DOI:
10.1039/D3MD00286A
(Research Article)
RSC Med. Chem., 2023,
14, 2277-2300
α-Aminophosphonate inhibitors of metallo-β-lactamases NDM-1 and VIM-2†
Received
22nd June 2023
, Accepted 31st July 2023
First published on 2nd August 2023
Abstract
The upswing of antibiotic resistance is an escalating threat to human health. Resistance mediated by bacterial metallo-β-lactamases is of particular concern as these enzymes degrade β-lactams, our most frequently prescribed class of antibiotics. Inhibition of metallo-β-lactamases could allow the continued use of existing β-lactam antibiotics, such as penicillins, cephalosporins and carbapenems, whose applicability is becoming ever more limited. The design, synthesis, and NDM-1, VIM-2, and GIM-1 inhibitory activities (IC50 4.1–506 μM) of a series of novel non-cytotoxic α-aminophosphonate-based inhibitor candidates are presented herein. We disclose the solution NMR spectroscopic and computational investigation of their NDM-1 and VIM-2 binding sites and binding modes. Whereas the binding modes of the inhibitors are similar, VIM-2 showed a somewhat higher conformational flexibility, and complexed a larger number of inhibitor candidates in more varying binding modes than NDM-1. Phosphonate-type inhibitors may be potential candidates for development into therapeutics to combat metallo-β-lactamase resistant bacteria.
Introduction
Antimicrobial resistance is a major threat to human health that already causes over a million deaths annually.1 It is expected to become the leading cause of mortality by 2050.2 Out of the multiple resistance mechanisms that bacteria possess,3 metallo-β-lactamase mediated degradation pathways are currently the most worrisome4 as they render β-lactam antibiotics inactive (Fig. 1),5 including also carbapenems, a class of drugs of last-resort.6,7 Historically, β-lactams constitute the most widely used, cheapest to produce and most prescribed class of antibiotics.4,8 The New Delhi metallo-β-lactamase 1 (NDM-1)9 and the Verona integron-encoded metallo-β-lactamase 2 (VIM-2)10 are of particular concern as they are commonly found in clinical isolates and are spread worldwide.11 The transmutation of the plasmid-borne β-lactamase genes (bla) between Gram-negative bacteria12 made blaNDM-1 the predominant β-lactamase in Europe and endemic in the Indian subcontinent.4
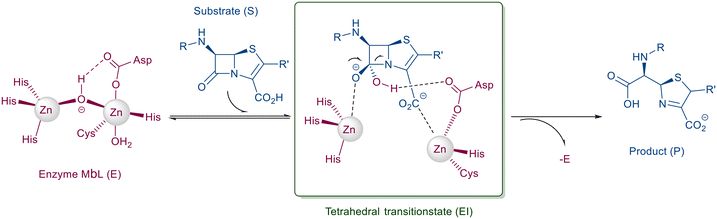 |
| Fig. 1 The schematic mechanism of β-lactam hydrolysis facilitated by metallo-β-lactamases.5 | |
The mutation rate of metallo-β-lactamases outnumbers that of antibiotic discovery by far, leading to a rapid increase in the number of bacteria insensitive to β-lactam antibiotics. Out of the 7279 β-lactamases reported so far, 38 are NDM-1 and 74 are VIM-2 variants,13 with further β-lactamases being continuously identified.14
Besides the design of novel β-lactams reluctant to hydrolysis by metallo-β-lactamases, the development of metallo-β-lactamase inhibitors is the most promising strategy to counteract metallo-β-lactamase-based antibiotic resistance.15 These inhibitors, when combined with antibiotics and formulated into a single product, may prolong the usefulness of existing β-lactam antibiotics. This strategy was demonstrated to be successful by the introduction of serine-β-lactamase inhibitors, such as tazobactam16 and clavulanic acid.17
Metallo-β-lactamases do not share an evolutionary relationship with serine-β-lactamases. They possess different structures, active sites and catalytic mechanisms. Consequently, serine-β-lactamase inhibitors are inactive against metallo-β-lactamases, except for the recently developed taniborbactam.18 Captopril derivatives, thiorphan, tiopronin, 2,3-dimercaprol, ML302F, L-CS319, 2-(4-fluorobenzoyl)benzoic acid, 3-formylchromone, cyclic boronates and ANT-2681 are examples of compounds that act by ligand replacement, whereas SIT-Z5 and aspergillomarasmine A19 act by metal sequestration. These are both Zn-dependent strategies.20,21 Schofield's isoquinolines (IC50 10–1000 μM)22 are the most successful Zn-independent inhibitors. Most inhibitors discovered so far have IC50s in the μM range, although a few compounds with nM potency have also been reported.23 The field has been extensively reviewed.24–27 Importantly, no clinically applicable metallo-β-lactamase inhibitors are available to date, and merely two candidates have yet been reported to have reached clinical trials.8 Consequently, the development of new classes of metallo-β-lactamase inhibitors that provide protection to some of our clinically most precious antibiotics is expected to be one of the possibly most valuable contributions to combating antibiotic resistance.28
Results and discussion
Design
Mimetics of the tetrahedral transition state of β-lactam hydrolysis29 (Fig. 1) are expected to yield potential metallo-β-lactamase inhibitors. The active site of NDM-1 and of VIM-2 are structurally similar (Fig. 2, and section S3 of ESI†), and involve the same amino acid residues to facilitate zinc coordination. In addition, both enzymes use flexible loops to allow for adjustment of the active site to enable the binding of a wide range of β-lactam antibiotics.
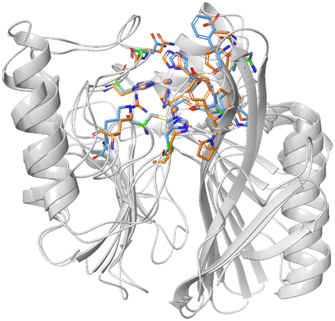 |
| Fig. 2 Superimposed structures of NDM-1 (blue, pdb: 6NY7) and VIM-2 (orange, pdb: 6O5T).29 The amino acid residues that are most important for binding of β-lactam antibiotics are highlighted to indicate the conserved part of the active site. | |
In our design of novel inhibitors, we introduced a phosphonic acid and a piperidine ring as key binding motives (Scheme 1). Phosphonic acid derivatives30 and phosphonamidates31 have previously been studied in the context of metallo-β-lactamase inhibition. The phosphonate group was incorporated to mimic the amide carbonyl of β-lactams and is expected to coordinate the zinc ions of metallo-β-lactamases.32,33 The piperidine possibly interacts with the catalytic site of metallo-β-lactamases. Initially, phospholactams (I) showed low stability in aqueous solutions in preliminary experiments,34 so we turned to their chemically stable analogues II, as their tetrahedral phosphonate motif resembles the tetrahedral transition state of hydrolysed β-lactams (Scheme 1). The phosphonic acid core is thus expected to interact within the active site, by strongly coordinating the zinc ions with its oxygens, analogous to that reported for structurally related phosphonate-based metallo-β-lactamase inhibitors (see for example pdb: 5HH6).32,35
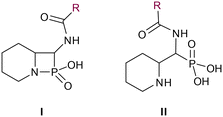 |
| Scheme 1 Piperidine substituted phospholactams and α-aminophosphonates are expected to bind metallo-β-lactamases as the geometry of their P O motif resembles the tetrahedral intermediate of β-lactam hydrolysis. | |
We further introduced thiophene moieties with variation in their regioisomeric pattern, steric bulk and flexibility (Scheme 2) as sulfur substitution is common in several classes of β-lactams (penams, penems, cephems, etc.). The (thiophene-2-yl)acet-amido and thiophene moieties, which are bioisosteres of benzyl and phenyl groups, respectively,36 were anticipated to interact with the hydrophobic and aromatic amino acid residues of loop 3 (Phe70/Tyr65). These sidechains vary in hydrophobicity, which we deemed worth exploring. In SAR studies of β-lactamase inhibitors, the properties of aromatic moieties were found important as they contribute to crucial π–π interactions.37 The structural variation of the aromatic moiety in the present series is also expected to affect the solubility of the compounds.
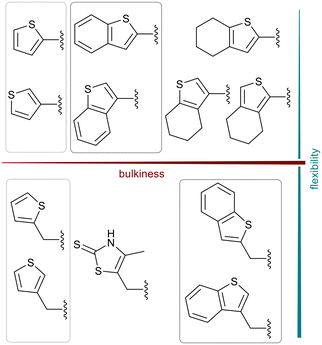 |
| Scheme 2 Thiophenes with varying regioisomer patterns, steric bulk, polarity and flexibility have been used as the “R”-substituents (Scheme 1) of the α-aminophosphonate-type metallo-β-lactamase inhibitors developed herein. | |
Synthesis
To synthesize 5a–l, we used a pathway (Scheme 3) that relies on the hafnium chloride catalyzed Kabachnik–Fields reaction,38 which simultaneously introduces the phosphonic acid and the amine functional groups, in its key first step. Next, the benzyl protecting group of 2 was hydrogenated under mild conditions using Pearlman's catalyst,39 Pd(OH)2/C and H2 under atmospheric pressure, yielding 3. Subsequently, a variety of amides 4a–l were generated from this intermediate with a range of carboxylic acids (Scheme 3). Out of the variety of coupling reagents and solvents examined, HATU in combination with dry EtOAc as solvent provided the highest yields.40 The diastereomers of 4a–l were separated using preparative HPLC, and one set of enantiomeric pairs was crystalized to identify the relative configuration of the intermediates by single-crystal X-ray diffraction (section 5, ESI†). The phosphonic ester was then hydrolyzed with McKenna's method using TMSBr, resulting in simultaneous Boc-deprotection of 4a–l. However, it was observed that ethyl bromide liberated as a side product led to unwanted N-alkylation of the target compounds 5a–l. This could be avoided by the addition of Et3N in combination with 3-mercaptopropyl functionalized silica gel to the reaction mixture. To enhance the aqueous solubility, the final products were converted to their hydrochloric salts (5a–l). In our hands, the compounds were chemically stable in solution for a prolonged time (weeks to months, Fig. S187–S192, ESI†).
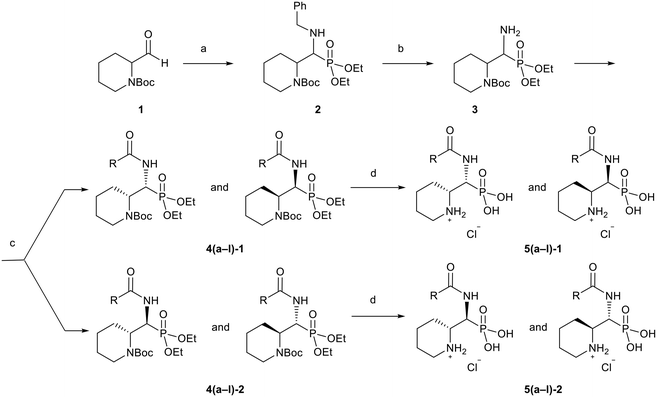 |
| Scheme 3 Synthesis of the target compounds. Reagents and conditions: (a) BnNH2, HP(O)(OEt)2, HfCl4 (cat), EtOH, 60 °C, 12 h, 32%; (b) H2 (1 atm), Pd(OH2)/C (cat), EtOH, r.t., 16 h, 99%; (c) R–COOH, HATU, DIPEA, dry EtOAc, r.t., 12–24 h, 60–80%; (d) TMSBr, 3-mercaptopropyl functionalized silica gel, Et3N, MeCN, r.t., 12–48 h, 19–94%. For the full structures of 5(a–l), and hence of the R-groups, see Table 1 below. | |
Metallo-β-lactamase inhibition and cytotoxic activities
The 24 synthesized compounds 5a–l were tested for their inhibitory activities against NDM-1, VIM-2 (Table 1), and GIM-1 in an in vitro enzymatic assay (ESI†).41 The inhibitory activity was measured using enzyme concentrations of 0.1 nM VIM-2, 10 nM NDM-1 or 1 nM GIM-1, with an excess Zn(II) (100 μM, see Experimental methods), with inhibitor compounds with concentrations from 0 to 800 μM. Each data point was measured in triplicates. Almost all compounds showed metallo-β-lactamase inhibitory activities with 5g-2 being the most active (IC50 = 4.1 μM, VIM-2). A larger number of compounds inhibited VIM-2 than NDM-1 or GIM-1, and those with RR/SS configuration typically inhibited VIM-2 at lower concentrations. Our observation that a larger number of compounds inhibited VIM-2 than NDM-1 may be unexpected as both enzymes belong to the B1-subclass of metallo-β-lactamases. This is in line with previous reports.42,43 In general, the more rigid compounds showed higher affinity (compare 5b to 5j, for instance). Accordingly, upon introduction of a flexible methylene group, inhibitors with a 3-thiophene ring showed a 10-fold reduction of activity, whilst 2-thiophene substituted ones showed up to 45-fold decreased activity. Importantly, none of the compounds showed cytotoxicity against human MCF-7 or HepG cells (section 2, ESI†).
Table 1 The NDM-1 and VIM-2 inhibitory activities of compounds 5a–l
No. |
Compound |
Stereochemistrya |
Enzyme IC50c (μM) |
NDM-1 |
VIM-2 |
The compounds were separated as diastereomeric pairs, which are shown here with the stereocenters being highlighted with *.
N.I. means no enzyme inhibitory activity was observed.
IC50 values were calculated from 3 parallel measurements.
|
5a-1
|
|
SR/RS |
N.I.b |
353 ± 62 |
5a-2
|
RR/SS |
N.I. |
N.I. |
5b-1
|
|
SR/RS |
230 ± 28 |
118 ± 60 |
5b-2
|
RR/SS |
N.I. |
7.2 ± 0.4 |
5c-1
|
|
SR/RS |
219 ± 14 |
33 ± 3 |
5c-2
|
RR/SS |
N.I. |
14.6 ± 2.3 |
5d-1
|
|
SR/RS |
N.I. |
244 ± 35 |
5d-2
|
RR/SS |
N.I. |
4.5 ± 0.8 |
5e-1
|
|
SR/RS |
76 ± 12 |
69.7 ± 26.2 |
5e-2
|
RR/SS |
N.I. |
46.1 ± 15.6 |
5f-1
|
|
SR/RS |
N.I. |
37 ± 3 |
5f-2
|
RR/SS |
N.I. |
18.8 ± 0.5 |
5g-1
|
|
SR/RS |
46.7 ± 9.7 |
45 ± 5 |
5g-2
|
RR/SS |
46.8 ± 5.5 |
4.1 ± 2.1 |
5h-1
|
|
SR/RS |
N.I. |
40.6 ± 3.9 |
5h-2
|
RR/SS |
N.I. |
11.6 ± 4.7 |
5i-1
|
|
SR/RS |
296 ± 87 |
N.I. |
5i-2
|
RR/SS |
271 ± 34 |
328 ± 116 |
5j-1
|
|
RS/SR |
506 ± 333 |
319 ± 69 |
5j-2
|
RR/SS |
N.I. |
N.I. |
5k-1
|
|
SR/RS |
N.I. |
56.6 ± 1.0 |
5k-2
|
RR/SS |
22.4 ± 6.3 |
28.7 ± 10.1 |
5l-1
|
|
RR/SS |
7.9 ± 1.6 |
N.I. |
5l-2
|
SR/RS |
27.8 ± 21.0 |
94.4 ± 5.8 |
Bacterial membrane permeability
To evaluate the compounds' ability to cross bacterial outer membranes we prepared outer membrane vesicles carrying VIM-2 inside their lumen.44 Outer membrane vesicles maintain the protein as well as the lipid composition of the bacterial membrane they originate from, and thus serve as an in situ model system for assessing transmembrane uptake.45 The activity of VIM-2 encapsulated inside the outer membrane vesicles was determined using the colorimetric β-lactamase substrate CENTA in presence and absence of the compounds.46 We observed a reduction in VIM-2 activity for most compounds (Fig. 3), in agreement with the in vitro assay, indicating that they effectively cross the Gram-negative outer membrane.
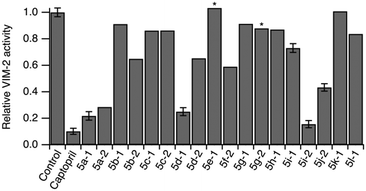 |
| Fig. 3 Normalized relative activity of VIM-2 encapsulated in outer membrane vesicles in absence (control) and presence of compounds. Error bars represent S.D. of 3 measurements. * indicates ambiguous readout due to compound precipitation. Compound concentrations are specified in Table S189.† | |
Protein–inhibitor interaction studies
Solution NMR spectroscopy is a powerful tool to assess protein–ligand interactions under close to physiological conditions.47 We, therefore, performed NMR chemical shift perturbation (CSP, for details, see section S8, ESI†) experiments to identify the amino acid residues of NDM-1 and VIM-2 that are involved in inhibitor binding, making use of the previously published protein assignments.48,49 Titrations were conducted using 15N-labelled proteins with 0, 0.5, 1, 2, 4, 8, and 16 equiv. of 5 inhibitors for NDM-1 and of 7 inhibitors for VIM-2.
As performing NMR titrations for all inhibitors in combination with both proteins was deemed unrealistic, we selected compounds based on their inhibitory activity and aqueous solubility. Titrations with concentrated ligand samples were preferred to minimize dilution-induced chemical shift changes. Titrations were carried out with NDM-1 and VIM-2 due to their high clinical relevance.
Throughout the titrations of NDM-1 with selected inhibitors (Table 2), Phe70, Asp124, and Lys211 surrounding the suggested β-lactam binding site showed significant chemical shift perturbations (Fig. 4 and S164–S168, ESI†). We also observed large chemical shift perturbations for Ser191, which has previously been reported to take part in substrate binding (numbered as Ser198).44,50 The observed spectral changes are characteristic for binding whereas not compatible with zinc(II)-depletion of the enzyme.44,51 Some amino acid residues in the C-terminal increased in intensity with increasing concentration of the added inhibitor, suggesting binding-induced alteration corresponding to the rate of the conformational dynamics of this enzyme.52
Table 2 NDM-1 and VIM-2 binding affinities as determined via NMR chemical shift perturbation experimentsa
Protein |
NDM-1 Kd [mM] |
VIM-2 Kd [mM] |
Compound |
For details, see section S8 of the ESI;† n.d., not determined.
|
5a-1
|
n.d. |
0.9 ± 0.1 |
5b-1
|
2.0 ± 0.1 |
0.4 ± 0.04 |
5b-2
|
n.d. |
1.4 ± 0.1 |
5c-1
|
3.1 ± 0.3 |
0.4 ± 0.03 |
5c-2
|
n.d. |
n.d. |
5i-1
|
0.5 ± 0.1 |
1.5 ± 0.2 |
5i-2
|
1.4 ± 0.1 |
1.1 ± 0.1 |
5j-1
|
2.2 ± 0.2 |
0.9 ± 0.1 |
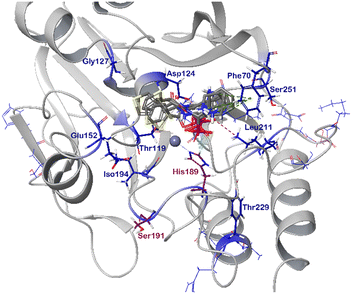 |
| Fig. 4 The chemical shift perturbation of the backbone amides (CSP) of 15N-labelled NDM-1 (pdb: 6O3R) upon addition of 16 equivalents of 5b-1/2, 5c-1/2, 5i-2 and 5j-2 (grey). CSPs greater than the population mean and one standard deviation (μ + 1σ) were considered significant and are colored. Amino acid residues highlighted in blue were influenced by binding of each inhibitor and thus are essential for binding. Those highlighted in purple were affected in the majority of the titrations. | |
We observed significant chemical shift perturbations for Tyr65, His177, Tyr199, Arg203, Ser205, and His238 of VIM-2, in the expected binding site (Fig. 5 and S169–S174, section S7, ESI†), and for a few additional amino acid residues at the N-terminal, the latter presumably due to binding induced conformational change of the enzyme. The line broadening was observed for Tyr65, Gly69, Thr83, His177, Tyr199, Ser202, Ser205, Ala206, His238, and Gly239 throughout at least one of the titrations, indicating the presence of a process with intermediate rate exchange, possibly related to the binding of the inhibitor and/or a binding induced conformational change.
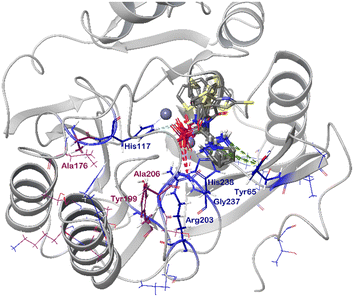 |
| Fig. 5 The chemical shift perturbation (CSP) of the backbone amides of 15N-labelled VIM-2 (pdb: 6O5T) upon titration with ligands 5b-1/2, 5c-1/2, 5i-1/2 and 5j-1 (grey). CSPs greater than the population mean plus one standard deviation (μ + 1σ) were considered significant and are colored as for Fig. 4. | |
Comparable chemical shift perturbations were observed for NDM-1 upon titrations with 5i-1, 5b-1, 5c-1 and 5j-1 to that observed for 5i-2 (R2 ∼ 0.81–0.84, Fig. 6), a compound that inhibits both NDM-1 and VIM-2, and revealed that the α-amino-phosphonate inhibitors bind to the same binding cleft and have comparable binding modes. Due to the structural differences leading to certain amino acid residues being involved in the binding of one but not of another inhibitor, the chemical shift perturbations are not expected to show perfect correlation. However, a reasonable correlation reveals the involvement of certain amino acid residues in the binding of all inhibitors studied herein. We also observed correlation of the chemical perturbations of the 1H,15N resonances of the amino acid residues of VIM-2 upon titration with 5b-1/5b-2, 5c-1, 5a-1, 5c-2 and 5j-1 to that observed for 5i-2 (Fig. 7).
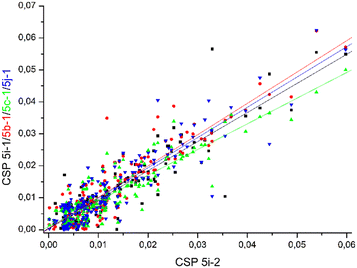 |
| Fig. 6 The chemical shift perturbation of NDM-1 upon titration with 5b-1, 5c-1, 5i-1, and 5j-1 correlates to that of 5i-2 with R2 ∼ 0.81–0.84, suggesting comparable binding modes of all ligands. For details, see Fig. S175–S178, ESI.† | |
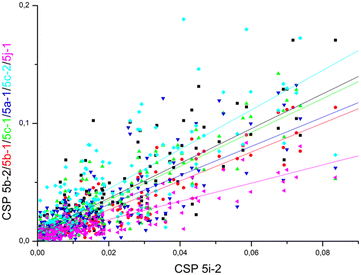 |
| Fig. 7 The chemical shift perturbation of VIM-2 upon addition of 16 equivalents of 5b-1/5b-2, 5c-1, 5a-1, 5c-2 and 5j-1 correlate to that observed upon titration with 5i-2 (R2 ∼ 0.61–0.82), suggesting comparable binding modes for the ligands. For details, see Fig. S179–S184, ESI.† | |
By superimposing the chemical shift perturbations observed for NDM-1 and VIM-2 upon titration with 5b-1, the amino acid residues important for binding of these compounds to both enzymes were identified (Fig. 8). Hence, we observed that His177 and His238 of VIM-2, and Asp124 of NDM-1, holding the zinc ions of the catalytic sites, showed large chemical shift perturbations. Moreover, Phe70/Tyr65 (NDM-1/VIM-2) of the lipophilic loop 3 and Lys214/Arg203 (NDM-1/VIM-2) of loop 10 are also pointed out as key residues for inhibitor binding.
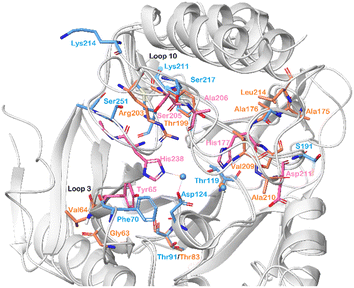 |
| Fig. 8 Chemical shift perturbations observed for NDM-1 (blue, pdb: 6NY7) and VIM-2 (pink and orange, pdb: 6O5T), shown superimposed, upon titration with 5b-1 revealed the amino acid residues that are important for α-aminophosphonate-type inhibitor binding for both metallo-β-lactamases. The zinc ions are shown as blue spheres. | |
The sequestration of Zn2+ ions from NDM-1 or VIM-2 were reported to cause extensive changes in their 1H,15N HSQC spectrum.44 Importantly, such large spectral alterations have not been observed at the titrations described above, which indicates that 5a–j do not act by metal sequestration, but rather by ligand replacement in the active site.
Computational docking
In order to visualize the binding of the studied inhibitors, we performed flexible docking for the compounds that have been selected for NMR titration above, using the software Glide (Schrödinger Inc.). The calculations were started from the experimental protein structures pdb 6O3R32 and pdb 6O5T,32 followed by MM-GBSA rescoring, which was selected as it is known as the quickest force-field based method for calculation of the free energy of binding.53 Ligands were prepared with LigPrep (Schrödinger Inc.), generating all possible stereoisomers and reasonable protonation states at pH 7 ± 2. Factors such as tautomerisation, metal binding, and the presence or absence of salts were also taken into account. To avoid bias or forced positions, docking was performed up to 40 times for each generated state for each ligand without experimental restraints. The resulting docking modes were evaluated in comparison to the experimentally observed 1H,15N NMR chemical shift perturbations (see the ESI†) and those compatible with the experimental data were kept. Next, they were ranked according to their binding free energies (ΔG) obtained by MM-GBSA rescoring, and the most feasible binding pose was selected. The stronger binding of the RS as compared to the SR, and of the RR as compared to the SS diastereomers of the studied inhibitors to both VIM-2 and NDM-1 (Tables S8 and S9, ESI†) was observed, as a general trend. This suggests that these stereoisomers (RS, RR) are primarily responsible for the enzyme inhibitory activities are shown in Table 1. The superposition of the binding modes (Fig. 4, VIM-2, and Fig. 5, NDM-1, pdb coordinates are provided as ESI†) indicates that the α-aminophosphonate-type inhibitors coordinate Zn1 of both NDM-1 (Fig. 9) and VIM-2 (Fig. 10) via an oxygen of the phosphonic acid. This computational observation is corroborated by the line broadening of the 1H,15N HSQC signal of His177 that directly coordinates Zn-1, and is in agreement with our design (inhibition by a tetrahedral phosphonate). Moreover, another oxygen of the phosphonic acid forms a hydrogen bond with Asn220 of NDM-1 or a salt bridge to Arg203 of VIM-2. The piperidine nitrogen of the inhibitors is involved in a π–cation interaction53,54 with Phe70 of NDM-1 or Tyr65 in case of VIM-2, whereas the thiophene ring of the inhibitors is oriented towards loop 10 of the enzyme. The binding poses of the inhibitors are similar, as revealed by the average RMSD55 of 2.6 ± 0.1 Å for NDM-1, and 2.8 ± 0.4 Å for VIM-2 binding (Fig. 4 and 5). The latter similarity is expected for structurally related inhibitors, and is supported by the good correlation of the NMR chemical shift perturbations observed for the same protein upon titration with different α-aminophosphonates (Fig. 6 and 7). Overall, the energetically preferred binding modes (Fig. 9 and 10) are in good agreement with the NMR chemical shift perturbations.
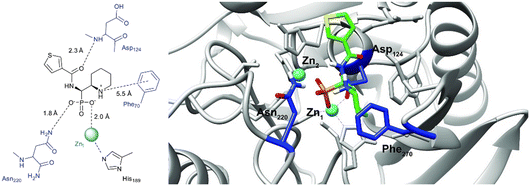 |
| Fig. 9 The interactions of (RS)-5c-1 with the active site of NDM-1 (pdb: 6NY7), as determined by computational docking directed by NMR (ligand induced protein chemical shift perturbation). The inhibitor is shown in green, the amino acids involved in the interaction in blue whereas the zinc(II) ions in cyan. The lowest ΔGbind docked positions of all inhibitor candidates (pdb) are provided as ESI, uploaded to the open access repository Zenodo (DOI: https://doi.org/10.5281/zenodo.6971841). | |
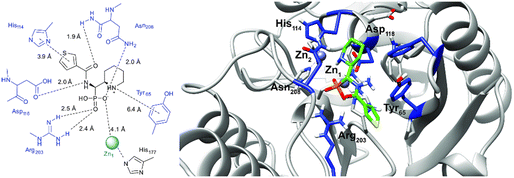 |
| Fig. 10 The interactions of (RS)-5c-1 with the active site of VIM-2 (pdb: 6O5T), as determined by computational docking directed by NMR (ligand induced protein chemical shift perturbation). The inhibitor is shown in green, the amino acids involved in the interaction in blue whereas the zinc(II) ions in cyan. The lowest ΔGbind docked positions of all inhibitor candidates (pdb) are provided as ESI, uploaded to the open access repository Zenodo (DOI: https://doi.org/10.5281/zenodo.6971841). | |
Pemberton et al.32 have shown that the binding pose of phosphonate-bearing coumarin inhibitors of NDM-1 may be pH dependent. At pH 7.5, the active site hydroxide is retained, and the phosphonate group interacts with this hydroxide and Zn2, rather than with both Zn(II) ions as at lower pH. The NMR titrations reported herein were performed at pH 7, however, based on the 15N NMR chemical shift perturbation data the possibility that 5a–l could bind via a bridging hydroxide ion cannot be excluded. The binding poses of phosphonates presented herein and of the coumarin phosphonate derivatives of Pemberton, an example being depicted in Fig. 11, show noticeable similarities.
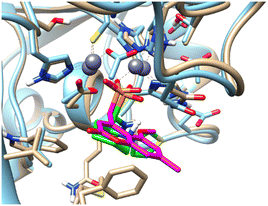 |
| Fig. 11 Superimposition of the computationally predicted binding mode of (RS)-5c-1 (green) to NDM-1 (grey) with the X-ray structure of [(5-methoxy-7-methyl-2-oxo-2H-chromen-4-yl)methyl]-phosphonic acid (magenta) in complex with NDM-1 (blue, PDB code 6D1E).32 | |
Comparing our data, we note that the ligand-induced chemical shift perturbation observed upon titration with 5a–l showed a wider spread of chemical shift changes for VIM-2 (Fig. 6) as compared to NDM-1 (Fig. 7), and that the binding modes of the inhibitors to VIM-2 are being slightly more dissimilar (Fig. 4) as compared to those of NDM-1 (Fig. 5). This may indicate somewhat higher flexibility of VIM-2 as compared to NDM-1, which may allow it to offer a larger degree of substrate promiscuity. This is corroborated by the observation of a larger number of α-aminophosphonate inhibitors showing activity against VIM-2 than NDM-1 in this study.
Conclusions
We designed and synthesized a series of α-aminophosphonate-type inhibitors for NDM-1 and VIM-2. Their evaluation in enzyme assays revealed several compounds to possess <10 μM inhibitory activity (IC50) against these metallo-β-lactamases, while showing no cytotoxicity, indicating their potential for further development. These compounds were designed to enhance the activity of β-lactam antibiotics, such as meropenem. Being the first members of a new type of inhibitors, they showed moderate to low inhibitory potency and are obviously not directly applicable in the clinical treatment of NDM-1 and VIM-2 expressing bacterial strains. As none of the enantiomers of 5a–l can be expected to have nM activity, it was not worth to separate or selectively generate their isomers. The development of stereoselective synthetic routes to α-aminophosphonates will therefore be the task for upcoming studies at the identification of more active members of this structural group. This may be achieved, for instance, by the structural modifications of the piperidine moiety of 5a–l. Hence, whereas these compounds aren't clinically applicable, their future structural modifications may yield compounds applicable for the treatment of NDM-1 and VIM-2 expressing bacterial strains. The inhibitor binding sites were indicated by 1H/15N NMR chemical shift perturbation experiments, evaluating the data of selected compounds in the series. Comparable magnitudes of chemical shift perturbation on the same amino acid residues upon titration with differently substituted inhibitors indicated that the α-aminophosphonic acid derivatives have a common binding site on both enzymes. Computational docking revealed that the RS and RR stereoisomers of the synthesized inhibitors are primarily responsible for NDM-1 and VIM-2 binding (Kd = 0.4–3.1 mM). A stronger correlation of the inhibitor induced chemical shift perturbations on NDM-1 as compared to that observed for VIM-2 may indicate a lower substrate specificity of VIM-2. This was corroborated by the observation of VIM-2 binding a larger number of phosphonates with more different binding poses, as determined by NMR guided computational docking. The amino acid residues Phe70, Asp124, Lys211, Ser191 and Ser249 for NDM-1, and Tyr65, His177, Tyr199, Arg203, Ser205, and His238 for VIM-2 Phe70/Tyr65 (VIM-2/NDM-1) were suggested to be necessary for inhibitor binding. This structural knowledge is expected to be useful for the development of future wide spectrum metallo-β-lactamase inhibitors. Accordingly, the compounds presented herein may be useful in fragment-based drug discovery, in addition to possibly providing a starting point for the development clinically applicable metallo-β-lactamase inhibitors.
Experimental methods
General methods
Reagents and reactants for synthesis were purchased from commercial suppliers (Sigma-Aldrich, Merck, Fluorochem, VWR, Arcos, Fischer Scientific) and were used without further purification. Reactions were monitored by LCMS (Agilent 1100 Series) equipped with an ESI-MS detector (Waters Micromass ZQ 2000) with a UV range of 200–600 nm, and an analytical column (Phenomenex, Gemini C18 column, 5 μm, 110 Å, ∅ 3.00 mm, L 50 mm) or by TLC-MS (API, Advion Express). Purification of the compounds was performed on Merck silica gel (0.035–0.079 mm, 5 Å) by manual column chromatography or by using Biotage Isolera One flash column chromatography system with solvent gradients. Additionally, diastereomers were separated using preparative RP-HPLC (VWR LaPrep P110) with single wavelength detection, using a Kromasil C8 column (10 μm, 100 Å, ∅ 39 mm, L 250 mm), and chiral column Phenomenex Lux Amylose-1 (5 μm, 1000 Å, ∅ 21.2 mm, L 250 mm) and gradients of CH3CN/H2O with/without 0.1% formic acid as mobile phase at 10/15 mL min−1 flow rate. NMR spectra of the synthetic intermediates were recorded at room temperature on a Varian Agilent MR400-DD2 with OneNMR probe (1H, 400 MHz), and a Bruker Avance Neo with TXO cryogenic probe (1H, 500 MHz). In order to assign diastereomers and rotamers, diastereomers were separated by preparative chiral HPLC. Protein-related spectra (series of 1H–15N HSQC spectra was used for the CSP experiment) were recorded in 90% H2O/10% D2O + 2.5% DMSO-d6 with a protein concentration of 0.25 mM at a Bruker Avance Neo (1H, 600 MHz) with TCI cryogenic probe, using 3 mm NMR tubes.
Crystallography
Crystallization of 4a–l was done by vapour diffusion or by layering. Layering dioxane over H2O at 12 °C provided single crystals suitable for X-ray diffraction analysis. Vapour diffusion was performed with THF/cyclohexane or methylformate/n-pentane at −20 °C, 12 °C, or at r.t. The crystallographic data were collected with either a Rigaku SuperNova single-source diffractometer equipped with an Eos CCD detector using mirror-monochromated Mo Kα (λ = 0.71073 Å) or a Rigaku SuperNova dual wave-length diffractometer equipped with an Atlas CCD area detector with Cu-Kα radiation (λ = 1.54184 Å). Data collection, reduction, and Gaussian or analytical face-index based absorption correction were performed with CrysAlisPro.56 Structures were solved using SHELXT57 and refined by full-matrix least-squares on F2 using SHELXL.58 In all data, anisotropic displacement parameters were introduced for all atoms except hydrogens, which were calculated or refined into their ideal positions using isotropic displacement parameters of 1.2 or 1.5 times that of the host atom. The figures were made using the Mercury program.59 The structures have been deposited to CCDC with codes 2203659 (4a-2), 2203660 (4b-2), 2203661 (4c-2), 2203662 (4d-2), 2203663 and 2203670 (4e-2), 2203664 (4f-1), 2203665 (4g-1), 2203666 (4h-2), 2209101 (4i-1), 2203667 (4j-1), 2203668 (4k-1), and 2203669 (4l-1). For a detailed description of the crystallographic data, see section S5 of the ESI.†
Synthetic procedures
The synthetic route towards 5a–l-1/2 is summarized in Scheme 3, and the details are given below.
tert-Butyl 2-((benzylamino)(diethoxyphosphoryl)methyl)-piperidine-1-carboxylate (2)
Compound 1 (3.55 g, 16.7 mmol), benzylamine (1.84 mL, 16.8 mmol, 1.0 equiv.) and diethyl phosphite (2.19 mL, 17.0 mmol, 1.0 equiv.) were dissolved in absolute EtOH (17 mL). HfCl4 (107 mg, 2 mol%) was added in one portion and the reaction was stirred at 60 °C for 12 h under an inert atmosphere. The solvent was removed under reduced pressure and the crude product was purified by FCC (SiO2, gradient EtOAc in hexane 80% to 100%), Rf (EtOAc
:
Hex 4
:
1) = 0.47. The product was obtained as a pale yellow solid (2.36 g, 5.35 mmol, 32%). HRESIMS m/z 441.2529 [M + H]+ (441.2513 calcd for C22H38N2O5P).
Compound 2, diastereomer 1 (rotamer ratio 1
:
1)
1H NMR (500 MHz, CDCl3) δ 7.31–7.27 (m, 4H, 11-H, 12-H, 14-H, 15-H), 7.22 (m, 1H, 13-H), 4.53 (br. s, 1H, 2-H), 4.26–4.16 (m, 4H, 1-P(OCH2)2), 4.07 (d, 2J = 12.9 Hz, 1H, part A of AB, 9-H), 3.98 (br. d, J = 14.1 Hz, 1H, 4-H), 3.78 (d, 2J = 12.9 Hz, 1H, part B of AB, 9-H), 3.24 (dd, 2JHP =10.6 Hz, J = 10.6 Hz, 1H, 1-H), 2.27 (m, 1H, 4-H), 2.14 (br. d, J = 13.8 Hz, 1H, 7-H), 1.71–1.42 (m, 5H, 5-H2, 6-H2, 7-H), 1.38 (s, 1H, 9H, 2-NCq(O)OCq–(CH3)3), 1.37–1.32 (m, 6H, 1-P(OCH2CH3)2). 13C NMR (126 MHz, CDCl3) δ 155.5 (2-N–Cq(O)–O), 140.0 (10-Cq), 128.7 (11-CH, 15-CH or 12-CH, 14-CH), 128.5 (11-CH, 15-CH or 12-CH, 14-CH), 127.3 (13-CH), 79.9 (2-NCq(O)O–Cq–(CH3)3), 62.6 (d, 2JCP = 7.4 Hz, 1-POCH2), 62.1 (br. s., 1-POCH2), 53.8 (9-CH2), 53.4 (d, 1JCP = 152.4 Hz, 1-CH), 51.0 (d, 2JCP = 13.8 Hz, 2-CH), 38.9 (4-CH2), 28.6 (2-NCq(O)OCq–(CH3)3), 27.1 (7-CH2), 25.2 (5-CH2), 19.5 (6-CH2), 16.8 (d, 3JCP = 5.4 Hz, 1-POCH2CH3), 16.8 (d, 3JCP = 4.8 Hz, 1-POCH2CH3). 31P NMR (162 MHz, CDCl3) δ 27.02.
Compound 2, diastereomer 2
1H NMR (500 MHz, CDCl3) δ 7.37–7.27 (m, 4H, 11-H, 12-H, 14-H, 15-H), 7.25 (m, 1H, 13-H), 4.33 (ddd, J = 13.7, 4.4, 4.4 Hz, 1H, 2-H), 4.22–4.07 (m, 4H, 1-P(OCH2)2), 4.06 (dd, 2J = 12.7 Hz, J = 1.7 Hz, 1H, part A of AB, 9-H), 3.98 (m, 1H, 4-H), 3.86 (dd, 2J = 12.7 Hz, J = 2.2 Hz, 1H, part B of AB, 9-H), 3.33 (dd, J = 14.0, 8.7 Hz, 1H, 1-H), 2.96 (dd, J = 11.8, 11.8 Hz, 1H, 4-H), 2.09 (m, 1H, 7-H), 1.59–1.37 (m, 5H, 5-H2, 6-H2, 7-H), 1.44 (s, 9H, 2-NCq(O)OCq–(CH3)3), 1.34 (t, J = 7.1 Hz, 3H, 1-POCH2CH3), 1.33 (t, J = 7.0 Hz, 3H, 1-POCH2CH3). 13C NMR (126 MHz, CDCl3) δ 154.8 (2-N–Cq(O)–O), 140.2 (10-Cq), 128.7 (11-CH, 15-CH or 12-CH, 14-CH), 128.5 (11-CH, 15-CH or 12-CH, 14-CH), 127.28 (13-CH), 79.4 (2-NCq(O)O–Cq–(CH3)3), 61.9 (d, 2JCP = 8.1 Hz, 1-POCH2), 61.8 (d, 2JCP = 8.1 Hz, 1-POCH2), 54.3 (d, 1JCP = 139.6 Hz, 1-CH), 53.3 (9-CH2), 51.7 (br. s, 2-CH), 40.2 (br. s, 4-CH2), 28.6 (2-NCq(O)OCq–(CH3)3), 24.8 (5-CH2), 24.7 (d, 3JCP = 7.9 Hz, 7-CH2), 19.4 (6-CH2), 16.7 (d, 3JCP = 5.8 Hz, 1-POCH2CH3), 16.7 (d, 3JCP = 5.7 Hz, 1-POCH2CH3). 31P NMR (162 MHz, CDCl3) δ 27.35.
tert-Butyl 2-(amino(diethoxyphosphoryl)methyl)piperi-dine-1-carboxylate (3)
Compound 2 (2.36 g, 5.35 mmol) and Pd(OH)2/C, 50% wet (10% w/w, 1.28 g, 0.91 mmol, 0.17 equiv.) were dissolved in EtOH (54 mL). A strong argon flow was used to bubble argon through the reaction mixture for 5 min. Subsequently, H2 was bubbled through the solution for 30 s and the reaction vial was equipped with an H2-filled balloon. The reaction was stirred at r.t. for 16 h. The reaction mixture was filtered through a plug of Celite and a syringe filter to remove Pd traces. The solvent was removed under reduced pressure. The product was obtained as a colorless liquid (1.87 g, 5.33 mmol, 99%). HRESIMS m/z 351.2066 [M + H]+ (351.2043 calcd for C15H32N2O5P).
Compound 3, diastereomer 1
1H NMR (400 MHz, CDCl3) δ 4.32 (m, 1H, 2-H), 4.13 (q, J = 7.1 Hz, 2H, 1-POCH2 or 1-P(OCH)2), 4.13 (q, J = 7.1 Hz, 2H, 1-POCH2 or 1-P(OCH)2), 4.00 (m, 1H, 4-H), 3.42 (dd, 1JHP = 10.4 Hz, J = 10.4 Hz, 1H, 1-H), 2.89 (dd, J = 12.1, 12.1 Hz, 1H, 4-H), 2.09 (br. d, J = 11.7 Hz, 1H, 7-H), 1.67–1.37 (m, 5H, 5-CH2, 6-H2, 7-H), 1.45 (s, 9H, 2-NCq(O)OCq–(CH3)3), 1.31 (t, J = 6.8 Hz, 6H, 1-P(OCH2CH3)2).13C NMR (101 MHz, CDCl3) δ 154.7 (2-N–Cq(O)–O), 79.6 (2-NCq(O)O–Cq–(CH3)3), 62.3 (d, 2JCP = 7.3 Hz, 1-POCH2), 62.1 (d, 2JCP = 7.3 Hz, 1-POCH2), 51.9 (d, 2JCP = 2.5 Hz, 2-CH), 48.2 (d, 1JCP = 147.0 Hz, 1-CH), 40.3 (br. s, 4-CH2), 28.6 (2-NCq(O)OCq–(CH3)3), 25.0 (5-CH2), 24.8 (d, 3JCP = 9.4 Hz, 7-CH2), 19.3 (6-CH2), 16.7 (d, 3JCP = 3.8 Hz, 1-POCH2CH3), 16.6 (d, 3JCP = 4.1 Hz, 1-POCH2CH3). 31P NMR (162 MHz, CDCl3) δ 27.42.
Compound 3, diastereomer 2 (rotamer ratio 1
:
1.3)
1H NMR (400 MHz, CDCl3) δ 4.36 (br. s, 1H, 2-H), 4.24–4.08 (m, 5H, 1-P(OCH2)2, 4-H), 3.37 (dd, 2JHP = 11.6 Hz, J = 11.6 Hz, 1H, 1-H), 2.59 (br. dd, J = 12.7, 12.7 Hz, 1H, 4-H), 2.16 (br. d, J = 13.0 Hz, 1H, 7-H), 1.73–1.50 (m, 5H, 5-H2, 6-CH2, 7-H), 1.45 (s, 9H), 1.34 (dd, J = 7.1, 7.1 Hz, 3H, 1-POCH2CH3), 1.33 (dd, J = 7.0, 7.0 Hz, 3H, 1-POCH2CH3). 13C NMR (126 MHz, CDCl3) δ 155.7 (2-N–Cq(O)–O), 80.0 (2-NCq(O)O–Cq–(CH3)3), 62.5 (d, 2JCP = 7.2 Hz, 1-POCH2), 62.3 (d, 2JCP = 6.9 Hz, 1-POCH2), 52.4 (d, 2JCP = 10.5 Hz, 2-H), 47.4 (d, 1JCP = 149.5 Hz, 1-H), 38.8 (4-CH), 28.6 (2-NCq(O)OCq–(CH3)3), 26.9 (7-CH2), 25.2 (5-CH2), 19.4 (6-CH2), 16.7 (d, 3JCP = 5.5 Hz, 1-P(OCH2CH3)2). 31P NMR (162 MHz, CDCl3) δ (27.83), 27.62.
General procedure A for 4a–l
The carboxylic acid (2.0 equiv.) and HATU (2.1 equiv.) were dissolved in ethyl acetate. DIPEA (4.0 equiv.) was added dropwise and the reaction mixture was stirred at r.t. for at least 10 min. The reaction mixture was added to a solution of 3 in EtOAc. The reaction was stirred at r.t. for 12–24 h. The reaction mixture was then diluted with EtOAc and washed (3×) with a sat. solution of NaHCO3 (or alternatively, with a mixture of NaHCO3 and brine). The aqueous layer was re-extracted with EtOAc (2×) and the combined organic layers were washed with brine and dried over Na2SO4. The solvent was removed under reduced pressure. The crude product was purified using preparative HPLC (C8 column, MeCN:H2O). The product was obtained as separated diastereomers (in a total yield of 60–80%).
tert-Butyl 2-((diethoxyphosphoryl)(2-(4-methyl-2-thioxo-2,3-dihydrothiazol-5-yl)acetamido)methyl)piperidine-1-carboxylate (4a). The synthesis was performed according to general procedure A, 3 (316 mg, 0.902 mmol) and 2-mercapto-4-methyl-5-thiazoleacetic acid (341 mg, 1.80 mmol, 2.0 equiv.) gave the total product (330 mg, 0.632 mmol, 70%). 4a-1 was obtained as a light orange solid (152 mg, 0.291 mmol, 32%). 4a-2 was obtained as a light orange solid (178 mg, 0.341 mmol, 38%).
Compound 4a-1, diastereomer 1
1H NMR (500 MHz, DMSO-d6) δ 12.93 (s, 1H, 13-NH), 8.67 (d, J = 9.9 Hz, 1H, 1-NH), 4.71 (ddd, 2JHP = 15.7 Hz, J = 10.2, 10.2 Hz, 1H, 1-H), 4.42 (m, 1H, 2-H), 4.09–3.69 (m, 5H, 4-H, 1-P(OCH2)2), 3.46 (s, 2H, 10-H2), 2.74 (m, 1H, 4-H), 2.06 (s, 3H, 15-CqCH3), 1.61 (br. d, J = 13.4 Hz, 1H), 1.53–1.43 (m, 3H, 5-H, 6-H2), 1.40 (s, 9H, 2-NCq(O)OCq–(CH3)3), 1.36–1.19 (m, 2H, 5-H, 7-H), 1.17 (dd, J = 7.1 Hz, 3H, 1-POCH2CH3), 1.15 (dd, J = 7.1 Hz, 3H, 1-POCH2CH3). 13C NMR (126 MHz, DMSO-d6) δ 186.9 (13-CH), 168.2 (d, 3JCP = 4.8 Hz), 153.5 (2-N–Cq(O)–O), 135.0 (15-Cq), 117.0 (11-Cq), 78.7 (2-NCq(O)O–Cq–(CH3)3), 62.1 (br. s, 1-POCH2), 61.4 (br. s, 1-POCH2), 50.2 (br. s, 2-CH), 43.6 (d, 1JCP = 156.7 Hz, 1-CH), 38.5 (br. s, 4-CH), 32.3 (10-CH2), 28.1 (2-NCq(O)OCq–(CH3)3), 24.7 (br. s, 7-CH2), 24.5 (br. s, 5-CH2), 18.8 (6-CH2), 16.3 (d, 3JCP = 5.5 Hz, 1-POCH2CH3), 16.1 (d, 3JCP = 6.0 Hz, 1-POCH2CH3), 11.3 (15-CqCH3). 31P NMR (162 MHz, DMSO-d6) δ 22.48. HRESIMS m/z 522.2179 [M + H]+ (calcd 522.1856 for C21H37N3O6PS2).
Compound 4a-2, diastereomer 2 (rotamer ratio 2.3
:
1)
1H NMR (500 MHz, DMSO-d6) δ 12.89 (s, 1H, 13-NH), 8.45 (d, J = 10.2 Hz, 1H, 1-NH), 4.71 (ddd, 2JHP = 17.4 Hz, J = 10.9, 10.9 Hz, 1H, 1-H), 4.46 (m, 1H, 2-H), 4.10–3.95 (m, 4H, 1-P(OCH2)2), 3.74–3.65 (m, 1H, 4-CH), 3.47 (d, 2J = 15.9 Hz, 1H, part A of AB, 10-H), 3.33 (d, 2J = 15.9 Hz, part B of AB, 10-H), 2.71 (dd, J = 13.7, 13.7 Hz, 1H, 4-CH), 2.05 (m, 1H, 7-CH), 2.03 (s, 3H, 15-CqCH3), 1.58–1.47 (m, 4H, 5-CH, 6-CH2, 7-CH), 1.36 (s, 9H, 2-NCq(O)OCq–(CH3)3), 1.24 (m, 1H, 5-CH), 1.19 (t, J = 7.1 Hz, 6H, 1-(POCH2CH3)2). 13C NMR (101 MHz, DMSO-d6) δ 187.4 (13-Cq), 168.8 (d, 3JCP = 4.9 Hz, 9-Cq), 154.6 (2-N–Cq(O)–O), 135.1 (15-Cq), 117.5 (11-Cq), 79.1 (2-NCq(O)O–Cq–(CH3)3), 62.9 (d, 2JCP = 7.0 Hz, 1-POCH2), 62.1 (d, 2JCP = 6.7 Hz, 1-POCH2), 50.1 (d, 2JCP = 13.6 Hz, 2-CH), 44.1 (d, 1JCP = 151.3 Hz, 1-CH), 39.8 (4-CH2), 32.5 (10-CH2), 28.5 (2-NCq(O)OCq–(CH3)3), 26.4 (7-CH2), 25.3 (5-CH2), 19.2 (6-CH2), 16.7 (d, 3JCP = 5.5 Hz, 1-POCH2CH3), 16.5 (d, 3JCP = 6.0 Hz, 1-POCH2CH3), 11.7 (15-CqCH3). 31P NMR (162 MHz, DMSO-d6) δ 22.92 (22.71). HRESIMS m/z 522.2255 [M + H]+ (calcd for C21H37N3O6PS2 522.18559). The solution tautomer was confirmed by 1H–15N HSQC, and by X-ray diffraction.
tert-Butyl 2-((diethoxyphosphoryl)(thiophene-2-carbox-amido)methyl)piperidine-1-carboxylate (4b)
The synthesis was performed according to general procedure A, 3 (211 mg, 0.603 mmol) and thiophene-2-carboxylic acid (154 mg, 1.19 mmol, 2.0 equiv.) gave the total product (212 mg, 0.460 mmol, 76%). 4b-1 was obtained as a colorless solid (100 mg, 0.217 mmol, 36%). 4b-2 was obtained as a colorless solid (112 mg, 0.243 mmol, 40%).
Compound 4b-1, diastereomer 1
1H NMR (500 MHz, CD3CN) δ 7.71 (d, J = 3.8 Hz, 1H, 14-H), 7.64 (d, J = 5.0 Hz, 1H, 12-H), 7.35 (br. m, 1H, 1-NH), 7.12 (dd, J = 5.0, 3.8 Hz, 1H, 13-H), 5.04 (ddd, 2JHP = 15.7 Hz, J = 10.5, 10.5 Hz, 1H), 4.74 (m, 1H, 2-H), 4.12–3.89 (m, 5H, 1-P(OCH2)2, 4-H), 2.94 (m, 1H, 4-H), 1.74 (br. d, J = 11.5 Hz, 1H, 7-H), 1.69–1.49 (m, 4H, 5-H, 6-H2, 7-H), 1.44 (s, 9H, 2-NCq(O)OCq–(CH3)3), 1.34 (m, 1H, 5-H), 1.24 (t, J = 7.1 Hz, 3H, 1-POCH2CH3), 1.19 (t, J = 7.1 Hz, 3H, 1-POCH2CH3). 13C NMR (101 MHz, CD3CN) δ 162.3 (d, J = 4.8 Hz, 9-Cq), 155.2 (2-N–Cq(O)–O), 139.9 (10-Cq), 132.1 (12-CH), 129.5 (14-CH), 129.0 (13-CH), 79.9 (2-NCq(O)O–Cq–(CH3)3), 63.5 (d, 2JCP = 8.1 Hz, 1-POCH2), 63.0 (d, 2JCP = 6.7 Hz, 1-POCH2), 51.1 (2-CH), 45.4 (d, 1JCP = 157.2 Hz, 1-CH), 41.4 (4-CH2), 28.6 (2-NCq(O)OCq–(CH3)3), 26.0 (d, 3JCP = 10.0 Hz, 7-CH2), 25.8 (5-CH2), 20.1 (6-CH2), 16.8 (d, 3JCP = 5.7 Hz, 1-POCH2CH3), 16.7 (d, 3JCP = 6.1 Hz, 1-POCH2CH3). 31P NMR (162 MHz, CDC3N) δ (21.77), 21.67. HRESIMS m/z 461.1854 [M + H]+ (calcd for C20H34N2O6PS 461.18697).
Compound 4b-2, diastereomer 2 (rotamer ratio 2.3
:
1)
1H NMR (500 MHz, CD3CN) δ 7.61 (d, J = 4.8 Hz, 1H, 14-H), 7.55 (d, J = 3.8 Hz, 1H, 12-H), 7.08 (dd, J = 4.8, 3.8 Hz, 1H, 13-), 6.88 (d, J = 9.6 Hz, 1H, 1-NH), 4.95 (ddd, 2JHP = 16.0 Hz, J = 10.7, 10.7 Hz, 1H, 1-H), 4.60 (m, 1H, 2-H), 4.18–4.02 (m, 4H, 1-P(OCH2)2), 3.84 (dd, J = 13.5, 3.4 Hz, 1H, 4-H), 2.77 (ddd, J = 13.2, 13.2, 1.9 Hz, 1H, 4-H), 2.26 (br. d, J = 11.9 Hz, 1H, 7-H), 1.68–1.58 (m, 4H, 5-H, 6-H2, 7-H), 1.34 (m, 1H, 5-H), 1.25 (t, J = 7.0 Hz, 3H, 1-POCH2CH3), 1.25 (t, J = 7.1 Hz, 3H, 1-POCH2CH3), 1.20 (s, 9H, 2-NCq(O)OCq–(CH3)3). 13C NMR (101 MHz, CD3CN) δ 162.0 (9-Cq), 157.0 (2-N–Cq(O)–O), 139.4 (10-Cq), 132.1 (12-CH), 129.5 (14-CH), 128.9 (13-CH), 80.5 (2-NCq(O)O–Cq–(CH3)3), 63.8 (d, 2JCP = 7.0 Hz, 1-POCH2), 63.3 (d, 2JCP = 6.5 Hz, 1-POCH2), 51.0 (d, 3JCP = 12.4 Hz, 2-CH), 46.5 (d, 1JCP = 153.3 Hz, 1-CH), 40.8 (4-CH2), 28.3 (2-NCq(O)OCq–(CH3)3), 26.9 (7-CH2), 25.87 (5-CH2), 19.8 (6-CH2), 16.8 (d, 3JCP = 5.7 Hz, 1-POCH2CH3), 16.7 (d, 3JCP = 6.0 Hz, 1-POCH2CH3). 31P NMR (162 MHz, CD3CN) δ (21.78), 21.74. HRESIMS m/z 461.2128 [M + H]+ (461.18697 calcd for C20H34N2O6PS).
tert-Butyl 2-((diethoxyphosphoryl)(thiophene-3-carbox-amido)methyl)piperidine-1-carboxylate (4c)
The synthesis was performed according to general procedure A, 3 (211 mg, 0.601 mmol) and thiophene-3-carboxylic acid (153 mg, 1.20 mmol, 2.0 equiv.) gave the total product (219 mg, 0.476 mmol, 79%). 4c-1 was obtained as a colorless solid (101 mg, 0.219 mmol, 37%); 4c-2 was obtained as a colorless solid (118 mg, 0.256 mmol, 43%).
Compound 4c-1, diastereomer 1
1H NMR (400 MHz, CDCl3) δ 7.98 (d, J = 2.2 Hz, 1H, 11-H), 7.46 (dd, J = 5.1, 1.3 Hz, 1H, 13-H), 7.34 (dd, J = 5.1, 3.0 Hz, 1H, 14-H), 6.96 (m, 1H, 1-NH), 5.08 (m, 1H, 1-H), 4.60 (m, 1H, 2-H), 4.17–4.04 (m, 5H, 1-P(OCH2)2, 4-H), 3.01 (m, 1H, 4-H), 1.85–1.68 (m, 2H, 6-H, 7-H), 1.63–1.53 (m, 3H, 5-H, 6-H, 7-H), 1.48 (s, 9H, 2-NCq(O)OCq–(CH3)3), 1.40 (m, 1H, 5-H), 1.29 (t, J = 7.1 Hz, 3H, 1-POCH2CH3), 1.23 (t, J = 7.1 Hz, 3H, 1-POCH2CH3). 13C NMR (101 MHz, CDCl3) δ 162.4 (d, 3JCP = 4.8 Hz, 9-Cq), 154.8 (2-N–Cq(O)–O), 136.8 (10-Cq), 129.1 (11-CH), 126.7 (13-CH), 126.4 (14-CH), 79.9 (2-NCq(O)O–Cq–(CH3)3), 63.1 (d, 2JCP = 5.5 Hz, 1-POCH2), 62.5 (d, 2JCP = 6.9 Hz, 1-POCH2), 51.4 (2-CH), 45.1 (d, 1JCP = 156.7 Hz, 1-CH), 40.4 (4-CH2), 28.6 (2-NCq(O)OCq–(CH3)3), 25.4 (d, 3JCP = 9.7 Hz, 7-CH2), 24.8 (5-CH2), 19.5 (6-CH2), 16.6 (d, 3JCP = 5.6 Hz, 1-POCH2CH3), 16.5 (d, 3JCP = 6.2 Hz, 1-POCH2CH3). 31P NMR (162 MHz, CDCl3) δ (23.06), 22.83. HRESIMS m/z 461.1781 [M + H]+ (461.1870 calcd for C20H34N2O6PS).
Compound 4c-2, diastereomer 2 (rotamer ratio 6.6
:
1)
1H NMR (400 MHz, CDCl3) δ 7.89 (d, J = 2.8 Hz, 1H, 11-H), 7.40 (m, 1H, 13-H), 7.28 (dd, J = 4.2, 3.0 Hz, 1H, 14-H), 6.72 (d, J = 9.6 Hz, 1H, 1-NH), 5.03 (ddd, 2JHP = 15.4, J = 10.7, 10.7 Hz, 1H, 1-H), 4.70 (m, 1H, 2-H), 4.19–4.09 (m, 4H, 1-P(OCH2)2), 3.85 (m, 1H, 4-H), 2.80 (ddd, J = 13.4, 13.4, 2.9 Hz, 1H, 4-H), 2.44–2.30 (m, 1H, 7-H), 1.73–1.55 (m, 4H, 5-H, 6-H2, 7-H), 1.37 (m, 1H, 5-H), 1.30 (t, J = 6.9 Hz, 1H, 1-POCH2CH3), 1.28 (t, J = 6.8 Hz, 1H, 1-POCH2CH3), 1.23 (s, 9H, 2-NCq(O)OCq–(CH3)3). 13C NMR (101 MHz, CDCl3) δ 162.3 (d, 3JCP = 3.8 Hz, 9-Cq), 156.7 (2-N–Cq(O)–O), 136.8 (10-Cq), 129.0 (11-CH), 126.5 (13-CH), 126.4 (14-CH), 80.1 (2-NCq(O)O–Cq–(CH3)3), 62.9 (d, 2JCP = 5.6 Hz, 1-POCH2), 62.9 (d, J = 5.5 Hz, 1-POCH2), 50.1 (d, 2JCP = 12.0 Hz, 2-CH), 45.6 (d, 1JCP = 152.8 Hz, 1-CH), 40.2 (4-CH2), 28.3 (2-NCq(O)OCq–(CH3)3), 26.3 (7-CH2), 25.2 (5-CH2), 19.2 (6-CH2), 16.6 (d, 3JCP = 5.4 Hz, 1-POCH2CH3), 16.4 (d, 3JCP = 6.2 Hz, 1-POCH2CH3). 31P NMR (162 MHz, CDCl3) δ (23.43), 23.10. HRESIMS m/z 461.1836 [M + H]+ (461.1870 calcd for C20H34N2O6PS).
tert-Butyl 2-((diethoxyphosphoryl)(4,5,6,7-tetrahydro-benzo-[b]thiophene-2-carboxamido)methyl)piperidine-1-carboxylate (4d)
The synthesis was performed according to general procedure A, 3 (241 mg, 0.689 mmol) and 4,5,6,7-tetrahydrobenzo[b]thiophene-2-carboxylic acid (251 mg, 1.38 mmol, 2.0 equiv.) gave the total product (269 mg, 0.523 mmol, 76%). 4d-1 was obtained as a colorless solid (127 mg, 0.247 mmol, 36%). 4d-2 was obtained as a colorless solid (142 mg, 0.256 mmol, 43%).
Compound 4d-1, diastereomer 1
1H NMR (400 MHz, CDCl3) δ 7.26 (s, 1H, 18-H), 6.23 (br. s, 1H, 1-NH), 5.06 (ddd, 2JHP = 16.7 Hz, J = 9.9, 9.9 Hz, 1H, 1-H), 4.57 (br. s, 1H, 2-H), 4.19–3.98 (m, 5H, 1-P(OCH2)2, 4-H), 2.94 (br. s, 1H, 4-H), 2.78 (dd, J = 6.1, 6.1 Hz, 1H, 16-H2), 2.60 (dd, J = 6.1, 6.1 Hz, 1H, 13-H2), 1.91–1.66 (m, 8H, 6-H, 7-H, 14-H2, 15-H2), 1.64–1.53 (m, 3H, 5-H, 6-H, 7-H), 1.49 (s, 9H, 2-NCq(O)OCq–(CH3)3), 1.40 (m, 1H, 5-H), 1.29 (t, 3JHP = 6.9 Hz, 3H, 1-POCH2CH3), 1.26 (t, 3JHP = 7.2 Hz, 3H, 1-POCH2CH3). 13C NMR (126 MHz, CDCl3) δ 161.6 (d, 3JCP = 4.8 Hz, 9-Cq), 154.7 (2-N–Cq(O)–O), 142.4 (17-Cq), 136.6 (12-Cq), 133.5 (10-Cq), 129.8 (18-CH), 79.9 (2-NCq(O)O–Cq–(CH3)3), 63.1 (br. s, 1-POCH2), 62.5 (d, 2JCP = 6.9 Hz, 1-POCH2), 51.2 (2-CH), 45.0 (d, 1JCP = 159.9 Hz, 1-CH), 40.7 (4-CH2), 28.6 (2-NCq(O)OCq–(CH3)3), 25.6 (13-CH2), 25.5 (16-CH2), 25.4 (d, 3JCP = 8.5 Hz, 7-CH2), 24.9 (5-CH2), 23.4 (15-CH2), 22.7 (14-CH2), 19.5 (6-CH2), 16.6 (d, 3JCP = 5.5 Hz, 1-POCH2CH3), 16.4 (d, 3JCP = 6.3 Hz, 1-POCH2CH3). 31P NMR (162 MHz, CDCl3) δ 22.72. HRESIMS m/z 515.2324 [M + H]+ (515.2339 calcd for C22H38N2O5P).
Compound 4d-2, diastereomer 2 (rotamer ratio 4.9
:
1)
1H NMR (400 MHz, CDCl3) δ 7.12 (s, 1H, 18-H), 6.55 (d, J = 9.6 Hz, 1H, 1-NH), 4.99 (ddd, 2JHP = 15.0 Hz, J = 11.6, 9.6 Hz, 1H, 1-H), 4.68 (m, 1H, 2-H), 4.19–4.10 (m, 4H, 1-P(OCH2)2), 3.84 (m, 1H, 4-H), 2.78 (ddd, J = 13.3, 13.3, 2.9 Hz, 1H, 4-H), 2.76–2.72 (m, 2H, 13-H2 or 16-H2), 2.64–2.44 (m, 2H, 16-H2 or 13-H2), 2.37 (br. d, J = 11.5 Hz, 1H, 7-H), 1.87–1.72 (m, 4H, 14-H2, 15-H2), 1.69–1.56 (m, 4H, 5-H, 6-H2, 7-H), 1.40 (m, 1H, 5-H), 1.31 (t, 3JCP = 7.1 Hz, 3H, 1-POCH2CH3), 1.28 (s, 9H, 2-NCq(O)OCq–(CH3)3), 1.28 (t, J = 7.3 Hz, 3H, 1-POCH2CH3). 13C NMR (101 MHz, CDCl3) δ 161.7 (d, J = 3.8 Hz, 9-Cq), 156.7 (2-N–Cq(O)–O), 142.1 (17-Cq), 136.2 (12-Cq), 133.9 (10-Cq), 129.1 (18-CH), 80.1 (2-NCq(O)O–Cq–(CH3)3), 62.9 (d, J = 5.5 Hz, 1-POCH2), 62.8 (d, 2JCP = 6.8 Hz, 1-POCH2), 50.0 (d, 2JCP = 12.2 Hz, 2-CH), 45.7 (d, 1JCP = 152.7 Hz, 1-CH), 40.2 (4-CH2), 28.3 (2-NCq(O)OCq–(CH3)3), 26.3 (7-CH2), 25.5 (13-CH2 or 16-CH2), 25.4 (13-CH2 or 16-CH2), 25.2 (5-CH2), 23.4 (15-CH2), 22.8 (14-CH2), 19.2(6-CH2), 16.7 (d, 3JCP = 5.3 Hz, 1-POCH2CH3), 16.5 (d, 3JCP = 6.3 Hz, 1-POCH2CH3). 31P NMR (162 MHz, CDCl3) δ (23.42), 23.05. HRESIMS m/z 515.2316 [M + H]+ (515.2339 calcd for C22H38N2O5P).
tert-Butyl 2-((diethoxyphosphoryl)(4,5,6,7-tetrahydro-benzo-[b]thiophene-3-carboxamido)methyl)piperidine-1-carboxylate (4e)
Synthesis was performed according to the general procedure A, 3 (322 mg, 0.919 mmol) and 4,5,6,7-tetrahydrobenzo[b]thiophene-3-carboxylic acid (336 mg, 1.84 mmol) gave the total product (317 mg, 0.616 mmol, 67%). 4e-1 was obtained as a colorless solid (117 mg, 0.227 mmol, 25%). 4e-2 was obtained as a colorless solid (201 mg, 0.391 mmol, 43%).
Compound 4e-1, diastereomer 1
1H NMR (400 MHz, CDCl3) δ 7.50 (s, 1H, 11-H), 6.35 (br. s, 1H, 1-NH), 5.07 (ddd, 2JHP = 16.8 Hz, J = 9.9, 9.9 Hz, 1H, 1-H), 4.55 (m, 1H, 2-H), 4.18–4.03 (m, 4H, 1-P(OCH2)2), 3.97 (m, 1H, 4-H), 3.00 (m, 1H, 4-H), 2.88–2.70 (m, 4H, 14-CH2, 17-CH2), 1.90–1.72 (m, 6H, 6-H, 7-H, 15-CH2, 16-CH2), 1.65–1.53 (m, 4H, 5-H, 6-H, 7-H), 1.48 (s, 9H, 2-NCq(O)OCq–(CH3)3), 1.40 (m, 1H, 5-H), 1.29 (m, 6H, 1-P(OCH2CH3)2). 13C NMR (126 MHz, CDCl3) δ 163.9 (d, J = 4.7 Hz, 9-Cq), 154.8 (2-N–Cq(O)–O), 138.3 (13-Cq or 18-Cq), 135.6 (10-Cq), 134.7 (13-Cq or 18-Cq), 124.8 (11-CH), 79.8 (2-NCq(O)O–Cq–(CH3)3), 62.8 (br. s, 1-POCH2), 62.5 (d, 2JCP = 6.6 Hz, 1-POCH2), 51.2 (2-CH), 44.4 (d, 1JCP = 156.2 Hz, 1-CH), 40.6 (4-CH2), 28.5 (2-NCq(O)OCq–(CH3)3), 25.7 (14-CH2 or 17-CH2), 25.5 (14-CH2 or 17-CH2), 25.5 (d, 3JCP = 8.1 Hz, 7-CH2), 24.9 (5-CH2), 23.2 (15-CH2 or 16-CH2), 22.7 (15-CH2 or 16-CH2), 19.5 (6-CH2), 16.6 (d, 3JCP = 5.7 Hz, 1-POCH2CH3), 16.5 (d, 3JCP = 6.1 Hz, 1-POCH2CH3). 31P NMR (162 MHz, CDCl3) δ (22.91), 22.54. HRESIMS m/z 515.2349 [M + H]+ (515.2339 calcd for C22H38N2O5P).
Compound 4e-2, diastereomer 2 (rotamer ratio 3.6
:
1)
1H NMR (400 MHz, CDCl3) δ 7.48 (s, 1H, 11-H), 6.39 (d, J = 9.9 Hz, 1H, 1-NH), 5.02 (ddd, 2JHP = 15.4 Hz, J = 11.6, 9.9 Hz, 1H, 1-H), 4.67 (m, 1H, 2-H), 4.27–4.08 (m, 4H, 1-P(OCH2)2), 3.85 (br. dd, J = 13.52, 3.7 Hz, 1H, 4-H), 2.88–2.59 (m, 5H, 4-H, 14-H2, 17-H2), 2.39 (br. d, J = 10.1 Hz 1H, 7-H), 1.92–1.72 (m, 4H, 15-H2, 16-H2), 1.70–1.56 (m, 4H, 5-H, 6-H2, 7-H), 1.40 (m, 1H, 5-H), 1.34 (t, J = 7.2 Hz, 3H, 1-POCH2CH3), 1.29 (t, J = 7.1 Hz, 3H, 1-POCH2CH3), 1.24 (s, 9H, 2-NCq(O)OCq–(CH3)3). 13C NMR (101 MHz, CDCl3) δ 163.7 (d, J = 4.0 Hz, 9-Cq), 156.4 (2-N–Cq(O)–O), 137.8 (13-Cq or 18-Cq), 135.3 (10-Cq), 134.3 (13-Cq or 18-Cq), 124.9 (11-CH), 79.8 (2-NCq(O)O–Cq–(CH3)3), 62.7 (d, 2JCP = 6.0 Hz, 1-POCH2), 62.5 (d, 2JCP = 7.2 Hz, 1-POCH2), 49.8 (d, 2JCP = 12.4 Hz, 2-H), 44.8 (d, 1JCP = 152.2 Hz, 1-H), 40.0 (4-CH2), 28.1 (2-NCq(O)OCq–(CH3)3), 26.1 (7-CH2), 25.5 (14-CH2 or 17-CH2), 25.3 (14-CH2 or 17-CH2), 25.1 (5-CH2), 23.0 (15-CH2 or 16-CH2), 22.6 (15-CH2 or 16-CH2), 19.00 (6-CH2), 16.5 (d, 3JCP = 5.4 Hz, 1-POCH2CH3), 16.3 (d, 3JCP = 6.2 Hz, 1-POCH2CH3). 31P NMR (162 MHz, CDCl3) δ (23.57), 23.27. HRESIMS m/z 515.2302 [M + H]+ (515.2339 calcd for C22H38N2O5P).
tert-Butyl 2-((diethoxyphosphoryl)(4,5,6,7-tetrahydro-benzo[c]thiophene-1-carboxamido)methyl)piperidine-1-carboxylate (4f)
The synthesis was performed according to general procedure A, 3 (303 mg, 0.865 mg) and 4,5,6,7-tetrahydrobenzo[c]thiophene-1-carboxylic acid (336 mg, 1.84 mg, 2.1 equiv.) gave the total product (275 mg, 0.534 mmol, 62%). 4f-1 was obtained as a colorless solid (121 mg, 0.235 mmol, 27%). 4f-2 was obtained as a colorless solid (201 mg, 0.391 mmol, 45%).
Compound 4f-1, diastereomer 1
1H NMR (400 MHz, CDCl3) δ 6.99 (s, 1H, 12-H), 6.18 (s, 1H, 1-NH), 5.08 (ddd, 2JHP = 16.4 Hz, J = 9.5, 9.5 Hz, 1H, 1-H), 4.55 (m, 1H, 2-H), 4.27–3.86 (m, 5H, 1-P(OCH2)2), 3.10–2.86 (m, 3H, 4-H, 17-H2), 2.71 (br. dd, J = 6.0, 6.0 Hz, 2H, 14-H2), 1.85–1.70 (m, 6H, 6-H, 7-H, 15-H2, 16-H2), 1.58 (m, 3H, 5-H, 6-H, 7-H), 1.48 (s, 9H, 2-NCq(O)OCq–(CH3)3), 1.40 (m, 1H, 5-H), 1.29 (t, J = 7.0 Hz, 6H, 1-POCH2CH3). 13C NMR (126 MHz, CDCl3) δ 162.4 (d, J = 4.6 Hz, 9-Cq), 154.9 (2-N–Cq(O)–O), 141.9 (13-Cq), 140.7 (18-Cq), 129.0 (10-Cq), 122.7 (12-CH), 79.8 (2-NCq(O)O–Cq–(CH3)3), 62.8 (br. s, 1-POCH2), 62.6 (d, J = 6.6 Hz, 1-POCH2), 51.3 (d, 2JCP = 2.9 Hz, 2-CH), 44.8 (d, 1JCP = 155.3 Hz, 1-CH), 40.6 (4-CH2), 28.6 (2-NCq(O)OCq–(CH3)3), 27.0 (17-CH2), 26.6 (14-CH2), 25.5 (d, 3JCP = 9.5 Hz, 7-CH2), 24.8 (5-CH2), 23.1 (16-CH2), 22.9 (15-CH2), 19.5 (6-CH2), 16.6 (d, 3JCP = 5.7 Hz, 1-POCH2CH3), 16.5 (d, 3JCP = 6.0 Hz, 1-POCH2CH3). 31P NMR (162 MHz, CDCl3) δ 22.81, 22.42. HRESIMS m/z 515.2312 [M + H]+ (515.2339 calcd for C22H38N2O5P).
Compound 4f-2, diastereomer 2 (rotamer ratio 3.5
:
1)
1H NMR (400 MHz, CDCl3) δ 6.96 (s, 1H, 12-H), 6.34 (d, J = 9.8 Hz, 1H, 1-NH), 5.06 (ddd, 2JHP = 15.3 Hz, J = 11.6, 9.8 Hz, 1H, 1-H), 4.68 (m, 1H, 2-H), 4.25–4.09 (m, 4H, 1-P(OCH2)2), 3.85 (br. dd, J = 13.6, 2.6 Hz, 1H, 4-H), 3.08–2.91 (m, 2H, 14-CH2 or 17-CH2), 2.87 (ddd, J = 13.4, 13.4, 2.8 Hz, 1H, 4-H), 2.75–2.57 (m, 2H, 14-CH2 or 17-CH2), 2.41 (m, J = 9.0 Hz 1H, 7-H), 1.91–1.56 (m, 8H, 5-H, 6-H2, 7-H, 15-CH2, 16-CH2), 1.39 (m, 1H, 5-H), 1.34 (t, J = 7.1 Hz, 3H, 1-POCH2CH3), 1.28 (t, J = 7.1 Hz, 3H, 1-POCH2CH3), 1.20 (s, 9H, 2-NCq(O)OCq–(CH3)3). 13C NMR (101 MHz, CDCl3) δ 162.6 (d, J = 4.4 Hz, 9-Cq), 156.6 (2-N–Cq(O)–O), 140.8 (13-Cq or 18-Cq), 140.2 (13-Cq or 18-Cq), 130.9 (10-Cq), 123.1 (12-CH), 79.9 (2-NCq(O)O–Cq–(CH3)3), 62.9 (d, 2JCP = 6.1 Hz, 1-POCH2), 62.7 (d, 2JCP = 7.2 Hz, 1-POCH2), 49.9 (d, 2JCP = 12.3 Hz, 2-CH), 45.3 (d, 1JCP = 151.9 Hz, 1-CH), 40.2 (4-CH2), 28.1 (2-NCq(O)OCq–(CH3)3), 26.7 (14-CH2 or 17-CH2), 26.6 (14-CH2 or 17-CH2), 26.2 (7-CH2), 25.2 (5-CH2), 23.2 (15-CH2 or 16-CH2), 22.9 (15-CH2 or 16-CH2), 19.2 (6-CH2), 16.7 (d, 3JCP = 5.5 Hz, 1-POCH2CH3), 16.5 (d, 3JCP = 6.0 Hz, 1-POCH2CH3). 31P NMR (162 MHz, CDCl3) δ (23.42), 23.15. HRESIMS m/z 515.2329 [M + H]+ (515.2339 calcd for C22H38N2O5P).
tert-Butyl 2-((benzo[b]thiophene-2-carboxamido)(di-ethoxyphosphoryl)methyl)piperidine-1-carboxylate (4g)
The synthesis was performed according to the general procedure A, 3 (236 mg, 0.673 mmol) and benzo[b]thiophene-2-carboxylic acid (238 mg, 1.34 mmol, 2.0 equiv.) gave the total product (270 mg, 0.529 mmol, 79%). 4g-1 was obtained as a colorless solid (110 mg, 0.215 mmol, 32%). 4g-2 was obtained as a colorless solid (153 mg, 0.300 mmol, 44%).
Compound 4g-1, diastereomer 1
1H NMR (400 MHz, CDCl3) δ 7.90 (s, 1H, 18-H), 7.86 (dd, J = 8.1, 1.1 Hz, 1H, 13-H), 7.84 (dd, J = 7.7, 1.7 Hz, 1H, 16-H), 7.43 (ddd, J = 7.1, 7.1, 1.7 Hz, 1H, part C of ABCD, 14-CH), 7.40 (ddd, J = 7.1, 7.1, 1.6 Hz, 1H, part D of ABCD, 15-CH), 7.00 (s, 1H, 1-NH), 5.14 (m, 1H, 1-H), 4.65 (m, 1H, 2-H), 4.22–3.91 (m, 5H, 1-P(OCH2)2, 4-H), 2.92 (m, 1H, 4-H), 1.85 (m, 1H, 7-H), 1.75 (m, 1H, 6-H), 1.66–1.54 (m, 3H, 5-H, 6-H, 7-H), 1.49 (s, 9H, 2-NCq(O)OCq–(CH3)3), 1.42 (m, 1H, 5-H), 1.31 (t, J = 7.1 Hz, 3H, 1-POCH2CH3), 1.24 (t, J = 7.2 Hz, 3H, 1-POCH2CH3). 13C NMR (126 MHz, CDCl3) δ 160.8 (d, J = 5.0 Hz, 9-Cq), 153.9 (2-N–Cq(O)–O), 140.2 (12-Cq), 138.1 (17-Cq), 136.8 (10-Cq), 125.5 (14-CH), 124.7 (18-CH), 124.2 (16-CH), 123.9 (15-CH), 121.7 (13-CH), 78.8 (2-NCq(O)O–Cq–(CH3)3), 62.2 (br s, 1-POCH2), 61.5 (br s, 1-POCH2), 50.1 (2-CH), 44.7 (d, 1JCP = 148.9 Hz, 1-CH), 39.4 (4-CH2), 27.4 (2-NCq(O)OCq–(CH3)3), 24.3 (d, 3JCP = 9.8 Hz, 7-CH2), 23.7 (5-CH2), 18.3 (6-CH2), 15.5 (d, 3JCP = 5.6 Hz, 1-POCH2CH3), 15.3 (d, 3JCP = 6.3 Hz, 1-POCH2CH3). 31P NMR (162 MHz, CDCl3) δ 22.79, 22.43. HRESIMS m/z 511.2017 [M + H]+ (511.2026 calcd for C24H36N2O6PS).
Compound 4g-2, diastereomer 2 (rotamer ratio 6.6
:
1)
1H NMR (400 MHz, CDCl3) δ 7.84 (d, J = 7.9, 1.2 Hz, 1H, 13-CH), 7.78 (dd, J = 7.1, 1.6 Hz, 1H, 16-H), 7.73 (s, 1H, 18-H), 7.41 (ddd, J = 7.4, 7.4, 1.4 Hz, 1H, part C of ABCD 14-CH), 7.37 (ddd, J = 7.4, 7.4, 1.4 Hz, 1H, part D of ABCD, 15-CH), 6.90 (d, J = 9.5 Hz, 1H, 1-NH), 5.05 (ddd, 2JHP = 15.0 Hz, J = 11.6, 9.5 Hz, 1H, 1-H), 4.75 (m, 1H, 2-H), 4.32–4.14 (m, 4H, 1-P(OCH2)2), 3.89 (dd, J = 14.0, 4.0 Hz, 1H, 4-H), 2.82 (ddd, J = 13.3, 13.3, 2.7 Hz, 1H, 4-H), 2.38 (br. d, J = 12.4 Hz, 1H, 7-H), 1.80–1.58 (m, 4H, 5-H, 5-H2, 7-H), 1.41 (m, 1H, 5-H), 1.33 (t, J = 7.1 Hz, 3H, 1-POCH2CH3), 1.30 (t, J = 7.1 Hz, 3H, 1-POCH2CH3), 1.23 (s, 9H, 2-NCq(O)OCq–(CH3)3). 13C NMR (126 MHz, CDCl3) δ 161.8 (d, J = 3.6 Hz, 9-Cq), 156.9 (2-N–Cq(O)–O), 141.4 (12-Cq), 139.2 (17-Cq), 138.2 (10-Cq), 126.5 (14-CH), 125.3 (18-CH), 125.1 (16-CH), 125.0 (15-CH), 122.8 (13-CH), 80.3 (2-NCq(O)O–Cq–(CH3)3), 63.1 (d, 2JCP = 6.3 Hz, 1-POCH2), 63.0 (d, 2JCP = 7.5 Hz, 1-POCH2), 50.0 (d, 2JCP = 11.7 Hz, 2-H), 46.2 (d, 1JCP = 152.7 Hz, 1-H), 40.2 (4-CH2), 28.3 (2-NCq(O)OCq–(CH3)3), 26.3 (7-CH2), 25.2 (5-CH2), 19.2 (7-CH2), 16.7 (d, 3JCP = 5.4 Hz, 1-POCH2CH3), 16.5 (d, 3JCP = 6.3 Hz, 1-POCH2CH3). 31P NMR (162 MHz, CDCl3) δ (22.97), 22.53. HRESIMS m/z 511.2029 [M + H]+ (511.2026 calcd for C24H36N2O6PS).
tert-Butyl 2-((benzo[b]thiophene-3-carboxamido)(di-ethoxyphosphoryl)methyl)piperidine-1-carboxylate (4h)
Synthesis was performed according to the general procedure A, 3 (241 mg, 0.689 mmol) and benzo[b]thiophene-3-carboxylic acid (245 mg, 1.38 mmol, 2.0 equiv.) gave the total product (257 mg, 0.503 mmol, 73%). 4h-1 was obtained as a colorless solid (104 mg, 0.204 mmol, 30%). 4h-2 was obtained as a colorless solid (153 mg, 0.300 mmol, 44%).
Compound 4h-1, diastereomer 1
1H NMR (400 MHz, CDCl3) δ 8.43 (d, J = 7.8 Hz, 1H, 17-H), 8.07 (br. s, 1H, 11-H), 7.87 (d, J = 7.8 Hz, 1H, 14-H), 7.45 (ddd, J = 7.6, 7.6, 1.3 Hz, 1H, 16-CH), 7.40 (ddd, J = 7.6, 7.2, 1.4 Hz, 1H, 15-CH), 7.11 (m, 1H, 1-NH), 5.20 (ddd, 2JHP = 17.7 Hz, J = 9.9, 9.9 Hz, 1H, 1-H), 4.66 (m, 1H, 2-H), 4.25–3.92 (m, 5H, 1-P(OCH2)2, 4-H), 3.05 (m, 1H, 4-H), 2.13–1.72 (m, 2H, 7-H, 6-H), 1.67–1.55 (m, 3H, 5-H, 6-H, 7-H), 1.48 (s, 9H, 2-NCq(O)OCq–(CH3)3), 1.40 (m, 1H, 5-H), 1.31 (t, J = 7.1 Hz, 3H, 1-POCH2CH3), 1.24 (t, J = 6.9 Hz, 3H, 1-POCH2CH3). 13C NMR (126 MHz, CDCl3) δ 163.4 (d, J = 4.7 Hz, 9-Cq), 155.0 (2-N–Cq(O)–O), 140.3 (13-Cq), 137.1 (18-Cq), 131.1 (10-Cq), 130.6 (br. s, 11-Cq), 125.4 (16-CH), 125.3 (15-CH), 124.5 (17-CH), 122.6 (14-CH), 79.9 (2-NCq(O)O–Cq–(CH3)3), 63.1 (d, 2JCP = 4.4 Hz, 1-POCH2), 62.6 (d, 2JCP = 5.5 Hz, 1-POCH2), 51.2 (2-CH), 44.9 (d, 1JCP = 155.7 Hz, 1-CH), 40.6 (4-CH2), 28.6 (2-NCq(O)OCq–(CH3)3), 25.5 (d, 3JCP = 10.1 Hz, 7-CH2), 24.9 (5-CH2), 19.5 (6-CH2), 16.6 (d, 3JCP = 5.6 Hz, 1-POCH2CH3), 16.5 (d, 3JCP = 6.2 Hz, 1-POCH2CH3). 31P NMR (162 MHz, CDCl3) δ 22.71. HRESIMS m/z 511.2016 [M + H]+ (511.2026 calcd for C24H36N2O6PS).
Compound 4h-2, diastereomer 2 (rotamer ratio 4.8
:
1)
1H NMR (400 MHz, CDCl3) δ 8.46 (d, J = 7.9 Hz, 1H, 17-H), 7.92 (s, 1H, 11-H), 8.46 (d, J = 7.9 Hz, 1H, 14-H), 7.45 (ddd, J = 8.2, 7.1, 1.2 Hz, 1H, 16-H), 7.39 (ddd, J = 8.0, 6.9, 1.2 Hz, 1H, 15-H), 6.66 (d, J = 9.9 Hz, 1H, 1-NH), 5.13 (ddd, 2JHP = 15.4 Hz, J = 11.6, 9.8 Hz, 1H, 1-H), 4.74 (m, 1H, 2-H), 4.27–4.15 (m, 4H, 1-P(OCH2)2), 3.89 (ddd, J = 13.6, 4.7, 2.1 Hz, 1H, 4-H), 2.90 (ddd, J = 13.4, 13.4, 2.8 Hz, 1H, 4-H), 2.42 (m, 1H, 7-H), 1.77–1.58 (m, 4H, 5-H, 6-H2, 7-H), 1.43 (m, 1H, 5-H), 1.35 (t, J = 7.1 Hz, 3H, 1-POCH2CH3), 1.31 (t, J = 7.2 Hz, 3H, 1-POCH2CH3), 1.16 (s, 9H, 2-NCq(O)OCq–(CH3)3) ppm. 31P NMR (162 MHz, CDCl3) δ (23.40), 22.99 ppm. HRESIMS m/z 511.2008 [M + H]+ (511.2026 calcd for C24H36N2O6PS).
tert-Butyl 2-((diethoxyphosphoryl)(2-(thiophen-2-yl)-acetamido)methyl)piperidine-1-carboxylate (4i)
The synthesis was performed according to general procedure A, 3 (255 mg, 0.897 mmol) and 2-(thiophen-2-yl)acetic acid (255 mg, 1.79 mmol, 2.0 equiv.) gave the total product (256 mg, 0.539 mmol, 60%). 4i-1 was obtained as a colorless solid (125 mg, 0.238 mmol, 27%). 4i-2 was obtained as a colorless solid (139 mg, 0.293 mmol, 33%).
Compound 4i-1, diastereomer 1
1H NMR (400 MHz, CDCl3) δ 7.23 (dd, J = 4.7, 1.7 Hz, 1H, 13-CH), 6.98 (dd, J = 3.5, 3.5 Hz, 1H, 14-H), 6.97 (m, 1H, 15-H), 6.46 (m, 1H, 1-NH), 4.90 (ddd, 2JHP = 16.0 Hz, J = 10.2, 10.2 Hz, 1H, 1-H), 4.41 (m, 1H, 2-H), 4.16–3.88 (m, 5H, 1-P(OCH2)2, 4-H), 3.83 (s, 2H, 10-H2), 2.85 (m, 1H, 4-H), 1.69–1.42 (m, 5H, 5-H, 6-H2, 7-H2), 1.44 (s, 9H, 2-NCq(O)OCq–(CH3)3), 1.37 (m, 1H, 5-H), 1.27 (t, J = 7.1 Hz, 3H, 1-POCH2CH3), 1.22 (t, J = 7.1 Hz, 3H, 1-POCH2CH3). 13C NMR (101 MHz, CDCl3) δ 169.5 (d, J = 3.7 Hz, 9-Cq), 154.6 (2-N–Cq(O)–O), 135.9 (11-Cq), 127.4 (14-CH or 15-CH), 127.4 (14-CH or 15-CH), 125.6 (13-CH), 79.8 (2-NCq(O)O–Cq–(CH3)3), 62.9 (d, 2JCP = 7.2 Hz, 1-POCH2), 62.4 (d, 2JCP = 6.5 Hz, 1-POCH2), 50.7 (2-CH), 44.8 (d, 1JCP = 155.2 Hz, 1-CH), 40.6 (4-CH2), 37.5 (10-CH2), 28.5 (2-NCq(O)OCq–(CH3)3), 25.2 (d, 3JCP = 9.8 Hz, 7-CH2), 24.9 (5-CH2), 19.4 (6-CH2), 16.5 (d, 3JCP = 6.0 Hz, 1-POCH2CH3), 16.5 (d, 3JCP = 6.5 Hz, 1-POCH2CH3). 31P NMR (162 MHz, CDCl3) δ 22.15. HRESIMS m/z 475.1967 [M + H]+ (475.2026 calcd for C21H36N2O6PS).
Compound 4i-2, diastereomer 2 (rotamer ratio 3.2
:
1)
1H NMR (400 MHz, CDCl3) δ 7.21 (d, J = 5.0 Hz, 1H, 13-H), 6.96 (dd, J = 5.0, 3.5 Hz, 1H, 14-H), 6.93 (m, 1H, 15-H), 6.10 (m, 1H, 1-NH), 4.84 (ddd, 2JHP = 15.4 Hz, J = 11.5, 10.1 Hz, 1H, 1-H), 4.55 (m, 1H, 2-H), 4.19–3.98 (m, 4H, 1-P(OCH2)2), 3.88 (m, 1H, 4-H), 3.69 (s, 2H, 10-H2), 2.76 (m, 1H, 4-H), 2.30 (m, 1H, 7-H), 1.70–1.53 (m, 4H, 5-H, 6-H2, 7-H), 1.45 (s, 9H, 2-NCq(O)OCq–(CH3)3), 1.35 (m, 1H, 5-H), 1.25 (t, J = 7.1 Hz, 6H, 1-P(OCH2CH3)2). 13C NMR (101 MHz, CDCl3) δ 169.5 (d, J = 3.2 Hz, 9-Cq), 156.3 (2-N–Cq(O)–O), 135.8 (11-Cq), 127.2 (14-CH), 127.1 (15-CH), 125.4 (13-CH), 80.0 (2-NCq(O)O–Cq–(CH3)3), 62.9 (br. s, 1-POCH2), 62.6 (br. s, 1-POCH2), 49.9 (2-CH), 45.5 (d, 1JCP = 163.1 Hz, 1-CH), 40.1 (4-CH2), 37.7 (10-CH2), 28.6 (2-NCq(O)OCq–(CH3)3), 26.3 (7-CH2), 25.2 (5-CH2), 19.1 (6-CH2), 16.5 (d, 3JCP = 5.6 Hz, 1-POCH2CH3), 16.4 (d, 3JCP = 6.2 Hz, 1-POCH2CH3). 31P NMR (162 MHz, CDCl3) δ (22.97), 22.44. HRESIMS m/z 475.1997 [M + H]+ (475.2026 calcd for C21H36N2O6PS).
tert-Butyl 2-((diethoxyphosphoryl)(2-(thiophen-3-yl)-acetamido)methyl)piperidine-1-carboxylate (4j)
The synthesis was performed according to general procedure A, 3 (235 mg, 0.670 mmol) and 2-(thiophene-3-yl)acetic acid (191 mg, 1.34 mmol, 2.0 equiv.) gave the total product (254 mg, 0.535 mmol, 80%). 4j-1 was obtained as s slightly yellow solid (109 mg, 0.230 mmol, 34%). 4j-2 was obtained as a slightly yellow solid (145 mg, 0.306 mmol, 46%).
Compound 4j-1, diastereomer 1
1H NMR (400 MHz, CDCl3) δ 7.32 (dd, J = 5.0, 2.9 Hz, 1H, 14-H), 7.18 (d, J = 2.2 Hz, 1H, 12-H), 7.01 (d, J = 5.0 Hz, 1H, 15-H), 6.09 (br. s, 1H, 1-NH), 4.90 (ddd, 2JCP = 17.2 Hz, J = 10.2, 10.2 Hz, 1H, 1-H), 4.37 (m, 1H, 2-H), 4.14–3.89 (m, 5H, 1-P(OCH2)2, 4-H), 3.63 (s, 2H, 10-H2), 2.85 (m, 1H, 4-H), 1.63–1.48 (m, 5H, 5-H, 6-H2, 7-H2), 1.45 (s, 9H, 2-NCq(O)OCq–(CH3)3), 1.35 (m, 1H, 5-H), 1.27 (t, J = 7.1 Hz, 3H, 1-POCH2CH3), 1.22 (t, J = 7.1 Hz, 3H, 1-POCH2CH3). 13C NMR (126 MHz, CDCl3) δ 170.0 (9-Cq), 154.6 (2-N–Cq(O)–O), 134.5 (11-Cq), 128.5 (15-CH), 126.8 (14-CH), 123.5 (12-CH), 79.8 (2-NCq(O)O–Cq–(CH3)3), 62.7 (br. s, 1-POCH2), 62.5 (d, 2JCP = 6.6 Hz, 1-POCH2), 50.8 (2-CH), 44.7 (d, 1JCP = 154.7 Hz, 1-CH), 40.5 (4-CH2), 38.2 (10-CH2), 28.5 (2-NCq(O)OCq–(CH3)3), 25.2 (d, 3JCP = 9.7 Hz, 7-CH2), 24.8 (5-CH2), 19.4 (6-CH2), 16.5 (d, 3JCP = 5.9 Hz, 1-POCH2CH3), 16.5 (d, 3JCP = 6.6 Hz, 1-POCH2CH3). 31P NMR (162 MHz, CDCl3) δ 22.37. HRESIMS m/z 475.2032 [M + H]+ (475.2026 calcd for C21H36N2O6PS).
Compound 4j-2, diastereomer 2 (rotamer ratio 2.8
:
1)
1H NMR (400 MHz, CDCl3) δ 7.27 (dd, J = 5.0, 3.1 Hz, 1H, 14-H), 7.15–7.12 (m, 1H, 12-H), 7.00 (dd, J = 5.0, 1.3 Hz, 1H, 15-H), 6.12 (d, J = 9.9 Hz, 1H, 1-NH), 4.84 (ddd, 2JCP = 15.5 Hz, J = 11.6, 10.0 Hz, 1H, 1-H), 4.56 (m, 1H, 2-H), 4.19–3.95 (m, 4H, 1-P(OCH2)2), 3.88 (m, 1H, 4-H), 3.50 (s, 2H, 10-H), 2.78 (dd, J = 13.4, 13.4, 2.6 Hz, 1H, 4-H), 2.32 (m, 1H, 7-H), 1.67–1.55 (m, 4H, 5-H, 6-H2, 7-H), 1.44 (s, 9H, 2-NCq(O)OCq–(CH3)3), 1.36 (m, 1H, 5-H), 1.23 (t, J = 6.9 Hz, 3H, 1-POCH2CH3), 1.22 (t, J = 6.7 Hz, 3H, 1-POCH2CH3). 13C NMR (126 MHz, CDCl3) δ 169.9 (d, 3JCP = 3.6 Hz, 9-Cq), 156.4 (2-N–Cq(O)–O), 134.5 (11-Cq), 128.5 (15-CH), 126.2 (14-CH), 123.0 (12-CH), 80.0 (2-NCq(O)O–Cq–(CH3)3), 62.8 (d, 2JCP = 6.0 Hz, 1-POCH2), 62.6 (d, 2JCP = 7.2 Hz, 1-POCH2), 49.8 (d, 2JCP = 12.0 Hz, 2-CH), 45.3 (d, 1JCP = 151.5 Hz, 1-CH), 40.1 (4-CH2), 38.3 (10-CH2), 28.6 (2-NCq(O)OCq–(CH3)3), 26.2 (7-CH2), 25.2 (5-CH2), 19.2 (6-CH2), 16.5 (d, 3JCP = 5.4 Hz, 1-POCH2CH3), 16.4 (d, 3JCP = 6.2 Hz, 1-POCH2CH3). 31P NMR (162 MHz, CDCl3) δ (23.29), 22.69. HRESIMS m/z 475.2012 [M + H]+ (475.2026 calcd for C21H36N2O6PS).
tert-Butyl 2-((2-(benzo[b]thiophen-2-yl)acetamido)(di-ethoxyphosphoryl)methyl)piperidine-1-carboxylate (4k)
The synthesis was performed according to general procedure A, 3 (308 mg, 0.879 mmol) and 2-(benzo[b]thiophen-2-yl)acetic acid (330 mg, 1.72 mmol, 2.0 equiv.) gave the total product (280 mg, 0.534 mmol, 61%). 4k-1 was obtained as a slightly orange solid (125 mg, 0.238 mmol, 27%). 4k-2 was obtained as a slightly orange solid (154 mg, 0.294 mg, 33%).
Compound 4k-1, diastereomer 1
1H NMR (400 MHz, CDCl3) δ 7.74 (dd, J = 7.8, 1.0 Hz, 1H, 14-H), 7.67 (dd, J = 6.9, 1.1 Hz, 1H, 17-H), 7.30 (ddd, J = 7.3, 7.3, 1.3 Hz, 1H, part C of ABCD, 16-H), 7.24 (ddd, J = 7.3, 7.3, 1.3 Hz, 1H, part D of ABCD, 15-H), 7.18 (d, J = 1.1 Hz, 1H, 19-H), 7.00 (br. s, 1H, 1-NH), 4.89 (m, 1H, 1-H), 4.44 (m, 1H, 2-H), 4.16–3.90 (m, 5H, 1-P(OCH2)2, 4-H), 3.88 (s, 2H, 10-H2), 2.99–2.69 (m, 1H, 4-H), 1.73–1.41 (m, 5H, 5-H, 6-H2, 7-H2), 1.40 (s, 9H, 2-NCq(O)OCq–(CH3)3), 1.34 (m, 1H, 5-H), 1.24 (t, J = 7.1 Hz, 3H, 1-POCH2CH3), 1.12 (t, J = 7.1 Hz, 3H, 1-POCH2CH3). 13C NMR (126 MHz, CDCl3) δ 168.9 (9-Cq), 154.5 (2-N–Cq(O)–O), 140.1 (13-Cq), 139.8 (18-Cq), 137.2 (11-Cq), 124.4 (16-CH), 124.2 (14-CH), 123.8 (19-CH), 123.3 (17-CH), 122.2 (14-CH), 79.6 (2-NCq(O)O–Cq–(CH3)3), 62.8 (d, 2JCP = 7.1 Hz, 1-POCH2), 62.3 (d, 2JCP = 6.7 Hz, 1-POCH2), 50.7 (2-CH), 44.7 (d, 1JCP = 156.0 Hz, 1-CH), 40.4 (4-CH2), 38.28 (10-CH2), 28.4 (2-NCq(O)OCq–(CH3)3), 25.2 (d, 3JCP = 10.0 Hz, 7-CH2), 24.8 (5-CH2), 19.3 (6-CH2), 16.4 (d, 3JCP = 5.9 Hz, 1-P(OCH2CH3)2). 31P NMR (162 MHz, CDCl3) δ 22.30. HRESIMS m/z 525.2177 [M + H]+ (525.2183 calcd for C25H38N2O6PS).
Compound 4k-2, diastereomer 2 (rotamer ratio 2.7
:
1)
1H NMR (500 MHz, CDCl3) δ 7.69 (d, J = 7.8 Hz, 1H, 14-H), 7.62 (d, J = 7.5 Hz, 1H, 17-H), 7.24 (m, 1H, 16-H), 7.21 (m, 1H, 15-H), 7.09 (s, 1H, 19-H), 6.43 (m, 1H, 1-NH), 4.80 (ddd, 2JHP = 15.5 Hz, J = 10.7, 10.7 Hz, 1H, 1-H), 4.51 (m, 1H, 2-H), 4.10–3.89 (m, 4H, 1-P(OCH2)2), 3.83 (m, 1H, 4-H), 3.70 (d, 2J = 15.9 Hz, 1H, part A of AB, 10-H), 3.68 (d, 2J = 15.9 Hz, 1H, part B of AB, 10-H), 2.74 (ddd, J = 13.4, 13.4, 2.6 Hz, 1H, 4-H), 2.25 (br. d, J = 10.5 Hz, 1H, 7-H), 1.60–1.49 (m, 4H, 5-H, 6-H2, 7-H), 1.37 (s, 9H, 2-NCq(O)OCq–(CH3)3), 1.32 (m, 1H, 5-H), 1.15 (t, J = 7.1 Hz, 3H, 1-POCH2CH3), 1.08 (t, J = 7.0 Hz, 3H, 1-POCH2CH3). 13C NMR (126 MHz, CDCl3) δ 168.5 (d, J = 3.3 Hz, 9-Cq), 156.2 (2-N–Cq(O)–O), 140.0 (13-Cq), 139.7 (18-Cq), 137.1 (11-Cq), 124.3 (16-CH), 124.1 (15-CH), 123.3 (19-CH), 123.2 (17-CH), 122.0 (14-CH), 79.9 (2-NCq(O)O–Cq–(CH3)3), 62.7 (d, 2JCP = 5.2 Hz, 1-POCH2), 62.6 (d, 2JCP = 7.3 Hz, 1-POCH2), 49.7 (d, 2JCP = 11.9 Hz, 2-CH), 45.4 (d, 1JCP = 151.6 Hz, 1-CH), 40.0 (4-CH2), 38.3 (10-CH2), 28.4 (2-NCq(O)OCq–(CH3)3), 26.1 (7-CH2), 25.1 (5-CH2), 19.0 (6-CH2), 16.3 (d, 3JCP = 3.9 Hz, 1-POCH2CH3), 16.2 (d, 3JCP = 4.9 Hz, 1-POCH2CH3). 31P NMR (162 MHz, CDCl3) δ (22.87), 22.42. HRESIMS m/z 525.2191 [M + H]+ (525.2183 calcd for C25H38N2O6PS).
tert-Butyl 2-((2-(benzo[b]thiophen-3-yl)acetamido)(di-ethoxyphosphoryl)methyl)piperidine-1-carboxylate (4l)
The synthesis was performed according to general procedure A, 3 (301 mg, 0.860 mmol) and 2-(benzo[b]thiophen-3-yl)acetic acid (330 mg, 1.72 mmol, 2.0 equiv.) gave the total product (304 mg, 0.579 mg, 68%). 4l-1 was obtained as a colorless solid (141 mg, 0.269 mmol, 31%). 4l-2 was obtained as a colorless solid (163 mg, 0.311 mmol, 36%).
Compound 4l-1, diastereomer 1
1H NMR (400 MHz, CDCl3) δ 7.86 (m, 1H, 15-H), 7.78 (m, 1H, 18-H), 7.44–7.32 (m, 3H, 16-H, 17-H, 12-H), 6.30 (br. s, 1H, 1-NH), 4.88 (ddd,2JPH = 18.8 Hz, J = 10.5, 10.5 Hz, 1H, 1-H), 4.32 (m, 1H, 2-H), 4.07–3.88 (m, 4H, 1-P(OCH2)2), 3.86 (s, 2H, 10-H2), 3.83 (m, 1H, 4-H), 2.82 (m, 1H, 4-H), 1.61–1.47 (m, 4H, 5-H, 6-H2, 7-H), 1.41 (s, 9H, 2-NCq(O)OCq–(CH3)3), 1.38–1.27 (m, 2H, 5-H, 7-H), 1.23 (t, J = 7.1 Hz, 3H, 1-POCH2CH3), 1.09 (t, J = 7.1 Hz, 3H, 1-POCH2CH3). 13C NMR (126 MHz, CDCl3) δ 169.5 (9-Cq), 154.7 (2-N–Cq(O)–O), 140.6 (14-Cq), 138.4 (19-Cq), 129.2 (11-Cq), 125.3 (12-CH), 124.9 (16-CH), 124.5 (17-CH), 123.0 (15-CH), 122.1 (18-CH), 79.7 (2-NCq(O)O–Cq–(CH3)3), 62.6 (d, 2JCP = 4.3 Hz, 1-POCH2), 62.4 (d, 2JCP = 6.5 Hz, 1-POCH2), 50.7 (2-CH), 44.8 (d, 1JCP = 154.6 Hz, 1-CH), 40.4 (4-CH2), 36.89 (10-CH2), 28.5 (2-NCq(O)OCq–(CH3)3), 25.2 (d, 3JCP = 9.4 Hz, 7-CH2), 24.7 (5-CH2), 19.27 (6-CH2), 16.4 (d, 3JCP = 6.1 Hz, 1-POCH2CH3), 16.4 (d, 3JCP = 5.9 Hz, 1-POCH2CH3). 31P NMR (162 MHz, CDCl3) δ 22.05. HRESIMS m/z 525.2181 [M + H]+ (525.2183 calcd for C25H38N2O6PS).
Compound 4l-2, diastereomer 2 (rotamer ratio 2.5
:
1)
1H NMR (400 MHz, CDCl3) δ 7.84 (d, J = 7.0 Hz, 1H, 15-H), 7.74 (d, J = 7.5 Hz, 1H, 18-H), 7.38 (dd, J = 7.0, 7.0 Hz, 1H, 17-H), 7.35 (m, 1H, 12-H), 7.33 (m, 1H, 16-H), 6.17 (d, J = 9.9 Hz, 1H, 1-NH), 4.84 (ddd, 2JHP = 15.4 Hz, J = 11.7, 9.9 Hz, 1H, 1-H), 4.55 (m, 1H, 2-H), 4.14–3.80 (m, 5H, 1-P(OCH2)2, 4-H), 3.71 (s, 2H, 10-H2), 2.82 (ddd, J = 13.5, 13.5, 2.6 Hz, 1H, 4-H), 2.34 (m, 1H, 7-H), 1.67–1.55 (m, 4H, 5-H, 6-H2, 7-H), 1.46 (s, 9H, 2-NCq(O)OCq–(CH3)3), 1.43 (m, 1H, 5-H), 1.14 (t, J = 7.1 Hz, 3H, 1-POCH2CH3), 1.08 (t, J = 7.1 Hz, 3H, 1-POCH2CH3).13C NMR (126 MHz, CDCl3) δ 169.2 (d, J = 3.6 Hz, 9-Cq), 156.3 (2-N–Cq(O)–O), 140.3 (14-Cq), 138.4 (19-Cq), 129.1 (11-Cq), 124.5 (16-CH or 12-CH), 124.5 (16-CH or 12-CH), 124.2 (17-CH), 122.8 (15-CH), 121.9 (18-CH), 79.9 (2-NCq(O)O–Cq–(CH3)3), 62.7 (d, 2JCP = 6.0 Hz, 1-POCH2), 62.4 (d, 2JCP = 7.3 Hz, 1-POCH2), 49.7 (d, 2JCP = 11.9 Hz, 2-CH), 45.3 (d, 1JCP = 151.5 Hz, 1-CH), 40.1 (4-CH2), 36.8 (10-CH2), 28.5 (OCq–(CH3)3), 26.1 (7-CH2), 25.1 (5-CH2), 19.1 (6-CH2), 16.2 (d, 3JCP = 1.6 Hz, 1-POCH2CH3), 16.2 (d, 3JCP = 2.2 Hz, 1-POCH2CH3). 31P NMR (162 MHz, CDCl3) δ (22.93), 22.32. HRESIMS m/z 525.2153 [M + H]+ (525.2183 calcd for C25H38N2O6PS).
General procedure B for 5a–l
Corresponding compounds 4 and 3-mercaptopropyl-functionalized silica gel (3.5 equiv., 1.2 mmol g−1 loading) were dried under high vacuum for at least 30 min. Dry MeCN and Et3N (6.0 equiv.) were added, and the mixture was stirred at r.t. for 5 min. The reaction mixture was subsequently cooled to 0 °C. TMSBr (5.0 equiv.) in MeCN was added dropwise. The reaction was stirred for 12–48 h. All the compounds were converted to HCl salts by adding 0.1 M HCl solution (aq) and the solvent was removed by freezedrying, due to poor solubility of either the free base or formate salts.
((2-(4-Methyl-2-thioxo-2,3-dihydrothiazol-5-yl)acet-amido)(piperidin-2-yl)methyl)phosphonic acid hydro-chloride (5a)
The synthesis was performed according to general procedure B. Compound 4a-1 (50.8 mg, 0.0974 mmol) gave 5a-1 as a slightly orange solid (18.8 mg, 0.0515 mmol, 48%). Compound 4a-2 (50.6 mg, 0.0970 mmol) gave 5a-2 as a slightly orange solid (8.6 mg, 0.0235 mmol, 22%).
Compound 5a-1, diastereomer 1
1H NMR (500 MHz, DMSO-d6) δ 12.94 (s, 1H, 15-NH), 8.32 (s, 1H, 1-NH), 3.96 (dd, 2JHP = 15.9 Hz, J = 8.5, 8.5 Hz, 1H, 1-H), 3.55 (d, 2J = 17.3 Hz, 1H, part A of AB, 10-H), 3.54 (d, 2J = 17.3 Hz, 1H, part B of AB, 10-H), 3.23 (br. d, 12.3 Hz, 1H, 4-H), 3.09 (ddd, J = 8.8, 8.8, 8.8 Hz, 1H, 2-H), 2.84 (ddd, J = 12.0, 12.0, 2.3 Hz, 1H, 4-H), 2.06 (s, 3H, 15-CH3), 1.81 (br. d, J = 10.5 Hz, 1H, 7-H), 1.76–1.59 (m, 2H, 5-H, 6-H), 1.52 (m, 1H, 6-H), 1.45–1.30 (m, 2H, 5-H, 7-H). 13C NMR (126 MHz, DMSO-d6) δ 186.9 (13-Cq), 168.9 (d, 3JCP = 6.2 Hz, 9-Cq), 134.7 (15-Cq), 117.5 (11-Cq), 57.3 (2-CH), 48.6 (d, 1JCP = 134.7 Hz, 1-CH), 43.7 (4-CH2), 32.2 (10-CH2), 25.0 (d, 3JCP = 7.6 Hz, 7-CH2), 22.0 (5-CH2), 21.6 (6-CH2), 11.4 (15-CH3). 31P NMR (162 MHz, DMSO-d6) δ 11.71. HRESIMS m/z 366.0718 [M + H–Cl]+ (366.0706 calcd for C12H21N3O4PS2).
Compound 5a-2, diastereomer 2
1H NMR (500 MHz, DMSO-d6) δ 12.92 (s, 1H, 15-NH), 8.69 (s, 1H, 1-NH), 4.01 (ddd, 2JHP = 17.1 Hz, J = 8.8, 3.5 Hz, 1H, 1-H), 3.61 (d, 2J = 16.7 Hz, 1H, part A of AB, 10-H), 3.56 (d, 2J = 16.7 Hz, 1H, part B of AB, 10-H), 3.34–3.13 (m, 2H, 2-H, 4-H), 2.82 (dd, J = 12.4, 12.4 Hz, 1H, 4-H), 2.07 (s, 3H, 15-CH3), 1.82–1.70 (m, 2H, 6-H, 7-H), 1.69–1.47 (m, 3H, 5-H2, 7-H), 1.41 (m, 1H, 6-H). 13C NMR (126 MHz, DMSO-d6) δ 186.9 (13-Cq), 168.8 (d, 3JCP = 6.7 Hz, 9-Cq), 134.7 (15-CH), 117.5 (11-CH), 57.1 (2-CH), 48.9 (d, 1JCP = 134.7 Hz, 1-CH), 44.3 (4-CH2), 32.3 (10-CH2), 25.6 (d, 3JCP = 5.2 Hz, 7-CH2), 22.1 (5-CH2), 21.8 (6-CH2), 11.4 (15-CH3). 31P NMR (162 MHz, DMSO-d6) δ 11.79. HRESIMS m/z 366.0699 [M + H–Cl]+ (calcd for C12H21N3O4PS2).
(Piperidin-2-yl(thiophene-2-carboxamido)methyl)phos-phonic acid hydrochloride (5b)
The synthesis was performed according to general procedure B. Compound 4b-1 (31.5 mg, 0.0684 mmol) gave 5b-1 as a colorless solid (15.8 mg, 0.0464 mmol, 68%). Compound 4b-2 (40.1 mg, 0.0871 mmol) gave 5b-2 as a colorless solid (22.5 mg, 0.0660 mmol, 76%).
Compound 5b-1, diastereomer 1
1H NMR (400 MHz, DMSO-d6) δ 8.53 (m, 1H, 1-NH), 8.10 (m, 1H, 14-H), 7.80 (d, J = 4.9 Hz, 1H, 12-H), 7.16 (dd, J = 5.0, 3.7 Hz, 1H, 13-H), 4.57 (m, 1H, 1-H), 3.42 (m, 1H, 2-H), 3.24 (m, 1H, 4-H), 2.94 (m, 1H, 4-H), 2.06 (br. d, J = 13.5 Hz, 1H, 7-H), 1.78–1.38 (m, 1H, 5-H2, 6-H2), 1.57 (m, 1H, 7-H). 13C NMR (126 MHz, DMSO-d6) δ 161.7 (d, 3JCP = 5.6 Hz, 9-Cq), 139.2 (10-Cq), 131.3 (12-CH), 129.4 (14-CH), 127.9 (13-CH), 57.1 (d, 2JCP = 8.1 Hz, 2-CH), 48.6 (d, 1JCP = 145.5 Hz, 1-CH), 44.8 (4-CH2), 24.6 (7-CH2), 21.5 (5-CH2, 6-CH2). 31P NMR (162 MHz, DMSO-d6) δ 14.26. HRESIMS m/z 305.0751 [M + H–Cl]+ (305.0719 calcd for C11H18N2O4PS).
Compound 5b-2, diastereomer 2
1H NMR (400 MHz, DMSO-d6) δ 8.68 (d, J = 9.7 Hz, 1H, 1-NH), 8.14 (d, J = 3.7, 1.1 Hz, 1H, 14-H), 7.79 (dd, J = 5.0, 1.1 Hz, 1H, 12-H), 7.17 (dd, J = 5.0, 3.7 Hz, 1H, 13-H), 4.44 (ddd, 2JHP = 17.7 Hz, J = 9.7, 7.2 Hz, 1H, 1-H), 3.45 (m, 1H, 2-H), 3.28 (br. d, J = 12.2 Hz, 1H, 4-H), 2.86 (dd, J = 11.3, 11.3 Hz, 1H, 4-H), 2.07 (br. d, J = 12.1 Hz 1H, 7-H), 1.81–1.72 (m, 1H, 6-H or 5-H), 1.68 (m, 1H, 5-H or 6-H), 1.65–1.55 (m, 2H, 7-H, 5-H or 6-H), 1.47 (m, 1H, 5-H or 6-H). 13C NMR (126 MHz, DMSO-d6) δ 161.3 (d, J = 4.0 Hz, 9-Cq), 139.2 (10-CH), 131.2 (12-CH), 129.5 (14-CH), 127.9 (13-CH), 56.3 (d, 2JCP = 7.1 Hz, 2-CH), 49.1 (d, 1JCP = 147.1 Hz, 1-CH), 44.9 (4-CH2), 26.1 (d, 3JCP = 4.5 Hz, 7-CH2), 21.7 (5-CH2 or 6-CH2), 21.5 (6-CH2 or 5-CH2). 31P NMR (162 MHz, DMSO-d6) δ 14.67. HRESIMS m/z 305.0761 [M + H–Cl]+ (305.0719 calcd for C11H18N2O4PS).
(Piperidin-2-yl(thiophene-3-carboxamido)methyl)phos-phonic acid hydrochloride (5c)
The synthesis was performed according to general procedure B. Compound 4c-1 (32.6 mg, 0.0708 mmol) gave 5c-1 as a colorless solid (22.6 mg, 0.0663 mmol, 94%). Compound 4c-1 (32.6 mg, 0.0974 mmol) gave 5c-2 as a colorless solid (21.8 mg, 0.0640 mmol, 90%).
Compound 5c-1, diastereomer 1
1H NMR (400 MHz, DMSO-d6) δ 8.37 (dd, J = 2.9, 1.4 Hz, 1H, 11-H), 8.23 (d, J = 8.1 Hz, 1H, 1-NH), 7.58 (m, 1H, 14-H), 7.56 (m, 1H, 13-H), 4.48 (ddd, 2JHP = 16.7 Hz, J = 9.0, 6.5 Hz, 1H, 1-H), 3.35 (m, 1H, 2-H), 2.89 (ddd, J = 12.3, 12.3, 3.5 Hz, 1H, 4-H), 2.01 (br. d, J = 12.9 Hz, 1H, 7-H), 1.78–1.61 (m, 2H, 5-H, 6-H), 1.61–1.47 (m, 1H, 5-H or 6-H, 7-H), 1.40 (m, 1H, 5-H or 6-H). 13C NMR (126 MHz, DMSO-d6) δ 162.4 (d, 3JCP = 5.7 Hz, 9-Cq), 137.1 (10-Cq), 129.5 (11-CH), 127.4 (14-CH), 126.4 (13-CH), 57.3 (d, 2JCP = 6.2 Hz, 2-CH), 48.4 (d, 1JCP = 141.9 Hz, 1-CH), 44.5 (4-CH2), 24.8 (d, 3JCP = 5.2 Hz, 7-CH2), 21.7 (5-CH2 or 6-CH2), 21.6 (5-CH2 or 6-CH2). 31P NMR (162 MHz, DMSO-d6) δ 13.77. HRESIMS m/z 305.0737 [M + H–Cl]+ (305.0719 calcd for C11H18N2O4PS).
Compound 5c-2, diastereomer 2
1H NMR (500 MHz, DMSO-d6) δ 8.44 (m, 1H, 11-H), 7.65 (d, J = 5.0 Hz, 1H, 14-H), 7.58 (dd, J = 5.1, 2.9 Hz, 1H, 13-H), 4.47 (ddd, 2JHP = 17.7 Hz, J = 9.7, 6.9 Hz, 1H, 1-H), 3.44 (m, 1H, 2-H), 3.29 (br. d, J = 12.9 Hz, 1H, 4-H), 2.86 (dd, J = 12.6, 12.6 Hz, 1H, 4-H), 2.06 (br. d, J = 11.9 Hz, 1H, 7-H), 1.81–1.39 (m, 4H, 5-H2, 6-H2), 1.61 (m, 1H, 7-H). 13C NMR (126 MHz, DMSO-d6) δ 162.2 (d, 3JCP = 4.0 Hz, 9-Cq), 137.1 (10-Cq), 129.8 (11-CH), 127.5 (14-CH), 126.3 (13-CH), 56.4 (d, 2JCP = 6.9 Hz, 2-CH), 48.8 (d, 1JCP = 147.0 Hz, 1-CH), 44.9 (4-CH2), 26.2 (d, 3JCP = 4.8 Hz, 7-CH2), 21.7 (5-CH2 or 6-CH2), 21.5 (5-CH2 or 6-CH2). 31P NMR (162 MHz, DMSO-d6) δ 12.03. HRESIMS m/z 305.0745 [M + H–Cl]+ (305.07194 calcd for C11H18N2O4PS).
(Piperidin-2-yl(4,5,6,7-tetrahydrobenzo[b]thiophene-2-carboxamido)methyl)phosphonic acid hydrochloride (5d)
The synthesis was performed according to general procedure B. Compound 4d-1 (52.1 mg, 0.101 mmol) gave 5d-1 as a slightly yellow solid (26.8 mg, 0.0679 mmol, 67%). Compound 4d-2 (45.8 mg, 0.0890 mmol) gave 5d-2 as a colorless solid (21.4 mg, 0.0542 mmol, 61%).
Compound 5d-1, diastereomer 1
1H NMR (500 MHz, DMSO-d6) δ 8.30 (d, J = 9.7 Hz, 1H, 1-NH), 7.74 (s, 1H, 18-H), 4.53 (ddd, 2JHP = 19.4 Hz, J = 9.5, 5.3 Hz, 1H, 1-H), 3.40 (m, 1H, 2-H), 3.26 (br. d, J = 12.4 Hz, 1H, 4-H), 2.97–2.88 (m, 1H, 4-H), 2.76–2.69 (m, 2H, 13-H2), 2.62–2.53 (m, 2H, 16-H2), 2.03 (br. d, J = 12.3 Hz, 1H, 7-H), 1.84–1.63 (m, 5H, 14-H2, 15-H2, 5-H or 6-H), 1.61–1.49 (m, 2H, 7-H, 5-H or 6-H), 1.42 (m, 1H, 5-H or 6-H). 13C NMR (126 MHz, DMSO-d6) δ 161.9 (d, 3JCP = 5.6 Hz, 9-Cq), 140.9 (12-Cq), 135.8 (17-Cq), 134.7 (10-Cq), 130.1 (18-CH), 57.1 (d, 2JCP = 8.3 Hz, 2-CH), 48.5 (d, 1JCP = 145.5 Hz, 1-CH), 44.8 (4-CH2), 25.0 (16-CH2), 24.7 (13-CH2), 24.5 (d, 3JCP = 4.3 Hz, 7-CH2), 22.9 (14-CH2), 22.2 (15-CH2), 21.5 (6-CH2, 5-CH2). 31P NMR (162 MHz, DMSO-d6) δ 14.58. HRESIMS m/z 359.1207 [M + H–Cl]+ (359.1189 calcd for C15H24N2O4PS).
Compound 5d-2, diastereomer 2
1H NMR (500 MHz, DMSO-d6) δ 8.42 (d, J = 9.5 Hz, 1H, 1-NH), 7.75 (s, 1H, 18-H), 4.40 (ddd, 2JHP = 17.7 Hz, J = 9.7, 7.4 Hz, 1H, 1-H), 3.41 (m, 1H, 2-H), 3.27 (br d, J = 10.6 Hz, 1H, 4-H), 2.84 (dd, J = 12.6, 12.6 Hz, 1H, 4-H), 2.76–2.68 (m, 2H, 13-H2), 2.66–2.53 (m, 2H, 16-H2), 2.08 (m, 1H, 7-H), 1.85–1.65 (m, 5H, 14-H2, 15-H2, 5-H or 6-H), 1.63–1.53 (m, 2H, 7-H, 5-H or 6-H), 1.44 (m, 1H, 5-H or 6-H). 13C NMR (126 MHz, DMSO-d6) δ 160.9 (d, 3JCP = 3.9 Hz, 9-Cq), 140.3 (12-Cq), 135.2 (17-Cq), 134.2 (10-Cq), 129.5 (18-CH), 55.7 (d, 2JCP = 7.2 Hz, 2-CH), 48.4 (d, 1JCP = 147.1 Hz, 1-CH), 44.3 (4-CH2), 25.6 (d, 3JCP = 4.2 Hz, 7-CH2), 24.5 (16-CH2), 24.1 (13-CH2), 22.3 (14-CH2), 21.7 (15-CH2), 21.1 (5-CH2 or 6-CH2), 20.9 (5-CH2 or 6-CH2). 31P NMR (162 MHz, DMSO-d6) δ 15.36. HRESIMS m/z 359.1219 [M + H–Cl]+ (359.1189 calcd for C15H24N2O4PS).
(Piperidin-2-yl(4,5,6,7-tetrahydrobenzo[b]thiophene-3-carboxamido)methyl)phosphonic acid hydrochloride (5e)
The synthesis was performed according to the general procedure B. Compound 4e-1 (59.4 mg, 0.115 mmol) gave 5e-1 as a colorless solid (17.6 mg, 0.0446 mmol, 39%). Compound 4e-2 (68.5 mg, 0.133 mmol) gave 5e-2 as a colorless solid (11.5 mg, 0.0291 mmol, 22%).
Compound 5e-1, diastereomer 1
1H NMR (400 MHz, DMSO-d6) δ 8.07 (s, 1H, 11-H), 7.95 (dd, J = 9.7, 3.5 Hz, 1H, 1-NH), 4.54 (ddd, 2JHP = 19.2 Hz, J = 9.5, 5.5 Hz, 1H, 1-H), 3.39 (m, 1H, 2-H), 3.26 (br. d, J = 11.7 Hz, 1H, 4-H), 2.93 (ddd, J = 11.7, 11.7, 3.6 Hz, 1H, 1-H), 2.84–2.65 (m, 4H, 14-H2, 17-H2), 2.01 (br. d, J = 12.2 Hz, 1H, 7-H), 1.83–1.64 (m, 5H, 15-H2, 16-H2, 5-H or 6-H), 1.63–1.51 (m, 2H, 7-H, 5-H or 6-H), 1.44 (m, 1H, 5-H or 6-H). 13C NMR (101 MHz, DMSO-d6) δ 163.8 (9-Cq), 136.0 (10-Cq or 13-Cq or 18-Cq), 134.9 (10-Cq or 13-Cq or 18-Cq), 134.8 (10-Cq or 13-Cq or 18-Cq), 125.9 (11-CH), 57.1 (d, 2JCP = 7.3 Hz, 2-CH), 47.9 (d, 1JCP = 145.4 Hz, 1-CH), 44.7 (4-CH2), 25.4 (14-CH2 or 17-CH2), 24.7 (14-CH2 or 17-CH2), 24.5 (d, 3JCP = 3.8 Hz, 7-CH2), 22.7 (14-CH2 or 17-CH2), 22.1 (14-CH2 or 17-CH2), 21.5 (5-CH2, 6-CH2). 31P NMR (162 MHz, DMSO-d6) δ 14.73. HRESIMS m/z 359.1161 [M + H–Cl]+ (359.1189 calcd for C15H24N2O4PS).
Compound 5e-2, diastereomer 2
1H NMR (500 MHz, DMSO-d6) δ 8.22 (s, 1H, 11-H), 8.17 (d, J = 9.9 Hz, 1H, 1-NH), 4.45 (ddd, 2JHP = 17.9 Hz, J = 8.8, 8.8 Hz, 1H, 1-H), 3.45 (m, 1H, 2-H), 3.26 (br. d, J = 12.4 Hz, 1H, 4-H), 2.85 (m, 1H, 4-H), 2.81–2.67 (m, 4H, 14-H2, 17-H2), 2.10 (br. d, J = 13.9 Hz, 1H, 7-H), 1.79–1.36 (m, 9H, 5-H2, 6-H2, 7-H, 15-H2, 16-H2). 13C NMR (126 MHz, DMSO-d6) δ 163.5 (d, 3JCP = 3.4 Hz, 9-Cq), 135.9 (10-Cq or 13-Cq or 18-Cq), 135.2 (10-Cq or 13-Cq or 18-Cq), 135.0 (10-Cq or 13-Cq or 18-Cq), 126.0 (11-CH), 55.9 (d, 2JCP = 8.5 Hz, 2-CH), 48.4 (d, 1JCP = 147.7 Hz, 1-CH), 44.7 (4-CH2), 26.0 (7-CH2), 25.4 (14-CH2 or 17-CH2), 24.7 (14-CH2 or 17-CH2), 22.8 (15-CH2 or 16-CH2), 22.2 (15-CH2 or 16-CH2), 21.6 (5-CH2 or 6-CH2), 21.5 (5-CH2 or 6-CH2). 31P NMR (162 MHz, DMSO-d6) δ 14.34. HRESIMS m/z 3
591
170 [M + H–Cl]+ (359.1189 calcd for C15H24N2O4PS).
(Piperidin-2-yl(4,5,6,7-tetrahydrobenzo[c]thiophene-1-carboxamido)methyl)phosphonic acid hydrochloride (5f)
The synthesis was performed according to general procedure B. Compound 4f-1 (45.3 mg, 0.0880 mmol) gave 5f-1 as a colorless solid (28.9 mg, 0.0732 mmol, 83%). Compound 4f-2 (56.1 mg, 0.109 mmol) gave 5f-2 as a colorless solid (32.2 mg, 0.0815 mmol, 75%).
Compound 5f-1, diastereomer 1
1H NMR (500 MHz, DMSO-d6) δ 7.32 (s, 1H, 12-H), 7.02 (m, 1H, 1-NH), 4.33 (m, 1H, 1-H), 3.32 (m, 1H, 2-H), 3.28 (m, 1H, 4-H), 3.00–2.92 (m, 2H, 17-CH2), 2.88 (m, 1H, 4-H), 2.72–2.62 (m, 2H, 14-H2), 1.95 (br. d, J = 13.2 Hz, 1H, 7-H), 1.81–1.63 (m, 6H, 15-H2, 16-H2, 5-H, 6-H), 1.61–1.48 (m, 2H, 7-H, 5-H or 6-H), 1.43 (m, 1H, 5-H or 6-H). 13C NMR (126 MHz, DMSO-d6) δ 162.5 (d, 3JCP = 6.2 Hz, 9-Cq), 140.2 (13-Cq), 139.5 (18-Cq), 131.0 (10-Cq), 123.5 (12-CH), 57.4 (d, 2JCP = 5.7 Hz, 2-CH), 48.5 (d, 1JCP = 139.5 Hz, 1-CH), 44.4 (4-CH2), 26.2 (17-CH2), 26.0 (14-CH2), 24.8 (d, 3JCP = 5.5 Hz, 7-CH2), 22.7 (15-CH2 or 16-CH2), 22.4 (15-CH2 or 16-CH2), 21.7 (5-CH2 or 6-CH2), 21.6 (5-CH2 or 6-CH2). 31P NMR (162 MHz, DMSO-d6) δ 13.89. HRESIMS m/z 359.1149 [M + H–Cl]+ (359.1189 calcd for C15H24N2O4PS).
Compound 5f-2, diastereomer 2
1H NMR (500 MHz, DMSO-d6) δ 7.30 (s, 1H, 12-H), 4.33 (m, 1H, 1-H), 3.42 (m, 1H, 2-H), 3.28 (br. d, J = 12.1 Hz, 1H, 4-H), 2.98 (m, 2H, 17-H2), 2.83 (dd, J = 12.7, 12.7, 3.0 Hz, 1H, 4-H), 2.66 (m, 2H, 14-CH2), 1.99 (m, 1H, 7-H), 1.81–1.49 (m, 6H, 15-CH2, 16-CH2, 7-H, 5-H′, 6H', 5-H′′ or 6-H′′), 1.42 (m, 1H, 5-H′′ or 6-H′′). 13C NMR (126 MHz, DMSO-d6) δ 162.3 (d, 3JCP = 5.4 Hz, 9-Cq), 140.6 (13-Cq), 139.3 (18-Cq), 130.7 (10-Cq), 123.3 (12-Cq), 56.3 (d, 2JCP = 4.8 Hz, 2-CH), 49.0 (d, 1JCP = 142.7 Hz, 1-CH), 44.7 (4-CH2), 26.2 (17-CH2), 26.0 (14-CH2), 25.9 (d, 3JCP = 4.8 Hz, 7-CH2), 22.7 (15-CH2 or 16-CH2), 22.4 (15-CH2 or 16-CH2), 21.9 (5-CH2 or 6-CH2), 21.6 (5-CH2 or 6-CH2). 31P NMR (162 MHz, DMSO-d6) δ 13.37. HRESIMS m/z 359.1155 [M + H–Cl]+ (359.1189 calcd for C15H24N2O4PS).
((Benzo[b]thiophene-2-carboxamido)(piperidin-2-yl)methyl)phosphonic acid hydrochloride (5g)
The synthesis was performed according to general procedure B. Compound 4g-1 (49.7 mg, 0.0973 mmol) gave 5g-1 as a colorless solid (19.7 mg, 0.0504 mmol, 52%). Compound 4g-2 (51.5 mg, 0.101 mmol) gave 5g-2 as a colorless solid (20.8 mg, 0.0532 mmol, 53%).
Compound 5g-1, diastereomer 1
1H NMR (400 MHz, DMSO-d6) δ 8.81 (m, 1H, 1-NH), 8.47 (s, 1H, 18-H), 8.02 (d, J = 7.7 Hz, 1H, 13-H), 7.95 (d, J = 7.8 Hz, 1H, 18-H), 7.47 (ddd, J = 8.1, 8.1, 1.5 Hz, 1H, part C of ABCD, 14-H), 7.44 (ddd, J = 8.1, 8.1, 1.6 Hz, 1H, part D of ABCD, 15-H), 4.66 (ddd, 2JHP = 19.7 Hz, J = 9.9, 5.0 Hz, 1H, 1-H), 3.49 (m, 1H, 2-H), 3.28 (br. d, J = 12.7 Hz, 1H, 4-H), 2.95 (br. dd, J = 11.9, 11.9 Hz, 1H, 4-H), 2.11 (br. d, J = 13.6 Hz, 1H, 7-H), 1.76 (m, 1H, 5-H), 1.71–1.53 (m, 4H, 6-H2, 7-H), 1.45 (m, 1H, 5-H). 13C NMR (126 MHz, DMSO-d6) δ 161.7 (d, 3JCP = 5.5 Hz, 10-Cq), 139.8 (12-Cq), 138.6 (10-Cq), 138.5 (17-Cq), 125.8 (18-CH), 125.8 (14-CH), 124.8 (16-CH), 124.4 (15-CH), 122.2 (13-CH), 56.5 (d, 2JCP = 8.5 Hz, 2-H), 48.3 (d, 1JCP = 146.0 Hz, 1-H), 44.3 (4-CH2), 23.9 (7-CH2), 21.0 (5-CH2 or 6-CH2), 21.0 (5-CH2 or 6-CH2). 31P NMR (162 MHz, DMSO-d6) δ 14.19. HRESIMS m/z 355.0803 [M + H–Cl]+ (355.0876 calcd for C15H20N2O4PS).
Compound 5g-2, diastereomer 2
1H NMR (400 MHz, DMSO-d6) δ 8.93 (d, J = 9.8 Hz, 1H, 1-NH), 8.42 (s, 1H, 18-CH), 8.03 (dd, J = 6.7, 1.7 Hz, 1H, 13-H), 7.96 (dd, J = 7.6, 1.7 Hz, 1H, 6-H), 7.48 (ddd, J = 5.8, 5.8, 1.7 Hz, 1H, part C of ABCD, 14-H), 7.45 (ddd, J = 5.8, 5.8, 1.7 Hz, 1H, part D of ABCD, 15-H), 4.48 (ddd, 3JCP = 17.8 Hz, J = 9.6, 8.0 Hz, 1H, 1-H), 3.48 (m, 1H, 2-H), 3.31 (m, 1H, 4-H), 2.87 (br. dd, J = 10.1, 10.1 Hz, 1H, 4-H), 2.23–2.13 (br. d, J = 12.0 Hz, 1H, 7-H), 1.86–1.55 (m, 4H, 7-H, 5′-H, 6′-H, 5′′-H or 6′′-H), 1.48 (m, 1H, 5′′-H or 6′′-H). 13C NMR (126 MHz, DMSO-d6) δ 161.8 (d,3JCP = 3.8 Hz, 9-Cq), 140.3 (12-Cq), 139.2 (10-Cq), 139.1 (17-Cq), 126.3 (14-H), 126.2 (18-H), 125.2 (16-H), 125.0 (15-H), 122.8 (13-H), 56.3 (d, 2JCP = 7.7 Hz, 2-CH), 49.4 (d, 1JCP = 147.4 Hz, 1-CH), 44.8 (4-CH2), 26.2 (d, 3JCP = 3.5 Hz, 7-CH2), 21.7 (5-CH2 or 6-CH2), 21.5 (5-CH2 or 6-CH2). 31P NMR (162 MHz, DMSO-d6) δ 14.41. HRESIMS m/z 355.0806 [M + H–Cl]+ (355.0876 calcd for C15H20N2O4PS).
((Benzo[b]thiophene-3-carboxamido)(piperidin-2-yl)met-hyl)phosphonic acid hydrochloride (5h)
The synthesis was performed according to general procedure B. Compound 4h-1 (49.4 mg, 0.0973 mmol) gave 5h-1 as a colorless solid (25.8 mg, 0.0660 mmol, 68%). Compound 4h-2 (39.3 mg, 0.0770 mmol) gave 5h-2 as a colorless solid (15.2 mg, 0.0389 mmol, 51%).
Compound 5h-1, diastereomer 1
1H NMR (400 MHz, DMSO-d6) δ 8.70 (s, 1H, 11-H), 8.48 (d, J = 7.5 Hz, 1H, 17-H), 8.04 (dd, J = 7.5, 1.6 Hz, 1H, 13-H), 7.45 (ddd, J = 7.5, 7.5, 1.3 Hz, 1H, part C of ABCD, 16-H), 7.42 (ddd, J = 7.5, 7.5, 1.6 Hz, 1H, part D of ABCD, 15-H), 4.71 (ddd, 2JHP = 19.5 Hz, J = 9.5 Hz, 4.8 Hz, 1H, 1-H), 3.46 (m, 1H, 2-H), 3.29 (br. d, J = 12.5 Hz, 1H, 4-H), 2.96 (ddd, J = 12.5, 12.5, 2.08 Hz, 1H, 4-H), 2.09 (br. d, J = 12.1 Hz, 1H), 1.81–1.38 (m, 5H, 5-H2, 6-H2, 7-H). 13C NMR (126 MHz, DMSO-d6) δ 163.5 (d, 3JCP = 5.4 Hz, 9-Cq), 139.2 (13-Cq), 137.3 (18-Cq), 132.4 (11-CH), 130.0 (10-Cq), 124.9 (15-CH), 124.8 (16-CH), 124.6 (17-CH), 122.8 (14-CH), 57.2 (d, 2JCP = 8.7 Hz, 2-CH), 48.2 (d, 1JCP = 146.3 Hz, 1-CH), 44.8 (4-CH2), 24.5 (d, 3JCP = 3.8 Hz, 7-CH2), 21.6 (5-CH2, 6-CH2). 31P NMR (162 MHz, DMSO-d6) δ 14.68. HRESIMS m/z 355.0807 [M + H–Cl]+ (355.0876 calcd for C15H19N2O4PS).
Compound 5h-2, diastereomer 2
1H NMR (500 MHz, DMSO-d6) δ 8.75 (s, 1H, 11-H), 8.54 (d, J = 9.8 Hz, 1H, 1-NH), 8.47 (d, J = 7.2 Hz, 1H, 17-H), 8.06 (d, J = 7.0 Hz, 1H, 14-H), 7.46 (ddd, J = 7.3, 7.3, 1.2 Hz, 1H, part C of ABCD, 16-H), 7.45 (ddd, J = 7.3, 7.3, 1.2 Hz, 1H, part D of ABCD, 15-H), 4.56 (ddd, 2JHP = 18.0 Hz, J = 9.1 Hz, 1H), 3.47 (m, 1H, 2-H), 3.30 (br. d, J = 12.0 Hz, 1H, 4-H), 2.87 (ddd, J = 11.9, 11.9, 11.9 Hz, 1H, 4-H), 2.23 (d, J = 13.3 Hz, 1H, 7-H), 1.85–1.42 (m, 5H, 5-H2, 6-H2, 7-H). 13C NMR (126 MHz, DMSO-d6) δ 163.0 (d, 3JCP = 3.3 Hz), 139.3 (13-Cq), 137.3 (18-Cq), 132.2 (11-CH), 130.2 (10-Cq), 124.9 (15-CH or 15-CH), 124.8 (15-CH or 15-CH), 124.6 (17-CH), 122.7 (14-CH), 56.2 (d, 2JCP = 8.8 Hz, 2-H), 48.7 (d, 1JCP = 148.0 Hz, 1-H), 44.7 (4-H2), 26.1 (7-CH2), 21.7 (5-CH2 or 6-CH2), 21.5 (5-CH2 or 6-CH2). 31P NMR (162 MHz, DMSO-d6) δ 14.94. HRESIMS m/z 355.0849 [M + H–Cl]+ (355.0876 calcd for C15H20N2O4PS).
(Piperidin-2-yl(2-(thiophen-2-yl)acetamido)methyl)phos-phonic acid hydrochloride (5i)
The synthesis was performed according to general procedure B. Compound 4i-1 (60.8 mg, 0.128 mmol) gave 5i-1 as a colorless solid (21.3 mg, 0.0600 mmol, 47%). Compound 4i-2 (61.1 mg, 0.129 mmol) gave 5i-2 as a colorless solid (31.0 mg, 0.0874 mmol, 68%).
Compound 5i-1, diastereomer 1
1H NMR (400 MHz, DMSO-d6) δ 8.36 (d, J = 9.8 Hz, 1H, 1-NH), 7.36 (dd, J = 4.9, 1.6 Hz, 1H, 13-H), 7.04–6.89 (m, 2H, 14-H, 15-H), 4.43 (ddd, 3JHP = 20.1 Hz, J = 9.7, 4.5 Hz, 1H, 1-H), 3.90 (d, 2J = 16.1 Hz, 1H, part A of B, 10-H), 3.79 (d, 2J = 16.1 Hz, 1H, part B of AB, 10-H), 3.37 (m, 1H, 2-H), 3.24 (br. d, J = 12.4 Hz, 1H, 4-H), 2.93 (dd, J = 11.5, 11.5 Hz, 1H, 4-H), 2.01 (br. d, J = 12.6 Hz, 1H, 7-H), 1.81–1.37 (m, 5H, 5-H2, 6-H2, 7-H). 13C NMR (126 MHz, DMSO-d6) δ 170.1 (d, 3JCP = 5.6 Hz, 9-Cq), 137.2 (11-Cq), 126.4 (14-CH), 126.2 (15-CH), 124.9 (13-CH), 56.9 (d, 2JCP = 10.0 Hz, 2-CH), 48.1 (d, 1JCP = 148.5 Hz, 1-CH), 44.8 (4-CH2), 36.00 (10-CH2), 24.0 (d, 3JCP = 3.4 Hz, 7-CH2), 21.5 (5-CH2, 6-CH2). 31P NMR (162 MHz, DMSO-d6) δ 14.76. HRESIMS m/z 319.0848 [M + H–Cl]+ (319.0876 calcd for C12H20N2O4PS).
Compound 5i-2, diastereomer 2
1H NMR (400 MHz, DMSO-d6) δ 8.49 (m, 1H, 1-NH), 7.35 (dd, J = 4.6, 1.7 Hz, 1H, 13-H), 6.97–6.92 (m, 2H, 14-H, 15-H), 4.22 (ddd, 2JHP = 17.8 Hz, J = 9.6, 6.4 Hz, 1H, 1-H), 3.83 (s, 2H, 10-CH2), 3.35–3.21 (m, 2H, 2-H, 4-H), 2.87 (br. dd, J = 11.4, 11.4 Hz, 1H, 4-H), 2.00 (br. d, J = 13.2 Hz, 1H, 7-H), 1.79–1.39 (m, 5H, 5-H2, 6-H2, 7-H). 13C NMR (126 MHz, DMSO-d6) δ 169.5 (d, 3JCP = 5.1 Hz, 9-Cq), 137.2 (11-Cq), 126.4 (14-CH), 126.3 (15-CH), 124.9 (13-CH), 56.8 (d, 2JCP = 5.7 Hz, 2-CH), 48.4 (d, 1JCP = 146.4 Hz, 1-CH), 44.9 (4-CH2), 36.1 (10-CH2), 25.8 (d, 3JCP = 4.9 Hz, 7-CH2), 21.8 (5-CH2 or 6-CH2), 21.5 (5-CH2 or 6-CH2). 31P NMR (162 MHz, DMSO-d6) δ 15.26. HRESIMS m/z 319.0833 [M + H–Cl]+ (319.0876 calcd for C12H20N2O4PS).
(Piperidin-2-yl(2-(thiophen-3-yl)acetamido)methyl)phos-phonic acid hydrochloride (5j)
The synthesis was performed according to general procedure B. Compound 4j-1 (48.0 mg, 0.101 mmol) gave 5j-1 as a colorless solid (24.4 mg, 0.0688 mmol, 68%). Compound 4j-2 (58.2 mg, 0.122 mmol) gave 5j-2 as a slightly yellow solid (26.8 mg, 0.0755, 62%).
Compound 5j-1, diastereomer 1
1H NMR (400 MHz, DMSO-d6) δ 8.30 (dd, J = 9.9, 3.2 Hz, 1H, 1-NH), 7.44 (dd, J = 4.9, 2.9 Hz, 1H, 14-H), 7.27 (dd, J = 2.9, 1.2 Hz, 1H, 12-H), 7.07 (dd, J = 4.9, 1.2 Hz, 1H, 15-H), 4.46 (ddd, 3JHP = 20.4 Hz, J = 9.9, 4.3 Hz, 1H, 1-H), 3.69 (d, 2J = 15.2 Hz, 1H, part A of AB, 10-H), 3.56 (d, 2J = 15.2 Hz, 1H, part B of AB, 10-H), 3.39 (m, 1H, 2-H), 3.24 (br. d, J = 12.3 Hz, 1H, 4-H), 2.93 (m, 1H, 4-H), 2.00 (br. d, J = 13.5 Hz, 1H, 7-H), 1.81–1.35 (m, 4H, 5-H2, 6-H2, 7-H). 13C NMR (126 MHz, DMSO-d6) δ 170.8 (d, 3JCP = 5.5 Hz, 9-Cq), 136.0 (11-Cq), 129.0 (15-CH), 125.4 (14-CH), 122.3 (12-CH), 56.9 (d, 2JCP = 10.3 Hz, 2-CH), 48.0 (d, 1JCP = 148.9 Hz, 1-CH), 44.8 (4-CH2), 36.7 (10-CH2), 24.0 (d, 3JCP = 3.3 Hz, 7-CH2), 21.5 (5-CH2 or 6-CH2), 21.4 (5-CH2 or 6-CH2). 31P NMR (162 MHz, DMSO-d6) δ 15.07. 15N NMR (51 MHz, DMSO-d6) δ −272.06 (1-NH), −331.80 (2-NH2). HRESIMS m/z 319.0801 [M + H–Cl]+ (319.0876 calcd for C12H20N2O4PS).
Compound 5j-2, diastereomer 2
1H NMR (400 MHz, DMSO-d6) δ 8.40 (d, J = 7.1 Hz, 1H, 1-NH), 7.44 (dd, J = 4.9, 3.0 Hz, 1H, 14-H), 7.30 (d, J = 3.0 Hz, 1H, 12-H), 7.08 (d, J = 4.9 Hz, 1H, 15-H), 4.27 (ddd, 2JHP = 17.3 Hz, J = 9.7, 7.1 Hz, 1H), 3.60 (s, 2H, 10-H2), 3.38–3.22 (m, 2H, 2-H, 4-H), 2.89 (dd, J = 12.1, 12.1 Hz, 1H, 4-H), 2.06 (br. d, J = 13.8 Hz, 1H, 7-H), 1.78–1.36 (m, 5H, 5-H2, 6-H2, 7-H). 13C NMR (126 MHz, DMSO-d6) δ 169.5 (d, J = 4.4 Hz, 9-Cq), 135.3 (11-Cq), 128.5 (15-CH), 124.8 (14-CH), 121.8 (12-CH), 55.9 (d, 2JCP = 6.8 Hz, 2-CH), 47.7 (d, 1JCP = 148.3 Hz, 1-CH), 44.2 (4-CH2), 36.3 (10-CH2), 25.3 (d, 3JCP = 4.1 Hz, 7-CH2), 21.2 (5-CH2 or 6-CH2), 20.9 (5-CH2 or 6-CH2). 31P NMR (162 MHz, DMSO-d6) δ 15.26. HRESIMS m/z 319.0885 [M + H–Cl]+ (319.0876 calcd for C12H20N2O4PS).
((2-(Benzo[b]thiophen-2-yl)acetamido)(piperidin-2-yl)methyl)phosphonic acid hydrochloride (5k)
The synthesis was performed according to general procedure B. Compound 4k-1 (52.2 mg, 0.100 mmol) gave 5k-1 as a slightly orange solid (17.5 mg, 0.0432 mmol, 43%). Compound 4k-2 (53.7 mg, 0.102 mmol) gave 5k-2 as a colorless solid (13.4 mg, 0.0331 mmol, 32%).
Compound 5k-1, diastereomer 1
1H NMR (400 MHz, DMSO-d6) δ 8.45 (d, J = 9.3 Hz, 1H, 1-NH), 7.87 (d, J = 7.7 Hz, 1H, 14-H), 7.80–7.69 (dd, J = 7.8, 1.0 Hz, 1H, 17-H), 7.32 (ddd, J = 7.4, 7.4, 1.3 Hz, 1H, part C of ABCD, 16-H), 7.28 (ddd, J = 7.4, 7.4, 1.3 Hz, 1H, part D of ABCD, 15-H), 7.25 (s, 1H, 19-H), 4.39 (ddd, 2JHP = 19.5 Hz, J = 9.7, 4.9 Hz, 1H, 1-H), 4.01 (d, 2J = 16.0 Hz, 1H, part A of AB, 10-H), 3.90 (d, 2J = 16.0 Hz, 1H, part B of AB,10-H), 3.35 (m, 1H, 2-H), 3.25 (br. d, J = 12.4 Hz, 1H, 4-H), 2.92 (br. dd, J = 11.7, 11.7 Hz, 1H, 4-H), 2.02 (br. d, J = 13.1 Hz, 1H, 7-H), 1.80–1.35 (m, 5H, 5-H2, 6-H2, 7-H). 13C NMR (126 MHz, DMSO-d6) δ 169.6 (d, 3JCP = 5.8 Hz, 9-Cq), 139.4 (13-Cq), 139.3 (18-Cq), 138.9 (11-Cq), 124.1 (16-CH), 123.7 (15-CH), 122.9 (17-CH), 122.7 (19-CH), 122.1 (14-CH), 57.0 (d, 2JCP = 8.7 Hz, 2-H), 48.2 (d, 1JCP = 146.6 Hz, 1-CH), 44.7 (4-CH2), 36.9 (10-CH2), 24.2 (d, 3JCP = 3.8 Hz, 7-CH2), 21.6 (5-CH2 or 6-CH2), 21.5 (5-CH2 or 6-CH2). 31P NMR (162 MHz, DMSO-d6) δ 14.27. HRESIMS m/z 369.1008 [M + H–Cl]+ (369.1032 calcd for C16H22N2O4PS).
Compound 5k-2, diastereomer 2
1H NMR (500 MHz, DMSO-d6) δ 8.57 (d, J = 10.0 Hz, 1H, 1-NH), 7.88 (d, J = 7.8 Hz, 1H, 14-H), 7.75 (d, J = 7.7 Hz, 1H, 17-H), 7.33 (dd, J = 7.4, 7.4 Hz, 1H, 16-H), 7.29 (dd, J = 7.4, 7.4 Hz, 1H, 15-H), 7.27 (s, 1H, 19-H), 4.29 (ddd, 2JHP = 17.3 Hz, J = 9.6, 7.4 Hz, 1H, 1-H), 3.97 (d, 2J = 16.2 Hz, 1H, part A of AB, 10-H), 3.93 (d, 2J = 16.2 Hz, 1H, part A of AB, 10-H), 3.34–3.25 (m, 2H, 2-H, 4-H), 2.88 (br. dd, J = 12.2, 11.5 Hz, 1H, 4-H), 2.11 (br. d, J = 13.9 Hz, 1H, 7-H), 1.85–1.40 (m, 5H, 5-H2, 6-H2, 7-H). 13C NMR (126 MHz, DMSO-d6) δ 168.9 (d, 3JCP = 4.2 Hz, 9-Cq), 139.5 (13-Cq or 18-Cq), 139.4 (18-Cq or 13-Cq), 138.8 (11-Cq), 124.2 (16-CH), 123.8 (15-CH), 123.0 (17-CH), 122.9 (19-CH), 122.2 (14-CH), 56.2 (d, 2JCP = 7.6 Hz, 2-CH), 48.5 (d, 1JCP = 148.5 Hz, 1-CH), 44.7 (4-CH2), 37.2 (10-CH2), 25.9 (7-CH2), 21.7 (5-CH2 or 6-CH2), 21.5 (5-CH2 or 6-CH2). 31P NMR (162 MHz, DMSO-d6) δ 13.32. HRESIMS m/z 369.1052 [M + H–Cl]+ (369.1032 calcd for C16H22N2O4PS).
((2-(Benzo[b]thiophen-3-yl)acetamido)(piperidin-2-yl)methyl)phosphonic acid hydrochloride (5l)
The synthesis was performed according to general procedure B. Compound 4l-1 (53.0 mg, 0.101 mmol) gave 5l-1 as a colorless solid (7.9 mg, 0.0195 mmol, 19%). Compound 4l-2 (50.7 mg, 0.0966 mmol) gave 5l-2 as a colorless solid (15.9 mg, 0.0393 mmol, 41%).
Compound 5l-1, diastereomer 1
1H NMR (400 MHz, DMSO-d6) δ 8.49 (d, J = 9.5 Hz, 1H, 1-NH), 7.97 (d, J = 6.7 Hz, 1H, 15-H), 7.88 (d, J = 6.9 Hz, 1H, 18-H), 7.54 (s, 1H, 12-H), 7.39 (m, 1H, 17-H), 7.36 (m, 1H, 16-H), 4.46 (m, 1H, 1-H), 3.93 (d, 2J = 15.6 Hz, 1H, part A of AB, 10-H), 3.83 (d, 2J = 15.6 Hz, 1H, part B of AB, 10-H), 3.39 (m, 1H, 2-H), 3.25 (br. d, J = 12.1 Hz, 1H, 4-H), 2.93 (br. dd, J = 12.6, 12.6 Hz, 1H, 4-H), 2.03 (br. d, J = 13.0 Hz, 1H, 7-H), 1.86–1.35 (m, 5H, 5-H2, 6-H2, 7-H). 13C NMR (126 MHz, DMSO-d6) δ 170.4 (d, 3JCP = 5.5 Hz, 9-Cq), 139.3 (14-Cq), 138.9 (19-Cq), 130.6 (11-Cq), 124.3 (16-CH), 124.1 (12-CH), 124.0 (17-CH), 122.7 (15-CH), 122.4 (18-CH), 56.9 (d, 2JCP = 9.9 Hz, 2-CH), 48.1 (d, 1JCP = 148.4 Hz, 1-CH), 44.8 (4-CH2), 35.1 (10-CH2), 24.1 (d, 3JCP = 2.5 Hz, 7-CH2), 21.5 (5-CH2 or 6-CH2), 21.5 (5-CH2 or 6-CH2). 31P NMR (162 MHz, DMSO-d6) δ 14.97. HRESIMS m/z 3
690
859 [M + H–Cl]+ (369.1032 calcd for C16H22N2O4PS).
Compound 5l-2, diastereomer 2
1H NMR (400 MHz, DMSO-d6) δ 8.56 (d, J = 9.3 Hz, 1H, 1-NH), 7.96 (m, 1H, 15-H), 7.90 (m, 1H, 18-H), 7.57 (s, 1H, 12-H), 7.38 (m, 1H, 17-H), 7.36 (m, 1H, 16-H), 4.28 (ddd, 3JHP = 17.1 Hz, J = 9.7, 6.7 Hz, 1H, 1-H), 3.88 (d, 2J = 16.3 Hz, 1H, part A of AB, 10-H), 3.86 (dd, J = 16.3 Hz, 1H, part B of AB, 10-H), 3.36–3.22 (m, 2H, 2-H, 4-H), 2.88 (dd, J = 11.8, 11.8 Hz, 1H, 4-H), 2.04 (d, J = 13.8 Hz, 1H, 7-H), 1.82–1.38 (m, 5H, 5-H2, 6-H2, 7-H). 13C NMR (126 MHz, DMSO-d6) δ 169.8 (d, 3JCP = 4.7 Hz, 9-Cq), 139.3 (14-Cq), 138.9 (19-Cq), 130.5 (11-Cq), 124.3 (16-CH), 124.3 (12-CH), 123.9 (17-CH), 122.7 (15-CH), 122.4 (18-CH), 56.7 (d, 2JCP = 6.4 Hz, 2-CH), 48.4 (d, 1JCP = 147.5 Hz, 1-CH), 44.9 (4-CH2), 35.2 (10-CH2), 25.8 (d, 3JCP = 4.3 Hz, 7-CH2), 21.8 (5-CH2 or 6-CH2), 21.5 (5-CH2 or 6-CH2). 31P NMR (162 MHz, DMSO-d6) δ 15.13. HRESIMS m/z 369.0975 [M + H–Cl]+ (369.10324 calcd for C16H22N2O4PS).
1H,15N HSQC titration experiments
For the titrations, 15N-labelled NDM-1 and 15N-labelled VIM-2 (250 μM), and titrated ligands (0.5 equiv. in 1 μL) were prepared in the same buffer as the protein (20 mM KPO4, 0.1 mM ZnCl2, pH 7.0 with 10% D2O and 2.5% DMSO-d6). 1H,15N HSQC spectra were acquired with 128 × 1024 complex points (F1 × F2) and spectral width of 9090 × 2740 Hz on 15N-labeled NDM-1/VIM-2 and with every titration step up to 1
:
16 ratio between protein
:
ligand (0, 0.5, 1, 2, 4, 8, and 16 equiv. of ligand). All experiments were recorded on a Bruker 600 MHz spectrometer at 310 K equipped with a 5 mm TCI cryogenic probe. The NMR data were processed on the MestReNova software with the Mnova Binding plugin. The weighted average chemical shift perturbations (CSP) for the backbone amides were calculated from the observed chemical shift differences in the proton and nitrogen dimensions using the equation (chemical shift scaling factors: FH = 1, FN = 0.156) CSP = Δδ1H,15N =
.
Molecular docking
Schrödinger software (Schrödinger, LLC, New York, NY, 2019) was used and unless otherwise stated, default settings were used in the computations. The crystal structures of VIM-2 (pdb 6O5T) and NDM-1 (pdb 6NY7) were prepared using the Protein Preparation Wizard;60 involving Prime52 to fill in missing side chains and loops, Epik61,62 to generate heteroatom states (pH 7.0 ± 2.0), PropKa (pH 7) to optimize the hydrogen bond network (sampling of water orientations when appropriate), and the OPLS3e force field63 in the restrained minimization (hydrogens only). The Receptor Grid Generation module in Glide64–66 was used to define the active site by creating a rectangular box centered on the native ligand (crystal structure) and extending in all directions to encompass ligands of similar size (i.e. 16.5 Å) or ligands <22 Å. Grids with no water molecules in the active site were generated and validated. The ligands were converted to 3D all atom structures using LigPrep. Epik was used to generate possible ionization states at target pH 7 ± 2, including metal binding states. Tautomers were selected to be generated. The molecular docking was performed using Glide. The inhibitors were docked using the flexible docking mode, with sampling of nitrogen inversions and ring conformations, and with extra precision (XP) rescoring. Biased sampling of torsions was selected for amides by penalizing non-planar conformations. Epik state penalties was selected to be added to the docking score. A maximum of 40 poses were selected to be generated for each ligand. Post-docking minimization was used, and per-residue interaction scores were written for residues within 10 Å from the grid center. Prime MM-GBSA rescoring of docking poses (VSGB 2.0 solvation model and OPLS3e force field) were performed providing poses ranked based on free energy of binding (ΔGbind in kcal mol−1). For these calculations, flexibility was allowed for residues within 10 Å from the ligands, and minimization was selected as sampling method. Structural interaction fingerprints (SIFts) in Canvas was used for representation and analysis of the docking poses. Side chain, zinc ion, and hydrogen bonding interactions were used to generate the SIFts. The similarity between the SIFts were evaluated using the Tanimoto similarity metric, and clustering was performed using the Flexible Beta linkage method. Epik (Schrödinger, LLC, New York, NY, 2020) was used for empirical predictions of aqueous pKa values.
Dose rate inhibition studies for IC50 determination
The half-maximal inhibitory concentration (IC50) against the NDM-1, VIM-2 and GIM-1 enzymes were determined by using twelve different concentration of inhibitor compounds ranging from 0 μM to 800 μM. A 100 μl solution with buffer (50 mM HEPES (pH 7.2), 100 μM ZnCl2, 2.5% DMSO), purified enzyme (0.1 nM VIM-2, 10 nM NDM-1 or 1 nM GIM-1) and 800–0 μM inhibitor was incubated in a 96 well plate at 25 °C for 5 min. In addition, the enzyme buffer contained 400 μg ml−1 bovine serum albumin (BSA) to prevent protein unfolding and loss of activity due to low concentrations.67,68 100 μM of the reporter substrate nitrocefin (VIM-2, GIM-1) or imipenem (NDM-1) was added to the enzyme–inhibitor solution and the increase in absorbance at 482 nm (nitrocefin) or 300 nm (imipenem) was recorded on a Spectramax M4 spectrophotometer (Molecular Devices). Each data point was performed in triplicates and the initial velocity for each inhibitor concentration was analyzed by a log [inhibitor] vs. response curve fitting to calculate IC50 in GraphPad Prism 9.2 software.
Cytotoxicity assay
MCF-7 and HepG2 cells were cultured in Dulbecco's modified Eagle's medium supplemented with 10% fetal bovine serum and 1% of penicillin–streptomycin antibiotics (Sigma-Aldrich). Cells were cultured in a permanent exponential growth at 37 °C, 5% CO2.69 Briefly, cells were seeded in 96-well plates (TPP® tissue culture plates 92097) at a density that allowed continued exponential growth. Stock solutions of the compounds dissolved in DMSO were added to a final DMSO concentration of 0.3% v/v in the cell culture medium. Two-fold serial dilutions were made to estimate EC50. After 24 h of incubation in the presence of the compounds, cell viability was determined using PrestoBlue cell viability reagent (ThermoFisher). POLARstar Omega plate reader (BMG Lab Tech) was used to measure resorufin fluorescence (544 nm/590 nm). Cells with no treatment, cells treated only with DMSO 0.3% v/v, and cells treated with SDS 1% w/v were used as controls. Three independent replicate experiments were used to calculate the EC50. The statistical analysis and plots were performed using R version 3.6.3 (R core team).70 The viability of the cells was plotted using ggplot 2,71 as the fluorescence of treated cells/fluorescence of the lowest concentration of the compound.
Protein expression
The expression and purification of uniformly [15N]-labelled NDM-1 and VIM-2 were performed according to the procedure reported previously.48,49 For enzymatic assays, NDM-1, VIM-2 and GIM-1 were expressed and purified as published earlier.41
Molecular cloning
The full-length blaVIM-2 gene was amplified using primers ATA CAT ATG TTC AAA CTT TTG AGT AAG TTA TTG and TCA CTC GAG CTA CTC AAC GAC TGA GCG ATT TG and subcloned into a pET30a vector between the NdeI and XhoI restriction sites resulting in plasmid pY339.
Preparation of outer membrane vesicles (OMVs) and in situ activity testing
OMVs carrying active VIM-2 inside their lumen were prepared as described.72 Briefly, E. coli strain BL21(DE3)ΔompA carrying plasmid pY339 was grown at 37 °C in LB media supplemented with 10 μM ZnCl2 and 50 μg mL−1 Kanamycin. When cultures reached an optical density of 0.4 protein expression was induced by addition of 50 μM IPTG and growth was maintained for 2 h. Cells were removed by centrifugation at 10
000 × g for 10 min and cleared supernatants were filtered using 0.45 μm bottle top filters. OMVs were collected by centrifugation at 38
400 × g for 2 h, washed in OMV buffer (DPBSS, Sigma-Aldrich D8662, supplemented with 10 μM ZnCl2), filtered using 0.45 μm syringe filters, recollected by centrifugation at 74
500 × g for 30 min and resuspended in OMV buffer.
Activity of the encapsulated VIM-2 in presence of the compounds was determined in clear flat bottom half area 96 well microplates (Corning 3696) in a total volume of 50 μL containing 400 μg ml−1 OMVs, 250 μg ml−1 CENTA β-lactamase substrate (Sigma-Aldrich 219475), and 2.5% DMSO in OMV buffer using a Fluostar Omega plate reader (BMG Lab Tech) by measuring the increase in absorbance at 405 nm 10 minutes after addition of the substrate.
Author contributions
KP and FD contributed equally. FD and KP synthesized the molecules, KP performed the NMR titrations and computational docking, SS and HKSL determined IC50s, GPDSM and PS performed the cytotoxicity assay, JT determined membrane permeability, AAR expressed and purified proteins, and AV determined X-ray structures. HA, KP and ME designed the structures. The synthesis was developed by HA, KP and FD. PS, HKSL, KP, HA and ME analysed and discussed the data. The manuscript was written with the contributions of all authors, and all authors have approved the final version of the manuscript.
Conflicts of interest
There are no conflicts to declare.
Acknowledgements
We acknowledge the Swedish Research Council for financial support (2013-8804), Sven and Lilly Lawski Foundation, the Center for Antibiotics Resistance Research (CARe) at University of Gothenburg, Svenska Sällskapet för Medicinsk Forskning (PD20-0191), the Academy of Finland (grant no. 314343 and 335600) and the Åke Wiberg Foundation (M21-0225). The Lund Protein Production Platform (LP3) at Lund University, managed by Wolfgang Knecht, is acknowledged for NDM-1 and VIM-2 protein expression. This project made use of the NMR Uppsala infrastructure, which is funded by the Department of Chemistry – BMC and the Disciplinary Domain of Medicine and Pharmacy and had high-resolution mass spectra obtained by Stenhagen Analyslab AB. Part of the computations studies was performed on resources provided by Swedish National Infrastructure for Computing (SNIC) through National Supercomputer Center (NSC) under Project SNIC 2020/5-435. Crystallographic infrastructure in the Department of Chemistry at the University of Jyväskylä were successfully utilized.
Notes and references
- Antimicrobial Resistance Collaborators, Lancet, 2022, 399, 629–655 CrossRef PubMed.
-
J. O'Neil, Review on Antimicrobial Resistance, Antimicrobial Resistance: Tackling a Crisis for the Health and Wealth of Nations, 2014 Search PubMed.
- W. C. Reygaert, AIMS Microbiol., 2018, 4, 482–501 CAS.
- M. F. Mojica, M. A. Rossi, A. J. Vila and R. A. Bonomo, Lancet Infect. Dis., 2022, 22, e28–e34 CrossRef CAS PubMed.
- J. Brem, R. Cain, S. Cahill, M. A. McDonough, I. J. Clifton, J.-C. Jimenez-Castellanos, M. B. Avison, J. Spencer, C. W. G. Fishwick and C. J. Schofield, Nat. Commun., 2016, 7, 12406 CrossRef CAS PubMed.
- M. McKenna, Nature, 2013, 499, 394–396 CrossRef CAS PubMed.
- G. Meletis, Ther. Adv. Infect. Dis., 2016, 3, 15–21 CAS.
- K. Bush and P. A. Bradford, Nat. Rev. Microbiol., 2019, 17, 295–306 CrossRef CAS PubMed.
- D. Yong, M. A. Toleman, C. G. Giske, H. S. Cho, K. Sundman, K. Lee and T. R. Walsh, Antimicrob. Agents Chemother., 2009, 53, 5046–5054 CrossRef CAS PubMed.
- N. Tijet, G. Macmullin, O. Lastovetska, C. Vermeiren, P. Wenzel, T. Stacey-Works, D. E. Low, S. N. Patel and R. G. Melano, Emerging Infect. Dis., 2013, 19, 1156–1158 CrossRef PubMed.
- V. Bavetsias, A. Faisal, S. Crumpler, N. Brown, M. Kosmopoulou, A. Joshi, B. Atrash, Y. Perez-Fuertes, J. A. Schmitt, K. J. Boxall, R. Burke, C. Sun, S. Avery, K. Bush, A. Henley, F. I. Raynaud, P. Workman, R. Bayliss, S. Linardopoulos and J. Blagg, J. Med. Chem., 2013, 56, 9122–9135 CrossRef CAS PubMed.
- J. M. Munita and C. A. Arias, Microbiol. Spectrum, 2016, 4 Search PubMed.
- T. Naas, S. Oueslati, R. A. Bonnin, M. L. Dabos, A. Zavala, L. Dortet, P. Retailleau and B. I. Iorga, J. Enzyme Inhib. Med. Chem., 2017, 32, 917–919 CrossRef CAS PubMed.
- J. Schauer, S. G. Gatermann, J. Eisfeld, J. B. Hans, S. Ziesing, D. Schlüter and N. Pfennigwerth, J. Antimicrob. Chemother., 2022, 77, 1247–1253 CrossRef CAS PubMed.
- C. Chen, P. Oelschlaeger, D. Wang, H. Xu, Q. Wang, C. Wang, A. Zhao and K. W. Yang, J. Med. Chem., 2022, 65, 5954–5974 CrossRef CAS PubMed.
-
U.S. Food and Drug Administration, C. f. D. E. a. R. Zosyn NDA 50-684/S-045 and NDA 50-750/S-012 approval letter, September 9, 2005.
-
U.S. Food and Drug Administration, C. f. D. E. a. R. Augmentin NDA 50-575/S-037, NDA 50-597/S-044, NDA 50-725/S-025 and NDA 50-726/S-019 approval letter 1996 May 5.
- B. Liu, R. E. L. Trout, G.-H. Chu, D. McGarry, R. W. Jackson, J. C. Hamrick, D. M. Daigle, S. M. Cusick, C. Pozzi, F. De Luca, M. Benvenuti, S. Mangani, J.-D. Docquier, W. J. Weiss, D. C. Pevear, L. Xerri and C. J. Burns, J. Med. Chem., 2020, 63, 2789–2801 CrossRef CAS PubMed.
- A. M. King, S. A. Reid-Yu, W. Wang, D. T. King, G. De Pascale, N. C. Strynadka, T. R. Walsh, B. K. Coombes and G. D. Wright, Nature, 2014, 510, 503–506 CrossRef CAS PubMed.
- C. M. Rotondo and G. D. Wright, Curr. Opin. Microbiol., 2017, 39, 96–105 CrossRef CAS PubMed.
- D. T. Davies, S. Leiris, N. Sprynski, J. Castandet, C. Lozano, J. Bousquet, M. Zalacain, S. Vasa, P. K. Dasari, R. Pattipati, N. Vempala, S. Gujjewar, S. Godi, R. Jallala, R. R. Sathyap, N. A. Darshanoju, V. R. Ravu, R. R. Juventhala, N. Pottabathini, S. Sharma, S. Pothukanuri, K. Holden, P. Warn, F. Marcoccia, M. Benvenuti, C. Pozzi, S. Mangani, J. D. Docquier, M. Lemonnier and M. Everett, ACS Infect. Dis., 2020, 6, 2419–2430 CrossRef CAS PubMed.
- G. B. Li, M. I. Abboud, J. Brem, H. Someya, C. T. Lohans, S. Y. Yang, J. Spencer, D. W. Wareham, M. A. McDonough and C. J. Schofield, Chem. Sci., 2017, 8, 928–937 RSC.
- M. Mandal, L. Xiao, W. Pan, G. Scapin, G. Li, H. Tang, S. W. Yang, J. Pan, Y. Root, R. K. de Jesus, C. Yang, W. Prosise, P. Dayananth, A. Mirza, A. G. Therien, K. Young, A. Flattery, C. Garlisi, R. Zhang, D. Chu, P. Sheth, I. Chu, J. Wu, C. Markgraf, H. Y. Kim, R. Painter, T. W. Mayhood, E. DiNunzio, D. F. Wyss, A. V. Buevich, T. Fischmann, A. Pasternak, S. Dong, J. D. Hicks, A. Villafania, L. Liang, N. Murgolo, T. Black, W. K. Hagmann, J. Tata, E. R. Parmee, A. E. Weber, J. Su and H. Tang, J. Med. Chem., 2022, 65, 16234–16251 CrossRef CAS PubMed.
- X. Gu, M. Zheng, L. Chen and H. Li, Microbiol. Res., 2022, 261, 127079 CrossRef CAS PubMed.
- S. Alfei and A. M. Schito, Pharmaceuticals, 2022, 15, 476 CrossRef CAS PubMed.
- V. Agarwal, A. Tiwari and P. Varadwaj, Curr. Med. Chem., 2023, 30, 783–808 CrossRef CAS PubMed.
- U. Hofer, Nat. Rev. Microbiol., 2022, 20, 125 Search PubMed.
- K. Bush and P. A. Bradford, Cold Spring Harbor Perspect. Med., 2016, 6, a025247 CrossRef PubMed.
- P. Linciano, L. Cendron, E. Gianquinto, F. Spyrakis and D. Tondi, ACS Infect. Dis., 2019, 5, 9–34 CrossRef CAS PubMed.
- J. A. Day and S. M. Cohen, J. Med. Chem., 2013, 56, 7997–8007 CrossRef CAS PubMed.
- L. Riccardi, V. Genna and M. De Vivo, Nat. Rev. Chem., 2018, 2, 100–112 CrossRef CAS.
- O. A. Pemberton, P. Jaishankar, A. Akhtar, J. L. Adams, L. N. Shaw, A. R. Renslo and Y. Chen, J. Med. Chem., 2019, 62, 8480–8496 CrossRef CAS PubMed.
- K. W. Yang, L. Feng, S. K. Yang, M. Aitha, A. E. LaCuran, P. Oelschlaeger and M. W. Crowder, Bioorg. Med. Chem. Lett., 2013, 23, 5855–5859 CrossRef CAS PubMed.
- M. I. Page, A. P. Laws, M. J. Slater and J. R. Stone, Pure Appl. Chem., 1995, 67, 711–717 CrossRef CAS.
- P. Hinchliffe, C. A. Tanner, A. P. Krismanich, G. Labbe, V. J. Goodfellow, L. Marrone, A. Y. Desoky, K. Calvopina, E. E. Whittle, F. X. Zeng, M. B. Avison, N. C. Bols, S. Siemann, J. Spencer and G. I. Dmitrienko, Biochemistry, 2018, 57, 1880–1892 CrossRef CAS PubMed.
- M. A. M. Subbaiah and N. A. Meanwell, J. Med. Chem., 2021, 64, 14046–14128 CrossRef CAS PubMed.
- M. Santucci, F. Spyrakis, S. Cross, A. Quotadamo, D. Farina, D. Tondi, F. De Luca, J. D. Docquier, A. I. Prieto, C. Ibacache, J. Blazquez, A. Venturelli, G. Cruciani and M. P. Costi, Sci. Rep., 2017, 7, 17716 CrossRef PubMed.
- X. C. Li, S. S. Gong, D. Y. Zeng, Y. H. You and Q. Sun, Tetrahedron Lett., 2016, 57, 1782–1785 CrossRef CAS.
- R. C. Bernotas and R. V. Cube, Synth. Commun., 1990, 20, 1209–1212 CrossRef CAS.
- D. S. MacMillan, J. Murray, H. F. Sneddon, C. Jamieson and A. J. B. Watson, Green Chem., 2013, 15, 596–600 RSC.
- T. Christopeit and H.-K. S. Leiros, Bioorg. Med. Chem. Lett., 2016, 26, 1973–1977 CrossRef CAS PubMed.
- Y. Guo, J. Wang, G. Niu, W. Shui, Y. Sun, H. Zhou, Y. Zhang, C. Yang, Z. Lou and Z. Rao, Protein Cell, 2011, 2, 384–394 CrossRef CAS PubMed.
- Y. Kim, M. A. Cunningham, J. Mire, C. Tesar, J. Sacchettini and A. Joachimiak, FASEB J., 2013, 27, 1917–1927 CrossRef CAS PubMed.
- G. Riviere, S. Oueslati, M. Gayral, J. B. Crechet, N. Nhiri, E. Jacquet, J. C. Cintrat, F. Giraud, C. van Heijenoort, E. Lescop, S. Pethe, B. I. Iorga, T. Naas, E. Guittet and N. Morellet, ACS Omega, 2020, 5, 10466–10480 CrossRef CAS PubMed.
- C. Schwechheimer, D. L. Rodriguez and M. J. Kuehn, MicrobiologyOpen, 2015, 4, 375–389 CrossRef CAS PubMed.
- R. N. Jones, H. W. Wilson, W. J. Novick, Jr., A. L. Barry and C. Thornsberry, J. Clin. Microbiol., 1982, 15, 954–958 CrossRef CAS PubMed.
- D. M. Dias and A. Ciulli, Prog. Biophys. Mol. Biol., 2014, 116, 101–112 CrossRef CAS PubMed.
- L. H. E. Wieske, J. Bogaerts, A. A. M. Leding, S. Wilcox, A. A. Rasmussen, K. Leszczak, L. Turunen, W. A. Herrebout, M. Hubert, A. Bayer and M. Erdelyi, ACS Med. Chem. Lett., 2022, 13, 257–261 CrossRef CAS.
- K. Palica, M. Voracova, S. Skagseth, A. Andersson Rasmussen, L. Allander, M. Hubert, L. Sandegren, H. K. Schroder
Leiros, H. Andersson and M. Erdelyi, ACS Omega, 2022, 7, 4550–4562 CrossRef CAS PubMed.
- Z. Sun, L. Hu, B. Sankaran, B. V. V. Prasad and T. Palzkill, Nat. Commun., 2018, 9, 4524 CrossRef PubMed.
- J. B. Baell and G. A. Holloway, J. Med. Chem., 2010, 53, 2719–2740 CrossRef CAS PubMed.
- M. P. Jacobson, D. L. Pincus, C. S. Rapp, T. J. Day, B. Honig, D. E. Shaw and R. A. Friesner, Proteins, 2004, 55, 351–367 CrossRef CAS PubMed.
- X. Zhang, H. Perez-Sanchez and F. C. Lightstone, Curr. Top. Med. Chem., 2017, 17, 1631–1639 CrossRef CAS PubMed.
- M. R. Davis and D. A. Dougherty, Phys. Chem. Chem. Phys., 2015, 17, 29262–29270 RSC.
- I. Kufareva and R. Abagyan, Methods Mol. Biol., 2012, 857, 231–257 CrossRef CAS PubMed.
-
CrysAlisPro, Rigaku Oxford Diffraction Ltd., Yarnton, Oxfordshire, England, 2015 Search PubMed.
- G. M. Sheldrick, Acta Crystallogr., Sect. A: Found. Adv., 2015, 71, 3–8 CrossRef PubMed.
- G. M. Sheldrick, Acta Crystallogr., Sect. C: Struct. Chem., 2015, 71, 3–8 CrossRef PubMed.
- C. F. Macrae, I. Sovago, S. J. Cottrell, P. T. A. Galek, P. McCabe, E. Pidcock, M. Platings, G. P. Shields, J. S. Stevens, M. Towler and P. A. Wood, J. Appl. Crystallogr., 2020, 53, 226–235 CrossRef CAS PubMed.
- G. M. Sastry, M. Adzhigirey, T. Day, R. Annabhimoju and W. Sherman, J. Comput.-Aided Mol. Des., 2013, 27, 221–234 CrossRef PubMed.
- J. R. Greenwood, D. Calkins, A. P. Sullivan and J. C. Shelley, J. Comput.-Aided Mol. Des., 2010, 24, 591–604 CrossRef CAS PubMed.
- J. C. Shelley, A. Cholleti, L. L. Frye, J. R. Greenwood, M. R. Timlin and M. Uchimaya, J. Comput.-Aided Mol. Des., 2007, 21, 681–691 CrossRef CAS PubMed.
- E. Harder, W. Damm, J. Maple, C. Wu, M. Reboul, J. Y. Xiang, L. Wang, D. Lupyan, M. K. Dahlgren, J. L. Knight, J. W. Kaus, D. S. Cerutti, G. Krilov, W. L. Jorgensen, R. Abel and R. A. Friesner, J. Chem. Theory Comput., 2016, 12, 281–296 CrossRef CAS PubMed.
- R. A. Friesner, J. L. Banks, R. B. Murphy, T. A. Halgren, J. J. Klicic, D. T. Mainz, M. P. Repasky, E. H. Knoll, M. Shelley, J. K. Perry, D. E. Shaw, P. Francis and P. S. Shenkin, J. Med. Chem., 2004, 47, 1739–1749 CrossRef CAS PubMed.
- T. A. Halgren, R. B. Murphy, R. A. Friesner, H. S. Beard, L. L. Frye, W. T. Pollard and J. L. Banks, J. Med. Chem., 2004, 47, 1750–1759 CrossRef CAS PubMed.
- R. A. Friesner, R. B. Murphy, M. P. Repasky, L. L. Frye, J. R. Greenwood, T. A. Halgren, P. C. Sanschagrin and D. T. Mainz, J. Med. Chem., 2006, 49, 6177–6196 CrossRef CAS PubMed.
- N. Laraki, N. Franceschini, G. M. Rossolini, P. Santucci, C. Meunier, E. de Pauw, G. Amicosante, J. M. Frère and M. Galleni, Antimicrob. Agents Chemother., 1999, 43, 902–906 CrossRef CAS PubMed.
- S. Siemann, D. P. Evanoff, L. Marrone, A. J. Clarke, T. Viswanatha and G. I. Dmitrienko, Antimicrob. Agents Chemother., 2002, 46, 2450–2457 CrossRef CAS PubMed.
- S. Chen, D. Chen, R. Cai, H. Cui, Y. Long, Y. Lu, C. Li and Z. She, J. Nat. Prod., 2016, 79, 2397–2402 CrossRef CAS PubMed.
- R: A language and environment for statistical computing, R Foundation for Statistical Computing, Vienna, Austria, URL https://www.R-project.org/).
-
H. Wickham, ggplot2: Elegant Graphics for Data Analysis, 2016 Search PubMed.
- J. Thoma and B. M. Burmann, Bio-Protoc., 2020, 10, e3853 CAS.
Footnotes |
† Electronic supplementary information (ESI) available: Dose–response curves, protein sequence alignment, NMR spectra for all synthesized compounds, crystal data, chemical shift perturbation data and histograms. The backbone resonance assignments are deposited to the BMRBI with codes 50945 (NDM-1) 51165 (VIM-2). CCDC 2203659–2203670 and 2209101. The original NMR data, computational coordinates (pdb), and crystallographic structure files (cif) files are available free of charge on Zenodo as DOI: https://doi.org/10.5281/zenodo.6971841. For ESI and crystallographic data in CIF or other electronic format see DOI: https://doi.org/10.1039/d3md00286a |
‡ These authors contributed equally. |
§ Present address: Arxada AG, Lonzastrasse, 3920 Visp, Switzerland. |
¶ Present address: Otto-Diels-Institute für Organische Chemie, Christian-Albrechts-Universität, 24118 Kiel, Germany. |
|| Present address: Lund Protein Production Platform LP3, Sölvegatan 35, SE-223 62 Lund, Sweden. |
** Present address: Red Glead Discovery AB, Medicon Village, SE-223 81 Lund, Sweden. |
|
This journal is © The Royal Society of Chemistry 2023 |
Click here to see how this site uses Cookies. View our privacy policy here.