DOI:
10.1039/D3MD00261F
(Research Article)
RSC Med. Chem., 2023,
14, 1662-1666
En route to local glioblastoma treatment with temozolomide doped hyaluronan fibres: formulation and in vitro cell studies
Received
5th June 2023
, Accepted 2nd August 2023
First published on 3rd August 2023
Abstract
We report the preparation, characterisation and in vitro tests of hyaluronan fibres containing up to 50 w/w% of temozolomide for local glyoblastoma treatment. These fibres form a hydrogel upon contact with cerebrospinal fluid on the treatment spot.
Introduction
Brain tumours are a group of primary and metastatic neoplasms in the central nervous system and are a life-threatening disease with a low survival rate.1 Only 6.9% of patients survive five years post-diagnosis.2 Gliomas of higher-grade malignancy, collectively referred to as high-grade gliomas (HGGs), are the most common primary adult brain tumours. They account for half of all malignant brain tumours and 60% of all glial tumours.3 A general feature of these aggressive tumours is their relatively rapid growth and locally invasive behaviour, characterised by the ability of tumour cells to directly infiltrate the brain parenchyma.4
Brain tumours are removed surgically. Currently, the resection of the lesion is carried out as follows. Under general anaesthesia, after preparation of the surgical field and skin incision, the bone plates are removed. Subsequently, the dura is cut through, under which the lesion itself is located. This is microsurgically removed. This creates a post-resection cavity. Currently, the cavity is left empty; in some cases, haemostatic material is left behind. In high-grade gliomas, a completely radical resection is not possible due to the microinfiltration of distant tissue, so there is a high probability of leaving tumour infiltration in the resection cavity margin.5 This residue tends to cause recurrences. For this reason, after surgery, patients are given a drug that kills the tumour cells.6 Currently, the standard treatment for glioblastoma multiforme is the Stupp protocol, which is a combination of radiotherapy and the use of temozolomide.7 Temozolomide (TMZ) acts as a chemotherapeutic agent and an alkylating agent, and is a first-generation drug used to treat brain tumours. It is administered orally or intravenously. TMZ has a short half-life and needs a high systemic dose to reach therapeutic concentrations in the brain. As a result, various systemic side effects are associated with TMZ therapy, such as headache, nausea, vomiting, fatigue, bone marrow attenuation, and oral ulceration.8 Several nanotherapeutic systems for local treatment have been proposed to increase the concentration of cytostatic drugs in the CNS system, such as micro-reservoir devices, drug-eluting beads, microelectromechanical systems, discrete nanoparticles, magnetic nanoparticles, non-viral gene therapy, PEGylated nanoparticles, radio-sensitizing nanoparticles, convection-enhanced delivery and injectable hydrogels.9 Self-assembling prodrugs (SAPDs), which are capable of spontaneously associating in aqueous solutions to form filamentous nanostructures that allow sustained release of the cytostatic drug, have recently emerged as a new tool for the local treatment of glioblastoma.10 In 2023, Honggang Cui and colleagues presented such a system, in which the anticancer drug paclitaxel was linked to the peptide-based hydrogelator by a disulphide bond.11
In this work, we created new staple microfibres based on hyaluronic acid (HA)12 cross-linked with temozolomide (salt bridges are formed between acidic carboxyl groups of HA and basic nitrogens of the temozolomide molecule) in the form of non-woven fabric or wadding,13 where, after the dissolution of these fibres in physiological fluids, a gel is formed. The resulting gel then releases the chemotherapeutic agent into the surrounding parenchyma in the postresection cavity, thereby increasing its bioavailability at the lesion site and preventing eventual recurrence (Fig. 1).
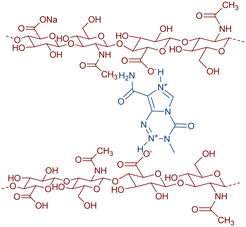 |
| Fig. 1 Temozolomide (in blue) cross-linked with hyaluronan polymer (in red). | |
Results and discussion
Preparation of temozolomide doped hyaluronan fibre
Preparation of a wound cover from stapled hyaluronic acid microfibers containing 50% (mass weight) temozolomide was prepared as follows: sodium hyaluronan (1.8 MDa, 1.3 g) was dissolved in 130 mL of distilled water at 20 °C, forming a clear viscous solution. This solution was used for the preparation of staple microfibers by coagulation in propane-2-ol in a non-stationary coagulation bath. For further processing, the obtained staple microfibers were shortened on a knife mixer to an average length of 0.7 mm and a fibre diameter of around 250 nm. A suspension of the obtained fibres was processed using papermaking technology for wound covers on a substrate–polyamide knitted fabric. Five pieces of wound coverings measuring 11 × 11 cm were obtained. The surface weight of a sheet made of mixed staple microfibers is 2.1 g cm−2. A homogeneous suspension of 0.21 g of temozolomide in 250 mL of propane-2-ol was filtered through a sheet of staple microfibers from hyaluronic acid weighing 0.21 g on a polyamide knitted fabric. After suction of propane-2-ol, immediately without drying, the sheet was calendered on a cloth with a pressure of 45 kg cm−1 and finally dried at 50 °C. After removing the sheet from the underlying fabric, a 11 × 11 cm hyaluronic acid wound cover weighing 0.42 g containing 50% temozolomide was obtained.
The HA fibre forms a hydrogel with water or physiological fluid; after the addition of water, a viscous liquid is formed which turns to a gel within hours. 15 mg of hyaluronic acid is the minimum amount of carrier required to gel 1 mL of water. This process takes 2 hours. The presence of temozolomide has no significant effect on hydrogel formation. 30 mg of the conjugate (50 w/w% temozolomide–HA) in 1 mL of water forms the same gel within two hours. The hydrogel does not change its consistency after three months in an oven at 37 °C.
Cell culture and chemicals
The neuroblastoma cell line UKF-NB-4 and the glioblastoma cell lines U87 and U373 were grown in Iscove's modified Dulbecco's medium (IMDM) supplemented with 10% (v/v) fetal bovine serum (FBS) (both from Thermo Fisher Scientific) and incubated at 37 °C with 5% CO2 and 95% air humidity. Cell lines were passaged at regular intervals twice a week. The cells were washed with PBS and then detached from the surface of the culture flasks with a 0.05% solution of trypsin in PBS and resuspended in a sufficient amount of medium. The cells were cultured in flasks with a culture area of 25 cm2 and 75 cm2.
Preparation of temozolomide solution, sodium hyaluronan gel and hyaluronic acid staple fibers containing temozolomide gel
Sodium hyaluronate (15 mg, HA) with a molecular weight of 1.8 MDa was sterilely dissolved in 1 mL of physiological solution. 15 mg of hyaluronic acid staple microfibers with temozolomide (30% or 50%) was sterilely dissolved in 1 mL of saline. The prepared gels were stored at room temperature. The temozolomide solution was prepared by dissolving 10 g in 1 mL of DMSO (dimethyl sulfoxide) and was stored at 4 °C.
Cell viability assay
The cells were seeded into a 24-well culture plate at 2 × 105 cells per ml and incubated for 24 hours at 37 °C with 5% CO2 and 95% humidity. Subsequently, the tested substances were added to the cell lines diluted in two-fold series: sodium hyaluronan gel with an initial concentration of 4 mg ml−1, temozolomide with an initial concentration of 4 mM, and hyaluronic acid staple microfiber gel containing temozolomide (30% w/w or 50% w/w) with an initial concentration of 4 mM. As a control, cells without the addition of the monitored substances were used. The cells were incubated for 72 hours at 37 °C with 5% CO2 and 95% air humidity. PrestoBlue cell viability reagent (Thermo Fisher Scientific) was then added for 30 min at 37 °C. Fluorescence was measured at an excitation wavelength of 560 nm and an emission wavelength of 590 nm on a SpectraMax i3x Multi-Mode Microplate Reader (Molecular Devices). Each sample was analysed in triplicate.
Monitoring the effect of temozolomide and its combination with sodium hyaluronan
Cells were seeded into a 24-well culture plate at 2 × 105 cells per ml and incubated for 24 hours at 37 °C with 5% CO2 and 95% humidity. Subsequently, temozolomide (TMZ) at a concentration of 500 μM, 2 mg ml−1 sodium hyaluronan gel (HA) and their combination (HA + TMZ) were added to the cell lines. As a control, cells without the addition of the monitored substances were used. Cells were then incubated for 48 and 72 hours at 37 °C with 5% CO2 and 95% humidity. PrestoBlue Cell Viability Reagent (Thermo Fisher Scientific) was then added for 30 min at 37 °C. Fluorescence was measured at an excitation wavelength of 560 nm and an emission wavelength of 590 nm on a SpectraMax i3x Multi-Mode Microplate Reader (Molecular Devices). Each sample was analysed three times.
Cell proliferation
Cell lines were seeded into a 16-well E-plate for impedance-based detection at 104 cells per well. Hyaluronic acid staple fibers containing temozolomide (30% w/w or 50% w/w) at a concentration of 5.4 mM (1.6 mM or 2.6 mM temozolomide) were added to the cells. The xCELLigence RTCA DP Instrument (ACEA Bioscience Inc), placed in a humidified incubator at 37 °C and 5% CO2, was used for real-time monitoring of cell proliferation. The cell index was monitored every 30 min for 174 h and data were analysed with the RTCA software. Each sample was analysed in triplicate.
Statistical analysis
All experiments were independently repeated at least three times and data are shown as averages ± standard error. One-way ANOVA with post hoc Tukey HSD test was utilized to compare the situations. Significance (p < 0.05 was considered as significant) of the statistical analyses is shown in individual figures and described in their legends.
Hyaluronic acid does not affect cell viability
To determine the effect of sodium hyaluronan on the UKF-NB-4 neuroblastoma cell line and glioblastoma cell lines U87 and U373, the cells were exposed to this compound in different concentrations for 48 hours with subsequent measurement of cell viability. The results show no adverse effect of sodium hyaluronan on cell viability in all cell lines (Fig. 2).
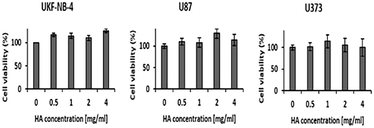 |
| Fig. 2 Effect of sodium hyaluronan on UKF-NB-4 cells. Tested cells were exposed to sodium hyaluronan (HA) gel for 48 hours and its effect on viability was monitored, which did not change in any way compared to the control. | |
Temozolomide reduces cell viability
Temozolomide reduces viability (p < 0.01) for the UKF-NB-4 neuroblastoma cell line, and the U87 and U373 glioblastoma cell lines after 72 h (Fig. 3A), depending on its concentration starting from 500 μM. Based on the results of the viability tests, a temozolomide concentration of 500 μM was selected and its effect on viability was monitored in combination with the sodium hyaluronan gel; after 72 hours there was a decrease in viability (p < 0.01) in both neuroblastoma and glioblastoma cell lines (Fig. 3B).
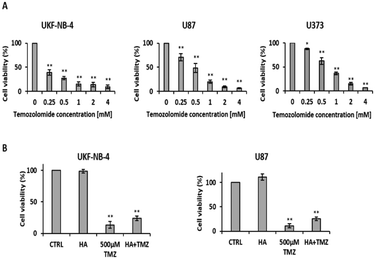 |
| Fig. 3 Temozolomide reduces cell viability. Viability of UKF-NB-4 and U87 measured by PrestoBlue at 72 h with increasing concentration of temozolomide (A) and after incubation with 500 μM temozolomide (TMZ), 2 mg ml−1 sodium hyaluronan gel (HA) and their combination (HA + TMZ) (B). Significance ** p < 0.01, * p < 0.05 (ANOVA with post hoc Tukey HSD test). | |
Staple fibres with bound temozolomide affect viability and proliferation of tumour cells
After establishing the cytotoxicity of temozolomide alone and the influence of sodium hyaluronan on cell lines, the staple microfibers were tested with a temozolomide content of 30% w/w and 50% w/w. There was a decrease (p < 0.01) in viability after 72 h incubation with the staple microfiber gel containing 30% w/w temozolomide in UKF-NB-4 at a temozolomide concentration of 1 mM, in U87 at a temozolomide concentration of 2 mM and in U373 at a temozolomide concentration of 4 mM. The microfiber gel containing 50% w/w temozolomide decreased viability at lower concentrations compared to the gel containing 30% w/w temozolomide (Fig. 4A and B). Proliferation was also reduced in both neuroblastoma and glioblastoma cell lines (Fig. 4C).
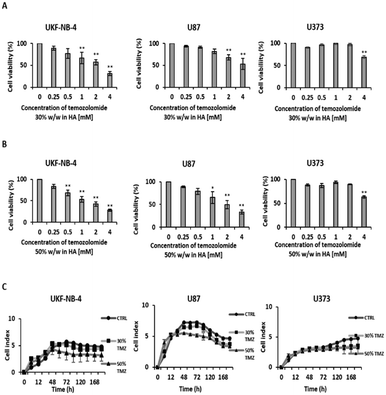 |
| Fig. 4 Staple fibres with bound temozolomide have an effect on viability and proliferation of tumour cells. Viability was measured by PrestoBlue at 72 h with increasing concentrations of temozolomide in a hyaluronic acid staple microfiber gel containing 30% w/w (A) and 50% w/w (B) temozolomide. Proliferation was tested using the xCELLigence system after exposure of cell lines to hyaluronic acid staple microfibers containing temozolomide (30% w/w or 50% w/w) at a concentration of 5.4 mM (1.6 mM or 2.6 mM temozolomide) (C). Significance ** p < 0.01 (ANOVA with post hoc Tukey HSD test). | |
Conclusions
We have developed a method for the preparation of hyaluronic microfibers containing up to 50% by weight of temozolomide, both in the form of staple microfibers and in the form of cotton wool. Both modifications form a hydrogel in contact with cerebrospinal fluid and thus can be applied to load the required amount of cancerostatic in proximity to the tumour. Both modifications are subjected to further tests on infected mice.
Author contributions
M. B. and A. V. performed in vitro tests of fibres containing the anticancer agent temozolomide, L. B. and R. H. established a new protocol for the formation of hyaluronic acid–temozolomide conjugate fibres, I. B. and L. M. performed biocompatibility and biodegradability tests of fibres with and without temozolomide, T. M. and D. N. proposed a formulation of the material for surgeons' needs, and Z. M. and R. H. contributed to the concept and wrote the manuscript. All authors approved the final version of the manuscript.
Conflicts of interest
There are no conflicts to declare.
Acknowledgements
This work was supported by the Cooperatio Program, research area “Oncology and Haematology”. We thank the Faculty of Chemical Technology, University of Pardubice, for the financial support of LM within the project SG 331003.
Notes and references
- J. R. McFaline-Figueroa and E. Q. Lee, Am. J. Med., 2018, 131, 874–882 CrossRef PubMed.
- Q. T. Ostrom, M. Price, C. Neff, G. Cioffi, K. A. Waite, C. Kruchko and J. S. Barnholtz-Sloan, Neuro-Oncology, 2022, 24, v1–v95 CrossRef CAS PubMed.
- Q. T. Ostrom, H. Gittleman, P. Farah, A. Ondracek, Y. Chen, Y. Wolinsky, N. E. Stroup, C. Kruchko and J. S. Barnholtz-Sloan, Neuro-Oncology, 2013, 15, ii1–ii56 CrossRef PubMed.
- M. P. Pospíšil, M. T. Kazda, P. Šlampa and R. Jančálek, Neurol. Praxi., 2016, 17, 287–292 CrossRef.
-
(a) M. Weller, M. van den Bent, M. Preusser, E. Le Rhun, J. C. Tonn, G. Minniti, M. Bendszus, C. Balana, O. Chinot, L. Dirven, P. French, M. E. Hegi, A. S. Jakola, M. Platten, P. Roth, R. Rudà, S. Short, M. Smits, M. J. B. Taphoorn, A. von Deimling, M. Westphal, R. Soffietti, G. Reifenberger and W. Wick, Nat. Rev. Clin. Oncol., 2021, 18, 170–186 CrossRef PubMed;
(b) C. C. Parkins, J. H. McAbee, L. Ruff, A. Wendler, R. Mair, R. J. Gilbertson, C. Watts and O. A. Scherman, Biomaterials, 2021, 276, 120919 CrossRef CAS PubMed.
- R. Vengoji, M. A. Macha, R. K. Nimmakayala, S. Rachagani, J. A. Siddiqui, K. Mallya, S. Gorantla, M. Jain, M. P. Ponnusamy, S. K. Batra and N. Shonka, J. Exp. Clin. Cancer Res., 2019, 38, 1–13 CrossRef PubMed.
- R. Stupp, W. P. Mason, M. J. van den Bent, M. Weller, B. Fisher, M. J. B. Taphoorn, K. Belanger, A. A. Brandes, C. Marosi, U. Bogdahn, J. Curschmann, R. C. Janzer, S. K. Ludwin, T. Gorlia, A. Allgeier, D. Lacombe, J. G. Cairncross, E. Eisenhauer and R. O. Mirimanoff, N. Engl. J. Med., 2005, 352, 987–996 CrossRef CAS PubMed.
-
(a) S. H. Bae, M. J. Park, M. M. Lee, T. M. Kim, S. H. Lee, S. Y. Cho, Y. H. Kim, Y. J. Kim, C. K. Park and C. Y. Kim, J. Korean Med. Sci., 2014, 29, 980 CrossRef CAS PubMed;
(b) B. Neyns, A. Tosoni, W. J. Hwu and D. A. Reardon, Cancer, 2010, 116, 2868–2877 CrossRef CAS PubMed;
(c) W. Wick, M. Platten and M. Weller, Neuro-Oncology, 2009, 11, 69–79 CrossRef CAS PubMed.
- R. W. Chakroun, P. Zhang, R. Lin, P. Schiapparelli, A. Quiñones-Hinojosa and H. Cui, WIREs Nanomed. Nanobiotechnol., 2018, 10, e1479 Search PubMed.
- P. Schiapparelli, P. Zhang, M. Lara-Velazquez, H. Guerrero-Cazares, R. Lin, H. Su, R. W. Chakroun, M. Tusa, A. Quiñones-Hinojosa and H. Cui, J. Controlled Release, 2020, 319, 311–321 CrossRef CAS PubMed.
- F. Wang, Q. Huang, H. Su, M. Sun, Z. Wang, Z. Chen, M. Zheng, R. W. Chakroun, M. K. Monroe, D. Chen, Z. Wang, N. Gorelick, R. Serra, H. Wang, Y. Guan, J. S. Suk, B. Tyler, H. Brem, J. Hanes and H. Cui, Proc. Natl. Acad. Sci. U. S. A., 2023, 120, e2204621120 CrossRef CAS PubMed.
-
(a) P. Snetkov, K. Zakharova, S. Morozkina, R. Olekhnovich and M. Uspenskaya, Polymer, 2020, 12, 1800 CrossRef CAS PubMed;
(b) M. Barbarisi, R. V. Iaffaioli, E. Armenia, L. Schiavo, G. De Sena, S. Tafuto, A. Barbarisi and V. Quagliariello, J. Cell. Physiol., 2018, 233, 6550–6564 CrossRef CAS PubMed;
(c) R. R. Kudarha and K. K. Sawant, J. Drug Delivery Sci. Technol., 2021, 61, 102129 CrossRef CAS;
(d) P. M. Kasapidou, E. Laillet de Montullé, K.-P. Dembélé, A. Mutel, L. Desrues, V. Gubalaa and H. Castel, Soft Matter, 2021, 17, 10846–10861 RSC;
(e) B. Stawicki, T. Schacher and H. Cho, Gels, 2021, 7, 63 CrossRef CAS PubMed;
(f) S. Pedron, J. S. Hanselman, M. A. Schroeder, J. N. Sarkaria and B. A. C. Harley, Adv. Healthcare Mater., 2017, 6, 1700529 CrossRef PubMed;
(g) M. J. Rowland, C. C. Parkins, J. H. McAbee, A. K. Kolb, R. Hein, X. J. Loh, C. Watts and O. A. Scherman, Biomaterials, 2018, 179, 199–208 CrossRef CAS PubMed;
(h) A. Malfanti, G. Catania, Q. Degros, M. Wang, M. Bausart and V. Préat, Pharmaceutics, 2022, 14, 124 CrossRef CAS PubMed;
(i) A. E. Erickson, S. K. L. Levengood, J. Sun, F.-C. Chang and M. Zhang, Adv. Healthcare Mater., 2018, 7, 1800295 CrossRef PubMed;
(j) J. S. Hartheimer, S. Park, S. S. Rao and Y. Kim, Cancer Microenviron., 2019, 12, 47–56 CrossRef CAS PubMed;
(k) M. Ganau, Clin. Transl. Oncol., 2014, 16, 220–223 CrossRef CAS PubMed;
(l) M. S. Karbownik, T. Pietras, J. Szemraj, E. Kowalczyk and J. Z. Nowak, Contemporary Oncology, 2014, 324–328 Search PubMed.
-
(a) A. M. Abdel-Mohsen, R. Hrdina, L. Burgert, G. Krylová, R. M. Abdel-Rahman, A. Krejčová, M. Steinhart and L. Beneš, Carbohydr. Polym., 2012, 89, 411–422 CrossRef CAS PubMed;
(b) A. M. Abdel-Mohsen, R. Hrdina, L. Burgert, M. Rasha, R. M. Abdel-Rahman, M. Hašová, D. Šmejkalová, M. Kolář, M. Pekař and A. S. Aly, Carbohydr. Polym., 2013, 92, 1177–1187 CrossRef CAS PubMed;
(c)
L. Burgert, R. Hrdina, D. Masek and V. Velebny, PCT Int. Appl., WO2012089179, A120120705, 2012;
(d)
L. Burgert, R. Hrdina, V. Velebny, A. Lattif, A. Mohsen, R. Sulakova, L. Sobotka, J. Betak and D. Smirnou, PCT Int. Appl., WO2013167098, A220131114, 2013.
|
This journal is © The Royal Society of Chemistry 2023 |
Click here to see how this site uses Cookies. View our privacy policy here.