Curcumin-based molecular probes for fluorescence imaging of fungi†
Received
12th October 2022
, Accepted 10th January 2023
First published on 17th January 2023
Abstract
Fluorescence imaging is a powerful and widely used method to visualize and study living organisms. However, fungi are notoriously difficult to visualize using fluorescence microscopy, given that their cell wall represents a diffusion barrier, and the synthetic organic dyes available are very limited when compared to molecular probes available for other organisms. Moreover, these dyes are usually available in only one colour, preventing co-staining experiments. To fill this gap, curcumin-based molecular probes were designed based on the rationale that curcumin is fluorescent and has moderate toxicity toward fungi, implying its ability to cross the cell wall to reach targets in the intracellular compartments. A family of boron diketonate complexes was synthesized, based on a curcumin backbone, tuning their emission color from blue to red. These probes did not present noticeable toxicity to filamentous fungus and, when applied to their visualization, readily entered the cells and precisely localized in sub-cellular organelles, enabling their visualization.
Introduction
Live-cell imaging is crucial for the study of biological processes, and many efforts have been dedicated to improving this tool. Microscopy has evolved from a traditional white light transmitted microscope to fluorescence and super-resolution microscopy. In parallel, the library of fluorescent probes has increased, including proteins of the green fluorescent protein family, among other probes.1 Molecular probes based on synthetic organic fluorophores have been designed to stain specific molecular targets,2 to get information about the cellular environment or conditions.
Fungi are eukaryotic organisms that morphologically can be assigned to two main groups: unicellular fungi (yeasts) and filamentous fungi (molds). Fungi represent amenable biological models for the study of cell organization, organelle development and function, aging, and pathogenesis, among other applications. However, different from animal cells,3 the fungal cell has a rigid cell wall containing chitin, glucans, or chitosan, which serves as a protective layer.4 Due to the difficulty for molecular probes to cross this barrier, fluorescence imaging of fungi is difficult, and the synthetic organic dyes available are limited.5 Moreover, these dyes are usually available only in one color (calcofluor-white, Mito Tracker™ red, Nile red, DAPI, etc.),6 making co-staining experiments difficult, in which different dyes with different emission colors are used in combination.
Curcumin is a yellow spice found on the rhizome of Curcuma longa (turmeric). In solution, this polyphenolic molecule possesses a strong absorption band around 410 nm,7 and fluorescence emission between 460 and 550 nm.8 Curcumin also shows some phosphorescence in the range of 600 to 800 nm and demonstrates some ability to generate singlet oxygen and oxidize various cell structures, making it a potential photosensitizer.9 Curcumin derivatives have also been studied as potential tools in the diagnosis and treatment of neurodegenerative diseases such as Parkinson's and Alzheimer's.10 Since it has been demonstrated that curcumin accumulates in senile plaques, several derivatives have been synthesized to improve curcumin's spectroscopic properties (Fig. 1) retaining its ability to localize into Aβ aggregates, culminating with near-infrared molecular probes of the CRANAD family.11–13 Curcumin derivatives have also been applied to the staining of lysosomes, as polarity fluorescent probes.14,15 Nevertheless, to the best of our knowledge, they have not been used for imaging fungal subcellular organelles.
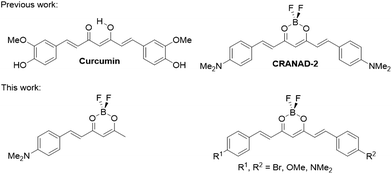 |
| Fig. 1 Curcumin-based molecular probes.16 | |
Here, a family of curcumin-based molecular probes (Fig. 1) has been designed, prepared and studied. They were applied to the staining of a filamentous fungus (Fusarium oxysporum) as a proof-of-concept, demonstrating that the probes enter the hyphae and selectively accumulate in particular intracellular compartments. These molecular probes therefore overcome the low uptake limitations of organic dyes by fungi, and open the way to the design of brighter molecular probes for live fungi imaging.
Results and discussion
Synthesis
In 1964, Pabon17 described an easy route for the synthesis of curcumin, which has been extensively used for the synthesis of curcumin derivatives.18–20 The synthetic strategy reported here is based on a modification previously reported,19 and is described in Scheme 1. The synthesis starts with the complexation of acetylacetone with boron difluoride, to produce complex 1. Then, one equivalent of aldehyde is reacted to form compound 2a, or two equivalents to form compounds 3a–c. If two different aldehydes are added (one equivalent of each), then compounds 3d–f are obtained. The purification of these compounds was difficult due to their low solubility and the presence of symmetric compounds in the cases of 3d–f, which explain the low yields obtained. Trying to run the aldol condensation in a stepwise manner, for example isolating compound 2a and reacting it again with a different aldehyde, did not produce the desired compound and the unreacted starting material was recovered. In the synthesis of derivative 4, curcumin (Cur) was directly subjected to complexation with boron trifluoride. All new compounds were fully characterized (see the ESI†).
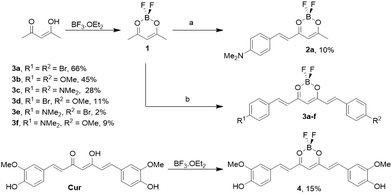 |
| Scheme 1 Synthesis of different curcumin analogues. One equivalent (a) or two equivalents (b) of aldehyde were used. | |
Single crystals suitable for X-ray diffraction were successfully grown from slow evaporation of a saturated tetrahydrofuran solution of 3b. In the process, hydrolysis of the boron complex also yielded single crystals of the free ligand,21 which were suitable for X-ray diffraction. The crystal structures of both compounds are shown in Fig. 2, without the disordered hydrogen or solvent molecule, for clarity.22 All bond lengths and angles are in the normal ranges.23
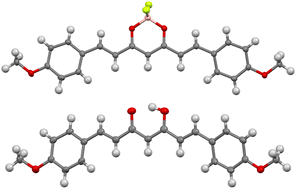 |
| Fig. 2 Crystal structure of 3b (top) and the free ligand (bottom), obtained by single crystal X-ray diffraction.22 Thermal ellipsoids are shown at the 50% probability level, hydrogen atoms are shown with an arbitrary radius (0.30 Å). C, grey; H, white; O, red; F, yellow; B, pink. | |
Photophysical properties
Absorption and emission spectra of curcuminoid derivatives 3c and 4 were recorded in dichloromethane and used as standards for the emission quantum yield calculation.18,24 The compounds are insoluble in aqueous solutions and in hexane; therefore the absorption and emission spectra of all the curcuminoid derivatives obtained were recorded in tetrahydrofuran (THF) (Fig. 3 and 4, respectively), in acetone and in methanol (see the ESI†) and their photophysical properties are presented in Table 1. The low solubility of the compounds in methanol prevented the measurement of absorption coefficient.
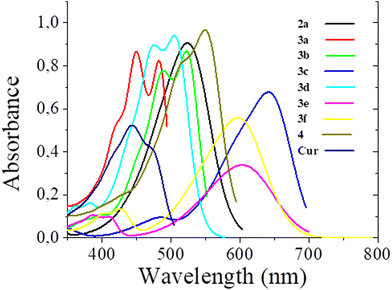 |
| Fig. 3 Absorption spectra of 2a, 3a–f, 4 and Cur in THF. | |
 |
| Fig. 4 Emission spectra of 2a, 3a–f, 4 and Curcumin in THF. | |
Table 1 Photophysical properties of 2a, 3a–f, 4 and Curcumin in different solvents
|
Tetrahydrofuran |
Acetone |
Methanol |
Dye |
λ
abs (nm) |
ε (M−1 cm−1) |
ΔvST (cm−1) |
λ
em (nm) |
φ
f
|
Brightness (M−1 cm−1) |
λ
abs (nm) |
ε (M−1 cm−1) |
λ
em (nm) |
φ
f
|
λ
abs (nm) |
λ
em (nm) |
φ
f
|
Standard: 4, φf = 0.58 (58%) in dichloromethane.
Standard: 3c, φf = 0.47 (47%) in dichloromethane.
|
2a
|
485 |
52 300 |
2666 |
557 |
0.06a |
34 000 |
490 |
7700 |
575 |
<0.01a |
491 |
568 |
<0.01a |
3a
|
453 |
30 000 |
889 |
472 |
0.35a |
10 500 |
452 |
53 200 |
479 |
0.56a |
424 |
488 |
0.46a |
3b
|
484 |
86 000 |
1319 |
517 |
0.69a |
60 000 |
484 |
92 800 |
530 |
0.98a |
470 |
545 |
0.87a |
3c
|
576 |
56 000 |
1463 |
629 |
0.60b |
33 000 |
588 |
79 900 |
668 |
0.07b |
587 |
690 |
0.04b |
3d
|
470 |
64 000 |
2655 |
537 |
0.60a |
38 000 |
469 |
32 000 |
547 |
0.90a |
465 |
551 |
0.57a |
3e
|
546 |
34 000 |
2492 |
632 |
0.10b |
3400 |
558 |
42 700 |
696 |
<0.01b |
553 |
671 |
<0.01b |
3f
|
542 |
58 000 |
2476 |
626 |
0.08b |
4500 |
551 |
63 300 |
681 |
<0.01b |
550 |
667 |
<0.01b |
4
|
505 |
39 000 |
1619 |
550 |
0.44a |
17 000 |
501 |
50 400 |
570 |
0.08a |
500 |
589 |
<0.01a |
Curcumin
|
423 |
53 000 |
2764 |
479 |
0.35a |
18 500 |
420 |
61 100 |
503 |
0.30a |
418 |
536 |
<0.01a |
The maximum absorption of curcumin derivatives varies between 418 nm and 576 nm, with a very small shift between the different solvents. Firstly, it can be noticed that the complexation with boron induces a red-shift of the absorption, as it has been observed before.25 In the series of boron complexes 3a–f and 4, the effect of the electron donating substituents is of shifting the absorption maximum toward longer wavelengths. A similar effect is observed for the emission spectra. The maximum emission varies between 472 nm and 696 nm, and the more the electron donating groups introduced, the higher red-shifted the emission.
According to the results described in Table 1, compounds with strong electron-donating substituents have a higher molar absorptivity and a higher emission quantum yield. The emission quantum yields of the compounds vary between 6% and 98%, and fluorophores 3b–d show a marked difference. This could be explained by the presence of the electron-donating groups that increase the push–pull character of the dyes.
Both the brightness and the emission wavelengths of the dyes reported here are in the same range as the best molecular probes currently used.1
Toxicity and biological imaging
The toxicity of the dyes 2a, 3a–f, 4 and Curcumin was assessed by measuring the mycelial growth over 7 days at 25 °C (Fig. 5). Since curcumin has been shown to be a potential photosensitizer for antimicrobial photodynamic therapy,26 the cytotoxicity was assessed in a dark environment to avoid potential photosensitization of Fusarium oxysporum by curcumin derivatives. As curcumin and its derivatives have not been reported as toxic in the dark, we did not expect the compounds reported here to be toxic, and only one (relatively high) concentration was tested here.
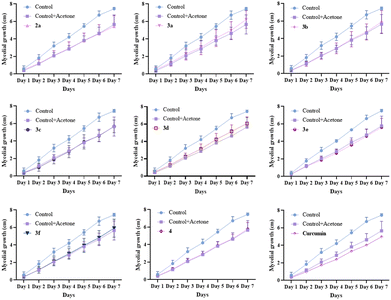 |
| Fig. 5 Radial mycelium growth of Fusarium oxysporum over 7 days, in the dark, under basal conditions (Control), in the presence of acetone (Control+Acetone) and in the presence of a solution of fluorophore in acetone at a concentration of 100 μM (2a, 3a–f, 4, Curcumin). The values correspond to the mean of 3 independent assays, with biological 3 replicates each, and the error bars represent the standard deviation. | |
The trend is similar for all dyes. In a control experiment, the addition of acetone without any dyes slowed down the growth of the mycelium, but did not prove to be acutely toxic. The addition of dyes (in acetone solution) resulted a delay in growth similar to that observed with acetone alone, indicating that for the tested concentrations, the dyes were not toxic in the dark.
According to the literature, the concentration for imaging fungi ranges from a few nM up to 1 mM, depending on the dyes used.27 As the dyes did not prove toxic for a concentration of 100 μM, we decided to use this concentration. Therefore, for the assessment of the performance of the dyes as molecular fluorescent probes, the mycelium was incubated 6 hours with the dye at a final concentration of 100 μM and lyophilized after removing the dye solution (see the ESI†). This relatively long incubation time allowed for most of the dyes to enter the cells, therefore making the washing process unnecessary. The hyphae were then visualized by confocal microscopy (Fig. 6). The probes were excited close to their absorption maximum, and the emission was screened in the blue, green and red parts of the visible spectrum (see the ESI†).
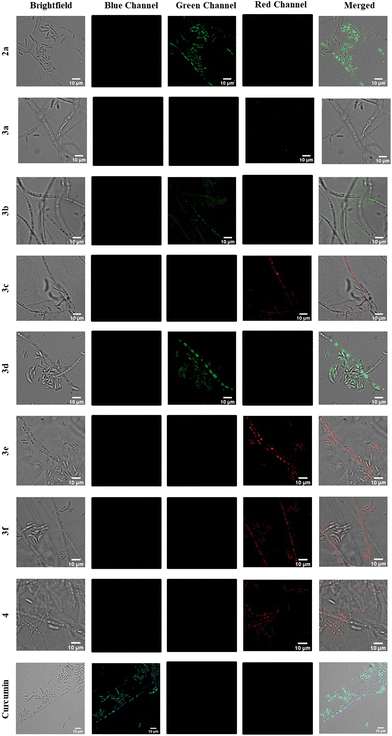 |
| Fig. 6 Images of fungi stained with the dyes (concentration 100 μM, 6 hours) under a confocal microscope. Excitation close to the absorption maximum (405 nm for 3a and Curcumin; 488 nm for 2a, 3b, 3d; 514 nm for 4; 561 nm for 3c, 3e, 3f), and the emission was recorded in an interval corresponding to the emission maximum of each fluorophore (503–654 nm for 2a; 443–592 nm for 3a; 497–654 nm for 3b; 571–731 nm for 3c; 503–654 nm for 3d; 582–735 nm for 3e; 582–735 nm for 3f; 518–678 nm for 4; 438–600 nm for Curcumin) corresponding to the blue, green or red part of the spectrum. | |
Almost all probes entered the fungal cells, and seemed to selectively accumulate in sub-cellular compartments. Only the dye 3a could not be seen in the images, inside or outside the cells. Curcumin emitted in the blue part of the spectrum, similarly to 3a (Table 1), and distinctively accumulated in sub-cellular organelles from where it emits brightly. The fluorescence of compounds 2a, 3b and 3d was recorded in the green part of the spectrum, with 3d giving the brightest intensity in that spectral interval. Compounds 3c,e,f and 4 emission could be collected in the red part of the spectrum. All dyes entering the cells seem to accumulate in the same organelles, depending on the morphology of the fungal cells observed, demonstrating the similarity of the targeting properties of the dyes. As the dyes localized precisely, and gave images without background noise, we did not test other concentrations, but lower concentrations could possibly also give a good staining.
Conclusions
A series of curcumin-based fluorescent dyes were successfully prepared, and all derivatives proved to be emissive in dilute solution. Their emission colour was tuned from 470 nm to 690 nm. They were applied to the staining of the model fungus – Fusarium oxysporum – and demonstrated to be non-toxic while most of them entered the cells. They selectively accumulate in sub-cellular compartments, and allow their visualization using different filter sets, depending on the dye used. Common dyes used to stain fungi are usually available in only one colour, restricting their use in co-staining experiments. These molecular probes are therefore promising, as they could be used in combination with other probes to visualize and study the morphology and dynamics of compartments in fungal cells.
Author contributions
Conceptualization: S. G., Â. C., R. N. S.; data curation: J. R. M. F., B. S.; formal analysis: J. R. M. F., M. A., B. S.; funding acquisition: S. I. V., A. M. S. S., Â. C., S. G.; investigation: J. R. M. F., B. S., S. G., R. N. S.; supervision: S. I. V., A. M. S. S., S. G., Â. C., R. N. S.; writing – original draft: J. R. M. F., S. G., R. N. S.; writing – review & editing: all authors.
Conflicts of interest
There are no conflicts to declare.
Acknowledgements
Thanks are due to University of Aveiro, FCT/MCTES, Centro 2020 and Portugal 2020, the COMPETE program, and the European Union (FEDER program) for the financial support to the LAQV-REQUIMTE (UIDB/50006/2020), to the CICECO-Aveiro Institute of Materials (UID/CTM/50011/2019, UIDB/50011/2020 & UIDP/50011/2020), to the IBiMED (UID/BIM/04501/2013, UID/BIM/04501/2019 – POCI-01-0145-FEDER-007628), to the CESAM (UIDP/50017/2020, UIDB/50017/2020, LA/P/0094/2020), to the iBiMED/UA LiM facility through PPBI (POCI-01-0145-FEDER-022122), to the Portuguese NMR Network, and to the projects PAGE “Protein aggregation across the lifespan” (CENTRO-01-0145-FEDER-000003, RNS research contract – BPD/UI98/6327/2018) and HALIUS “Halotolerant rhizobacteria for enhanced tolerance of Olea europaea to saline stress and infection” (PTDC/BIA-MIC/3157/2020). SG is supported by national funds (OE), through FCT, I.P., in the scope of the framework contract foreseen in the numbers 4, 5, and 6 of the article 23, of the Decree-Law 57/2016, of August 29, changed by Law 57/2017, of July 19. JRMF thanks the FCT for her PhD grant (UI/BD/151272/2021).
References
- L. D. Lavis and R. T. Raines, Bright Ideas for Chemical Biology, ACS Chem. Biol., 2008, 3, 142–155 CrossRef CAS PubMed.
- P. Gao, W. Pan, N. Li and B. Tang, Fluorescent probes for organelle-targeted bioactive species imaging, Chem. Sci., 2019, 10, 6035–6071 RSC.
- S. I. Vieira, R. Nunes Da Silva, M. Alves, R. A. Dias, A. M. Meireles Sousa, F. Camões, A. Maia, M. Almeida, J. Rocha, A. M. S. Silva and S. Guieu, Liprobe, a vital dye for lipid aggregates detection in imaging and highcontent screens, Front. Photonics, 2022, 3, 963778 CrossRef.
-
V. Farkaš, in Fungal protoplasts, ed. J. F. Peberdy and L. Ferenczy, Taylor & Francis, Boca Raton, 2020 Search PubMed.
- S. Brul, J. Nussbaum and S. K. Dielbandhoesing, Fluorescent probes for wall porosity and membrane integrity in filamentous fungi, J. Microbiol. Methods, 1997, 28, 169–178 CrossRef CAS.
- A. Lichius, Concentration, cellular exposure and specificity of organelle selective fluorescent dyes in fungal cell biology, Fungal Biol. Rev., 2022, 41, 45–51 CrossRef CAS.
- F. Zsila, Z. Bikádi and M. Simonyi, Unique, pH-dependent biphasic band shape of the visible circular dichroism of curcumin–serum albumin complex, Biochem. Biophys. Res. Commun., 2003, 301, 776–782 CrossRef CAS PubMed.
- K. I. Priyadarsini, Photophysics, photochemistry and photobiology of curcumin: Studies from organic solutions, bio-mimetics and living cells, J. Photochem. Photobiol., C, 2009, 10, 81–95 CrossRef CAS.
- C. F. Chignell, P. Bilskj, K. J. Reszka, A. G. Motten, R. H. Sik and T. A. Dahl, Spectral and photochemical properties of curcumin, Photochem. Photobiol., 1994, 59, 295–302 CrossRef CAS PubMed.
- T. Sato, M. Hotsumi, K. Makabe and H. Konno, Design, synthesis and evaluation of curcumin-based fluorescent probes to detect Aβ fibrils, Bioorg. Med. Chem. Lett., 2018, 28, 3520–3525 CrossRef CAS PubMed.
- R. Chongzhao, X. Xiaoyin, S. B. Raymond, B. J. Ferrara, K. Neal, B. J. Bacskai, Z. Medarova and A. Moore, Design, Synthesis, and Testing of Difluoroboron-Derivatized Curcumins as Near-Infrared Probes for in Vivo Detection of Amyloid-β Deposits, J. Am. Chem. Soc., 2009, 131, 15257–15261 CrossRef PubMed.
- X. Zhang, Y. Tian, Z. Li, X. Tian, H. Sun, H. Liu, A. Moore and C. Ran, Design and Synthesis of Curcumin Analogues for in Vivo Fluorescence Imaging and Inhibiting Copper-Induced Cross-Linking of Amyloid Beta Species in Alzheimer's Disease, J. Am. Chem. Soc., 2013, 135, 16397–16409 CrossRef CAS PubMed.
- J. Yang, R. Cheng, H. Fu, J. Yang, M. Kumar, J. Lu, Y. Xu, S. H. Liang, M. Cui and C. Ran, Half-curcumin analogues as PET imaging probes for amyloid beta species, Chem. Commun., 2019, 55, 3630–3633 RSC.
- L. Hu, D. Shi, X. Li, J. Zhu, F. Mao, X. Li, C. Xia, B. Jiang, Y. Guo and J. Li, Curcumin-based polarity fluorescent probes: Design strategy and biological applications, Dyes Pigm., 2020, 177, 108320 CrossRef CAS.
- D. Shi, L. Hu, X. Li, W. Liu, Y. Gao, X. Li, B. Jiang, C. Xia, Y. Guo and J. Li, Lysosomal polarity increases with aging as revealed by a lysosome-targetable near-infrared fluorescent probe, Sens. Actuators, B, 2020, 319, 128302 CrossRef CAS.
- M. Chen, Z.-Y. Du, X. Zheng, D.-L. Li, R.-P. Zhou and K. Zhang, Use of curcumin in diagnosis, prevention, and treatment of Alzheimer's disease, Neural Regener. Res., 2018, 13, 742–752 CrossRef CAS PubMed.
- H. J. J. Pabon, A synthesis of curcumin and related compounds, Recl. Trav. Chim. Pays-Bas, 1964, 83, 379–386 CrossRef CAS.
- G. Bai, C. Yu, C. Cheng, E. Hao, Y. Wei, X. Mu and L. Jiao, Syntheses and photophysical properties of BF2 complexes of curcumin analogues, Org. Biomol. Chem., 2014, 12, 1618–1626 RSC.
- K. Liu, J. Chen, J. Chojnacki and S. Zhang, BF3·OEt2-promoted concise synthesis of difluoroboron-derivatized curcumins from aldehydes and 2,4-pentanedione, Tetrahedron Lett., 2013, 54, 2070–2073 CrossRef CAS PubMed.
- W. Wichitnithad, U. Nimmannit, S. Wacharasindhu and P. Rojsitthisak, Synthesis, Characterization and Biological Evaluation of Succinate Prodrugs of Curcuminoids for Colon Cancer Treatment, Molecules, 2011, 16, 1888–1900 CrossRef CAS PubMed.
- V. A. Stepanova, A. Guerrero, C. Schull, J. Christensen, C. Trudeau, J. Cook, K. Wolmutt, J. Blochwitz, A. Ismail, J. K. West, A. M. Wheaton, I. A. Guzei, B. Yao and A. Kubatova, Hybrid Synthetic and Computational Study of an Optimized, Solvent-Free Approach to Curcuminoids, ACS Omega, 2022, 7, 7257–7277 CrossRef CAS PubMed.
- Crystallographic data for the structures reported in this paper have been deposited with the Cambridge Crystallographic Data Centre as supplementary publication No. CCDC 2161374 and 2161375.†.
- F. H. Allen, O. Kennard, D. G. Watson, L. Brammer, A. G. Orpen and R. Taylor, Tables of Bond Lengths determined by X-Ray and Neutron Diffraction. Part I. Bond Lengths in Organic Compounds, J. Chem. Soc., Perkin Trans. 2, 1987, 1–19 RSC.
- A. Chaicham, S. Kulchat, G. Tumcharern, T. Tuntulani and B. Tomapatanaget, Synthesis, photophysical properties, and cyanide detection in aqueous solution of BF2-curcumin dyes, Tetrahedron, 2010, 66, 6217–6223 CrossRef CAS.
- K. Kamada, T. Namikawa, S. Senatore, C. Matthews, P. F. Lenne, O. Maury, C. Andraud, M. Ponce-Vargas, B. le Guennic, D. Jacquemin, P. Agbo, D. D. An, S. S. Gauny, X. Liu, R. J. Abergel, F. Fages and A. D'Aléo, Boron Difluoride Curcuminoid Fluorophores with Enhanced Two-Photon Excited Fluorescence Emission and Versatile Living-Cell Imaging Properties, Chem. – Eur. J., 2016, 22, 5219–5232 CrossRef CAS PubMed.
- L. D. Dias, K. C. Blanco, I. S. Mfouo-Tynga, N. M. Inada and V. S. Bagnato, Curcumin as a photosensitizer: From molecular structure to recent advances in antimicrobial photodynamic therapy, J. Photochem. Photobiol., C, 2020, 45, 100384 CrossRef CAS.
-
P. C. Hickey, S. R. Swift, M. Gabriela Roca and N. D. Read, Live-cell Imaging of Filamentous Fungi Using Vital Fluorescent Dyes and Confocal Microscopy, Methods in Microbiology, Academic Press, 2004, vol. 34, pp. 63–87, ISSN 0580-9517, ISBN 9780125215343 Search PubMed.
Footnote |
† Electronic supplementary information (ESI) available: Synthetic procedures and characterization of the new compounds, details of crystal structure determination, description of the fungi culture and evaluation of the toxicity of the probes, and fluorescence microscopy protocols. CCDC 2161374 and 2161375. For ESI and crystallographic data in CIF or other electronic format see DOI: https://doi.org/10.1039/d2ob01872a |
|
This journal is © The Royal Society of Chemistry 2023 |
Click here to see how this site uses Cookies. View our privacy policy here.