 152.0, 145.6, 140.8, 138.5, 138.4, 137.2, 132.2, 132.0, 131.9, 131.0, 130.9, 130.7, 129.5, 129.5, 129.1, 127.7, 126.6, 126.3, 123.1, 120.9, 117.7, 108.5, 105.7, 76.7, 64.9, 55.9, 30.3. FTIR (cm−1): 3320, 2970, 2120, 1760, 1680, 1590, 1230, 1030. HRMS C30H24N2O3 [M + H+]: calculated: 461.1865, found: 461.1876.
6-Fluoro-3-(3-hydroxy-3-methylbut-1-yn-1-yl)-4-phenyl-2-(quinolin-8-yl)-1,2-dihydroisoquinolin-1-one (4eb).
M.P.: 218–220 °C; Appearance: light yellow solid; HPLC yield: 22%; 1H NMR (400 MHz, CDCl3, δ 7.26)δ 8.94 (dd, J = 4.4, 1.7 Hz, 1H), 8.55 (dd, J = 8.8, 5.9 Hz, 1H), 8.27 (dd, J = 8.3, 1.7 Hz, 1H), 7.98 (dd, J = 8.3, 1.4 Hz, 1H), 7.83 (dd, J = 7.4, 1.4 Hz, 1H), 7.69 (t, J = 7.8 Hz, 1H), 7.52 (m, 1H), 7.45 (m, 4H), 7.22 (td, J = 8.5, 2.6 Hz, 1H), 7.01 (dd, J = 10.3, 2.5 Hz, 1H), 0.73 (s, 3H), 0.69 (s, 3H). 13C NMR (101 MHz, CDCl3, δ 77.16)δ 165.6 (d, J = 252.3 Hz), 161.6, 151.1, 144.6, 139.7 (d, J = 10.0 Hz), 137.6, 136.4, 135.6, 131.9 (d, J = 10.0 Hz), 131.3, 130.9, 130.4, 129.3, 129.2, 128.5, 128.4, 128.2, 126.3, 126.2, 123.8 (d, J = 3.1 Hz), 123.2 (d, J = 1.9 Hz), 121.8, 116.0 (d, J = 23.5 Hz), 111.1 (d, J = 23.5 Hz), 103.7, 76.6, 64.8, 29.9. FTIR (cm−1): 3180, 2124, 1655, 1609, 1599, 1469, 1328, 775. HRMS C29H21FN2O3 [M + H+]: calculated: 449.1665, found: 449.1664.
6-Chloro-3-(3-hydroxy-3-methylbut-1-yn-1-yl)-4-phenyl-2-(quinolin-8-yl)-1,2-dihydroisoquinolin-1-one (4fb).
M.P.: 250–252 °C; Appearance: white crystal; HPLC yield: 21%; 1H NMR (400 MHz, DMSO-d6, δ 2.50)δ 8.88 (dd, J = 4.2, 1.7 Hz, 1H), 8.51 (dd, J = 8.4, 1.7 Hz, 1H), 8.31 (dd, J = 8.5, 0.4 Hz, 1H), 8.16 (dd, J = 8.3, 1.4 Hz, 1H), 7.89 (dd, J = 7.3, 1.4 Hz, 1H), 7.76 (dd, J = 8.2, 7.3 Hz, 1H), 7.66 (dd, J = 8.6, 2.1 Hz, 1H), 7.60 (dd, J = 8.3, 4.2 Hz, 1H), 7.55–7.41 (m, 5H), 7.21 (dd, J = 2.1, 0.5 Hz, 1H), 4.80 (s, 1H), 0.53 (s, 3H), 0.51 (s, 3H). 13C NMR (101 MHz, DMSO-d6, δ 39.52)δ 160.3, 150.9, 143.9, 138.0, 137.9, 137.0, 136.3, 134.6, 130.8, 130.8, 130.1, 130.1, 129.2, 128.7, 128.5, 128.1, 127.7, 126.7, 126.2, 124.3, 124.0, 121.9, 121.2, 106.0, 74.7, 62.8, 30.0. FTIR (cm−1): 3427, 2120, 1634, 1592, 1469, 1321, 775. HRMS C29H21ClN2O3 [M + H+]: calculated: 465.1370, found: 465.1362.
7-Bromo-3-(3-hydroxy-3-methylbut-1-yn-1-yl)-4-phenyl-2-(quinolin-8-yl)-1,2-dihydroisoquinolin-1-one (4gb).
M.P.: 130–132 °C; Appearance: light brown solid; HPLC yield: 18%; 1H NMR (400 MHz, Methanol-d4, δ 3.31)δ 8.84 (dd, J = 4.3, 1.7 Hz, 1H), 8.53 (d, J = 2.1 Hz, 1H), 8.49 (dd, J = 8.4, 1.7 Hz, 1H), 8.15 (dd, J = 8.2, 1.5 Hz, 1H), 7.90–7.86 (m, 1H), 7.84 (dd, J = 8.8, 2.2 Hz, 1H), 7.79 (dd, J = 8.2, 7.4 Hz, 1H), 7.61 (dd, J = 8.3, 4.3 Hz, 1H), 7.52–7.43 (m, 5H), 7.30 (d, J = 8.8 Hz, 1H), 0.65 (s, 3H), 0.61 (s, 3H). 13C NMR (101 MHz, Methanol-d4, δ 49.00)δ 162.9, 152.0, 145.4, 138.5, 138.2, 137.4, 137.1, 136.7, 132.1, 132.0, 131.8, 131.3, 131.0, 130.9, 129.6, 129.6, 129.3, 129.0, 128.7, 127.8, 126.7, 126.0, 123.2, 122.6, 106.3, 76.5, 64.9, 30.3. FTIR (cm−1): 3314.54, 2975.90, 2117.84, 1704.07, 1654.71, 1584.12, 1319.06, 1171.12, 789.85. HRMS C29H21BrN2O3 [M + H+]: calculated: 509.0865, found: 509.0869.
3-(3-Hydroxy-3,4-dimethylpent-1-yn-1-yl)-4-phenyl-2-(quinolin-8-yl)-1,2-dihydroisoquinolin-1-one (5ac).
M.P.: 90–94 °C; Appearance: Brown solid; HPLC yield: 32%; 1H NMR (400 MHz, CDCl3, δ 7.26)δ 8.90 (dt, 1H), 8.55 (dd, 1H), 8.21 (dt, 1H), 7.94 (dd, 1H), 7.82 (dt, 1H), 7.66 (ddd, 1H), 7.61 (ddd, 1H), 7.53 (m, 2H), 7.43 (m, 5H), 7.36 (m, 1H), 1.13 (hept, 1H), 0.58 (m, 3H), 0.24 (m, 6H). 13C NMR (101 MHz, CDCl3, δ 77.16)δ 162.2, 151.1, 144.8, 137.2, 136.3, 136.1, 132.6, 131.4, 131.0, 130.2, 129.3, 129.1, 128.6, 128.4, 128.3, 127.9, 127.5, 126.5, 126.2, 125.7, 125.0, 124.5, 121.7, 101.6, 78.8, 71.5, 38.0, 26.1, 17.2, 16.6. FTIR (cm−1): 3293.77, 2972.55, 2108.37, 1759.30, 1635.63, 1500.25, 1336.25, 1067.30, 726.11. HRMS C31H26N2O2 [M + H+]: calculated: 459.2073, found: 459.2072.
3-(3-Hydroxy-3-phenylbut-1-yn-1-yl)-4-phenyl-2-(quinolin-8-yl)-1,2-dihydroisoquinolin-1-one (6ad).
M.P.: 65–70 °C; Appearance: Yellow solid; HPLC yield: 29%; 1H NMR (400 MHz, CDCl3, δ 7.26)δ 8.91 (ddd, J = 5.9, 4.2, 1.7 Hz, 1H), 8.56 (ddt, J = 7.9, 1.5, 0.6 Hz, 1H), 8.22 (m, 1H), 7.95 (td, J = 8.1, 1.4 Hz, 1H), 7.86 (dd, J = 7.3, 1.4 Hz, 1H), 7.67 (ddd, J = 8.2, 7.4, 2.5 Hz, 1H), 7.63 (tt, J = 6.8, 1.3 Hz, 1H), 7.55 (m, 2H), 7.46 (m, 5H), 7.38 (dtd, J = 8.1, 1.2, 0.6 Hz, 1H), 7.12 (m, 1H), 7.03 (m, 2H), 6.67 (m, 2H), 0.94 (m, 3H). 13C NMR (101 MHz, CDCl3, δ 77.16)δ 162.3, 151.2, 144.9, 144.0, 137.9, 137.2, 136.2, 136.2, 132.7, 131.5, 131.1, 130.3, 129.3, 129.2, 128.7, 128.5, 128.5, 128.1, 128.0, 127.7, 127.6, 126.7, 126.3, 125.9, 124.9, 124.8, 124.5, 121.8, 101.5, 79.8, 69.8, 31.9. FTIR (cm−1): 3321.05, 2981.11, 2113.44, 1765.54, 1647.48, 1489.99, 1369.79, 1044.82, 752.49. HRMS C31H26N2O2 [M + H+]: calculated: 493.1916, found: 493.1918.
3-(4-Hydroxybut-1-yn-1-yl)-6-methoxy-4-phenyl-2-(quinolin-8-yl)-1,2-dihydroisoquinolin-1-one (7).
M.P.: 185–186 °C; Appearance: brown sticky solid; Isolated yield: 9%; 1H NMR (400 MHz, Methanol-d4)δ 8.83 (dd, J = 4.3, 1.7 Hz, 1H), 8.48 (dd, J = 8.4, 1.7 Hz, 1H), 8.33 (d, J = 8.9 Hz, 1H), 8.13 (dd, J = 8.2, 1.5 Hz, 1H), 7.87–7.82 (m, 2H), 7.80–7.74 (m, 1H), 7.63–7.57 (m, 1H), 7.52–7.42 (m, 5H), 7.18 (dd, J = 8.9, 2.5 Hz, 1H), 6.98 (d, J = 8.8 Hz, 1H), 6.71 (d, J = 2.5 Hz, 1H), 3.76 (s, 3H), 2.68 (t, J = 7.3 Hz, 2H), 1.78 (t, J = 7.5 Hz, 2H). 13C NMR (101 MHz, Methanol-d4)δ 164.9, 152.0, 145.6, 141.0, 138.6, 138.4, 137.5, 132.2, 132.0, 131.9, 131.0, 130.9, 130.7, 129.6, 129.5, 129.1, 127.7, 127.1, 125.8, 123.2, 120.8, 117.6, 108.4, 99.4, 76.9, 60.6, 55.9, 23.5. FTIR (cm−1): 772, 1252, 1377, 1486, 1603, 1654, 1705, 2928, 3356. HRMS C29H22N2O3 [M + H+]: calculated: 447.1709, found: 447.1699.
4-(2-Hydroxypropan-2-yl)-3-(2-phenylethynyl)-2-(quinolin-8-yl)-1,2-dihydroisoquinolin-1-one (8).
M.P.: 205 °C; Appearance: white sticky solid; Isolated yield: 3%; 1H NMR (400 MHz, Chloroform-d) δ 8.77 (d, J = 4.2 Hz, 1H), 8.48 (d, J = 8.0 Hz, 1H), 8.21 (d, J = 8.3 Hz, 1H), 8.04 (d, J = 8.2 Hz, 1H), 7.78 (d, J = 7.2 Hz, 1H), 7.71 (d, J = 8.3 Hz, 1H), 7.67 (d, J = 8.1 Hz, 1H), 7.62 (d, J = 8.1 Hz, 1H), 7.48 (t, J = 7.6 Hz, 1H), 7.35 (dd, J = 8.4, 4.2 Hz, 1H), 7.03 (d, J = 6.9 Hz, 4H), 6.95–6.89 (m, 1H), 3.84 (s, 1H), 1.84 (s, 6H). 13C NMR (101 MHz, Chloroform-d) δ 162.3, 150.6, 143.7, 135.1, 134.8, 134.5, 131.9, 130.9, 129.3, 129.1, 128.7, 128.2, 127.0, 126.9, 126.1, 125.3, 125.1, 124.5, 122.7, 121.4, 121.0, 100.4, 86.4, 76.2, 26.9, 26.8. FTIR (cm−1): 2971.55, 2123.88, 1730, 1459, 1257.79, 1085.05, 788.896. HRMS C29H22N2O2 [M + H+]: calculated: 431.1760, found: 431.1768.
3-(2-Hydroxypropan-2-yl)-8-methyl-4-(2-phenylethynyl)-2-(quinolin-8-yl)-1,2-dihydroisoquinolin-1-one (9).
M.P.: 212 °C; Appearance: Light Brown; Isolated yield: 2.0%; 1H NMR (400 MHz, Chloroform-d) δ 8.82 (s, 0H), 8.49 (dd, J = 9.1, 4.0 Hz, 0H), 8.17 (d, J = 8.3 Hz, 0H), 7.89 (t, J = 5.3 Hz, 0H), 7.71 (t, J = 5.6 Hz, 0H), 7.63 (d, J = 8.8 Hz, 1H), 7.47 (s, 1H), 7.35 (s, 1H), 7.25–7.16 (m, 1H), 7.09 (d, J = 7.5 Hz, 0H), 7.02 (d, J = 8.8 Hz, 1H), 6.43 (t, J = 5.8 Hz, 1H), 5.46 (s, 0H), 3.60 (s, 2H), 2.81 (d, J = 4.0 Hz, 1H), 2.58 (s, 0H), 2.12 (d, J = 4.0 Hz, 3H), 2.00–1.92 (m, 3H), 1.82 (s, 1H), 1.19 (s, 4H), 0.80 (d, J = 9.8 Hz, 1H). 13C NMR (101 MHz, Chloroform-d) δ 162.8, 151.1, 142.4, 139.0, 137.7, 136.1, 130.6, 130.5, 129.2, 128.8, 128.6, 128.1, 127.9, 126.4, 126.0, 123.2, 121.7, 102.6, 85.4, 74.3, 32.0, 24.8. FTIR (cm−1): 3440, 2980, 2120, 1675, 775. HRMS C29H22N2O2 [M + Na+]: calculated: 467.1735, found: 467.1724.
2-[6-Methoxy-1-oxo-3-(2-phenylethynyl)-2-(quinolin-8-yl)-1,2-dihydroisoquinolin-4-yl]ethyl acetate (10).
M.P.: 172–173 °C; Appearance: off-white solid; HPLC based yield: 9.3%; Isolated yield: 8.0 mg (4.3%); 1H NMR (400 MHz, CDCl3, TMS)δ 8.81 (dd, J = 4.0, 2.0 Hz, 1H), 8.39 (dd, J = 8.8, 1.5 Hz, 1H), 8.18 (d, J = 8.2 Hz, 1H), 7.93 (d, J = 8.1 Hz, 1H), 7.75 (d, J = 7.3 Hz, 1H), 7.64 (t, J = 7.6 Hz, 1H), 7.34 (dd, J = 8.6, 4.0 Hz, 1H), 7.28 (t, J = 2.0 Hz, 7H), 7.13 (m, 2H), 7.06 (m, 3H), 6.62 (m, 2H), 4.35 (m, 2H), 3.95 (s, 3H), 3.33 (t, J = 7.9 Hz, 2H), 1.97 (s, 3H). 13C NMR (101 MHz, CDCl3, TMS)δ 170.2, 162.3, 160.8, 150.1, 143.9, 137.4, 137.0, 135.0, 130.10, 130.0, 129.2, 128.1, 128.1, 127.8, 127.1, 125.9, 125.1, 120.6, 119.5, 115.5, 115.2, 104.4, 98.4, 81.9, 61.9, 54.7, 28.2, 20.0. FTIR (cm−1): 3321.42, 2844.40, 1734.40, 1605.65, 1336.63, 1022.75, 795.952. MALDI C31H24N2O4 [M + H+]: calculated: 489.18, found: 489.18.
4-(Hydroxymethyl)-6-methoxy-3-(2-phenylethynyl)-2-(quinolin-8-yl)isoquinolin-1-one (13).
M.P.: 211–212 °C Appearance: white solid; Isolated yield: 56 mg (51%); 1H NMR (400 MHz, CDCl3) δ 8.85 (dd, J = 4.3, 1.7 Hz, 1H), 8.42 (d, J = 8.9 Hz, 1H), 8.22 (dd, J = 8.3, 1.7 Hz, 1H), 7.98 (dd, J = 8.2, 1.4 Hz, 1H), 7.82 (dd, J = 7.3, 1.4 Hz, 1H), 7.69 (t, J = 7.8 Hz, 1H), 7.41 (d, J = 2.4 Hz, 1H), 7.38 (dd, J = 8.3, 4.2 Hz, 1H), 7.22–7.15 (m, 1H), 7.11 (dd, J = 8.9, 2.4 Hz, 1H), 7.09 (t, J = 7.6 Hz, 2H), 6.63 (dd, J = 8.3, 1.3 Hz, 2H), 5.11 (s, 2H), 3.97 (s, 3H), 2.40 (s, 1H). 13C NMR (101 MHz, CDCl3) δ 163.4, 162.2, 151.3, 144.9, 138.2, 137.9, 136.2, 131.1, 130.8, 130.5, 129.2, 129.2, 129.1, 128.2, 127.4, 126.3, 121.8, 121.3, 120.6, 119.7, 116.8, 106.0, 99.9, 82.4, 60.8, 55.7. FTIR (cm−1): 3414, 3059, 2107, 1654, 1605, 1492, 1250, 1207, 795. HRMS: C28H20N2O3 [M + H+]: calculated 433.1552, found: 433.1555.
6-Methoxy-4-(2-methoxyethyl)-3-(2-phenylethynyl)-2-(quinolin-8-yl)-1,2-dihydroisoquin olin-1-one (14).
M.P.: 215–216 °C; Appearance: white solid; Isolated yield: 84 mg (56%); 1H NMR (400 MHz, Chloroform-d) δ 8.80 (dd, J = 4.2, 1.7 Hz, 1H), 8.39 (d, J = 8.8 Hz, 1H), 8.16 (dd, J = 8.4, 1.7 Hz, 1H), 7.91 (dd, J = 8.3, 1.4 Hz, 1H), 7.74 (dd, J = 7.3, 1.4 Hz, 1H), 7.62 (dd, J = 8.2, 7.3 Hz, 1H), 7.31 (dd, J = 8.3, 4.2 Hz, 1H), 7.19–7.15 (m, 1H), 7.10 (d, J = 7.4 Hz, 1H), 7.07–7.01 (m, 3H), 6.64–6.57 (m, 2H), 3.89 (s, 3H), 3.69–3.62 (m, 2H), 3.35 (s, 3H). 13C NMR (101 MHz, CDCl3) δ 163.2, 161.8, 151.2, 144.9, 138.7, 138.1, 136.0, 131.1, 130.9, 130.2, 129.1, 129.0, 128.8, 128.2, 126.6, 126.1, 121.6, 121.6, 120.6, 117.7, 115.8, 105.8, 99.5, 83.1, 71.8, 58.8, 55.6, 30.1. FTIR (cm−1): 3044, 2866, 2113, 1645, 1599, 1238, 1106, 751. HRMS: C30H24N2O3 [M + H+]: calculated 461.1865, found: 461.1864.
Author contributions
PD and PA performed the syntheses and analyses. IAN and SK conceived the project. JW undertook the NMR studies. All authors contributed to the writing of the manuscript and gave approval for submission.
Conflicts of interest
There are no conflicts of interest to declare.
Acknowledgements
We thank Linnaeus University, the Swedish Research Council (Vetenskapsrådet, grant number 2014-4573), and the Swedish Knowledge Foundation (grant number 20190114) for financial support. We are grateful to Dr Subramanian Suriyanarayanan (LNU) and Prof. Kothandaraman Ramanjuam (IIT-Madras, Chennai) for help with XRD studies.
References
- T. Dalton, T. Faber and F. Glorius, ACS Cent. Sci., 2021, 7, 245–261 CrossRef CAS PubMed.
- R. H. Crabtree, J. Organomet. Chem., 2004, 689, 4083–4091 CrossRef CAS.
- M. Zhang, Y. Zhang, X. Jie, H. Zhao, G. Li and W. Su, Org. Chem. Front., 2014, 1, 843–895 RSC.
- Z. Chen, B. Wang, J. Zhang, W. Yu, Z. Liu and Y. Zhang, Org. Chem. Front., 2015, 2, 1107–1295 RSC.
- M. Moselage, J. Li and L. Ackermann, ACS Catal., 2016, 6, 498–525 CrossRef CAS.
- O. Daugulis, H.-Q. Do and D. Shabashov, Acc. Chem. Res., 2009, 42, 1074–1086 CrossRef CAS PubMed.
- T. K. Hyster and T. Rovis, J. Am. Chem. Soc., 2010, 132, 10565–10569 CrossRef CAS PubMed.
- J. M. Neely and T. Rovis, J. Am. Chem. Soc., 2013, 135, 66–69 CrossRef CAS PubMed.
- H. Wang, C. Grohmann, C. Nimphius and F. Glorius, J. Am. Chem. Soc., 2012, 134, 19592–19595 CrossRef CAS PubMed.
- L. Ackermann, Acc. Chem. Res., 2014, 47, 281–295 CrossRef CAS PubMed.
- K. Padala and M. Jeganmohan, Org. Lett., 2011, 13, 6144–6147 CrossRef CAS PubMed.
- P. B. Arockiam, C. Bruneau and P. H. Dixneuf, Chem. Rev., 2012, 112, 5879–5918 CrossRef CAS PubMed.
- X. Li, W. Ouyang, J. Nie, S. Ji, Q. Chen and Y. Huo, ChemCatChem, 2020, 12, 2358–2384 CrossRef CAS.
- P. Gandeepan, T. Müller, D. Zell, G. Cera, S. Warratz and L. Ackermann, Chem. Rev., 2019, 119, 2192–2452 CrossRef CAS PubMed.
- K. Gao and N. Yoshikai, Acc. Chem. Res., 2014, 47, 1208–1219 CrossRef CAS PubMed.
- D. Wei, X. Zhu, J.-L. Niu and M.-P. Song, ChemCatChem, 2016, 8, 1242–1263 CrossRef CAS.
- Y. Kommagalla and N. Chatani, Coord. Chem. Rev., 2017, 350, 117–135 CrossRef CAS.
- T. Yoshino and S. Matsunaga, Asian J. Org. Chem., 2018, 7, 1193–1205 CrossRef CAS.
- H. Zhang, M.-C. Sun, D. Yang, T. Li, M.-P. Song and J.-L. Niu, ACS Catal., 2022, 12, 1650–1656 CrossRef.
- F. H. Lutter, S. Graßl, L. Grokenberger, M. S. Hofmayer, Y.-H. Chen and P. Knochel, ChemCatChem, 2019, 11, 5188–5197 CrossRef CAS.
- L. Grigorjeva and O. Daugulis, Org. Lett., 2015, 17, 1204–1207 CrossRef CAS PubMed.
- T. T. Nguyen, L. Grigorjeva and O. Daugulis, ACS Catal., 2016, 6, 551–554 CrossRef CAS PubMed.
- T. T. Nguyen, L. Grigorjeva and O. Daugulis, Angew. Chem., Int. Ed., 2018, 57, 1688–1691 CrossRef CAS PubMed.
- D.-G. Yu, T. Gensch, F. de Azambuja, S. Vásquez-Céspedes and F. Glorius, J. Am. Chem. Soc., 2014, 136, 17722–17725 CrossRef CAS PubMed.
- D. Zhao, J. H. Kim, L. Stegemann, C. A. Strassert and F. Glorius, Angew. Chem., Int. Ed., 2015, 54, 4508–4511 CrossRef CAS PubMed.
- T. Gensch, F. J. R. Klauck and F. Glorius, Angew. Chem., Int. Ed., 2016, 55, 11287–11291 CrossRef CAS PubMed.
- A. Lerchen, T. Knecht, M. Koy, C. G. Daniliuc and F. Glorius, Chem. – Eur. J., 2017, 23, 12149–12152 CrossRef CAS PubMed.
- W. Song and L. Ackermann, Angew. Chem., Int. Ed., 2012, 51, 8251–8254 CrossRef CAS PubMed.
- N. Sauermann, R. Mei and L. Ackermann, Angew. Chem., Int. Ed., 2018, 57, 5090–5094 CrossRef CAS PubMed.
- B. V. Pati, P. S. Sagara, A. Ghosh, G. K. Das Adhikari and P. C. Ravikumar, J. Org. Chem., 2021, 86, 9428–9443 CrossRef CAS PubMed.
- M. Sen, R. Mandal, A. Das, D. Kalsi and B. Sundararaju, Chem. – Eur. J., 2017, 23, 17454–17457 CrossRef CAS PubMed.
- T. Yoshino and S. Matsunaga, Adv. Synth. Catal., 2017, 359, 1245–1262 CrossRef CAS.
- K. Bhadra and G. S. Kumar, Mini-Rev. Med. Chem., 2010, 10, 1235–1247 CrossRef CAS PubMed.
- M. Bian, L. Ma, M. Wu, L. Wu, H. Gao, W. Yi, C. Zhang and Z. Zhou, ChemPlusChem, 2020, 85, 388–388 CrossRef CAS.
- T. Bosanac, E. R. Hickey, J. Ginn, M. Kashem, S. Kerr, S. Kugler, X. Li, A. Olague, S. Schlyer and E. R. R. Young, Bioorg. Med. Chem. Lett., 2010, 20, 3746–3749 CrossRef CAS PubMed.
- Z. Jin, Nat. Prod. Rep., 2013, 30, 849–868 RSC.
- M. Itoh, M. Shimizu, K. Hirano, T. Satoh and M. Miura, J. Org. Chem., 2013, 78, 11427–11432 CrossRef CAS PubMed.
- D.-G. Yu, F. de Azambuja, T. Gensch, C. G. Daniliuc and F. Glorius, Angew. Chem., Int. Ed., 2014, 53, 9650–>9654 CrossRef CAS PubMed.
- S. Kathiravan and I. A. Nicholls, Org. Lett., 2017, 19, 4758–4761 CrossRef CAS PubMed.
- A. Dey and C. M. R. Volla, Org. Lett., 2020, 22, 7480–7485 CrossRef CAS PubMed.
- S. Kumar, S. Nunewar, T. K. Sabbi and V. Kanchupalli, Org. Lett., 2022, 24, 3395–3400 CrossRef CAS PubMed.
- R. Mei, W. Ma, Y. Zhang, X. Guo and L. Ackermann, Org. Lett., 2019, 21, 6534–6538 CrossRef CAS PubMed.
- S. K. Yadav, B. Ramesh and M. Jeganmohan, J. Org. Chem., 2022, 87, 4134–4153 CrossRef CAS PubMed.
- Y. Luo, H. Liu, J. Zhang, M. Liu and L. Dong, Org. Lett., 2020, 22, 7604–7608 CrossRef CAS PubMed.
- S. Qian, X. Pu, G. Chang, Y. Huang and Y. Yang, Org. Lett., 2020, 22, 5309–5313 CrossRef CAS PubMed.
- F. Zhao, X. Gong, Y. Lu, J. Qiao, X. Jia, H. Ni, X. Wu and X. Zhang, Org. Lett., 2021, 23, 727–733 CrossRef CAS PubMed.
|
This journal is © The Royal Society of Chemistry 2023 |