DOI:
10.1039/D3FO03085G
(Paper)
Food Funct., 2023,
14, 10163-10176
Acute effects of drinks containing blackcurrant and citrus (poly)phenols and dietary fibre on postprandial glycaemia, gut hormones, cognitive function and appetite in healthy adults: two randomised controlled trials†
Received
27th July 2023
, Accepted 23rd October 2023
First published on 24th October 2023
Abstract
(Poly)phenol (PP)-rich blackcurrant (BC) extracts reduce postprandial glucose concentrations. Combinations with other fruit (poly)phenols and fruit fibre may enhance the effect. This study investigated the acute effects of combinations of BC extracts, high (H-BC) and low (L-BC) (poly)phenol concentrations, sweet orange extracts (SO) and fibre-rich orange pulp (F) in reducing postprandial glycaemia. In two randomised, double-blind, crossover design studies, healthy participants consumed seven types of 200 mL beverages: in the GLU-FX trial, H-BC (1600 mg PP); L-BC (800 mg PP); SO (800 mg PP); BC + SO (1600 mg PP) or CON (placebo); in the GLU-MIX trial, BC + F (800 mg PP), F (1.5 g fibre), or CON2 (placebo), immediately followed by consumption of 75 g available carbohydrate (starch and sugars). Blood was sampled at baseline and postprandially to measure changes in glucose, insulin, and gut hormones; appetite changes were assessed by visual analogue scales and, in GLU-MIX, ad libitum food intake and cognitive function were assessed. Twenty-nine and thirty-seven adults completed GLU-FX and GLU-MIX, respectively. L-BC reduced early postprandial glycaemia (0–30 min) with no differences in glucose incremental Cmax or total glycaemic response. No significant effect was observed following other drinks relative to CON. L-BC and H-BC drinks inhibited insulin secretion up to 30 min and GIP up to 120 min. In GLU-MIX, BC + F improved some indicators of cognitive function but not all. Measures of appetite were unaffected. The impact of (poly)phenol-rich BC extracts on total postprandial glycaemia in healthy participants was minimal and not enhanced when administered in combination with an orange (poly)phenol extract or orange pulp. Clinical Trials registered at https://www.clinicaltrials.gov: NCT03184064 (GLU-FX) and NCT03572296 (GLU-MIX).
Introduction
Type 2 diabetes (T2D) prevalence and mortality rates are rising globally.1 One in 5 adults in Europe have impaired fasting glucose and/or impaired glucose tolerance;2 targeting this group with lifestyle interventions could reduce rates of progression to cardiometabolic diseases.3 Regular exposure to elevated postprandial glycaemia can increase intermediary risk factors for T2D and cardiovascular disease risk including increased oxidative stress, glycation of functional proteins, pancreatic beta cell dysfunction and endothelial cell dysfunction.4–7 Dietary modifications aiming to moderate postprandial glucose concentrations may contribute to improved long-term glycaemic control.
(Poly)phenols are a large group of phytochemicals containing one or more phenol rings, including flavonoids, stilbenes, phenolic acids and lignans, and are found widely in plant foods and beverages. Epidemiological associations between increased dietary (poly)phenol intake and lower risk of diabetes8 may be at least partly attributable to their capacity to slow the rate of glucose absorption.9–13 This may occur by several processes, including inhibition of digestive enzyme activity,14 reduction of sodium-glucose linked transporter 1 (SGLT-1)/glucose transporter 2 (GLUT-2) activity,12 or potentially by binding to starch molecules. Different (poly)phenols may impact glucose homeostasis in various ways. For example, (poly)phenols derived from citrus fruits (mainly flavanones), only demonstrate a weak inhibition of digestive enzyme activity but may moderate carbohydrate digestion by binding to starch.15 However, (poly)phenols derived from berries (anthocyanin and proanthocyanidin-rich extracts) may influence postprandial glycaemia primarily via inhibition of digestive carbohydrases.14 In addition, other fruit components, namely water-soluble forms of dietary fibre (e.g., pectins), may also impact on carbohydrate digestion by slowing gastric emptying rates,16 interacting with the intestinal mucous layer, and decreasing starch amylolysis.17–19 It should be noted, however, that doses of pectin shown to be effective in delaying gastric emptying are technically challenging to introduce into foods or beverages without compromising product acceptability. The effects of flavanones on postprandial glycaemia and related metabolites are poorly characterised. Furthermore, the effects on postprandial glycaemia when combining different subclasses of flavonoids, such as flavanones and anthocyanins, have not been studied. Finally, there is some evidence showing acute beneficial effects of (poly)phenols on cognitive function,20 thought to be mediated by increased cerebral blood flow,21 with potential applications in work and academic environments where optimal cognitive performance is desirable.22
The overarching hypothesis of our studies was that combining fruit (poly)phenol extracts and fruit fibre in a drink would have a greater inhibitory effect on the increase in blood glucose concentrations than individual constituents. The first specific aim was to determine the acute effects of drinks containing blackcurrant (BC) and sweet orange (SO) (poly)phenol extracts, separately and in combination, on postprandial glycaemia, insulinaemia, gut hormone secretion, and appetite following a high-carbohydrate (starch/sugar-based) meal. The hypothesis of this study was that a combination of BC and SO extracts would have a greater inhibitory effect on early postprandial glycaemia (iAUC0–30 min, mainly reflecting the rate of intestinal absorption) relative to placebo than either BC or SO (poly)phenol extracts alone (the GLU-FX study). The second specific aim was to determine the effects of drinks containing (poly)phenol-rich fruit extracts combined with fibre (orange pulp), on postprandial glycaemia, insulinaemia, cognitive function and appetite following a high-carbohydrate (starch/sugar based) meal. The hypothesis of the second study was that a combination of BC and fibre-rich citrus pulp would reduce iAUC0–30 min relative to placebo to a greater extent than the drink containing citrus pulp alone (the GLU-MIX study).
Materials and methods
Study participant selection and recruitment
Participants were recruited from King's College London (KCL) and the general public in the London area. The study was advertised using circular emails and posters within KCL, and via social media and newspapers. A participant information sheet was provided to volunteers who expressed interest. Respondents were initially interviewed over the telephone to establish eligibility. Inclusion criteria were: healthy men and women aged 18–70 years, BMI 18–35 kg m−2, able to understand the information sheet and willing to comply with the study protocol and able to give informed written consent. Exclusion criteria were: phenylketonuria, food intolerances, allergies or hypersensitivity, participation in another clinical trial, donation of more than 1500 mL blood in the previous 12 months, full blood counts and liver function test results outside of the normal range, current smokers, or reported giving up smoking within the last 6 months, history of substance abuse or alcoholism, reported history of cardiovascular disease, diabetes (or fasting glucose ≥7.1 mmol L−1), cancer, kidney, liver or bowel disease, gastrointestinal disorder or use of drug likely to alter gastrointestinal function, unwilling to restrict consumption of specified high (poly)phenol foods for 24 h before the study, weight loss >3 kg in preceding 2 months and BMI <18 or >35 kg m−2, blood pressure ≥160/100 mmHg, total cholesterol ≥7.5 mmol L−1, fasting triacylglycerol concentrations ≥5.0 mmol L−1, current use of medications that may interfere with the study such as α-glucosidase inhibitors (acarbose), insulin-sensitising drugs (metformin), sulfonylureas, and lipid-lowering drugs, and current use of nutritional supplements that may interfere with the study such as higher dose vitamins/minerals (>200% reference nutrient intake, B vitamins, vitamin C, calcium, copper, chromium, iodine, iron, magnesium, manganese, phosphorus, potassium and zinc).
Eligible participants were invited to a screening visit in the Metabolic Research Unit at the Department of Nutritional Sciences, KCL in a fasting state for measurements of height, weight, waist circumference, % body fat (Tanita Body Composition Analyzer, Tanita Corp., Tokyo, Japan), seated blood pressure, liver function tests, haematology, plasma glucose and lipid profile (total cholesterol, HDL, LDL and triglycerides). Subjects who met all inclusion and exclusion criteria were randomised to a treatment sequence schedule.
Study design
The GLU-FX trial (carried out from May to December 2017, registered at clinicaltrials.gov, NCT03184064) and GLU-MIX trial (carried out from June 2018 to January 2019, registered at clinicaltrials.gov, NCT03572296) were conducted at the Department of Nutritional Sciences’ metabolic research unit, King's College London, and received ethical approval from the King's College London ethics committee. Both studies abided by the principles outlined in the Declaration of Helsinki as revised in 2013, and were compliant with the UK General Data Protection Regulation (2018) and Human Tissues Act (2004).
The GLU-FX trial.
A randomised, controlled, double-blind, cross-over, incomplete block, study design was used with at least 7 days wash-out between each treatment (ESI Fig. 1†). The randomisation schedule was generated according to a balanced treatment incomplete block design, whereby each subject was assigned placebo in one of the study periods, plus 3 of the 4 test treatments in the other study periods. A power calculation was conducted to determine that a sample size of 28 was required to detect a difference of 0.42 mmol L−1 in the primary outcome (plasma glucose iAUC0–30 min), which represents a 30% reduction (using data from our previous study using an anthocyanin-rich blackcurrant extract,13 where the mean response was 1.42 and SD 0.39 mmol L−1 following control treatment, and the correlation between responses across periods within the same subject was 0.235), with 80% power to show that any 3 of the 4 test treatments are significantly different to placebo. These calculations were performed using 5000 simulations, under a 2-sided 5% significance level with Dunnett's adjustment for multiple comparisons. To account for a 12.5% attrition rate, 32 subjects were randomised into the trial.
Drinks were supplied by the New Product Development team Lucozade Ribena Suntory (LRS) and standardised to contain 0 mg (poly)phenols (Placebo),7 1600 mg BC (poly)phenols (H-BC), 800 mg BC (poly)phenols (L-BC), 800 mg SO (poly)phenols (L-SO) and 800 mg BC + 800 mg SO (poly)phenols (H-Blend). Highly purified extracts instead of whole fruits were used in test drinks. The liquid BC extract (BerryPharma® by Iprona AG, Lana, Italy) contained 2765.5 mg total (poly)phenols per 100 g extract (2001 mg total anthocyanins), analysed by Reading Scientific Services Ltd (Reading, UK) using the Folin–Ciocalteu method and the pH differential method with spectrophotometry23 for determination of total (poly)phenols and anthocyanin content, respectively. The liquid SO extract (Brewster Brand Citrus Bioflavonoids from Ingredients by Nature, California, USA) contained 90.2% hesperidin, confirmed in analysis by Ingredients by Nature, using HPLC. Drinks were approximately equivalent in total sugar and energy content and each provided 246–273 kJ and 15–16 g sugar in 200 mL (see ESI Table 1† for nutritional breakdown) and were all blackcurrant flavoured. Test drinks were dispensed into identical opaque brown bottles and labelled with codes by an external research technician. For information on control and test meals on treatment days see following subsection “GLU-FX and GLU-MIX Trial Protocol”.
Blood was collected for analysis of glucose, insulin, C-peptide, non-esterified fatty acid (NEFA), glucose-dependent insulinotropic polypeptide (GIP), peptide YY (PYY) and glucagon-like peptide-1 (GLP-1) concentrations prior to the test drink and afterwards at timepoints 10, 20, 30, 45, 60, 75, 90 and 120 min from the test drink and meal consumption (T0). Additionally, a 100 mm visual analogue scale24 questionnaire was used to gauge mood and satiety prior to the test drink and afterwards at timepoints 10, 30, 60, 90 and 120 min, as well as evaluating perceived palatability of the test drink at T10 min.
The GLU-MIX trial.
A randomised, controlled, double-blind, cross-over, study design was used to allow subjects to receive all 3 test drinks (control (CON2), BC (poly)phenols + fruit pulp fibre (BC + F), and fruit pulp fibre (F)) in random order at three separate study visits with at least 7 days wash-out between each visit (ESI Fig. 2†). The GLU-MIX randomisation schedule was generated according to a Williams square, for a 3 by 3 crossover study using SAS version 9.4. A sample size of 36 subjects completing all treatments had 90% power at the two-sided 5% significance level to detect differences in plasma glucose iAUC0–30 min of 0.23 mmol L−1 between BC + F and CON2, which represents a 18% reduction (based on a mean difference of 0.23 mmol L−1 between CON and 800 mg BC in the GLU-FX study, with an SD of 0.29 mmol L−1). To account for a ∼5% attrition rate, 38 subjects were randomised into the study.
Drinks were supplied by the New Product Development team at Lucozade Ribena Suntory (LRS) and standardized to contain 0 mg (poly)phenols and 0 mg fibre (CON2), 800 mg BC (poly)phenols + 1.5 g total fibre (BC + F), and 1.5 g total fibre (F). The liquid BC extract (BerryPharma® by Iprona AG, Lana, Italy) contained 3172 mg/100 g (Folin–Ciocalteu method) and 1959 mg/100 g anthocyanins (pH differential method with spectrophotometry23). The fruit pulp was derived from orange pomace and was supplied by Citresa, Orangina Schweppes Suntory (Citricos Y Refrescantes, Spain); BC + F and F drinks constituted 15% orange pulp which provided 1.5 g fibre and 232 mg pectin per 200 ml drink (ESI Table 1†). Total fibre was analysed by Eurofins Analisis Alimentario Nordeste SL, Barcelona, Spain by enzymatic-gravimetry (internal method). The in-house method for analysis of pectin content is provided in ESI.†
Drinks were matched for total sugar and energy content and each test drink consisted of a blackcurrant-flavoured fruit drink providing 112–136 kJ and 7 g total carbohydrate (ESI Table 1†).
Blood was collected for analysis of glucose, insulin, C-peptide and GIP concentrations prior to the test drink and meal consumption and afterwards at timepoints 10, 20, 30, 45, 60, 75, 90, 120 and 150 min. A 30 min cognitive battery test was completed at T-45 and 160 min. Additionally, a 100 mm VAS questionnaire was used to measure mood (happiness, alertness, energy) and appetite (hunger, fullness, desire to eat, prospective food consumption) prior to the test drink and meal consumption and afterwards at 10, 30, 60, 90, 120, 150 and 230 min and palatability of the test drink was assessed 10 min after consumption.
Participants were provided with an ad libitum vegetarian pasta lunch (tomato sauce and cheese; see ESI Table 2† for nutritional composition) at 215 min after the test drink to assess food intake as an additional measurement of appetite changes, as previously reported.25,26 Energy intake (kcal−1) was calculated from the total amount of food consumed (in grams) at the end of each test period.
GLU-FX and GLU-MIX trial protocol
The investigators, laboratory technicians and participants were blinded to allocation of treatment schedule until after the study was completed and data analysis was performed. Test drinks (200 mL) were provided in opaque brown glass bottles with codes so that the identity of the treatment was concealed from investigators and participants, with a black straw, to mask slight differences between treatment drinks for colour and flavour, respectively. Drinks were stored frozen and were removed from the freezer and placed in a fridge, allowing 48 h to defrost followed by thorough mixing, before each study visit. On the day before each study visit, participants were told not to participate in strenuous exercise and to avoid alcohol, caffeine, oily fish, high-(poly)phenol foods (from a list provided), and high-fat foods. In addition, the participants on the GLU-MIX study were also asked to avoid high-fibre foods (included in the list provided). Participants were asked to consume a standard low-fat meal the evening before each visit (a list of ready-meals, available from the main UK supermarkets, that conformed with the dietary guidance provided, was given to participants to choose from) and arrive at the metabolic research unit, between 0800 h and 1030 h (the same time on each visit), following a 12 h fast. On study days, a venous cannula was inserted in a vein in the antecubital fossa or a forearm vein by a trained phlebotomist, and baseline samples were collected for analysis of the respective study outcomes. Glucose baseline samples were taken in duplicate (at −10 and −5 min) and their average taken as baseline concentrations. After baseline measurements and blood sampling, test drinks were consumed within a 2 min period immediately followed by consumption of the standardised high-carbohydrate meal consisting of 100 g of white bread (Hovis, London, UK) spread with 30 g of a low-(poly)phenol apricot jam (<6 mg (poly)phenols/100 g fresh weight; Hartley's, Hain Daniels Group, Leeds, UK), providing 1284 kJ, 63 g available carbohydrate (41 g starch and 22 g as sugars), 2.4 g fibre, 8.9 g protein, 1.9 g fat (0.3 g saturated fatty acids). Altogether, test drinks and the high carbohydrate meal were consumed within 12 min. Blood samples were taken at regular intervals following the first sip of the drink (time zero min). Participants had access to water to sip as required over the study period. Adverse events were recorded at all study visits where necessary by asking the following question during each visit, including any follow-up visits or telephone calls and documenting the reply: “Have you felt unwell, experienced any symptoms or taken any medication today or since the last session?”.
Methods
Blood samples were collected into BD Vacutainer® tubes with: fluoride oxalate tubes for glucose analysis and SST™II tubes for TAG, insulin, NEFA and C-peptide analysis. Blood samples for GIP, GLP-1, and PYY analysis were collected into K3EDTA tubes with added aprotinin (500 KIU mL−1 blood, Nordic Pharma) and DPP-IV (10 μL mL−1 blood, Merck Millipore). All samples were centrifuged at 1300g, 4 °C for 15 min and plasma and serum aliquots were stored frozen at −80 °C until used for biochemical analyses, performed by a clinical pathology accredited biochemistry laboratory: Affinity Biomarkers Lab, London.
Plasma glucose was determined enzymatically on a Siemens Advia 1800 auto-analyser, and insulin and C-peptide by Siemens Centaur XP (Siemens Healthcare Diagnostics Ltd, Frimley, Surrey, UK). Plasma gut hormone concentrations (GIP, GLP-1 and PYY) were determined by electro-chemiluminescent multiplexed assay (Mesoscale Discovery, MD, USA).
Visual Analogue Scales24 were completed with participants marking responses to mood and satiety questions (“How happy do you feel?”, “How alert do you feel?”, “How hungry do you feel?”, “How full do you feel?”, “How energised do you feel?”, “How would you rate your digestive comfort?”, “How much do you think you can eat?”, “How strong is your desire to eat?”, “How mentally alert do you feel?”, “How much energy do you have?”) using a 100 mm scale where questions were anchored from “Not at all” to “A lot/extremely”. Palatability questions using 100 mm VAS were also completed after the test drink and meal and after the ad libitum meal.
Cognitive performance was assessed with a 30 min cognitive battery test using the Computerised Mental Performance Assessment System (COMPASS, Northumbria University, Newcastle Upon Tyne, UK). The computer-based cognitive test included the following tests and respective cognitive domains: choice reaction time (attention/response), RVIP (rapid visual information processing, a measure of working memory), simple reaction time (psychomotor speed/attention), Stroop task (attention/response inhibition), four-choice reaction time (attention), serial 3 subtraction (cognitive performance/working memory). Reaction times were measured in milliseconds and accuracy was measured as a percentage of correct responses.
Statistical analysis
Statistical analysis was performed on the intention-to-treat population using SAS version 9.4 by the study statistician. The intention-to-treat population was defined as all subjects who received at least one of the study treatments and who had at least one assessment post-baseline. The primary efficacy endpoint was iAUC0–30 min for plasma glucose, which is defined as the area under the change from baseline plasma glucose concentration versus time curve from zero to the 30 min sample time. The iAUC was calculated using the trapezoidal rule. To convert the results back to the original units of measurement (mmol L−1), the iAUC was divided by the total duration (30 min). The iAUC0–30 min was analysed using a linear mixed model. Terms in the model included treatment group and period as fixed effects, subject as a random effect, and subject-level and period-level baseline glucose as covariates. For GLU-FX, two-sided treatment comparison Dunnett's tests were performed with adjustments for multiple comparisons between placebo and test treatments; treatment differences are presented with Dunnett's adjusted 95% confidence intervals. Normality and homogeneity of variance assumptions were investigated. Violation of these assumptions were overcome by using suitable transformations or performing a non-parametric test.
The secondary endpoints were: iAUC0–120 min (GLU-FX) and iAUC0–150 min (GLU-MIX) for plasma glucose concentrations; iAUC0–30 min and iAUC0–120 min (GLU-FX) and iAUC0–150 min (GLU-MIX) for plasma insulin, C-peptide, NEFA, PYY, GLP-1 and GIP concentrations; incremental Cmax (iCmax) for plasma glucose, insulin, C-peptide and GIP concentrations, defined as the maximum change from baseline over the total sampling period; Tmax for plasma glucose, insulin, C-peptide, NEFA, PYY, GLP-1 and GIP concentrations; plasma concentrations of glucose, insulin, C-peptide, NEFA and GIP at each time point; responses to mood and satiety questions up to 150 min, using a 100 mm VAS questionnaire; ad libitum energy intake, determined from the total amount of pasta consumed at the end of each treatment period; and cognitive testing scores at baseline (−45 min) and 160 min. The same linear mixed model or non-parametric statistical procedures were conducted as for the primary outcome.
Results
GLU-FX trial
Thirty-two participants were randomised to treatment and 29 completed the study (Fig. 1a). The characteristics of those who completed the study are show in Table 1. Participants had an average age of 37 years with approximately 60% female, the majority being white (56%) followed by black (16%) ethnicity, normal systolic and diastolic blood pressures, and normal fasting glucose concentrations and lipids. One subject was not fully compliant on H-Blend (did not consume whole test drink within 2 minutes and high-carbohydrate meal within 7 minutes) and another subject was not fully compliant on placebo. Results are shown for the intention to treat population (subjects who received at least one of the study treatments and had at least one assessment post-baseline).
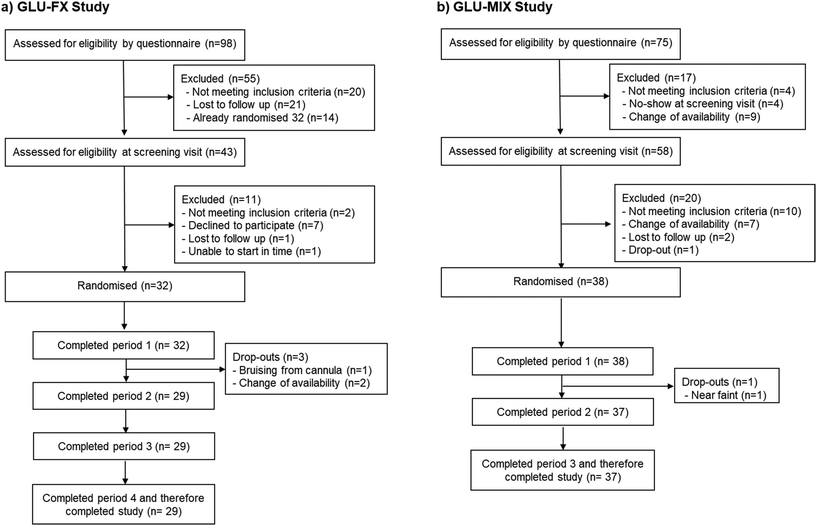 |
| Fig. 1 CONSORT flow diagram of participants in the GLU-FX and GLU-MIX trials. | |
Table 1 Baseline characteristics of healthy subjects who were randomised to the GLU-FX (n = 32) and GLU-MIX study (n = 38)
Variable |
GLU-FX |
GLU-MIX |
Values are expressed as means (SD) or n (%). Median (IQR). |
Age, year |
37.3 (16.3) |
37.3 (15.2) |
Sex, M : F ratio |
13 : 19 |
16 : 22 |
|
Ethnicity, n (%)
|
White |
18 (56.3) |
22 (57.9) |
Asian |
3 (9.4) |
3 (7.9) |
Chinese/East Asian |
3 (9.4) |
2 (5.3) |
Black |
5 (15.6) |
6 (15.8) |
Mixed |
2 (6.3) |
5 (13.2) |
Other |
1 (3.1) |
0 (0) |
Body mass index, kg m−2 |
23.1 (3.1) |
25.0 (4.5) |
Systolic blood pressure, mmHg |
110.3 (10.1) |
109.8 (15.0) |
Diastolic blood pressure, mmHg |
68.9 (8.0) |
73.3 (9.5) |
Fasting plasma glucose, mmol l−1 |
4.9 (0.6) |
4.9 (0.6) |
Fasting plasma triacylglycerol, mmol l−1 |
0.78 (0.25) |
0.94 (0.57) |
Fasting plasma total cholesterol, mmol l−1 |
4.4 (0.8) |
4.8 (0.9) |
|
Background dietary intake
|
Total energy (kcal day−1) |
1933 (549) |
1665 (538) |
Carbohydrate (% kcal) |
44.8 (7.17) |
44.9 (9.37) |
Protein (% kcal) |
16.6 (2.93) |
16.8 (4.11) |
Fat (% kcal) |
35.8 (6.17) |
37.0 (10.49) |
Fibre (g day−1) |
22.0 (9.11) |
22.1 (10.78) |
Total polyphenols (mg day−1) |
807 (497) |
892 (466) |
Anthocyanins (mg day−1) |
17.2 (78.0)a |
25.5 (55.6)a |
Postprandial glycaemia.
Only the L-BC drink significantly lowered plasma glucose concentrations (Table 2) in the early postprandial period compared to the placebo (mean difference by Dunnett's tests L-BC vs. CON (iAUC0–30 min) [95% CI] −0.22 mmol L−1 [−0.44, −0.01]). No treatment effects were observed over the full postprandial period (iAUC0–120 min), and there were no differences in incremental Cmax (iCmax) or Tmax. Glycaemic responses are shown graphically in Fig. 2.
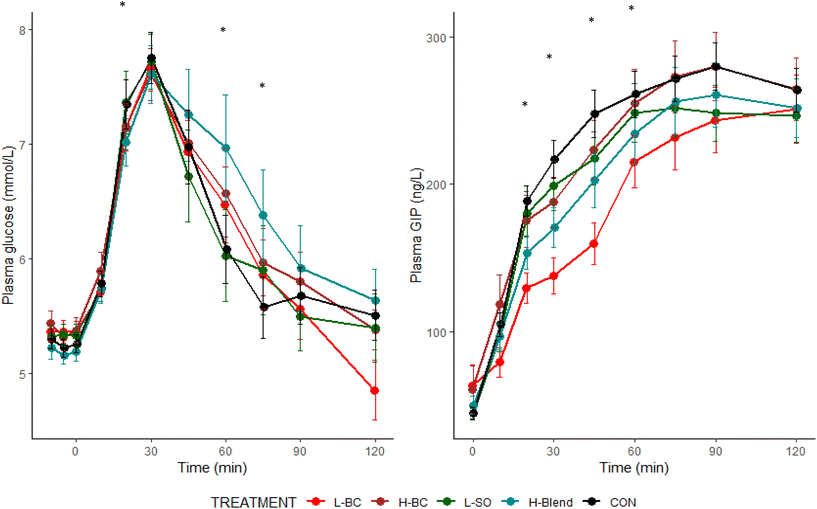 |
| Fig. 2 GLU-FX trial: mean plasma glucose and GIP concentrations by treatment over time after consumption of test drinks, from 0–120 min. CON, 0 mg of blackcurrant (BC) or sweet orange (SO) polyphenols; H-BC, 1600 mg of BC polyphenols; L-BC, 800 mg of BC polyphenols; L-SO, 800 mg of SO polyphenols; H-Blend, 800 mg BC polyphenols + 800 mg SO polyphenols. *Post hoc analysis of time point differences in change from baseline in glucose compared to CON with Dunnett's adjustment: 20 min, P < 0.05 for difference between L-BC and CON; 60 and 75 min, P < 0.01 for difference between H-Blend and CON. Post hoc analysis of time point differences in change from baseline in GIP compared to CON with Dunnett's adjustment: 20, 30 and 45 min, P < 0.001 for difference between L-BC and CON; 60 min, P < 0.01 for difference between L-BC and CON; 30 min, P < 0.01 for difference between H-BC and CON and P < 0.001 for difference between H-Blend and CON; 45 min, P < 0.001 for difference between H-BC and CON and P < 0.01 for difference between H-Blend and CON. Error bars represent standard error. | |
Table 2 GLU-FX study: summary of plasma glucose, serum insulin, serum NEFA and plasma gut hormones incremental AUC 0–30 min and 0–120 min, incremental Cmax and Tmax
|
H-BC (n = 23) |
L-BC (n = 22) |
L-SO (n = 21) |
H-Blend (n = 23) |
CON (n = 30) |
CON, 0 mg of blackcurrant (BC) or sweet orange (SO) polyphenols; H-BC, 1600 mg of BC polyphenols; L-BC, 800 mg of BC polyphenols; L-SO, 800 mg of SO polyphenols; H-Blend, 800 mg BC polyphenols + 800 mg SO polyphenols; iAUC, incremental area under the curve; iCmax, incremental maximum concentration; Tmax, time to maximum concentration. Results expressed as median (interquartile range, IQR). Results expressed as adjusted mean (95% CI) representing the adjusted mean from a linear mixed model with fixed factors for treatment and period, and subject as a random effect, and subject baseline and period baseline as covariates. The iAUC was calculated using the trapezoidal rule and divided by the total duration (30 min) to convert the results back to the original units of measurement (mmol L−1). Significant differences following test drinks compared to CON (placebo) are highlighted in bold (Wilcoxon signed ranks test, P < 0.05, except glucose where Dunnett's tests were conducted). |
iAUC
0–30 min
|
Glucosea (mmol L−1) |
1.22 (1.02, 1.41) |
1.05 (0.85, 1.25)
|
1.19 (0.99, 1.39) |
1.21 (1.01, 1.40) |
1.28 (1.09, 1.46) |
Insulin (mU L−1) |
19.6 (7.8)
|
19.5 (14.1)
|
23.7 (13.7) |
16.9 (13.0) |
25.8 (15.9) |
C-peptide (ng mL−1) |
1.01 (0.70)
|
1.10 (0.79)
|
1.21 (0.91) |
0.98 (0.87) |
1.41 (0.98) |
NEFA (mmol L−1) |
−0.07 (0.18) |
−0.08 (0.19) |
−0.09 (0.11) |
−0.10 (0.23) |
−0.09 (0.18) |
GIP (ng L−1) |
73.1 (39.0)
|
40.5 (29.6)
|
70.6 (53.5) |
64.7 (45.3)
|
94.7 (42.9) |
PYY (ng L−1) |
2.64 (16.5) |
9.25 (19.1) |
4.08 (15.7) |
4.50 (15.9) |
6.13 (15.8) |
GLP-1 (ng L−1) |
2.39 (3.71) |
2.92 (2.05) |
2.74 (2.05) |
2.58 (4.50) |
2.67 (2.08) |
|
iAUC
0–120 min
|
Glucosea (mmol L−1) |
1.08 (0.68, 1.48) |
0.88 (0.48, 1.29) |
0.75 (0.34, 1.36) |
1.36 (0.95, 1.76) |
0.96 (0.59, 1.34) |
Insulin (mU L−1) |
29.5 (21.8) |
30.3 (14.4) |
30.0 (17.7) |
34.2 (22.1) |
34.0 (25.9) |
C-peptide (ng mL−1) |
3.08 (1.56) |
3.24 (1.07) |
3.01 (1.52) |
3.38 (1.85) |
3.45 (2.02) |
NEFA (mmol L−1) |
−0.24 (0.22) |
−0.28 (0.32) |
−0.30 (0.27) |
−0.33 (0.26) |
−0.27 (0.19) |
GIP (ng L−1) |
164.0 (69.0)
|
115.7 (89.3)
|
142.4 (98.0) |
146.5 (112.1)
|
179.8 (106.0) |
PYY (ng L−1) |
3.19 (21.4) |
11.8 (19.8) |
5.09 (14.9) |
6.60 (18.4) |
5.72 (17.0) |
GLP-1 (ng L−1) |
3.26 (2.64) |
2.77 (2.10) |
2.38 (2.81) |
3.26 (2.57) |
2.16 (1.86) |
|
iC
max
|
Glucosea (mmol L−1) |
2.92 (2.48, 3.36) |
2.50 (2.06, 2.95) |
2.70 (2.24, 3.15) |
3.07 (2.63, 3.51) |
2.92 (2.51, 3.33) |
Insulin (mU L−1) |
56.7 (29.5) |
54.8 (33.7) |
56.3 (47.8) |
52.4 (53.0) |
54.7 (34.8) |
C-peptide (ng mL−1) |
4.86 (2.01) |
5.01 (1.86) |
5.44 (2.23) |
5.80 (3.43) |
5.25 (2.83) |
GIPa (ng L−1) |
226.7 (192.3, 261.0) |
209.9 (173.6, 246.2) |
242.2 (210.1, 274.3) |
230.5 (197.5, 263.6) |
253.2 (221.5, 285.0) |
PYY (ng L−1) |
10.1 (24.8) |
21.9 (41.7) |
11.6 (26.1) |
16.67 (31.0) |
15.6 (25.5) |
GLP-1 (ng L−1) |
4.88 (6.57) |
5.93 (4.36) |
4.72 (4.62) |
5.75 (5.79) |
5.32 (3.28) |
|
T
max
(min)
|
Glucose |
30 (15) |
30 (25) |
30 (25) |
30 (15) |
30 (10) |
Insulin |
60 (15) |
60 (45) |
30 (40) |
60 (30) |
45 (45) |
C-peptide |
60 (30) |
75 (37.5) |
90 (52.5) |
75 (30) |
75 (75) |
GIP |
90 (45) |
120 (45) |
90 (60) |
90 (45) |
90 (45) |
PYY |
20 (65) |
45 (58.75) |
30 (47.5) |
30 (40) |
30 (43.75) |
GLP-1 |
20 (35) |
20 (58.75) |
20 (25) |
20 (40) |
20 (10) |
Postprandial insulinaemia, NEFA and gut hormone secretion.
The H-BC and L-BC drinks significantly lowered plasma insulin and C-peptide concentrations (ESI Fig. 3 and 4†) in the early postprandial period compared to CON (Hodges-Lehmann estimator difference H-BC vs. CON (iAUC0–30 min) −7.34 mU L−1 insulin [95% CI −14.8, −0.23] and −0.37 ng ml−1 C-peptide [−0.76, −0.04]; L-BC vs. CON −5.67 mU L−1 insulin [−10.5, −0.76] and −0.29 ng ml−1 C-peptide [−0.50, −0.12]) (Table 2), but there were no significant differences between drinks over the whole postprandial period (iAUC0–120 min). Early postprandial plasma GIP concentrations were reduced by H-BC, L-BC and the H-blend drinks relative to CON (Hodges-Lehmann estimator difference H-BC vs. CON (iAUC0–30 min) −23.6 ng L−1 [-36.9, −5.82]; L-BC vs. CON −49.4 ng L−1 [−73.2, −29.5]; H-blend vs. CON −29.0 ng L−1 [−48.9, −7.29]). Furthermore, 120 min postprandial plasma GIP concentrations (Fig. 2) were also reduced by H-BC, L-BC and the H-blend drinks relative to CON (Hodges-Lehmann estimator difference H-BC vs. placebo (iAUC0–120 min) −33.2 ng L−1 [−55.6, −0.65]; L-BC vs. placebo −52.0 ng L−1 [−90.8, −24.8]; and H-blend vs. placebo −27.9 ng L−1 [−57.1–1.33]). Neither PYY nor GLP-1 responses differed between treatments. No significant differences in changes from baseline in Cmax and Tmax were observed (Table 2). No significant treatment effects were observed for NEFA in any of the outcomes measured. Insulin, C-peptide, GIP, GLP-1, PYY and NEFA responses are shown graphically in ESI Fig. 3–7.†
Appetite and mood scores.
Overall postprandial changes in hunger, desire to eat and fullness did not differ between test drinks compared with placebo (ESI Fig. 8†). There were no differences in postprandial ratings of digestive comfort, nausea, or thirst following test drinks compared with CON. No significant treatment differences were found for postprandial changes in happiness or alertness compared with placebo (data not shown).
Palatability of test drinks.
There were no treatment differences in VAS scores for enjoyment, taste ratings, bitterness, or aftertaste of the test drinks (ESI Fig. 9†). However, the H-blend and L-BC drinks were rated significantly harder to consume (H-blend vs. CON (from log ratio) 220% [112, 434]; and L-BC vs. CON (from log ratio) 238% [115, 492], ESI Fig. 10†). The texture of the L-SO drinks was rated significantly more pleasant than the H-BC, L-BC and H-Blend drinks (L-SO vs. H-BC 19 mm [5, 33]; L-SO vs. L-BC 13 mm [1, 26]; and L-SO vs. H-Blend 16 mm [3, 30], ESI Fig. 10†).
GLU-MIX trial
In the GLU-MIX trial 38 participants were randomised to treatment and 37 completed (Fig. 1b). Participant characteristics were very similar to GLU-FX (Table 1). Two subjects were not fully compliant on CON2 and BC + F drinks (did not consume whole test drink within 2 minutes and high-carbohydrate meal within 7 minutes) and 3 subjects were not fully compliant on the F drink. Results are shown for the intention to treat population.
Postprandial glycaemia.
The BC + F drink significantly lowered plasma glucose concentrations (Fig. 3) in the early postprandial period compared to CON2 and F drinks (mean difference by Dunnett's tests [95% CI] BC + F vs. CON2 (iAUC0–30 min), −0.16 mmol L−1 [−0.27, −0.05]; and BC + F vs. F, −0.21 [−0.32, −0.10]) (Table 3). No significant treatment effects for iAUC0–150 min or iCmax were observed. However, Tmax was significantly longer following the BC + F drink compared to the F drink (Hodges-Lehmann estimator difference [95%CI], 7.50 min [7.50, 15.00]), and shorter following the F drink compared to CON2 (−7.50 min [−12.50, 0.00]). No significant treatment differences in iAUC0–150 min or iCmax were observed.
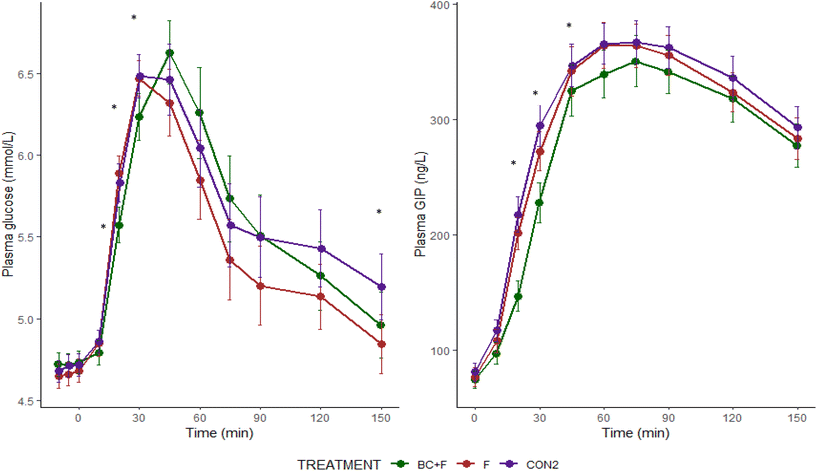 |
| Fig. 3 GLU-MIX trial: mean plasma glucose and GIP concentrations by treatment over time after consumption of test drinks, from 0–150 min. CON2, 0 mg BC polyphenols and 0 g fibre; F, 1.5 g total fibre; BC + F, 800 mg BC polyphenols + 1.5 g total fibre. Dietary fibre for BC + F and F treatments provided by orange pulp. *Post hoc analysis of time point differences in change from baseline in glucose compared to CON2 with Dunnett's adjustment: 10, 20 and 30 min, P < 0.05 for difference between BC + F and F; 20 and 30 min, P < 0.05 for difference between BC + F and CON2; 150 min, P < 0.05 for difference between F and CON2. Post hoc analysis of time point differences in change from baseline in GIP compared to CON2 with Dunnett's adjustment: 10, 20 and 30 min, P < 0.05 for difference between BC + F and CON2; 20 and 30 min, P < 0.05 for difference between BC + F and F; 30 min, P < 0.05 for difference between F and CON2. Error bars represent standard error. | |
Table 3 GLU-MIX study: summary of plasma glucose, serum insulin, serum NEFA and plasma gut hormone incremental AUC 0–30 min and 0–150 min, incremental Cmax and Tmax
|
CON2 (n = 37) |
F (n = 37) |
BC + F (n = 38) |
CON2, 0 mg BC polyphenols and 0 g fibre; F, 1.5 g total fibre; BC + F, 800 mg BC polyphenols + 1.5 g total fibre; NA, not analysed. Dietary fibre for BC + F and F treatments provided by orange pulp; iAUC, incremental area under the curve; iCmax, incremental maximum concentration; Tmax, time to maximum concentration. Results expressed as median (interquartile range, IQR). Results expressed as adjusted mean (95% CI) representing the adjusted mean from a linear mixed model with fixed factors for treatment and period, and subject as a random effect, and subject baseline and period baseline as covariates. The iAUC was calculated using the trapezoidal rule and divided by the total duration (30 min) to convert the results back to the original units of measurement (mmol L−1). Significant differences of treatment from placebo are highlighted in bold (Wilcoxon signed ranks test, P < 0.05) and # indicates a significant difference between BC + F and F. |
iAUC
0–30 min
|
Glucosea (mmol L−1) |
0.73 (0.62, 0.84) |
0.78 (0.66, 0.89) |
0.57 (0.45, 0.68)
#
|
Insulin (mmol L−1) |
14.0 (9.4) |
16.8 (14.2) |
12.5 (11.1)
#
|
C-peptide (ng mL−1) |
0.68 (0.54) |
0.79 (0.55) |
0.64 (0.42)# |
GIP (ng L−1) |
85.0 (51.4) |
76.6 (65.1)
|
43.6 (55.1)
#
|
|
iAUC
0–150 min
|
Glucosea (mmol L−1) |
0.95 (0.67, 1.23) |
0.83 (0.54, 1.11) |
0.90 (0.62, 1.19) |
Insulin (mmol L−1) |
27.8 (15.9) |
31.5 (24.3) |
26.5 (15.5) |
C-peptide (ng mL−1) |
2.21 (0.91) |
2.25 (1.37) |
2.33 (0.84) |
GIP (ng L−1) |
202.1 (84) |
210 (115) |
186.5 (92.2)
|
|
iC
max
|
Glucosea |
2.27 (1.95, 2.58) |
2.28 (1.97, 2.60) |
2.32 (2.00, 2.64) |
Insulin |
51.7 (36.2) |
58.4 (37.7) |
54.0 (33.7) |
C-peptide |
3.47 (1.60) |
3.56 (1.75) |
3.61 (1.75) |
GIP |
293 (116) |
314 (156) |
257 (170) |
|
T
max
|
Glucose |
45 (15) |
30 (15)
|
45 (30)# |
Insulin |
60 (30) |
60 (30) |
60 (30) |
C-peptide |
75 (60) |
75 (60) |
75 (30) |
GIP |
75 (30) |
75 (38) |
75 (30) |
Postprandial insulinaemia, C-peptide and GIP secretion.
The BC + F drink significantly lowered insulin concentrations in the early postprandial period (iAUC0–30 min) compared to CON2 and the F drink (Hodges-Lehmann estimator difference [95% CI] BC + F vs. CON2 (iAUC0–30 min), −2.67 mU L−1 [−0.27, −0.05]; and BC + F vs. F, −0.21 mU L−1 [−0.32, −0.10]) (Table 3). The BC + F drink also significantly lowered C-peptide concentrations during the first 30 min compared to the F drink (BC + F vs. F, −0.22 ng ml−1 [−0.33, −0.12]), but not compared with CON2 (Table 3). Neither insulin nor C-peptide were significantly affected over the whole 150 min postprandial period by treatment (ESI Fig. 11 and 12†). Both the BC + F and F drinks significantly attenuated the increase in GIP during the first 30 min of the postprandial period relative to CON2 (Table 3), and the BC + F drink reduced GIP concentrations relative to the F drink (Hodges-Lehmann estimator difference [95% CI] BC + F vs. CON2 (iAUC0–30 min) −36.3 ng L−1 [−49.2, −23.2]; F vs. CON2–11.2 ng L−1 [−22.3, −0.0]; and BC + F vs. F −29.1 ng L−1 [−41.7, −15.5]). The BC + F drink also significantly reduced GIP concentrations during the 150 min of the postprandial period relative to CON2 and the F drink (Hodges-Lehmann estimator difference [95% CI] BC + F vs. CON2 (iAUC0–150 min) −28.6 ng L−1 [−48.0, −6.2]; and BC + F vs. F −21.1 ng L−1 [−41.3, −1.9]), however this effect was not observed for drink F compared to CON2 (Fig. 3, Table 3). No significant differences in iCmax and Tmax were observed.
Cognitive function.
Results are shown in ESI Table 3.† The BC + F drink significantly reduced the amount of time to respond to the four choice reaction time task relative to CON2 (Hodges-Lehmann estimator difference [95% CI] in msec for overall reaction time [95% CI] −41.9 [−97.2, −4.6] and correct responses reaction time −40.9 [−96.6, −2.0]). There were significantly fewer errors in the serial subtraction of 3's, a measure of working memory, relative to CON2 and to the F drink (Hodges-Lehmann estimator difference [95% CI] −1.0 [−2.0, 0.0] and −1.0 [−2.0, 0.0], respectively). There were no statistically significant treatment effects on performance parameters (accuracy, reaction time) during the Stroop test, nor on the choice reaction time, RVIP or simple reaction time tests.
Ad libitum energy intake, appetite and mood scores.
There were no significant treatment effects on ad libitum energy intake (ESI Table 4†), nor were there any significant differences in postprandial changes in hunger, desire to eat, or fullness between treatments (ESI Fig. 13†). There were no significant differences in self-rated enjoyment, taste, smell, palatability, texture, aftertaste or feelings of fullness after consuming the buffet-style ad libitum meal between treatments (data not shown). Participants reported feeing significantly thirstier following the BC + F drink compared to both the F drink and CON2 (BC + F vs. F 4 mm [1, 8]; and BC + F vs. CON2 5 mm [1, 9]). There were no differences between test drinks and CON2 in postprandial ratings of digestive comfort (data not shown). Postprandially, happiness increased slightly more following the F drink compared to CON2 (F vs. placebo 4 mm [2, 7]), but there was no significant difference between BC + F and CON2. Postprandial alertness and feeling energised significantly increased following both test drinks compared to CON2 (alertness BC + F vs. CON2 4 mm [1, 6]; alertness F vs. CON2 3 mm [1, 6]; feeling energised BC + F vs. CON2 3 mm [0.3, 6]; and feeling energised F vs. CON2 4 mm [1, 7]; ESI Fig. 14†).
Palatability of test drinks.
The BC + F and F drinks were significantly rated harder to consume than CON2 (BC + F vs. CON2 11 mm [0.4, 21]; and F vs. CON2 12 [1, 22]). The BC + F drink was significantly rated more bitter than CON2 (11 mm [1, 22]) and the texture of the test drinks were significantly rated less pleasant than CON2 (BC + F vs. CON2 −11 mm [−22, −1]; F vs. CON2 −16 mm [−27, −5], ESI Fig. 15†). There were no treatment differences in VAS scores for enjoyment, taste ratings, or aftertaste of the test drinks (ESI Fig. 16†).
Discussion
In the present study, we aimed to compare the effects of combining (poly)phenol-rich fruit extracts and dietary fibre on glycaemia, insulinaemia, gut hormones, appetite, mood and cognitive function. The primary focus was on the increase in plasma glucose from 0–30 min in order to find an optimal combination of extracts that could be incorporated in a drink for the purpose of slowing down intestinal glucose absorption from meals containing readily available carbohydrate. Slowing the rate of absorption would be expected to avoid large excursions in blood glucose, which is important, particularly in pre-diabetics, because this would ameliorate glucotoxic effects on vascular tissue and pancreatic beta cells27,28 associated with cardiovascular risk factors such as endothelial dysfunction and oxidative stress.27–29 Retarding the rate of glucose absorption could also possibly prevent subsequent pronounced glucose nadirs, which could increase hunger and acutely impair cognitive function.29 Previous studies demonstrated that BC extracts, rich in anthocyanins and proanthocyanidins, inhibited the early phase of postprandial glycaemia,13,30 an effect that was amplified when combined with apple extracts rich in phlorizin, quercetin, proanthocyanidins and chlorogenic acid.31 Fruit (poly)phenols have a range of effects on inhibition of glucose transporters and carbohydrases (e.g., α-amylase),14,31,32 suggesting that additive effects might occur when combining different fruit extracts in a drink before ingestion of a mixed meal containing sucrose and starch. The same principle could be applied to combining fruit (poly)phenol extracts with fruit pulp preparations rich in water-soluble dietary fibre (‘soluble fibre’), such as pectic substances that inhibit starch digestion.33–35 The study findings showed that combining BC and SO extracts, or BC and citrus fibre, did not have a greater inhibitory effect on early postprandial glycaemia than BC extracts alone, explained by the negligible impact of either SO extract or citrus fibre on early postprandial glycaemia.
The GLU-FX study hypothesised that a combination of BC and SO extracts would have a greater effect on early postprandial glycaemia than either extract alone. The lower dose of BC extract, approximately equivalent to 100 g fresh raw blackcurrants,36 significantly attenuated the rate of increase in plasma glucose in the first 30 min postprandially but the difference was small. It is important to note that maximum glucose concentrations were not different between the lower BC (poly)phenol drink (or any of the other test drinks) and placebo, and there were no differences in glucose concentrations between the low BC (poly)phenol drink and placebo after 30 min. This result suggests that the delay in the rate of glucose absorption, indicated by the reduction in the early postprandial GIP response, did not affect overall postprandial glycaemia, as measured in the peripheral circulation (venous blood).
The SO extract alone did not show any significant effect relative to placebo suggesting that adding hesperidin-rich extracts to drinks would not reduce postprandial glycaemia. At the time of designing the GLU-FX trial, there were no human trials investigating the effects of hesperidin on postprandial glycaemia. Subsequently, the in vitro and in vivo effects of orange juice, standardised for hesperidin content, were reported on glucose transport in cells, sucrase activity in rats and glycaemic response to orange juice in human participants.37 It was shown that hesperidin inhibited glucose transport via inhibition of GLUT2 but that there was no effect on sucrase activity, and doses of around 300–600 mg total hesperidin (naturally present and additional) in 200 mL orange juice lowered peak glucose concentrations in healthy men and women in 2 separate crossover studies (n = 10).37 These studies were small and not randomised, and therefore additional randomised controlled trials are required to strengthen the evidence. Furthermore, the study design of Kerimi et al. was not directly comparable as no starch was consumed37 unlike the current study, which provided a realistic mixed-carbohydrate solid meal. It is likely that hesperidin naturally present (partially or wholly) in orange juice has different physiological properties in the intestinal lumen compared to a purified orange extract containing 90% hesperidin that is dispersed in a drink. Future studies investigating the effects of hesperidin in beverages on the glycaemic response should consider the solubility of the extract in different drinks (e.g. juice, water), their properties in the gut, such as changes in pH, and the impact of processing and composition of the test meal.
Despite the attenuating effect of the 800 mg BC (poly)phenol drink on early postprandial glycaemia and the corresponding GIP response, the drink containing both BC (poly)phenols and hesperidin did not significantly modify blood glucose concentrations in the first 30 min. Interestingly, the BC and SO drink led to significantly higher glucose responses at 60 and 75 min. These findings suggest that hesperidin-rich extracts might cancel out the gluco-inhibitory effects of blackcurrant (poly)phenols.
Unexpectedly, the higher dose of BC extract failed to show a significant lowering effect on blood glucose during the early postprandial period. The randomisation to treatments was such that all subjects had the control treatment but not all had the high and low doses of BC (n = 16 had both) and therefore inter-individual differences in responses to (poly)phenols may have been a factor. In addition, there may be other potential mechanisms such as interaction with the food matrix at high doses meaning that the inhibitory effect on digestive enzymes/glucose transporters plateaued at 800 mg total (poly)phenols.
GIP is a gut hormone that is secreted in the proximal section of the small intestine by K-cells just 5–10 minutes after carbohydrate (and fat) intake.38 Intestinal sodium-dependent glucose transporter SGLT1 is co-localised with and physiologically related to the expression of GIP, and therefore GIP secretion is tightly coupled to the rate of glucose absorption in the intestine24 and can be regarded as a more sensitive marker of glucose absorption than plasma glucose concentrations (which represent the sum effect of glucose appearance and disposal). Interaction with carbohydrases and SGLT1/GLUT2 are key mechanisms by which BC (poly)phenol extracts may modify the rate of intestinal glucose absorption.14,31 Therefore, the lowering of circulating postprandial GIP concentrations, following both the low and high dose BC extracts, reinforces existing evidence that BC (poly)phenols interact with glucose transporters, even if this did not result in lower peak postprandial glucose concentrations. The high dose blend extract showed a significant attenuation of the GIP response (albeit less than that of L-BC), indicating that there may have been a reduced rate of intestinal glucose absorption following this dose that was not detected by measuring circulating glucose concentrations. None of the drinks had an inhibitory effect on the satiety gut hormones, PYY and GLP-1, and consistent with this there were no differences in postprandial subjective scores for hunger, desire to eat or fullness, thus providing no evidence that there might be any modulatory effect of BC or SO (poly)phenols on short-term appetite regulation following a high-carbohydrate meal.
The GLU-MIX study aimed to investigate the effects of fruit (poly)phenol extracts combined with a fibre-rich orange pulp, on postprandial outcomes following a mixed carbohydrate (starch and sucrose) test meal. The BC (poly)phenol extract in combination with orange pulp significantly reduced the rate of increase in plasma glucose, as well as insulin and GIP concentrations, during the early postprandial period (0–30 min). However, pulp alone failed to elicit a blood glucose-lowering effect in the early postprandial phase suggesting that the lower glycaemia of BC + F was likely to be mainly attributable to the BC extract component. The fact that fibre was provided as pulp meant that it likely remained intact within cell wall structures. This may explain the lack of effect since pectic material would not have been released from the particles of pulp during digestion to dissolve in the water phase of digesta, resulting in negligible change in viscosity and interactions with carbohydrases and starch.18
Increased consumption of (poly)phenol-rich foods has been associated with reduced cognitive decline in prospective cohort studies,39,40 and interventional evidence in healthy individuals has linked acute flavonoid intake to improvements in cognitive function.22,41 Potential mechanisms are likely to be a host of contributors interacting to influence the outcome, including acutely increased cerebral blood flow, greater gut microbiota diversity and interactions with the gut-brain axis following (poly)phenol consumption.20 The BC + F significantly increased attention (measured as response speed to the four reaction choice task) and working memory (measured as reduced number of errors in the Serial of 3's subtraction test) capacity in comparison to the placebo drink when measured 165 min following consumption of the test drinks. Watson et al. (2015) showed improved attention (using RVIP) in a cross-over RCT with two blackcurrant drinks (one made with BC extract and the other was a cold-pressed juice – total (poly)phenols 525 ± 5 mg/60 kg bodyweight) compared to an energy- and sugar-matched control drink.46 Watson et al. (2019) showed in another cross-over RCT with healthy young adults (mean age 23 years), a significant increase in reaction time (using choice reaction time) by 19.8 s following consumption of a blackcurrant juice (250 ml, 500 mg total PP) compared to placebo.47 Although the significances found in the GLU-MIX study were not found in the same tests used by both Watson et al. papers,46,47 these measured the same cognitive domains. In the GLU-MIX study, cognitive function was a secondary outcome, and the trial was not statistically powered to detect changes. Although these results are promising, the risk of type I errors due to multiple testing should be taken into consideration. Future studies designed to detect differences in performance of cognitive tests as a primary outcome are needed to confirm and further elucidate the impact of BC (poly)phenols in acute cognition. Although the fibre-rich orange pulp did not acutely impact cognitive function nor postprandial metabolic health, there may be chronic effects of metabolites arising from colonic fermentation on central nervous system function as well as other potential health benefits not measured in this study, such as colonic and cardiovascular health, which should also be considered.
Strengths and limitations
Strengths of this study include the randomised and double-blinded trial designs and design of a placebo drink equivalent in taste, appearance, energy and nutrient composition. Nevertheless, there is a chance that the drinks containing pulp were perceived as different to the placebo (CON2) in the GLU-MIX study by participants, due to unavoidable differences in texture. Our approach of delivering 75 g carbohydrate as a starch and sucrose solid test meal is representative of a realistic meal and enabled the potentially additive effects of test components to be fully evaluated via different gluco-regulatory mechanisms. However, the highly available-carbohydrate meals contained only minimal amounts of protein and fat, and other macronutrients could modify the impact of fruit (poly)phenols on postprandial glycaemia. (Poly)phenol-rich extracts were added to the test drinks to avoid confounding influences of other fruit components in whole fruit purees or juices, and to use the waste streams (pomace) that would be adopted by industry should the research result in development of a new functional beverage. However, this approach is limited by constraints on drink formulation due to palatability, texture and physical properties. The sample population included both men and women across a broad age range, and results are therefore applicable to a general healthy population. However, the inclusion of healthy individuals, including highly insulin-sensitive pre-menopausal women, could also be considered a weakness in the study design, since rapid clearance of circulating glucose leads to a smaller likelihood of detecting differences in glycaemic responses arising from delayed intestinal absorption. Using stable isotopes to label carbohydrates in test meals would be the optimal methodological approach to investigating effects of fruit (poly)phenols/fibre on glucose absorption.
In retrospect, the use of an incomplete block study design was a limitation when comparing treatments due to high inter-individual variability. Furthermore, serum concentrations of postprandial circulating polyphenol metabolites were not analysed in this study since we were primarily focused on intra-lumenal intestinal mechanisms. Hypothesised treatment effects on the primary outcome (iAUC0–30 min) were expected to be primarily determined by (poly)phenol interactions with digestive enzymes and intestinal glucose transporters, not by blood (polyphenol)-induced effects on glucose disposal or insulin secretion, which would predominantly occur after 30 min post-ingestion. Nevertheless, interpretation of some of the secondary outcome variables (mood, cognitive function, appetite) would have benefited from data on circulating phenolic metabolites. Finally, orange pulp was added as a source of fibre in amounts that would be acceptable to the consumer. However, this provided a relatively low amount of total fibre (1.5 g) and a very low amount of pectin (232 mg as galacturonic acid equivalents). Pectin was previously reported to lower postprandial glycaemia when incorporated in a drink at doses ranging from 10–30 g (thereby increasing viscosity),42,43 but not at lower doses.44,45
Conclusions
Together with previous findings where only the highest dose of blackcurrant extract reduced glucose concentrations 0–30 min (1596 mg total (poly)phenols, but only 599 mg anthocyanins), the current results suggest there is no linear relationship between dose of blackcurrant (poly)phenols and early postprandial glycaemia beyond ∼600 mg anthocyanins, as demonstrated by the lack of effect of the high dose (>1000 mg anthocyanins) compared to the lower dose (578 mg anthocyanins). However, this result does not translate into reduced blood glucose peaks, which suggests there may be no impact on metabolic health in healthy, insulin-sensitive individuals. Furthermore, inclusion of a sweet orange extract, mostly comprising hesperidin, or fibre-rich orange pulp in the test meals did not enhance the inhibitory effects of blackcurrant extracts on the early rise in blood glucose, and therefore these specific citrus ingredients at the concentrations used are unlikely to support regulation of glucose levels following carbohydrate-containing meals if included in a low-sugar beverage. For translation to clinical practice, future research in this area should determine whether drinks formulated to be rich in berry anthocyanins and proanthocyanidins can lower total postprandial glycaemia in populations with impaired glucose tolerance/prediabetes.
Author contributions
MH was involved in the design of GLU-FX, conducted the clinical trial, and collected the data. AMP was involved in the design of GLU-MIX, conducted the clinical trial, collected the data, and co-wrote the initial manuscript draft, and reviewed and edited the manuscript. KB was involved in the design of both studies and was responsible for project administration. JAF acquired funding and had oversight of test material provision and project administration, and reviewed and edited the manuscript. DT carried out data curation and formal statistical analysis. YT co-wrote the initial manuscript draft. CPC and PE were involved in the interpretation of data and reviewed and edited the manuscript. MM, KS, SB, and CH performed the clinical trial research, collected, and analysed data. XN conducted pectin analysis. WLH conceptualised both studies, acquired funding, supervised research activity planning and execution, designed the trials, participated in the interpretation of data, and co-wrote the initial manuscript draft, and reviewed and edited subsequent versions. All authors read and approved the final draft.
Conflicts of interest
WLH, AMP, MRH, CPC, PRE, YT, KS, SB, CH, MM, XN declared they had no conflicts of interest.
JAF is an employee of Lucozade Ribena Suntory Ltd. KB was previously employed by Lucozade Ribena Suntory Ltd.
DT was paid as an independent statistical consultant by Lucozade Ribena Suntory Ltd.
Acknowledgements
This research was funded by Innovate UK and Lucozade Ribena Suntory Ltd. We would like to thank Anna Wittekind, Claire Lawrence and Dan Midgley for their assistance with study data monitoring. We thank the volunteers for taking the time to participate in this study. We also thank Tim Wingham for medical supervision and phlebotomy, Anne-Catherine Perz for her practical help, Tracy Neal at Affinity Biomarker Laboratories for analysis of blood samples, and Balazs Bajka for his advice on dietary fibre and pectin analysis.
References
- WHO, Factsheets – Diabetes, https://www.who.int/news-room/fact-sheets/detail/diabetes, (accessed 12/05/2023).
- C. E. Eades, E. F. France and J. M. Evans, Prevalence of impaired glucose regulation in Europe: a meta-analysis, Eur. J. Public Health, 2016, 26, 699–706 CrossRef PubMed.
- J. Beulens, F. Rutters, L. Rydén, O. Schnell, L. Mellbin, H. Hart and R. Vos, Risk and management of pre-diabetes, Eur. J. Prev. Cardiol., 2020, 26, 47–54 CrossRef PubMed.
- L. Monnier, E. Mas, C. Ginet, F. Michel, L. Villon, J. P. Cristol and C. Colette, Activation of oxidative stress by acute glucose fluctuations compared with sustained chronic hyperglycemia in patients with type 2 diabetes, J. Am. Med. Assoc., 2006, 295, 1681–1687 CrossRef CAS.
- H. S. Jung, Clinical Implications of Glucose Variability: Chronic Complications of Diabetes, Endocrinol. Metab., 2015, 30, 167–174 CrossRef CAS PubMed.
- E. Bonora, Postprandial peaks as a risk factor for cardiovascular disease: epidemiological perspectives, Int. J. Clin. Pract., Suppl., 2002, 5–11 Search PubMed.
- A. Ceriello, K. Esposito, L. Piconi, M. A. Ihnat, J. E. Thorpe, R. Testa, M. Boemi and D. Giugliano, Oscillating glucose is more deleterious to endothelial function and oxidative stress than mean glucose in normal and type 2 diabetic patients, Diabetes, 2008, 57, 1349–1354 CrossRef CAS.
- J. Rienks, J. Barbaresko, K. Oluwagbemigun, M. Schmid and U. Nothlings, Polyphenol exposure and risk of type 2 diabetes: dose-response meta-analyses and systematic review of prospective cohort studies, Am. J. Clin. Nutr., 2018, 108, 49–61 CrossRef PubMed.
- E. J. Prpa, C. P. Corpe, B. Atkinson, B. Blackstone, E. S. Leftley, P. Parekh, M. Philo, P. A. Kroon and W. L. Hall, Apple polyphenol-rich drinks dose-dependently decrease early-phase postprandial glucose concentrations following a high-carbohydrate meal: a randomized controlled trial in healthy adults and in vitro studies, J. Nutr. Biochem., 2020, 85, 108466 CrossRef CAS PubMed.
- L. Bell, D. J. Lamport, L. T. Butler and C. M. Williams, A study of glycaemic effects following acute anthocyanin-rich blueberry supplementation in healthy young adults, Food Funct., 2017, 8, 3104–3110 RSC.
- P. J. Curtis, L. Berends, V. van der Velpen, A. Jennings, L. Haag, P. Chandra, C. D. Kay, E. B. Rimm and A. Cassidy, Blueberry anthocyanin intake attenuates the postprandial cardiometabolic effect of an energy-dense food challenge: Results from a double blind, randomized controlled trial in metabolic syndrome participants, Clin. Nutr., 2022, 41, 165–176 CrossRef CAS.
- G. Williamson, Effects of Polyphenols on Glucose-Induced Metabolic Changes in Healthy Human Subjects and on Glucose Transporters, Mol. Nutr. Food Res., 2022, 66, e2101113 CrossRef PubMed.
- M. L. Castro-Acosta, L. Smith, R. J. Miller, D. I. McCarthy, J. A. Farrimond and W. L. Hall, Drinks containing anthocyanin-rich blackcurrant extract decrease postprandial blood glucose, insulin and incretin concentrations, J. Nutr. Biochem., 2016, 38, 154–161 CrossRef CAS PubMed.
- E. J. Prpa, B. H. Bajka, P. R. Ellis, P. J. Butterworth, C. P. Corpe and W. L. Hall, A systematic review of in vitro studies evaluating the inhibitory effects of polyphenol-rich fruit extracts on carbohydrate digestive enzymes activity: a focus on culinary fruits consumed in Europe, Crit. Rev. Food Sci. Nutr., 2021, 61, 3783–3803 CrossRef CAS PubMed.
- W. Shen, Y. Xu and Y. H. Lu, Inhibitory effects of Citrus flavonoids on starch digestion and antihyperglycemic effects in HepG2 cells, J. Agric. Food Chem., 2012, 60, 9609–9619 CrossRef CAS PubMed.
- C. Di Lorenzo, C. M. Williams, F. Hajnal and J. E. Valenzuela, Pectin delays gastric emptying and increases satiety in obese subjects, Gastroenterology, 1988, 95, 1211–1215 CrossRef CAS PubMed.
- T. Morita, H. Tanabe, H. Ito, K. Sugiyama and S. Kiriyama, Long-term ingestion of insoluble dietary fiber increases luminal mucin content, but has no effect on nutrient absorption in rats, Biosci., Biotechnol., Biochem., 2008, 72, 767–772 CrossRef CAS PubMed.
- M. M. Grundy, C. H. Edwards, A. R. Mackie, M. J. Gidley, P. J. Butterworth and P. R. Ellis, Re-evaluation of the mechanisms of dietary fibre and implications for macronutrient bioaccessibility, digestion and postprandial metabolism, Br. J. Nutr., 2016, 116, 816–833 CrossRef CAS PubMed.
- S. Satchithanandam, M. Vargofcak-Apker, R. J. Calvert, A. R. Leeds and M. M. Cassidy, Alteration of gastrointestinal mucin by fiber feeding in rats, J. Nutr., 1990, 120, 1179–1184 CrossRef CAS PubMed.
- D. J. Lamport and C. M. Williams, Polyphenols and Cognition In Humans: An Overview of Current Evidence from Recent Systematic Reviews and Meta-Analyses, Brain Plast., 2021, 6, 139–153 Search PubMed.
- P. A. Jackson, E. L. Wightman, R. Veasey, J. Forster, J. Khan, C. Saunders, S. Mitchell, C. F. Haskell-Ramsay and D. O. Kennedy, A Randomized, Crossover Study of the Acute Cognitive and Cerebral Blood Flow Effects of Phenolic, Nitrate and Botanical Beverages in Young, Healthy Humans, Nutrients, 2020, 12(8), 2254 CrossRef CAS PubMed.
- L. Bell, D. J. Lamport, L. T. Butler and C. M. Williams, A Review of the Cognitive Effects Observed in Humans Following Acute Supplementation with Flavonoids, and Their Associated Mechanisms of Action, Nutrients, 2015, 7, 10290–10306 CrossRef CAS PubMed.
- J. Lee, R. W. Durst and R. E. Wrolstad, Determination of total monomeric anthocyanin pigment content of fruit juices, beverages, natural colorants, and wines by the pH differential method: collaborative study, J. AOAC Int., 2005, 88, 1269–1278 CrossRef CAS.
- V. Gorboulev, A. Schurmann, V. Vallon, H. Kipp, A. Jaschke, D. Klessen, A. Friedrich, S. Scherneck, T. Rieg, R. Cunard, M. Veyhl-Wichmann, A. Srinivasan, D. Balen, D. Breljak, R. Rexhepaj, H. E. Parker, F. M. Gribble, F. Reimann, F. Lang, S. Wiese, I. Sabolic, M. Sendtner and H. Koepsell, Na(+)-D-glucose cotransporter SGLT1 is pivotal for intestinal glucose absorption and glucose-dependent incretin secretion, Diabetes, 2012, 61, 187–196 CrossRef CAS PubMed.
- J. Lara, M. A. Taylor and I. A. Macdonald, Is ad libitum energy intake in overweight subjects reproducible in laboratory studies using the preload paradigm?, Eur. J. Clin. Nutr., 2010, 64, 1028–1031 CrossRef CAS PubMed.
- M. R. Hobden, L. Guerin-Deremaux, D. M. Commane, I. Rowland, G. R. Gibson and O. B. Kennedy, A pilot investigation to optimise methods for a future satiety preload study, Pilot Feasibility Stud., 2017, 3, 61 CrossRef PubMed.
- E. E. Blaak, J. M. Antoine, D. Benton, I. Bjorck, L. Bozzetto, F. Brouns, M. Diamant, L. Dye, T. Hulshof, J. J. Holst, D. J. Lamport, M. Laville, C. L. Lawton, A. Meheust, A. Nilson, S. Normand, A. A. Rivellese, S. Theis, S. S. Torekov and S. Vinoy, Impact of postprandial glycaemia on health and prevention of disease, Obes. Rev., 2012, 13, 923–984 CrossRef CAS PubMed.
- J. Loader, D. Montero, C. Lorenzen, R. Watts, C. Meziat, C. Reboul, S. Stewart and G. Walther, Acute Hyperglycemia Impairs Vascular Function in Healthy and Cardiometabolic Diseased Subjects: Systematic Review and Meta-Analysis, Arterioscler., Thromb., Vasc. Biol., 2015, 35, 2060–2072 CrossRef CAS PubMed.
- C. M. Gaylor, D. Benton, A. Brennan and H. A. Young, The impact of glycaemic load on cognitive performance: A meta-analysis and guiding principles for future research, Neurosci. Biobehav. Rev., 2022, 141, 104824 CrossRef CAS PubMed.
- S. Coe and L. Ryan, Impact of polyphenol-rich sources on acute postprandial glycaemia: a systematic review, J. Nutr. Sci., 2016, 5, e24 CrossRef CAS PubMed.
- M. L. Castro-Acosta, S. G. Stone, J. E. Mok, R. K. Mhajan, C. I. Fu, G. N. Lenihan-Geels, C. P. Corpe and W. L. Hall, Apple and blackcurrant polyphenol-rich drinks decrease postprandial glucose, insulin and incretin response to a high-carbohydrate meal in healthy men and women, J. Nutr. Biochem., 2017, 49, 53–62 CrossRef CAS PubMed.
- C. Schulze, A. Bangert, G. Kottra, K. E. Geillinger, B. Schwanck, H. Vollert, W. Blaschek and H. Daniel, Inhibition of the intestinal sodium-coupled glucose transporter 1 (SGLT1) by extracts and polyphenols from apple reduces postprandial blood glucose levels in mice and humans, Mol. Nutr. Food Res., 2014, 58, 1795–1808 CrossRef CAS PubMed.
- T. Sasaki, I. Sotome and H. Okadome, In vitro starch digestibility and in vivo glucose response of gelatinized potato starch in the presence of non-starch polysaccharides, Starch/Staerke, 2015, 67, 415–423 CrossRef CAS.
- Y. Bai and R. G. Gilbert, Mechanistic Understanding of the Effects of Pectin on In Vivo Starch Digestion: A Review, Nutrients, 2022, 14(23), 5107 CrossRef CAS PubMed.
- Y. S. Ma, Y. Pan, Q. T. Xie, X. M. Li, B. Zhang and H. Q. Chen, Evaluation studies on effects of pectin with different concentrations on the pasting, rheological and digestibility properties of corn starch, Food Chem., 2019, 274, 319–323 CrossRef CAS PubMed.
- J. A. Rothwell, J. Perez-Jimenez, V. Neveu, A. Medina-Remon, N. M'Hiri, P. Garcia-Lobato, C. Manach, C. Knox, R. Eisner, D. S. Wishart and A. Scalbert, Phenol-Explorer 3.0: a major update of the Phenol-Explorer database to incorporate data on the effects of food processing on polyphenol content, Database, 2013, 2013, bat070 CrossRef PubMed.
- A. Kerimi, J. S. Gauer, S. Crabbe, J. W. Cheah, J. Lau, R. Walsh, P. F. Cancalon and G. Williamson, Effect of the flavonoid hesperidin on glucose and fructose transport, sucrase activity and glycaemic response to orange juice in a crossover trial on healthy volunteers, Br. J. Nutr., 2019, 121, 782–792 CrossRef CAS PubMed.
- W. Alsalim, O. Lindgren and B. Ahren, Glucose-dependent insulinotropic polypeptide and glucagon-like peptide-1 secretion in humans: Characteristics and regulation, J. Diabetes Invest., 2023, 14, 354–361 CrossRef CAS.
- E. E. Devore, J. H. Kang, M. M. Breteler and F. Grodstein, Dietary intakes of berries and flavonoids in relation to cognitive decline, Ann. Neurol., 2012, 72, 135–143 CrossRef CAS PubMed.
- L. Letenneur, C. Proust-Lima, A. Le Gouge, J. F. Dartigues and P. Barberger-Gateau, Flavonoid intake and cognitive decline over a 10-year period, Am. J. Epidemiol., 2007, 165, 1364–1371 CrossRef CAS PubMed.
- P. Hepsomali, A. Greyling, A. Scholey and D. Vauzour, Acute Effects of Polyphenols on Human Attentional Processes: A Systematic Review and Meta-Analysis, Front. Neurosci., 2021, 15, 678769 CrossRef PubMed.
- D. J. Jenkins, M. A. Gassull, A. R. Leeds, G. Metz, J. B. Dilawari, B. Slavin and L. M. Blendis, Effect of dietary fiber on complications of gastric surgery: prevention of postprandial hypoglycemia by pectin, Gastroenterology, 1977, 73, 215–217 CrossRef CAS.
- S. Holt, R. C. Heading, D. C. Carter, L. F. Prescott and P. Tothill, Effect of gel fibre on gastric emptying and absorption of glucose and paracetamol, Lancet, 1979, 1, 636–639 CrossRef CAS PubMed.
- M. Sanaka, T. Yamamoto, H. Anjiki, K. Nagasawa and Y. Kuyama, Effects of agar and pectin on gastric emptying and post-prandial glycaemic profiles in healthy human volunteers, Clin. Exp. Pharmacol. Physiol., 2007, 34, 1151–1155 CrossRef CAS PubMed.
- S. Y. Iftikhar, N. Washington, C.
G. Wilson, I. A. Macdonald and M. D. Homer-Ward, The effect of pectin on the gastric emptying rates and blood glucose levels after a test meal, J. Pharm. Pharmacol., 1994, 46, 851–853 CrossRef CAS PubMed.
- A. W. Watson, C. F. Haskell-Ramsay, D. O. Kennedy, J. M. Cooney, T. Trower and A. Scheepens, Acute supplementation with blackcurrant extracts modulates cognitive functioning and inhibits monoamine oxidase-B in healthy young adults, J. Funct. Foods, 2015, 17, 524–539 CrossRef CAS.
- A. W. Watson, E. J. Okello, H. J. Brooker, S. Lester, G. J. McDougall and K. A. Wesnes, The impact of blackcurrant juice on attention, mood and brain wave spectral activity in young healthy volunteers, Nutr. Neurosci., 2019, 22, 596–606 CrossRef CAS PubMed.
|
This journal is © The Royal Society of Chemistry 2023 |