DOI:
10.1039/D2OB01996E
(Communication)
Org. Biomol. Chem., 2023,
21, 59-64
Ce-catalyzed regioselective synthesis of pyrazoles from 1,2-diols via tandem oxidation and C–C/C–N bond formation†
Received
2nd November 2022
, Accepted 22nd November 2022
First published on 22nd November 2022
Abstract
A novel and efficient cerium-catalyzed tandem oxidation and intermolecular ring cyclization of vicinal diols with hydrazones has been achieved for the regioselective synthesis of pyrazole derivatives. The corresponding 1,3-di- and 1,3,5-trisubstituted pyrazoles were obtained in moderate to excellent yields. The reaction has the advantages of mild conditions, easily available starting materials, broad substrate scope and good functional group tolerance.
Substituted pyrazoles are an important structural motif present in molecules having diverse applications including pharmaceuticals, agrochemicals, chemosensors, polymers, etc.1 Interestingly, 1,3-di- and 1,3,5-trisubstituted pyrazoles constitute the core structure of many marketed drugs and crop protecting chemicals; for example, Celecoxib, a selective cox-2 inhibitor used as a non-steroidal anti-inflammatory drug.2 Further examples include CDPPB, a clinical trial drug used for the treatment of schizophrenia and Difenamizole which has been used as an analgesic as well as an anti-inflammatory drug (Fig. 1).3 In addition, C3-arylated/heteroarylated N-substituted pyrazoles have also been found in the field of coordination chemistry.4 Recently, 1,3,5-trisubstituted pyrazoles have also been employed for C–H activation reactions (Fig. 1).5 The broad applications of pyrazoles stimulate organic chemists to develop efficient, general and operationally simple new synthetic methodologies.
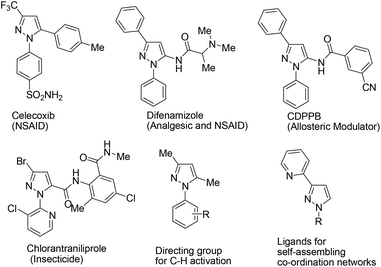 |
| Fig. 1 Representative examples of 1,3-di- and 1,3,5-trisubstituted pyrazoles. | |
The most common methods for the synthesis of pyrazoles involve the condensation of 1,3-dicarbonyl compounds with hydrazines and 1,3-dipolar cycloaddition between diazoalkanes or nitrile imines and olefins or alkynes.6 However, the instability of many 1,3-dicarbonyl compounds, particularly dialdehydes, the explosive nature of 1,3-dipoles and the difficulty in their synthesis and formation of regioisomeric mixtures restrict the access to substituted pyrazoles.7 In addition, the synthesis of unsymmetrical pyrazoles, and in particular C3-arylated N-substituted pyrazoles has remained challenging due to the lack of reactivity at the C3 position.8 To overcome these limitations, post-functionalization of the pyrazole core and metal-catalyzed C–C and/or C–N bond formation strategies have been employed for the regioselective synthesis of substituted pyrazoles.8,9 With the availability of a great variety of synthetic methods, a few reports describe diols as attractive, eco-friendly, readily available and stable building blocks for the construction of regioisomerically pure pyrazoles (Scheme 1).10 For example, Panda et al. disclosed a seminal work on Fe(III)-catalyzed regioselective synthesis of pyrazoles in the presence of an excess amount of diols.10a Later, they were able to synthesize substituted pyrazoles under neat conditions.10c Schmitt and co-workers reported Ru-catalyzed 1,4-disubstituted pyrazoles by reacting hydrazines with 1,3-diols. Recently, diols were also employed for the synthesis of other N-heterocycles.10d–f However, most of the methods reported to date for pyrazole synthesis by employing diols rely on the use of air-sensitive, hygroscopic catalysts as well as oxidants, stoichiometric amounts of ligands, and additives, and they have a narrow substrate scope and often require high temperature. So, the present challenge is to develop a simple and efficient catalytic system for the facile synthesis of regioisomerically pure pyrazoles under ligand-free conditions. In line with our continuing interest in metal-catalyzed C–C and C–heteroatom bond-forming reactions under ligand-free conditions,11 we herein report a straightforward method for the regioselective synthesis of 1,3-di- and 1,3,5-trisubstituted pyrazoles from easily accessible hydrazones and diols by employing inexpensive ceric ammonium nitrate (CAN) as an eco-friendly catalyst under mild conditions.
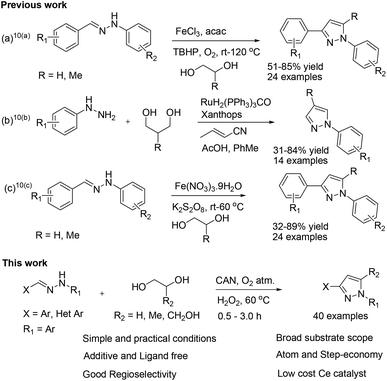 |
| Scheme 1 Synthesis of substituted pyrazoles using diols as a building block. | |
Although the combination of hydrogen peroxide (H2O2) and oxygen (O2) is considered an economical and environmentally friendly oxidant system,12 their applications in catalytic organic transformations are still limited.13 This is because of the radical nature of both hydrogen peroxide and molecular oxygen, which could trap the radical intermediate. Another issue is the generation of extremely reactive oxygen species (ROS) such as hydroxyl radicals (OH˙), peroxo-species (RO2˙) and superoxide anion radicals (O2˙−) which often result in undesired side reactions. These shortcomings may be overcome by using redox-active metal catalysts such as Cu, Fe and Ce under an oxygen atmosphere.14 Further synthesis of N-heterocycles by tandem oxidation of alcohols with the C–C/C–N bond formation strategy has not been explored by employing the Ce-catalyst. CAN which acts both as an oxidant and a Lewis acid has been utilized as a catalyst for alcohol oxidation and N-heterocycle synthesis.15 So, we envisaged the regioselective synthesis of pyrazoles by tandem oxidation/ring cyclization of 1,2-diols with hydrazones employing CAN as a redox-active metal catalyst under an oxygen atmosphere.
To demonstrate the rationality, we started our investigation by reacting diphenyl hydrazone 1a in ethylene glycol. Fortunately, the desired pyrazole 2a was isolated in 8% yield in the presence of 10 mol% CAN as the catalyst under an oxygen atmosphere (Table 1, entry 1). The formation of 1,3-diphenyl pyrazole discloses that ethylene glycol acts as a two-carbon source, which on sequential oxidation followed by ring cyclization with diphenylhydrazone 1a gave product 2a. This interesting finding inspired us to optimize the reaction conditions to provide a general methodology for the regioselective synthesis of substituted pyrazoles, in particular unsymmetrical ones, which are less accessible by traditional methods.7b,8 Then, we investigated the optimization reaction with other cerium catalysts and unfortunately, they were found to be ineffective (Table 1, entries 2 and 3). Next, various oxidants were screened to examine their effects on the efficacy of the reaction. Oxidants such as K2S2O8, oxone and I2 resulted in poor yields of the product (Table 1, entries 4–6). Interestingly, by employing TBHP as an oxidant, the yield of 2a was dramatically improved to 55% (Table 1, entry 7). Other oxidants such as DTBP and TBPB were found to be inferior to TBHP (Table 1, entries 8 and 9). Employing H2O2 as an oxidant and lowering the reaction temperature to 60 °C, the yield of the product was further enhanced to 62% along with a shortened reaction time. To our delight, increasing the amount of CAN resulted in a maximum yield of product 2a (Table 1, entry 12). Further decreasing the reaction temperature as well as increasing the amount of H2O2 resulted in lower yields of the product (Table 1, entries 13 and 14). Additionally, the reaction was found to be sluggish with a poor yield in the absence of H2O2 (Table 1, entry 15). Moreover, carrying out the reaction on a gram scale afforded pyrazole 2a in 65% yield (Table 1, entry 17).
Table 1 Optimization reaction of diphenylhydrazones with ethylene glycola

|
Entry |
Catalyst (mol%) |
Oxidant (equiv.) |
Temp. (°C) |
Time (h) |
Yieldb (%) |
Conditions: 1a (0.51 mmol), ethylene glycol (3.0 mL), oxidant (2.0 equiv.), H2O2 (2.0 equiv., 50%), under an O2 atm.
Isolated yields.
Oxidant (3.0 equiv.).
1a (5.1 mmol), ethylene glycol (20.0 mL), oxidant (2.0 equiv.), H2O2 (2.0 equiv., 50%), under an O2 atm.
|
1 |
CAN (10) |
— |
120 |
6 |
8 |
2 |
CeO2 |
— |
120 |
6 |
— |
3 |
Ce(SO4)2 |
— |
120 |
6 |
5 |
4 |
CAN (10) |
K2S2O8 |
120 |
6 |
12 |
5 |
CAN (10) |
Oxone |
120 |
6 |
14 |
6 |
CAN (10) |
I2 |
120 |
6 |
3 |
7 |
CAN (10) |
TBHP |
120 |
6 |
55 |
8 |
CAN (10) |
DTBP |
120 |
6 |
10 |
9 |
CAN (10) |
TBPB |
120 |
6 |
40 |
10 |
CAN (10) |
H2O2 |
120 |
6 |
50 |
11 |
CAN (10) |
H2O2 |
60 |
0.7 |
62 |
12 |
CAN (15) |
H2O2 |
60 |
0.7 |
82 |
13 |
CAN (15) |
H2O2 |
40 |
0.7 |
40 |
14c |
CAN (15) |
H2O2 |
60 |
0.7 |
75 |
15 |
CAN (15) |
— |
60 |
0.7 |
30 |
16 |
— |
H2O2 |
60 |
0.7 |
— |
17d |
CAN (15) |
H2O2 |
60 |
0.7 |
65 |
With the optimized conditions in hand (Table 1, entry 12), we then explored the scope and limitations of the reaction (Table 2). Ethylene glycol was evaluated with differently substituted hydrazones. Diphenylhydrazones bearing both electron-donating and electron-withdrawing groups such as hydroxy, methyl, methoxy, chloro, bromo, cyano and nitro present on the phenyl ring resulted in the corresponding 1,3-disubstituted pyrazoles in moderate to excellent yields (24–85%). Interestingly, unsymmetrical 1,3-disubstituted pyrazoles were formed with complete regioselectivity (Table 2, 2b–2n, 2p–2t, and 2v–2z). Thus, the electronic nature of the substituents has little influence on product yields (Table 2, 2a–2y). Under the optimum reaction conditions, electron-deficient hydrazones resulted in moderate to good yields of 1,3-disubstituted pyrazoles (Table 2, 2f–2l, 2s, 2u, 2v and 2y) that are of interest in the pharmaceutical industry. It is interesting to note that pyrazole 2f was prepared in good yields that may be en route to the formal synthesis of the non-steroidal anti-inflammatory drug Lonazolac.16 However, thiophene-substituted hydrazones when treated with ethylene glycol resulted in a low yield of product 2m. The possible reason may be due to the formation of a complex between heteroaryl-substituted hydrazone and CAN.17 Moreover, the reaction was unsuccessful with hydrazones derived from pyridine-2-carbaldehyde and pyridine-4-carbaldehyde (Table 2, 2z and 2aa). The reason was unclear.
Table 2 Ce-catalyzed cyclization of hydrazones with ethylene glycola
Unless otherwise mentioned, the reactions were performed with hydrazones 1 (0.51 mmol), H2O2 (1.01 mmol, 50%) and CAN (15 mol%) in ethylene glycol (3.0 mL) at 60 °C under an O2 atm.
Temperature, 80 °C.
|
|
After evaluating the scope of ethylene glycol with hydrazones, substituted diols such as 1,2-propanediol and glycerol were tested.
Gratifyingly, 1,3,5-trisubstituted pyrazoles 3a–3h were obtained in moderate yields when the reaction was carried out with 1,2-propanediol (Table 3). However, reactions of diarylhydrazones with glycerol resulted in the corresponding pyrazole derivatives 3i–3l in low yields. The reason for the low yield may be due to the poor solubility of the substrate under the optimized reaction conditions even at an elevated temperature.18 Competition experiments clearly indicate that reactions of diphenyl hydrazone 1a with ethylene glycol proceeded efficiently compared to those with propane-1,2-diol (Table 1, ESI†).
Table 3 Ce-catalyzed cyclization of hydrazones with alkyl-substituted 1,2-diolsa
Unless otherwise mentioned, the reactions were performed with hydrazones 1 (0.51 mmol), H2O2 (1.01 mmol, 50%) and CAN (15 mol%) in ethylene glycol (3.0 mL) at 60 °C under an O2 atm.
Temperature, 80 °C.
Temperature, 100 °C.
|
|
Finally, the reaction was extended to the one-pot synthesis of pyrazole 2a (Scheme 2). Thus, by employing simple and commercially available starting materials such as phenylhydrazine, benzaldehyde and ethylene glycol under the optimum conditions, 2a was afforded in good yields. This modular and efficient one-pot procedure significantly enhances the usefulness and practicality of the developed method.
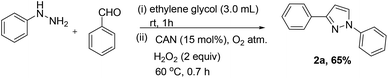 |
| Scheme 2 One-pot synthesis of pyrazoles. | |
A series of experiments were conducted to gain some insights into the reaction pathway and regioselectivity. For example, oxidation of 4-(hydroxymethyl)cyclohexanol was carried out using the Ce catalyst. The reaction afforded 4-(hydroxymethyl)cyclohexanone in 32% yield.19 Similarly, 1-phenylethane-1,2-diols resulted in 2-hydroxy-1-phenylethanone along with some unidentified impurities. These reactions indicate that the Ce catalyst selectively oxidizes the secondary alcoholic group over the primary alcohol under the optimum conditions. Thus, the16b reaction may proceed through a selective oxidation of 1,2-propanediol to 1-hydroxypropan-2-one and a subsequent nucleophilic attack of hydrazone on 1-hydroxypropan-2-one, followed by ring cyclization and aromatization to form the desired pyrazoles.
In conclusion, 1,3-di- and 1,3,5-trisubstituted pyrazoles can be synthesized directly using vicinal diols and hydrazones via a cerium-catalysed tandem oxidation and C–C/C–N bond formation method. A variety of regioisomerically pure pyrazoles were obtained in good to excellent yields. Considering the wide availability of a large variety of starting materials with operational simplicity (reaction conditions, work up and purification), a highly modular and practical synthesis of substituted pyrazoles has been developed. Further investigation of the detailed reaction mechanism and application of diols as dicarbonyl equivalents towards the synthesis of other heterocycles is ongoing in our laboratory.
Conflicts of interest
There are no conflicts to declare.
Acknowledgements
Financial support from SERB (File No. YSS/2014/001017) and UGC (F-30-378/2017 (BSR)) is gratefully acknowledged. AKJ and CKP thank Prof. Niranjan Panda, NIT Rourkela for helping with the characterization. The authors are also thankful to DST-FIST, India (No. SR/FST/CS-1/2017/19) and IDP-OHEPEE (World Bank Sponsored) for funding to develop and repair the instrumentation facility at the Department of Chemistry, Maharaja Sriram Chandra Bhanja Deo University, Baripada, Odisha, India.
References
-
(a) J. V. Faria, P. F. Vegi, A. G. C. Miguita, M. S. Dos Santos, N. Boechat and A. M. R. Bernardino, Recently reported biological activities of pyrazole compounds, Bioorg. Med. Chem., 2017, 25, 5891–5903 CrossRef CAS PubMed;
(b) P. K. Mykhailiuk, Fluorinated pyrazoles: From synthesis to applications, Chem. Rev., 2021, 121, 1670–1715 CrossRef CAS PubMed;
(c) C. Pettinari, A. Taabacaru and S. Galli, Co-ordination polymers and metal organic frameworks based on poly(pyrazole)-containing ligands, Coord. Chem. Rev., 2016, 307, 1–31 CrossRef CAS;
(d) J. Orrego-Hernandez and J. Portilla, Synthesis of dicyanovinyl-substituted 1-(2-Pyridyl)pyrazoles: Design of a fluorescent chemosensor for selective recognition of cyanide, J. Org. Chem., 2017, 82, 13376–13385 CrossRef CAS PubMed.
- P. L. Mc Cormack, Celecoxib: A review of its use for symptomatic relief in the treatment of osteoarthritis, rheumatoid arthritis and ankylosing spondylitis, Drugs, 2011, 71, 2457–2489 CrossRef CAS.
-
(a) N. Toshitaka, Y. Kazumasa and K. Tsutomu, Effects of difenamizole on content of catecholamines and metabolites in mouse brain, Jpn. J. Pharmacol., 1978, 28, 642–646 CrossRef;
(b) D. Secci, A. Bolasco, P. Chimenti and S. Carradori, The state of art of pyrazole derivatives as monoamine oxidase inhibitors and antidepressant/anticonvulsant agents, Curr. Med. Chem., 2011, 18, 5114–5144 CrossRef CAS PubMed.
- M. Sallmann and C. Limberg, Utilizing the trispyrazolyl borate ligand for the mimicking of O2-activating mononuclear nonheme iron enzymes, Acc. Chem. Res., 2015, 48, 2734–2743 CrossRef CAS PubMed.
-
(a) C. Zhang, S. Chen, C.-X. Ye, K. Harms, L. Zhang, K. N. Houk and E. Meggers, Asymmetric photocatalysis by Intramolecular hydrogen-atom transfer in photoexcited catalyst-substrate complex, Angew. Chem., Int. Ed., 2019, 58, 14462–14466 CrossRef CAS;
(b) S. Onodera, S. Ishikawa, T. Kochi and F. Kakiuchi, Direct alkenylation of allylbenzenes via chelation-assisted C-C bond cleavage, J. Am. Chem. Soc., 2018, 140, 9788–9792 CrossRef CAS;
(c) N. Gulia and O. Daugulis, Palladium-catalyzed pyrazole-directed sp3 C-H bond arylation for the synthesis of β-phenethylamines, Angew. Chem., Int. Ed., 2017, 56, 3630–3634 CrossRef CAS.
-
(a) M. V. Patel, R. Bell, S. Majest, R. Henry and T. Kolasa, Synthesis of 4,5-diaryl-1H-pyrazole-3-ol derivatives as potential COX-2 Inhibitors, J. Org. Chem., 2004, 69, 7058–7065 CrossRef CAS;
(b) R. Huisgen, 1,3-Dipolar cycloadditions: past and future, Angew. Chem., Int. Ed. Engl., 1963, 2, 565–632 CrossRef;
(c) K. Mohanan, A. R. Martin, L. Toupet, M. Smietana and J. Vasseur, Three-component reaction using the bestmann-ohira reagent: A regioselective synthesis of phosphonyl pyrazole rings, Angew. Chem., Int. Ed., 2010, 49, 3196–3199 CrossRef CAS.
-
(a) X. Deng and N. S. Mani, Base-mediated reaction of hydrazones and nitroolefins with a reversed regioselective: A novel synthesis of 1,3,4-trisubstituted pyrazoles, Org. Lett., 2008, 10, 1307–1310 CrossRef CAS;
(b) A. Huang, K. Wo, S. Y. C. Lee, N. Kneitschel, J. Chang, K. Zhu, T. Mello, L. Bancroft, N. J. Norman and S.-L. Zheng, Regioselective synthesis, NMR, and crystallographic analysis of N1-substituted pyrazoles, J. Org. Chem., 2017, 82, 8864–8872 CrossRef CAS.
- L. O'Sullivan, K. V. Patel, B. C. Rowley, D. K. Brownsey, E. Gorobets, B. S. Gelfand, J. F. Van Humbeck and D. J. Derksen, Regioselective synthesis of C3-hydroxyarylated pyrazoles, J. Org. Chem., 2022, 87, 846–854 CrossRef PubMed.
-
(a) Y. Lv, J. Meng, C. Li, X. Wang, Y. Ye and K. Sun, Update on the synthesis of N-heterocycles via cyclization of hydrazones (2017–2021), Adv. Synth. Catal., 2021, 363, 5235–5265 CrossRef CAS;
(b) M. Zora and A. Kivrak, Synthesis of pyrazoles via CuI-mediated electrophilic cyclization of α,β-alkynic hydrazones, J. Org. Chem., 2011, 76, 9379–9390 CrossRef CAS;
(c) J. J. Neumann, M. Suri and F. Glorius, Efficient synthesis of pyrazoles: Oxidative C-C/N-N bond formation cascade, Angew. Chem., Int. Ed., 2010, 49, 7790–7794 CrossRef CAS;
(d) Q. Zhang and M. Tang, Regioselective synthesis of highly functionalized pyrazoles from N-Tosylhydrazones, Org. Lett., 2019, 21, 1917–1920 CrossRef CAS PubMed.
-
(a) N. Panda and A. K. Jena, Fe-Catalyzed one-pot synthesis of 1,3-di-and 1,3,5-trisubstituted pyrazoles from hydrazones and vicinal diols, J. Org. Chem., 2012, 77, 9401–9406 CrossRef CAS PubMed;
(b) D. C. Schmitt, A. P. Taylor, A. C. Flick and R. E. Kyne Jr., Synthesis of pyrazoles from 1,3-diols via hydrogen transfer catalysis, Org. Lett., 2015, 17, 1405–1408 CrossRef CAS PubMed;
(c) N. Panda and S. Ojha, Facile synthesis of pyrazoles by iron-catalyzed regioselective cyclization of hydrazone and 1,2-diol under ligand free conditions, J. Organomet. Chem., 2018, 861, 244–251 CrossRef CAS;
(d) J. C. Arango-Daza, C. Lluna-Galán, L. Izquierdo-Aranda, J. R. Cabrero-Antonino and R. Adam, Heterogeneous Pd-catalyzed efficient synthesis of imidazolonones via dehydrogenative condensation between ureas and 1,2-diols, ACS Catal., 2022, 12, 6906–6922 CrossRef CAS;
(e) A. Mondal, M. K. Sahoo, M. Subaramanian and E. Balaraman, Manganese(I)-catalyzed sustainable synthesis of quinoxaline and quinazoline derivatives with the liberation of dihydrogen, J. Org. Chem., 2020, 85, 7181–7191 CrossRef CAS PubMed;
(f) S. Maity, S. K. Gupta and N. Panda, Iron(II)-catalyzed oxidative coupling of vicinal diols and 2-amino-1,4-naphthoquinone for the synthesis of pyrrolonaphthoquinones and furanonaphthoquinones, Asian J. Org. Chem., 2021, 12, 3355–3363 CrossRef.
-
(a) D. Das, A. K. Jena, C. K. Pal, L. Bourda and K. V. Hecke, CuI nanoparticle-catalyzed regioselective synthesis of 3-nitro-2-arylimidazo[1,2-a]pyridines using oxygen as oxidant, Asian J. Org. Chem., 2022, 11, e2021007–e2021011 CrossRef;
(b) C. K. Pal, A. K. Jena, D. Das, S. Sahu and R. K. Singh, Triethanolamine-mediated magnetically separable Fe3O4-Pd nanoparticles catalyzed heck reaction under ligand-free Conditions, ChemistrySelect, 2021, 6, 7944–7949 CrossRef;
(c) C. K. Pal, S. Sahu, R. K. Sahu, R. K. Singh and A. K. Jena, Magnetically recyclable palladium nanoparticles (Fe3O4-Pd) for oxidative coupling between amides and olefins at room temperature, Appl. Organomet. Chem., 2019, 33, e4985–e4993 CrossRef.
-
(a) Y. Yamamoto, M. Ota, S. Kodama, K. Michimoto, A. Nomoto, A. Ogawa, M. Furuya and K. Kawakami, Excellent catalytic performances of Au/C-CuO binary System in the Selective Oxidation of Benzylamines to Imines under atmospheric oxygen, ACS Omega, 2021, 6, 2239–2247 CrossRef CAS;
(b) A. N. Campbell and S. S. Stahl, Overcoming the ‘'oxidant problem”: Strategies to use O2 as the oxidant in organometallic C-H oxidation reactions catalyzed by Pd (and Cu), Acc. Chem. Res., 2012, 45, 851–863 CrossRef CAS.
-
(a) R. Zhao, J. Teng, Z. Wang, W. Dong, J. Lina and C. Chu, Copper on charcoal: Cu0 nanoparticles catalysed aerobic oxidation of α-diazoacetates, Org. Biomol. Chem., 2021, 19, 6120–6126 RSC;
(b) Q. Cao, L. M. Dornan, L. Rogan, L. Hughes and M. J. Muldoon, Aerobic oxidation catalysis with stable radicals, Chem. Commun., 2014, 50, 4524–4543 RSC.
-
(a) M. B. Gawande, A. Goswami, F.-X. Felpin, T. Asefa, X. Huang, R. Silva, X. Zou, R. Zboril and R. S. Varma, Cu and Cu-based nanoparticles: Synthesis and applications in catalysis, Chem. Rev., 2016, 116, 3722–3811 CrossRef CAS and references cited therein;
(b) M. Paul, S. Shirase, Y. Morimoto, L. Mathey, B. Murugesapandian, S. Tanaka, S. Itoh, H. Tsurugi and K. Mashima, Cerium-complex-catalyzed oxidation of arylmethanols under atmospheric pressure of dioxygen and its mechanism through a side-on μ-peroxo dicerium(IV)complex, Chem. – Eur. J., 2016, 22, 4008–4014 CrossRef CAS PubMed.
-
(a) V. Sridharan and J. C. Menendez, Cerium(IV) ammonium nitrate as a catalyst in organic synthesis, Chem. Rev., 2010, 110, 3805–3849 CrossRef CAS PubMed;
(b) J. J. Devery III, P. K. Mohanta, B. M. Casey and R. A. Flowers II, Facile route to tetrasubstituted pyrazoles utilizing ceric ammonium nitrate, Synlett, 2009, 1490–1494 Search PubMed;
(c) B. M. Casey, D. V. Sadasivam and R. A. Flowers II, Mechanistic studies on the CAN-mediated intramolecular cyclization of δ-aryl-β-dicarbonyl compounds, Beilstein J. Org. Chem., 2013, 9, 1472–1479 CrossRef.
-
(a) G. R. Bebernitz, G. Argentieri, B. Battle, C. Brennan, B. Balkan, B. F. Burkey, M. M. Eckhardt, J. Gao, P. Kapa, R. J. Strohschein, H. F. Schuster, M. Wilson and D. D. Xu, The effect of 1,3-diaryl-[1H]-pyrazole-4-acetamides on glucose utilization in ob/ob Mice, J. Med. Chem., 2001, 44, 2601–2611 CrossRef CAS PubMed;
(b) M. Raulf and W. Koenig, Effects of the nonsteroidal anti-inflammatory compounds Lonazolac Ca, indomethacin and NDGA on inflammatory mediator generation and release from various cells, Immunopharmacology, 1990, 19, 103–111 CrossRef CAS PubMed;
(c) R. R. Gaddam, K. Dhuri, Y.-R. Kim, J. S. Jacobs, V. Kumar, Q. Li, K. Irani, R. Bahal and A. Vikram, Substituent effects of N-(1,3-Diphenyl-1H-pyrazol-5-yl)benzamides on positive allosteric modulation of the metabotropic glutamate-5 receptor in rat cortical astrocytes, J. Med. Chem., 2006, 65, 3332–3342 CrossRef.
- J.-N. Zhu, W.-K. Wang, Z.-H. Jin, Q.-K. Wang and S.-Y. Zhao, Pyrrolo[3,4-c] pyrazoles synthesis via copper(I) chloride-catalyzed oxidative coupling of hydrazones to maleimides, Org. Lett., 2019, 21, 5046–5050 CrossRef CAS.
- X. Deng and N. S. Mani, Regioselective synthesis of 1,3,5-tri-and 1,3,4,5-tetrasubstituted pyrazoles from N-arylhydrazones and nitroolefins, J. Org. Chem., 2008, 73, 2412–2415 CrossRef CAS.
- H. Tomioka, K. Oshima and H. Nozaki, Cerium catalyzed selective oxidation of secondary alcohols in the presence of primary ones, Tetrahedron Lett., 1982, 23, 539–542 CrossRef CAS.
|
This journal is © The Royal Society of Chemistry 2023 |
Click here to see how this site uses Cookies. View our privacy policy here.