DOI:
10.1039/D2OB02134J
(Communication)
Org. Biomol. Chem., 2023,
21, 2504-2508
Addition of sulfonylphthalides to para-quinone methides: Selective 1,6-additions and oxidative annulations†
Received
23rd November 2022
, Accepted 2nd March 2023
First published on 10th March 2023
Abstract
KOtBu mediated addition of sulfonylphthalides to p-quinone methides led to the selective synthesis of isochroman-1,4-diones and addition products. Interestingly, isochroman-1,4-diones were obtained via an unprecedented oxidative annulation pathway. The present work highlights a wide variety of substrates, good yields, shorter reaction time and ambient reaction conditions. Furthermore, a few addition products were transformed into functionalized heterocyclic molecules. Additionally, the scale-up experiment suggests the practical feasibility of preparing isochroman-1,4-diones in higher-scale reactions.
Introduction
Sulfonylphthalide (SP) has been found to be a valuable donor for the Hauser–Kraus annulation reactions with a variety of Michael acceptors in the presence of a suitable base. Accordingly, several reviews1 on the development and application of these annulation reactions are well documented. The reactions of substituted phthalides with cyclic and acyclic Michael acceptors result in both addition products and annulation products depending on the nature of the acceptor and reaction conditions2 (Scheme 1a). On the other hand, p-quinone methides (p-QMs) have been receiving enormous attention from chemists because p-QMs serve as valuable Michael acceptors for annulations3 and a variety of nucleophilic additions using acids,4 bases,5 metal catalysts,6 or other catalysts7 (Scheme 1b). Furthermore, additions to p-QMs show the characteristics of step and atom economical reactions. As a result, several reviews8 are documented emphasizing the synthesis of structurally diverse molecules through 1,6-additions and annulation reactions of p-QMs. Additionally, p-QMs proved to be suitable precursors to construct a wide range of substituted phenols and substituted triarylmethane derivatives. In particular, heterocyclic triarylmethane molecules have shown diverse biological applications.9 Recently, we have also developed the reagent controlled regioselective addition of 1,2,3-triazoles to p-QMs for the synthesis of N1 and N2 substituted triazoles.10 Unexpectedly, very limited attention has been paid to the reaction of SP with p-QMs so far. Prof. I. N. N. Namboothiri's research group11 for the first time has employed o-hydroxyaryl substituted p-QM for the preparation of indenofurans, benzofurans, and spiro-lactones using a base (Scheme 1c). To the best of our knowledge, the reaction of p-QM (without the o-hydroxy group) with SP has not been reported to date.
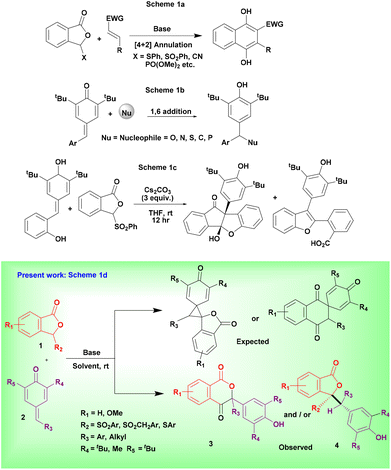 |
| Scheme 1 Synthetic approaches towards SP and p-QMs. | |
Based on the above literature observations, we hypothesized that the reaction of SP as donor and p-QM as acceptor units would furnish interesting multi-functionalized spiro molecules (Scheme 1d). Unpredictably, an unusual oxidative annulation took place in the reaction of SP with p-QM leading to the formation of isochroman-1,4-dione (Scheme 1d). Thus, in continuation of our research on the development of new synthetic methodologies,12 herein we present a conceptually new protocol for preparing isochroman-1,4-diones via oxidative annulation reactions.
Results and discussion
Initially, we reacted SP 1a with p-QM 2a in the presence of 1 equivalent of Cs2CO3 in 1,4-dioxane at room temperature (entry 1, Table 1). Unexpectedly, the substituted isochroman-1,4-dione 3a and addition product 4a were obtained instead of the expected H–K annulated spiro molecule. This unusual reactivity of 1a with 2a prompted us to optimize the reaction conditions. Subsequently, the reactions with increased and decreased quantities of Cs2CO3 led to the formation of products 3a and 4a, respectively, in slightly increased yields (entries 2–4, Table 1). Similarly, the reaction using NaH also produced product 3a but with a moderate yield (entry 5, Table 1). Among alkoxide bases, the reaction with 3 equivalents of KOtBu proceeded well and furnished 3a in a high yield (entries 6 and 7, Table 1). Interestingly, the addition product 4a was also obtained in a very good yield when a catalytic amount of KOtBu was used (entry 8, Table 1). We then examined organic bases such as DBU and DABCO for the present reaction. Reactions using these bases were not efficient and produced product 3a in moderate yields (entries 9 and 10, Table 1). Based on all the above reactions it is clear that KOtBu is a good choice for obtaining both 3a and 4a in a short time. Accordingly, further screening of solvents was performed using KOtBu. The reaction in THF gave a slightly decreased yield of 3a and an excellent yield of 4a (entries 11 and 12, Table 1). The reactions gave very lower yields of 3a and 4a in CHCl3 and did not work in toluene (entries 13 and 14, Table 1). Between polar aprotic DMF and DMSO solvents, the reactions in DMF were slightly better than those in DMSO and produced both 3a and 4a in good yields (entries 15 and 16, Table 1).
Table 1 Optimization of the reaction conditionsa
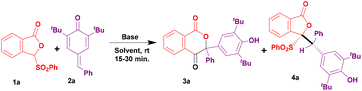
|
Entry |
Base (equiv.) |
Solvent |
Time (min) |
3a b yield (%) |
4a b yield (%) |
All reactions were carried out under a humid air atmosphere using 1a (1 equiv., 0.340 mmol) and 2a (1 equiv., 0.340 mmol) in a solvent (3.0 mL).
Isolated yields after column chromatography.
|
1 |
Cs2CO3 (1.0) |
1,4-Dioxane |
40 |
20 |
75 |
2 |
Cs2CO3 (2.0) |
1,4-Dioxane |
30 |
35 |
40 |
3 |
Cs2CO3 (3.0) |
1,4-Dioxane |
15 |
75 |
20 |
4 |
Cs2CO3 (0.2) |
1,4-Dioxane |
15 |
— |
89 |
5 |
NaH (3.0) |
1,4-Dioxane |
60 |
56 |
— |
6 |
NaOMe (3.0) |
1,4-Dioxane |
15 |
73 |
— |
7
|
KO
t
Bu (3.0)
|
1,4-Dioxane
|
15
|
86
|
10
|
8 |
KOtBu (0.2) |
1,4-Dioxane |
15 |
— |
85 |
9 |
DBU (3.0) |
1,4-Dioxane |
30 |
35 |
— |
10 |
DABCO (3.0) |
1,4-Dioxane |
60 |
35 |
— |
11 |
KOtBu (3.0) |
THF |
15 |
77 |
— |
12
|
KO
t
Bu (0.2)
|
THF
|
15
|
—
|
96
|
13 |
KOtBu (3.0) |
CHCl3 |
15 |
10 |
35 |
14 |
KOtBu (3.0) |
Toluene |
15 |
— |
— |
15 |
KOtBu (3.0) |
DMF |
15 |
77 |
— |
16 |
KOtBu (0.2) |
DMSO |
15 |
— |
52 |
Having established the optimized reaction conditions, we continued to explore the substrate scope for the formation of isochroman-1,4-dione and addition products. The p-QM derivatives containing electron-donating groups such as Me, OMe, OEt, NMe2, and isopropyl furnished the corresponding isochroman-1,4-diones in excellent yields (3b–f, Table 2). Halogen substituted p-QM derivatives also reacted with SP and produced the products in moderate to good yields (3g–j, Table 2). Similarly, heterocycle-embedded p-QM derivatives also reacted smoothly to afford the corresponding isochroman-1,4-diones in good yields (3k and3l, Table 2). The electron-withdrawing groups such as CN and NO2 substituted p-QM derivatives failed to react with SP. In these reactions, the starting materials were isolated back unaffected (3m and3n, Table 2). The p-QMs with naphthyl moieties also produced the desired products in very good yields (3o and3p, Table 2). Likewise, cyclohexyl incorporated p-QM also furnished the corresponding product under the optimized conditions in a good yield (3q, Table 2). In addition, unsymmetrically substituted p-QMs also underwent a reaction with SP to furnish the corresponding product in a very good yield (3r, Table 2). Furthermore, the reaction of di-methoxy substituted SP with p-QM proceeded smoothly and produced the product in a very good yield (3s, Table 2).
Table 2 Substrate scope for the formation of isochroman-1,4-dionea
All reactions were carried out under a humid air atmosphere using 1 (1 equiv., 0.340 mmol), 2 (1 equiv., 0.340 mmol) and KOtBu (3.0 equiv. 1.093 mmol) in 1,4-dioxane (3.0 mL) at room temperature for 15–30 min. Given yields: isolated yields after column chromatography.
|
|
We then continued to investigate the substrate scope of addition products and the results are tabulated in Table 3. The p-QM derivatives containing electron-donating groups such as Me, OMe, OEt, NMe2, and isopropyl furnished the corresponding addition products in excellent yields (4b–f, Table 3). Reactions using CN and NO2 substituted p-QM derivatives were unsuccessful with SP and the starting materials were isolated back unaffected (4g and4h, Table 3). Halogen-substituted p-QM derivatives also reacted with SP and furnished the addition products in moderate to good yields (4i–l, Table 3). Furthermore, the structure of 4l was confirmed by single crystal X-Ray diffraction analysis. Heterocycle-embedded p-QM derivatives also reacted smoothly to afford the corresponding addition products in good yields (4m–4q, Table 3). The p-QMs with naphthyl moieties also produced the desired products in very good yields (4r and 4s, Table 3).
Table 3 Substrate scope for the formation of 1,6 addition productsa
All reactions were carried out under a humid air atmosphere using 1 (1 equiv., 0.340 mmol), 2 (1 equiv., 0.340 mmol) and KOtBu (0.2 equiv. 0.072 mmol) in THF (3.0 mL) at room temperature for 15–30 min. Given yields: isolated yields after washing the crude product with hexane. dr values determined from 1H NMR analysis.
|
|
Likewise, cyclohexyl-incorporated p-QM also produced the product under the optimized conditions in a good yield (4t, Table 3). In addition, unsymmetrically substituted p-QM also underwent an addition reaction with SP to furnish the corresponding product in a very good yield (4u, Table 3). We then continued to explore substituted sulfonylphthalides for the addition reactions. Sulfonylphthalides containing electron-donating groups such as methyl and methoxy underwent additions to p-QM and furnished the desired addition products in very good yields (4aa and 4ab, Table 3). Similarly, halogen-substituted sulfonylphthalides reacted smoothly to give the addition products in good yields (4ac and 4ad, Table 3). 4-Chlorobenzylsulfonylphthalide also reacted with p-QM and produced the product in a good yield (4ae, Table 3). Similarly, phenylsulfanylphthalide also gave the corresponding addition product in a good yield under the optimized conditions (4af, Table 3).
To inspect the scale-up feasibility of preparing isochroman-1,4-diones, we carried out experiments using 1 gram each of 2b and 2g under the optimized reaction conditions (Scheme 2). We were pleased to find the formation of the products 3b and 3g with very good yields of 85% and 73%, respectively. Additionally, the structures of 3b and 3g were unambiguously confirmed by single crystal X-ray diffraction analysis.
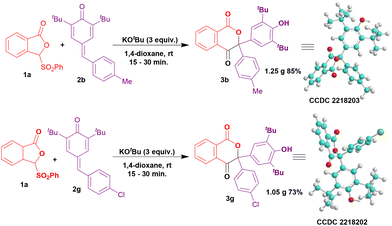 |
| Scheme 2 Scale-up reactions. | |
Furthermore, to showcase the synthetic utility of the corresponding addition products, we carried out a few reactions with hydrazine hydrate in ethanol under reflux conditions. These reactions transformed the addition products into substituted phthalazinone heterocyclic molecules 5a–c in very good yields (Scheme 3).
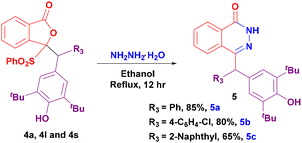 |
| Scheme 3 Synthesis of substituted phthalazinone derivatives. | |
To investigate the reaction mechanism of isochromanone synthesis, a few control experiments were performed (Scheme 4).
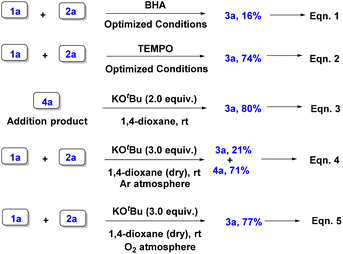 |
| Scheme 4 Control experiments. | |
Initially, SP (1a) was reacted with p-QM (2a) in the presence of radical scavengers such as BHA and TEMPO under standard conditions. Surprisingly, these reactions gave ambiguous results (eqn (1) and (2)).
Additionally, the reaction of addition product 4a with 2 equivalents of base also furnished isochromanone 3a in a good yield (eqn (3)). This particular reaction suggests the involvement of 4a as an intermediate in the synthesis of isochroman-1,4-dione 3a. Subsequently, the reaction of 1a and 2a under the optimized conditions using dry 1,4-dioxane and under an argon atmosphere furnished product 3a in a low yield (eqn (4)). Interestingly, a similar reaction of 1a and 2a under a molecular oxygen atmosphere produced the desired product 3a in a good yield (eqn (5)). Thus, the above two reactions clearly indicate that the source of the oxygen atom in the product is from molecular oxygen.
Thus, based on the above control experiments, herein we propose a tentative reaction mechanism (Scheme 5). Initially, the reaction of 1a and 2a in the presence of KOtBu produces the corresponding Michael addition product 4a. KOtBu then abstracts a proton to open the phthalide ring for the formation of species I which then cyclizes and reacts with molecular oxygen to form a peroxide species II. Species II then converts into species III by oxidizing tBuOH. Finally, species III eliminates the phenylsulfinate ion to furnish isochroman-1,4-dione 3a.
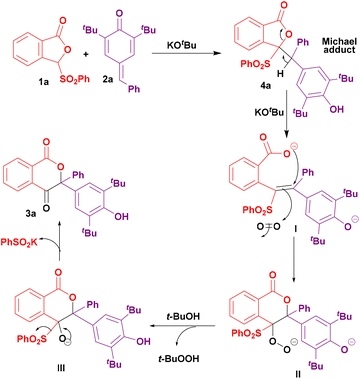 |
| Scheme 5 Tentative reaction mechanism. | |
Conclusions
In summary, we have synthesized KOtBu mediated selective addition and oxidative cyclization products from sulfonylphthalides and p-QMs in good yields. The reaction of SP and p-QMs catalyzed by KOtBu led to the formation of addition products which were found to be the intermediates for the preparation of isochromanone derivatives. The structures of both cyclized and addition products were confirmed by single crystal X-ray diffraction analysis. The highlights of the present method are a wide variety of substrates, good yields, shorter reaction time, and ambient reaction conditions. Interestingly, the scale-up experiment suggests the practical feasibility of preparing isochroman-1,4-diones in higher-scale reactions. Some of the addition products were transformed into functionalized heterocyclic molecules.
Conflicts of interest
There are no conflicts to declare.
Acknowledgements
The authors express gratitude to The Ministry of Tribal Affairs New Delhi, India, for financial support (201920-NFST-KAR-03093). The authors acknowledge DST-FIST Single Crystal X-ray Diffraction Facility at IIT Gandhinagar, Project No: SR/FST/CSI-277/2016. The authors also thank to Dr. Akshai Kumar A. S., IIT Guwahati and Dr. Venkatesha Rama Hathwar, Goa University, for their support and encouragements.
References
-
(a) A. S. Mitchell and R. A. Russell, Tetrahedron, 1995, 51, 5207 CrossRef CAS;
(b) D. Mal and P. Pahari, Chem. Rev., 2007, 107, 1892 CrossRef CAS PubMed;
(c) R. Karmakar, P. Pahari and D. Mal, Chem. Rev., 2014, 114, 6213 CrossRef CAS PubMed.
-
(a) F. M. Hauser and S. Prasanna, J. Am. Chem. Soc., 1981, 103, 6378 CrossRef CAS;
(b) G. Majetich, A. M. Casres, D. Chapman and M. Behnke, Tetrahedron Lett., 1983, 24, 1909 CrossRef CAS;
(c) N. Lebrasseur, G.-J. Fan, M. Oxoby, M. A. Looney and S. Quideau, Tetrahedron, 2005, 61, 1551 CrossRef CAS;
(d) M. P. Gore, S. J. Gould and D. D. Weller, J. Org. Chem., 1992, 57, 2774 CrossRef CAS;
(e) S. Kongsriprapan, C. Kuhakarn, P. Deelertpaiboon, K. Panthong, P. Tuchinda, M. Pohmakotr and V. Reutrakul, Pure Appl. Chem., 2012, 84, 1435 CAS;
(f) M. Watanabe, H. Morimoto, K. Nogami, S. Ijichi and S. Furukawa, Chem. Pharm. Bull., 1993, 41, 968 CrossRef CAS;
(g) C. S. Sankara, L. Satham and I. N. N. Namboothiri, J. Org. Chem., 2017, 82, 12944 Search PubMed;
(h) A. R. Katritzky, G. Zhang and L. Xie, Synth. Commun., 1997, 27, 3951 CrossRef CAS;
(i) J. Luo, C. Jiang, H. Wang, L. W. Xu and Y. Lu, Tetrahedron Lett., 2013, 54, 5261 CrossRef CAS;
(j) F. Zhong, J. Luo, G. Y. Chen, X. Dou and Y. Lu, J. Am. Chem. Soc., 2012, 134, 10222 CrossRef CAS PubMed.
-
(a) X. Z. Zhang, J. Y. Du, Y. H. Deng, W. D. Chu, X. Yan, K. Y. Yu and C. A. Fan, J. Org. Chem., 2016, 81, 2598 CrossRef CAS PubMed;
(b) S. H. Li, Y. Y. Liu, B. Huang, T. Zhou, H. M. Tao, Y. J. Xiao, L. Liu and J. L. Zhang, ACS Catal., 2017, 7, 2805 CrossRef CAS;
(c) K. Zhao, Y. Zhi, A. Wang and D. Enders, ACS Catal., 2016, 6, 657 CrossRef CAS;
(d) X. Z. Zhang, Y. H. Deng, X. Yan, K. Y. Yu, F. X. Wang, X. Y. Ma and C. A. Fan, J. Org. Chem., 2016, 81, 5655 CrossRef CAS PubMed;
(e) S. R. Angle and D. O. Arnaiz, J. Org. Chem., 1990, 55, 3708 CrossRef CAS;
(f) K. Zhao, Y. Zhi, T. Shu, A. Valkonen, K. Rissanen and D. Enders, Angew. Chem., Int. Ed., 2016, 55, 12104 CrossRef CAS PubMed;
(g) E. Amoah and R. K. Dieter, J. Org. Chem., 2017, 82, 2870 CrossRef CAS PubMed.
-
(a) S. Gao, X. Y. Xu, Z. B. Yuan, H. P. Zhou, H. Q. Yao and A. J. Lin, Eur. J. Org. Chem., 2016, 3006 CrossRef CAS;
(b) C. Lin, Y. Y. Shen, B. Huang, Y. Liu and S. L. Cui, J. Org. Chem., 2017, 82, 3950 CrossRef CAS PubMed;
(c) N. Dong, Z. P. Zhang, X. S. Xue, X. Li and J. P. Cheng, Angew. Chem., Int. Ed., 2016, 55, 1460 CrossRef CAS PubMed.
-
(a) L. Ge, X. H. Lu, C. Cheng, J. Chen, W. G. Cao, X. Y. Wu and G. Zhao, J. Org. Chem., 2016, 81, 9315 CrossRef CAS PubMed;
(b) W. D. Chu, L. F. Zhang, X. Bao, X. H. Zhao, C. Zeng, J. Y. Du, G. B. Zhang, F. X. Wang, X. Y. Ma and C. A. Fan, Angew. Chem., Int. Ed., 2013, 52, 9229 CrossRef CAS PubMed.
-
(a) V. Reddy and R. V. Anand, Org. Lett., 2015, 17, 3390 CrossRef CAS PubMed;
(b) Z. B. Yuan, W. W. Wei, A. J. Lin and H. Q. Yao, Org. Lett., 2016, 18, 3370 CrossRef CAS PubMed;
(c) A. Loopez, A. Parra, C. J. Barrera and M. Tortosa, Chem. Commun., 2015, 51, 17684 RSC;
(d) Y. Z. Lou, P. Cao, T. Jia, Y. L. Zhang, M. Wang and J. Liao, Angew. Chem., Int. Ed., 2015, 54, 12134 CrossRef CAS PubMed;
(e) M. Ke and Q. Song, Adv. Synth. Catal., 2017, 359, 384 CrossRef CAS;
(f) Y. Y. Shen, J. F. Qi, Z. J. Mao and S. L. Cui, Org. Lett., 2016, 18, 2722 CrossRef CAS PubMed;
(g) C. Yang, S. Gao, H. Q. Yao and A. J. Lin, J. Org. Chem., 2016, 81, 11956 CrossRef CAS PubMed.
-
(a) P. Arde and R. V. Anand, Org. Biomol. Chem., 2016, 14, 5550 RSC;
(b) P. W. Goswami, G. Singh and R. V. Anand, Org. Lett., 2017, 19, 1982 CrossRef CAS PubMed;
(c) Z. B. Yuan, X. X. Fang, X. Y. Li, J. Wu, H. Q. Yao and A. J. Lin, J. Org. Chem., 2015, 80, 11123 CrossRef CAS PubMed;
(d) X. Y. Li, X. Y. Xu, W. W. Wei, A. L. Lin and H. Q. Yao, Org. Lett., 2016, 18, 428 CrossRef CAS PubMed.
-
(a) B. Jiang, J. Wang, W. Hao and S. Tu, Org. Chem. Front., 2020, 7, 1743 RSC;
(b) C. G. S. Lima, F. P. Pauli, D. C. S. Costa, A. S. de Souza, L. S. M. Forezi, V. F. Ferreira and F. C. Silva, Eur. J. Org. Chem., 2020, 2692 Search PubMed;
(c) G. Singh, R. Pandey, Y. Pankhade, S. Fatma and R. V. Anand, Chem. Rec., 2021, 21, 4150 CrossRef CAS PubMed.
-
(a) P. M. Wood, L. W. L. Woo, J. R. Labrosse, M. N. Trusselle, S. Abbate, G. Longhi, E. Castiglioni, F. Lebon, A. Purohit, M. J. Reed and B. V. L. Potter, J. Med. Chem., 2008, 51, 4226 CrossRef CAS PubMed;
(b) M. Shiri, M. A. Zolfigol, H. G. Kruger and Z. Tanbakouchian, Chem. Rev., 2010, 110, 2250 CrossRef CAS PubMed;
(c) Q. L. He, F. L. Sun, X. J. Zheng and S. L. You, Synlett, 2009, 1111 CAS;
(d) S. Mondal and G. Panda, RSC Adv., 2014, 4, 28317 RSC;
(e) S. D. Cho, K. Yoon, S. Chintharlapalli, M. Abdelrahim and P. Lei, Cancer Res., 2007, 67, 674 CrossRef CAS PubMed;
(f) D. F. Duxbury, Chem. Rev., 1993, 93, 381 CrossRef CAS;
(g) X. Li, X. Xu, W. Wei, A. Lin and H. Yao, Org. Lett., 2016, 18, 428 CrossRef CAS PubMed;
(h) P. Arde and R. V. Anand, RSC Adv., 2016, 6, 77111 RSC;
(i) M. Recanatini, A. Cavalli and P. Valenti, Med. Res. Rev., 2002, 22, 282 CrossRef CAS PubMed.
- V. Rai, P. Kavyashree, S. S. Harmalkar, S. N. Dhuri and M. R. Maddani, Org. Biomol. Chem., 2022, 20, 345 RSC.
- P. Basu, N. Satam and I. N. N. Namboothiri, Org. Biomol. Chem., 2020, 18, 5677 RSC.
-
(a) G. S. Sorabad and M. R. Maddani, New J. Chem., 2019, 43, 6563 RSC;
(b) G. S. Sorabad and M. R. Maddani, New J. Chem., 2019, 43, 5996 RSC;
(c) G. S. Sorabad and M. R. Maddani, New J. Chem., 2020, 44, 2222 RSC;
(d) G. S. Sorabad and M. R. Maddani, Asian J. Org. Chem., 2019, 8, 1336 CrossRef CAS;
(e) V. Rai, G. S. Sorabad and M. R. Maddani, ChemistrySelect, 2020, 5, 6565 CrossRef CAS;
(f) V. Rai, G. S. Sorabad and M. R. Maddani, New J. Chem., 2021, 45, 1109 RSC;
(g) V. Rai, G. S. Sorabad and M. R. Maddani, New J. Chem., 2021, 45, 3969 RSC;
(h) V. Rai, G. S. Sorabad and M. R. Maddani, ChemistrySelect, 2021, 6, 6468 CrossRef CAS.
|
This journal is © The Royal Society of Chemistry 2023 |
Click here to see how this site uses Cookies. View our privacy policy here.