DOI:
10.1039/D3FO01737K
(Paper)
Food Funct., 2023,
14, 7562-7573
Cocoa flavanols improve peakVO2 and exercise capacity in a randomized double blinded clinical trial in healthy elderly people†
Received
28th April 2023
, Accepted 17th July 2023
First published on 25th July 2023
Abstract
Background: Loss of functional capacity is one of the hallmarks in cardiovascular aging. Cocoa flavanols (CF) exert favorable effects on endothelial function, blood pressure, and inflammation. These cardiovascular health markers worsen with increasing age and limit functional exercise capacity. Aim: To investigate the effect of CF on cardiorespiratory-fitness in healthy elderly people. Methods: In a randomized, double-masked, placebo-controlled, parallel-group dietary intervention trial, 68 healthy elderly people (55–79 years, 28 female) received either 500 mg of CF or a nutrient-matched control capsule twice a day for 30 days. Primary endpoint was defined as peak oxygen consumption (VO2) in a cardiopulmonary exercise test (CPET). Secondary endpoints were oxygen pulse (VO2 per heart rate (HR)), resting blood pressure (BP), and resting vascular function. Results: After 30 days of CF intake peakVO2 increased by 190 ml min−1 (95% CI 1–371 ml min−1) and peakVO2 per kg by 2.5 ml (min kg)−1 (95% CI 0.30–4.2 ml (min kg)−1). O2-pulse increased by 1.7 ml (95% CI 0.29–3.2 ml) and max exercise capacity by 9.6 W (95% CI 2.1–17.7 W). CF decreased resting systolic and diastolic BP by 5.4 mmHg (95% CI −10.7 to −0.1 mmHg) and 2.9 mmHg (95% CI −5.5 to −0.4 mmHg), respectively. Flow-mediated vasodilation (FMD) increased by an absolute 1.3% (95% CI 0.76–1.79%) in the CF group. Indexes of pulmonary function were not affected. No changes for primary and secondary endpoints were detected in control. Conclusion: CF substantially improve markers of cardiorespiratory fitness in healthy elderly humans highlighting their potential to preserve cardiovascular health with increasing age.
Introduction
Loss of functional capacity is a hallmark of cardiovascular aging. Age is one of the major risk factors for development of cardiovascular disease (CVD).1,2 The aging heart is characterized by increasing mass-to-volume ratio, reduced stroke volume and reduced maximum heart rate resulting in reduced cardiac output, which diminishes cardiac reserve capacity and functional status.3 Reduced cardiac regeneration capacity, oxidative stress and chronic low-grade inflammation are key factors for the development of age associated cardiac disease.4 Additionally, aging is associated with endothelial dysfunction and arterial remodeling culminating in luminal dilation and intimal thickening,5,6 which overall predispose for the development of vascular stiffness.
Cardiovascular fitness as a strong predictor of mortality7 is of socioeconomic importance in an aging population worldwide, where costs for care and nursing are growing.8 Cardiopulmonary exercise testing (CPET) is the standard non-invasive method for the quantification of exercise capacity and discrimination of underlying reasons for functional limitation.9 The ability of CPET to assess both peak and submaximal exercise response in a ramp protocol makes it a useful tool in the quantification of capability in daily activities. CPET is a valuable method to estimate prognosis in patients with heart failure and CAD.10 Peak oxygen consumption (peakVO2) has been independently associated with all-cause mortality, death from heart failure, sudden cardiac death, and functional deterioration in heart failure and hypertrophic cardiomyopathy.3,10 High levels of cardiorespiratory fitness are associated with the lowest risk-adjusted all-cause mortality.7
Cocoa flavanols (CF) are a subfamily of flavonoids found in various food groups like berries, chocolate, and wine. Flavanols are thought to increase endothelial NO synthase (eNOS) activity and nitric oxide (NO) bioavailability.11,12 Its major bioactive components in terms of vascular function improvement are (−)-epicatechin and its metabolites and procyanidins may decrease cholesterol and thereby contribute to long-term vascular effects.13,14 The methylxanthines in cocoa and cocoa extracts may contribute to the biological activities of cocoa via enhancing the bioavailability.15 They exert favorable effects on several cardiovascular health markers including low-grade inflammation, oxidative stress,16 endothelial function,17 cholesterol,13 and blood pressure.18
Improved endothelial function and reduced vascular stiffness and normotensive blood pressure positively affect ventricular-arterial-coupling19 leading to improved cardiac output and supposedly better exercise performance. Inorganic nitrate, which also increases NO in the circulation, was shown to improve exercise capacity in heart failure with preserved ejection fraction.20 Several studies have examined the effects of flavanol-rich compounds on exercise performance in healthy volunteers. Decaffeinated green tea extracts have been shown to increase power output and distance covered in a 40 minutes performance trial in recreationally active males.21 Also, higher ventilation threshold during exercise was recorded after graded cycle exercise in non-athlete males under 50 years of age.22 There is evidence for reduced oxygen cost and time trial performance in moderately trained subjects.23 In one study, dark chocolate ingestion containing only 18–36 mg (−)-epicatechin was not associated with overall better performance in high altitude trained cyclists despite significantly lower lactate levels in the chocolate group.24 Our previous work shows that the amount of (−)-epicatechin required to achieve half maximal effects on endothelial function is 31 mg and that the maximal is almost reached after the highest dose at 112 mg (−)-epicatechin (820 mg cocoa flavanols).15 Additionally, our previous studies showed a sustained improvement of FMD after one-month of ingestion of 900 mg cocoa flavanol (128 mg (−)-epicatechin) daily.25 Recently, we showed that the intake of 1000 mg of cocoa flavanols (160 mg (−)-epicatechin) protects radial arteries against intima hyperplasia after catheterization-induced injury.26 We hypothesized, that CF at a dose sufficient to maximally increase endothelial function have a positive impact on peakVO2 and exercise capacity in older adults without manifest cardiovascular disease. Therefore, the aim of the current study was to investigate the effect of dietary CF intake on cardiorespiratory fitness and functional status in healthy elderly individuals. Capsules containing a high-flavanol cocoa extract were given in a randomized, placebo-controlled, double-blinded trial to healthy elderly humans following structured exercise testing.
Methods
Study design
In a randomized, double-masked, placebo-controlled, parallel-group dietary intervention trial, 68 healthy elderly humans (55–79 years, 28 female) were recruited by advertisement from the general population. Posters advertising possible study participation were displayed in hallways of the University Hospital of Duesseldorf and the Heinrich-Heine University Duesseldorf. An initial screening visit was scheduled via E-Mail. Additionally, home-care physicians and cardiologists were asked to screen patients for potential participation. Participants underwent vascular function assessment, biomarker testing and CPET in this standardized order starting in the early afternoon. At baseline and after 30 days, several standard laboratory variables including total cholesterol, LDL cholesterol, HDL cholesterol, triglycerides, creatinine, HbA1C, CRP, hemoglobin, and cell counts were assessed in whole blood, separated and analyzed by the central laboratory of the University Hospital of Duesseldorf. Blood was withdrawn by standard venous puncture. Inclusion criteria were healthy elderly participants over 55 years of age and without diagnoses of cardiovascular disease. In addition to past medical history, heart rate, electrocardiogram, physical examination and laboratory parameters validated a healthy status in all participants (Table 1). Exclusion criteria included age under 55 or over 80 years, non-cardiac limitations of exercise capacity such as orthopedic or neurological disorders, BMI >35 kg m−2, active smoking, uncontrolled blood pressure, diabetes, chronic obstructive pulmonary disease, atrial fibrillation, cardiomyopathy, coronary heart disease, valvular heart disease, cardiac pacemaker, acute respiratory tract infections, regular intake of any kind of medication and missing ability or missing cooperation to participate in the study. Additionally, participants had to be community-dwelling and mobile in day-to-day activities.
Table 1 Baseline characteristics of the study cohort. There were no significant differences between groups at baseline, values are reported as means ± standard deviation (SD). BSA = body surface area; HbA1C = glycated hemoglobin; CRP = C-reactive protein; Hb = hemoglobin
Baseline characteristics |
Control (n = 33) |
Cocoa flavanols (n = 35) |
p-Value |
Age (y) |
64.5 ± 6.6 |
64.3 ± 5.4 |
0.8884 |
Sex
|
|
|
0.3246 |
Male |
17 |
23 |
|
Female |
16 |
12 |
|
Height (cm) |
174 ± 8.4 |
177 ± 8.9 |
0.0996 |
Weight (kg) |
80.2 ± 13.0 |
82.5 ± 14.1 |
0.4839 |
BSA (m2) |
1.99 ± 0.20 |
1.98 ± 0.19 |
0.8296 |
Blood Pressure (mmHg) – baseline |
|
|
|
Systolic |
139.5 ± 16.8 |
137.3 ± 14.6 |
0.3421 |
Diastolic |
81.0 ± 9.5 |
80.3 ± 8.5 |
0.7555 |
Heart rate (b per min) – baseline |
69.6 ± 12.7 |
67.6 ± 10.4 |
0.4654 |
Cholesterol (mg dl
−1
)
|
|
|
|
Total |
208 ± 41.6 |
212 ± 37.7 |
0.6815 |
Low-density |
126 ± 36.0 |
133 ± 33.9 |
0.4125 |
High-density |
65 ± 17.8 |
64 ± 22.0 |
0.8500 |
Triglycerides (mg dl−1) |
170 ± 154.5 |
163 ± 93.6 |
0.8034 |
Creatinine (mg dl−1) |
0.84 ± 0.15 |
0.89 ± 0.19 |
0.2194 |
HbA1C (%) |
5.48 ± 0.47 |
5.43 ± 0.32 |
0.6214 |
CRP (mg dl−1) |
0.25 ± 0.25 |
0.16 ± 0.13 |
0.0594 |
Hb (g dl−1) |
14.01 ± 0.76 |
13.87 ± 2.37 |
0.7367 |
White blood cells (×1000 μL−1) |
6.78 ± 1.25 |
6.64 ± 1.31 |
0.6442 |
After baseline assessment, participants were randomized to either the high-flavanol cocoa or the placebo group. The CF group received 1000 mg of high-flavanol cocoa extract, including 160 mg of (−)-epicatechin. Participants in the placebo group received nutrient-matched control capsules. Mars Edge provided the cocoa flavanol extract and the placebo capsules. Participants were instructed to continue their routines without changing activity or eating behavior. Both groups continued the supplementation of 500 mg twice a day for 30 days. The total amount of flavanols in mg represents the sum of all monomeric flavanols and oligomers, as published before.25 The predominant monomeric flavanol in our capsule was (−)-epicatechin (Table S1†). Capsule format was utilized to simulate the effects of a flavanol-rich diet. Placebo capsules were filled with microcrystalline cellulose and matched for theobromine and caffeine content. Thus, flavanols and (−)-epicatechin were absent in placebo capsules. Overall appearance and weight of placebo capsules were not distinguishable from those of active capsules. After 30 days, the same testing protocol was applied (see Fig. 1A, study protocol). After completing the baseline assessment following a prespecified protocol, participants were randomly assigned to the intervention or placebo group by an independent study nurse in the clinical trial unit. The group allocation was then disclosed via telephone. Capsule containers were labeled with double-blinded alphabetical character codes. The unblinding process was performed by a pre-determined protocol after study completion.
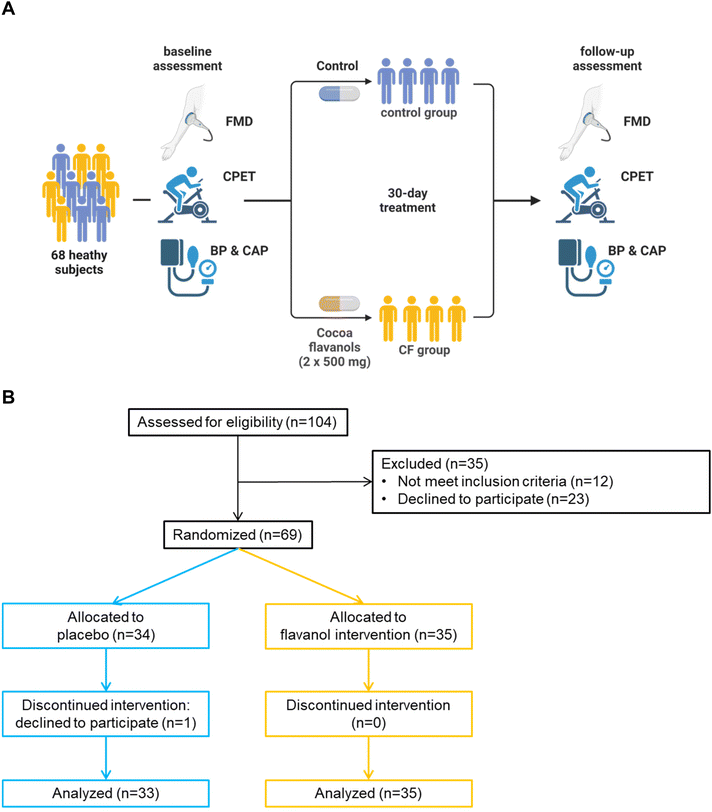 |
| Fig. 1 Study protocol. (A): 68 healthy elderly adults (aged 55 to 79 years) received either 500 mg of Cocoa flavanols (CF) capsule twice daily or a nutrient-matched control capsule for 30 days in a randomized, double-masked, placebo-controlled design. Cardiopulmonary exercise testing (CPET), flow-mediated dilatation (FMD), central aortic pressure (CAP) and blood pressure (BP) measurements were performed at baseline and after 30 days. Primary endpoint was exercise capacity and peakVO2. Secondary endpoints included CAP, BP, and resting vascular function, measured as FMD. (B): CONSORT flow diagram. | |
Written informed consent was obtained from each participant. The study protocol conforms to the ethical guidelines of the 1975 Declaration of Helsinki as reflected in a priori approval the ethics committee of the Heinrich Heine University Düsseldorf (Approval Number R5761R). Initial registration on clinicaltrials.gov was mistakenly done with 410 mg of CF twice daily. After the unblinding process, the registration form was corrected to 500 mg CF twice daily. All participants randomized to intervention (CF) arm received the same dose of CF. Registered on clinicaltrials.gov (NCT 05782309).
CPET
CPET was conducted using a standardized bicycle ergometer test protocol at baseline and after 30 days. The test was performed with an upright cycle ergometer (Ergoselect 100, Ergoline, Bitz, Germany) with corresponding CPET equipment (CORTEX METALYZER 3B, CORTEX Biophysik GmbH, Leipzig, Germany) at the same time of day for all participants. Before examination, there was a 2-minute load free warmup period to get accustomed to the mask and breathing pattern. The testing protocol was an incremental ramp protocol with increases in work rate of 25 watts every 2 minutes. Participants were instructed to try maintaining a 55–70 rounds per minute cadence. Site technicians were trained and certified for the protocol and followed a detailed CPET manual. Gas exchange analysis was done with an online display over 3 breaths and a standard 30 seconds moving average for export of the final data. The examination was terminated if one of the following criteria appeared: muscular exhaustion, dyspnea, fatigue, dizziness, systolic blood pressure >220 mmHg, ECG changes or arrhythmia, RER >1.15 or a leveling off. Heart rate reserve <5 bpm (predicted) was defined as a criterion for effort, not necessarily for termination. PeakVO2 was assessed as the primary endpoint. Also, the following prespecified exploratory cardiovascular parameters were recorded: VCO2, peak minute ventilation (VE), peak RER, peak heart rate, peak exercise capacity, peak oxygen pulse (VO2 per HR) and VO2 at ventilatory threshold. A trained Sports Cardiologist in a double-blinded approach analyzed the CPET data.
FMD
FMD was measured as previously described27,28 on the participant's right arm. Vessel diameter and flow velocity of the radial artery (RA) were measured using a 12 MHz transducer (Vivid I, GE) and automatic edge-detection software (Brachial Analyzer, Medical Imaging Applications, Iowa City, Iowa) yielding standard deviations of mean differences between repeated measurements of less than an absolute 1.0%. Reactive hyperemia was induced by 5 min of lower arm occlusion with a sphygmomanometer cuff inflated to 200 mmHg. Immediately after cuff deflation, and 20, 40, 60, and 80 s later, RA diameter was assessed and FMD was calculated as maximal relative diameter gain relative to baseline.
Blood pressure and central aortic pressure
Peripheral blood pressure was measured with an automated medical device (boso medicus, BOSCH + SOHN GmbH u. Co. KG) at both participant's left and right brachial artery to calculate the arithmetical mean, which was the used for analyses.
Central aortic pressure was assessed by noninvasive measurement of the central aortic pressure waveform using Sphygmocor® platform (Atcor medical, Sydney, Australia). Calculation of central aortic pressures is calculated via an integrated algorithm after entering individual patient characteristics and peripheral blood pressure.25
Statistical analysis
Data are presented as means ± standard deviation (SD). Statistical analyses of baseline demographics were performed using Fisher's exact test (case controls), and unpaired t-tests. Statistical analysis of the intervention effects were performed by repeated measurements two- or three-way ANOVA with the Bonferroni post hoc test. Significance was assumed if p was <0.05. Analyses were performed by GraphPad Prism version 9 and SPSS statistics, version 24 (IBM, Armonk, USA). During planning of the study, the sample size was estimated using G-Power V3.1. (Heinrich Heine University of Duesseldorf). Previous publications showed a mean peakVO2 per BW of 14.3 ± 4.8 (SD) ml kg−1 min−1 in elderly individuals.29 Since 30-day intake of CF led to a 30% increase in FMD,30 which has a positive relationship to peakVO2,31 we assumed an increase in peakVO2 per BW of 25% in the intervention group. Based on this data we calculated a sample size of n = 28 in each group as required with an alpha level of 5% and a power of 80%. To allow dropouts the final total sample size was set at 62 subjects.
Results
Study population
From April 2018 to November 2021, after screening of 104 possible participants, 68 elderly adults (>55 years) fulfilled inclusion criteria and were defined as healthy. One participant initially randomized to control declined participation after baseline examination and was excluded from the study. Baseline characteristics are presented in Table 1. Baseline exercise parameters were similar for both groups (Table 2). Baseline measures of FMD, peripheral blood pressure and central aortic pressure are presented in Table 3 and were not different between groups.
Table 2 Effects of dietary CFs on measures of exercise capacity during CPET. peakVO2, peakVO2 per kg, O2-pulse, and exercise capacity significantly improved with CFs; VE increased in both groups. BL = baseline; FU = follow up; HR = heart rate; RER = respiratory exchange ratio; VE = minute ventilation; peakVO2 = maximum oxygen uptake, peakVO2 per kg = maximum oxygen uptake per kg of bodyweight, O2-pulse = oxygen uptake per heartbeat; VT = tidal volume W = power in Watts; *p < 0.05; repeated measurements 2-way-(time × intervention) ANOVA with Bonferroni's post hoc test
Measures of exercise capacity during CPET |
Intervention group |
Control (n = 33) |
Cocoa flavanols (n = 35) |
BL |
FU |
p
|
BL |
FU |
p
|
VO2peak (l min−1) |
1.88 ± 0.60 |
1.93 ± 0.62 |
0.7742 |
1.83 ± 0.60 |
2.02 ± 0.60 |
0.0135*
|
VO2peak per kg (ml min−1 kg−1) |
22.40 ± 6.74 |
23.40 ± 6.94 |
0.4790 |
22.74 ± 6.88 |
25.25 ± 6.78 |
0.0124*
|
VO2 at VT1 (l min−1) |
1.33 ± 0.48 |
1.41 ± 0.48 |
0.7150 |
1.37 ± 0.45 |
1.36 ± 0.42 |
0.9396 |
VO2 at VT1/VO2peak [%] |
70.1% |
70.4% |
— |
74.9% |
67.7% |
— |
O2-pulse (VO2 per HR) (ml) |
13.29 ± 4.55 |
14.22 ± 4.55 |
0.3316 |
13.37 ± 4.78 |
15.11 ± 3.95 |
0.0175*
|
Exercise capacity (W) |
150.5 ± 49.04 |
151.3 ± 51.94 |
0.9667 |
151.5 ± 45.6 |
161.1 ± 53.8 |
0.0472*
|
HR max (beats per min) |
140.6 ± 20.4 |
140.7 ± 22.1 |
0.9929 |
136.0 ± 19.7 |
131.6 ± 17.7 |
0.3611 |
RER max |
1.110 ± 0.09 |
1.091 ± 0.08 |
0.3310 |
1.083 ± 0.08 |
1.075 ± 0.06 |
0.9992 |
VE max (l min−1) |
57.55 ± 21.0 |
64.03 ± 26.4 |
0.0229*
|
55.26 ± 17.1 |
63.69 ± 22.3 |
0.0025*
|
VT max (l) |
2.058 ± 0.68 |
2.153 ± 0.65 |
0.3722 |
2.025 ± 0.59 |
2.144 ± 0.63 |
0.2184 |
Table 3 Effects of dietary CFs on vascular function. FMD increased with CFs. Peripheral blood pressure and central aortic pressure decreased with CFs at follow up. No changes were seen in the control group. BL = baseline; FU = follow up FMD = flow-mediated-dilation; *p < 0.05; repeated measurements 2-way-(time × intervention) ANOVA with Bonferroni's post hoc test
Results in FMD and blood pressure |
Intervention group |
Control (n = 33) |
Cocoa flavanols (n = 35) |
BL |
FU |
p
|
BL |
FU |
p
|
FMD peak change [%] |
4.33 ± 1.16 |
4.23 ± 1.18 |
0.8631 |
4.59 ± 1.85 |
5.87 ± 1.93 |
<0.0001*
|
Peripheral blood pressure
|
Systolic (mmHg) |
139.5 ± 16.8 |
135.3 ± 17.7 |
0.1828 |
137.3 ± 14.6 |
131.9 ± 13.9 |
0.0456*
|
Diastolic (mmHg) |
81.0 ± 9.5 |
79.3 ± 6.5 |
0.3949 |
80.3 ± 8.5 |
77.4 ± 8.1 |
0.0362*
|
Central aortic pressure
|
Systolic (mmHg) |
129.2 ± 15.6 |
125.1 ± 16.1 |
0.1450 |
126.9 ± 14.2 |
120.6 ± 13.8 |
0.0118*
|
Diastolic (mmHg) |
82.6 ± 9.7 |
80.7 ± 6.5 |
0.3612 |
81.1 ± 7.8 |
78.5 ± 8.3 |
0.0292*
|
CF increase cardiorespiratory fitness
CF improved peak oxygen uptake (peakVO2) by 190 ml min−1 (1.83 ± 0.6 (SD) at baseline versus 2.02 ± 0.6 l min−1 at follow-up, Fig. 2A, p = 0.0135) and peakVO2 per kg of bodyweight (VO2peak per kg) by 2.51 ml (min kg)−1 (22.74 ± 6.88 at baseline versus 25.25 ± 6.78 ml (min kg)−1 at follow-up, Fig. 2B, p = 0.0124). In a post hoc analysis investigating sex as a third variable using a three-way ANOVA, we found a significant rise in peakVO2 (p = 0.0241) and VO2peak per kg (p = 0.0410) of male participants receiving CF. O2-Pulse increased by 1.74 ml (13.37 ± 4.78 at baseline versus 15.11 ± 3.95 ml at follow-up, Fig. 2C, p = 0.0175) and maximum exercise capacity by 9.6 W (151.5 ± 45.6 at baseline versus 161.1 ± 53.8 W at follow-up, Fig. 2D, p = 0.0472). No significant changes were seen for these parameters in the control group (peakVO2: 1.88 ± 0.6 at baseline versus 1.93 ± 0.62 l min−1 at follow-up, Fig. 2A, p = 0.7742; VO2peak per kg: 22.4 ± 6.74 at baseline versus 23.4 ± 6.94 ml (min kg)−1 at follow-up, Fig. 2B, p = 0.4790; O2-pulse: 13.29 ± 4.55 at baseline versus 14.22 ± 4.55 ml at follow-up, Fig. 2C, p = 0.3316; exercise capacity: 150.5 ± 49.1 at baseline versus 151.3 ± 52 W at follow-up, Fig. 2D, p = 0.0667). Maximum minute ventilation (VE) increased in CF and control group. No changes were observed in maximum heart rate (HR max) and RER max. VO2 at estimated ventilatory threshold (VO2 at VT1) remained unchanged in both groups after 30 days (Table 2).
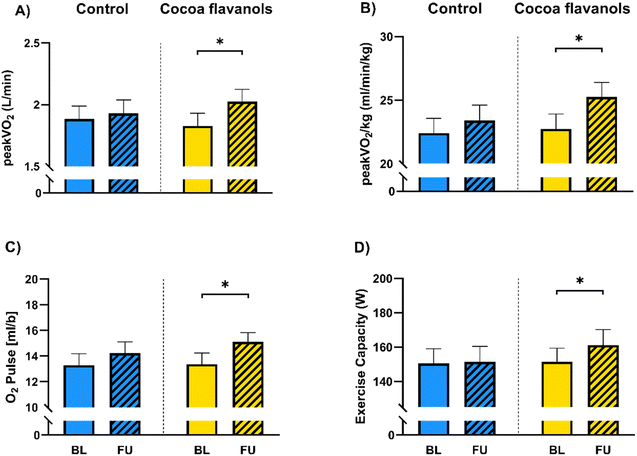 |
| Fig. 2 Dietary CFs increase maximum oxygen uptake and exercise capacity. Significant increase in (A) peakVO2, (B) peakVO2 per kg, (C) O2 pulse, and (D) exercise capacity at maximum workload after 30 days of CF intake compared to baseline. No changes were observed with control. *p < 0.05; repeated measurements 2-way-(time × intervention) ANOVA with Bonferroni's post hoc test. BL = baseline; FU = follow-up; peakVO2 = maximum oxygen uptake, peakVO2 per kg = maximum oxygen uptake per kg of bodyweight, O2-pulse = oxygen uptake per heartbeat. | |
CF decrease blood pressure and improve vascular function parameters
Resting systolic and diastolic BP decreased by 5.4 mmHg (137.3 ± 14.6 (SD) at baseline versus 131.9 ± 13.9 mmHg at follow-up, Fig. 3A, p = 0.0456) and 2.9 mmHg (80.3 ± 8.5 at baseline versus 77.4 ± 8.1 mmHg at follow-up, Fig. 3C, p = 0.0362), respectively in the CF group. Also, central systolic and diastolic aortic pressure decreased in the CF group by 6.3 mmHg (126.9 ± 14.2 at baseline versus 120.6 ± 13.8 mmHg at follow-up, Fig. 3B, p = 0.0118) and 2.6 mmHg (81.1 ± 7.8 at baseline versus 78.5 ± 8.3 mmHg at follow-up, Fig. 3D, p = 0.0292), respectively (Fig. 3). FMD increased by an absolute 1.28% (4.59 ± 1.85 at baseline versus 5.87 ± 1.93% at follow-up, Fig. 4B–D, p < 0.0001) in the CF group. No changes were documented in the control group (Fig. 4). In laboratory analyses levels of NT-proBNP were within the normal range for the assay utilized (ULN 125 ng L−1) and remained unchanged in both groups.
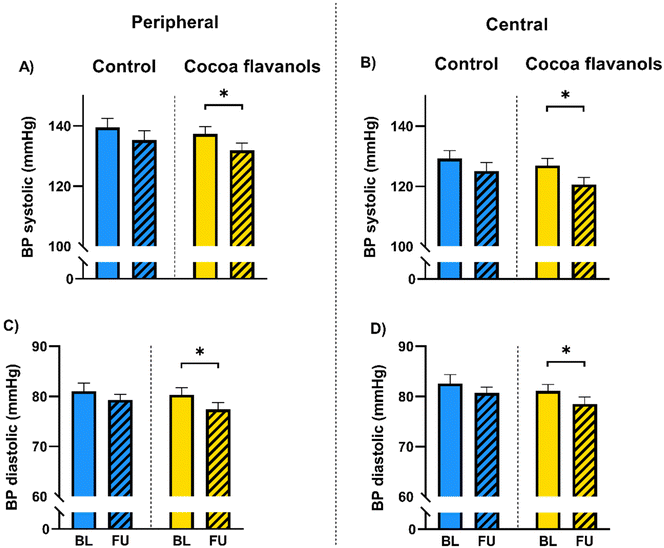 |
| Fig. 3 Dietary CFs decrease blood pressure. Significant decrease in peripheral blood pressure (A & C) and central aortic pressure (B & D) after 30 days of CF intake. No changes occurred with control. *p < 0.05; repeated measurements 2-way-(time × intervention) ANOVA with Bonferroni's post hoc test. AP = aortic pressure; BP = blood pressure; BL = baseline; FU = follow up. | |
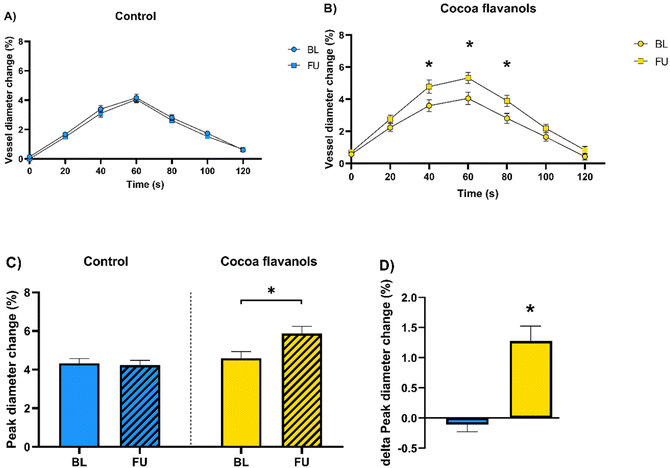 |
| Fig. 4 Dietary CFs improve endothelial function. (A) Unchanged FMD with control (B) significant increase at FU in FMD at 40 s, 60 s and at 80 s after CF intake compared to BL; (C) significantly higher Peak inner diameter change at FU vs. BL with CFs, (D) increased delta in peak diameter change from BL to FU with CFs but not with control; *p < 0.05; repeated measurements 2-way-(time × intervention) ANOVA with Bonferroni's post hoc test. BL = baseline; FU = follow up. FMD = flow-mediated-dilation. | |
Discussion
The present results demonstrate that dietary CF intake improves exercise capacity in healthy elderly humans. Improvement of cardiorespiratory fitness through dietary intervention may contribute to the maintenance of health in older individuals. The increase in exercise capacity was mainly driven by improvement of cardiac performance while pulmonary indices were not altered. The chosen amount of CFs enhanced endothelial function and lowered arterial blood pressure, both of which may have contributed to improvement in cardiac exercise capacity with significantly increased oxygen carrying capacity per heartbeat.
Exercise capacity in healthy elderly humans
According to the World Health Organization health is considered a “state of complete physical, mental and social well-being and not merely the absence of disease”.32 Participants in this study were screened prior to randomization to ensure the absence of manifest cardiovascular diseases. With increasing age large artery stiffness develops inducing mild increases in central arterial blood pressure.33 In our study population, elderly participants showed slightly elevated systolic blood pressure with an average of 139 and 137 mmHg. Also, LDL-cholesterol levels were mildly elevated. According to current guidelines lifestyle interventions are recommended as first line option to modify these parameters.34–36 Healthy aging comprises the primary prevention of functional limitations, occult and manifest disease with increasing age. Blood pressure, pulse wave velocity and FMD have been proposed to monitor the transition of healthy to dysfunctional arteries with increasing age.37 Young adults under 25 years of age are generally suspected healthy. After the age of 45 years subclinical disease starts building up depending on previous lifestyle behavior and risk factors. Beyond 65 years of age CVD starts manifesting and eliciting events.38 Age per se is considered an independent risk factor for CVD and mortality.39,40 CPET is a valuable tool to assess cardiac, circulatory, and pulmonary function and health. It is a highly reliable and reproducible method for measuring peakVO2 and to evaluate the functional capacity in healthy and diseased humans. PeakVO2 is also a strong predictor of long-term all-cause, CV- and cancer mortality.7,41 Limitations to cardiopulmonary fitness can often be assigned to one of the primary components: skeletal muscle oxygen extraction, pulmonary oxygen uptake, blood oxygen transport capacity and cardiac output for oxygen distribution. Cardiac performance in this regard is also modulated by preload and afterload. Cardiac afterload is amongst others determined by blood pressure and endothelial function, alterations of both have been associated with the cardiovascular aging process.25,37 The aging heart itself is less well understood but comprises a gradual deterioration of functional and structural characteristics including increased mass-to-volume-ratio and a decline in diastolic function.3,42 Reduced β-adrenergic response and consecutively reduced maximum heart rate are prevalent in aging hearts.43 Especially, age-related decline in cardiac functional parameters can be positively modified44,45 with aerobic interval training, while the effect of dietary interventions with CFs on exercise capacity in the elderly healthy population has not been well characterized.
Cocoa flavanols improve exercise capacity in healthy elderly humans
After a period of 30-days dietary intake CF, CF increased peakVO2, peakVO2 per kg, O2-pulse, and exercise capacity suggesting beneficial effects on cardiovascular fitness. Indeed, the increase in peakVO2 of 190 ml or 2.5 ml per kg of body weight that was achieved with dietary intake is comparable to the effects in structured exercise programs.46,47 In older adults over 70 years of age, a supervised exercise training with high-intensity intervals improved peakVO2.48 With increasing age applicability of physical exercise programs may be limited due to orthopedic diseases and thus dietary interventions may offer complementary tools to maintain cardiovascular health and fitness. Comparable to our results, dark chocolate supplementation previously has shown improvements in peakVO2 by 6% versus baseline and reduced oxygen cost in moderately trained participants.23 No overall performance benefit despite lower lactate levels changes appeared in trained cyclists when only 18–36 mg of (−)-epicatechin was administered.24 This suggests that CF induced improvements might depend on baseline fitness, vascular function status and sufficient CF to exert an effect. Similarly, in patients with heart failure with preserved ejection fraction other sources of NO like beet root juice induced about 8% improvement in peakVO2.20 In a post-hoc analysis, we asked whether sex might affect the benefits of CF intake on exercise capacity. Interestingly, the multiple comparisons of the three-way ANOVA showed a significant result for men receiving CF. Since our study was not powered to consider sex-specific changes, these data cannot answer possible sex-specific effects of CF on exercise capacity. Maximum heart rate was unaffected in our study groups. An increased O2-pulse suggests an improvement in stroke volume as a potential primary mechanism for improved oxygen transport. Stroke volume is amongst others dependent on the afterload. Determinants of left ventricular afterload are BP, CAP and aortic stiffness,37,49 all of them have been positively modulated through CFs. In our study population a reduction in systolic BP by 5.4 mmHg and CAP by 6.3 mmHg was achieved. Thus, an improved afterload and arterio-ventricular coupling can contribute to the increase in stroke volume. Decreased afterload, in the long term, prevents ventricular remodeling and hypertrophy.50 To support this process through a different mechanism, CFs have been linked to decreased inflammatory response,51 which further reduces progression of left ventricular remodeling, hypertrophy and fibrosis.40,52,53
Effectiveness of CFs to improve vascular function in healthy humans
In our study peripheral blood pressure and CAP decreased, whereas FMD, as a marker for endothelial function, increased in the CF group. These findings corroborate our previous findings that not only in diseased but also in healthy individuals, CFs improve vascular function and cardiovascular risk markers.12,25,54 The proposed increase in eNOS activity in healthy adults14 is one likely mechanism by which CFs and especially (−)-epicatechin improve vascular function, blood pressure25,37 and endothelial integrity.55 Overall, dietary interventions have been shown to reestablish disturbed redox signaling in the vasculature.56 As already demonstrated in the COSMOS trial57 a 27% lowering in cardiovascular deaths can be achieved through CF intake at half the amount in elderly individuals free from CVD after a 3.6-year follow-up. The amount of dietary CFs given in our study is above the average intake in a typical European diet. Dietary extracts of CFs are generally considered safe in concentrations up to 2 g day−1.58 Our results for vascular function assessment serve for indirect validation of the CF effect. Importantly, we did not see any non-responder regarding CF effect on FMD in the intervention group. (−)-Epicatechin is likely the major bioactive in cocoa responsible for vascular effects.13 Our data indicate that 31 mg are required to acutely achieve half-maximal effects on FMD.15 The (−)-epicatechin content in commercial chocolates varies greatly, but can be zero and as high as 269 mg per 100 g chocolate.59 The consumption of 60 g of a high (−)-epicatechin chocolate would achieve a similar amount as administered in the current study (160 mg).59–61
Limitations
A few limitations of the present study are worth mentioning. First, we did not assess individual exercise behavior nor food diaries addressing estimates of habitual CF intake in the participants’ regular diet. Second, we did not collect plasma nor urine levels of circulating flavanols and their structurally related or gut microbiome derived metabolites to ensure regular intake and sufficient absorption. However, the observed selective increase in FMD all participants within the CF group with a 10% percent response rate argues for a high adherence within the entire study cohort. Further, we did not assess participants’ physical activity status at baseline or throughout the study period and did not ask for caffeine intake prior to exercise testing. Further studies should consider activity trackers or questionnaires to meet this issue.
Conclusion
We here provide evidence, that intake of CFs at a dose efficient to improve endothelial function improves markers of cardiorespiratory fitness in healthy elderly humans highlighting their potential for supporting maintenance of cardiovascular health and fitness with increasing age. Future studies should evaluate whether these effects are sustained with long-term CF intake and translate into clinically relevant improvements in fitness, quality of life and lowering of cardiovascular events and mortality.
Author contributions
All authors take responsibility for all aspects of the reliability and freedom from bias of the data presented and their discussed interpretation.
M. G., R. S., C. H., M. K. and R. E. designed the study; M. G., N. O. and N. K. conducted the research; M. G., N. O., N. K., R. S. and R. E. analyzed the data; M. G., D. D., C. H., C. J., M. K. and R. E. wrote the manuscript.
Abbreviations
BMI | Body mass index |
BP | Blood pressure |
BSA | Body surface area |
CAP | Central aortic pressure |
CF | Cocoa flavanol |
CPET | Cardiopulmonary exercise testing |
CVD | Cardiovascular disease |
FMD | Flow-mediated vasodilation |
HR | Heart rate |
PeakVO2 | Maximum oxygen consumption |
RA | Radial artery |
RER | Respiratory exchange ratio |
VO2 | Volume flow of oxygen |
VT | Ventilatory threshold |
Conflicts of interest
There are no conflicts to declare.
Acknowledgements
Cocoa flavanol and placebo capsules were donated by Mars, Inc., USA.
References
- C. W. Tsao, A. W. Aday, Z. I. Almarzooq, A. Alonso, A. Z. Beaton, M. S. Bittencourt, A. K. Boehme, A. E. Buxton, A. P. Carson, Y. Commodore-Mensah, M. S. V. Elkind, K. R. Evenson, C. Eze-Nliam, J. F. Ferguson, G. Generoso, J. E. Ho, R. Kalani, S. S. Khan, B. M. Kissela, K. L. Knutson, D. A. Levine, T. T. Lewis, J. Liu, M. S. Loop, J. Ma, M. E. Mussolino, S. D. Navaneethan, A. M. Perak, R. Poudel, M. Rezk-Hanna, G. A. Roth, E. B. Schroeder, S. H. Shah, E. L. Thacker, L. B. VanWagner, S. S. Virani, J. H. Voecks, N. Y. Wang, K. Yaffe and S. S. Martin, Heart Disease and Stroke Statistics-2022 Update: A Report From the American Heart Association, Circulation, 2022, 145, e153–e639 CrossRef PubMed.
- M. M. Redfield, S. J. Jacobsen, J. C. Burnett Jr., D. W. Mahoney, K. R. Bailey and R. J. Rodeheffer, Burden of systolic and diastolic ventricular dysfunction in the community: appreciating the scope of the heart failure epidemic, J. Am. Med. Assoc., 2003, 289, 194–202 CrossRef PubMed.
- F. Triposkiadis, A. Xanthopoulos and J. Butler, Cardiovascular Aging and Heart Failure: JACC Review Topic of the Week, J. Am. Coll. Cardiol., 2019, 74, 804–813 CrossRef PubMed.
- E. G. Lakatta and D. Levy, Arterial and cardiac aging: major shareholders in cardiovascular disease enterprises: Part I: aging arteries: a “set up” for vascular disease, Circulation, 2003, 107, 139–146 CrossRef PubMed.
- C. C. Haudenschild, M. F. Prescott and A. V. Chobanian, Aortic endothelial and subendothelial cells in experimental hypertension and aging, Hypertension, 1981, 3, I148–I153 CrossRef CAS PubMed.
- M. Bapir, G. R. Untracht, J. E. A. Hunt, J. H. McVey, J. Harris, S. S. Skene, P. Campagnolo, N. Dikaios, A. Rodriguez-Mateos, D. D. Sampson, D. M. Sampson and C. Heiss, Age-Dependent Decline in Common Femoral Artery Flow-Mediated Dilation and Wall Shear Stress in Healthy Subjects, Life, 2022, 12(12), 2023 CrossRef PubMed.
- K. Mandsager, S. Harb, P. Cremer, D. Phelan, S. E. Nissen and W. Jaber, Association of Cardiorespiratory Fitness With Long-term Mortality Among Adults Undergoing Exercise Treadmill Testing, JAMA Network Open, 2018, 1, e183605 CrossRef PubMed.
- OECD, Health at a Glance https://www.oecd.org/health/health-at-a-glance/.
- M. Guazzi, M. Wilhelm, M. Halle, E. Van Craenenbroeck, H. Kemps, R. A. de Boer, A. J. S. Coats, L. Lund, D. Mancini, B. Borlaug, G. Filippatos and B. Pieske, Exercise testing in heart failure with preserved ejection fraction: an appraisal through diagnosis, pathophysiology and therapy - A clinical consensus statement of the Heart Failure Association and European Association of Preventive Cardiology of the European Society of Cardiology, Eur. J. Heart Failure, 2022, 24, 1327–1345 CrossRef CAS PubMed.
- M. Guazzi, F. Bandera, C. Ozemek, D. Systrom and R. Arena, Cardiopulmonary Exercise Testing: What Is its Value?, J. Am. Coll. Cardiol., 2017, 70, 1618–1636 CrossRef PubMed.
- A. Rodriguez-Mateos, N. Toro-Funes, T. Cifuentes-Gomez, M. Cortese-Krott, C. Heiss and J. P. Spencer, Uptake and metabolism of (-)-epicatechin in endothelial cells, Arch. Biochem. Biophys., 2014, 559, 17–23 CrossRef CAS PubMed.
- P. Horn, N. Amabile, F. S. Angeli, R. Sansone, B. Stegemann, M. Kelm, M. L. Springer, Y. Yeghiazarians, H. Schroeter and C. Heiss, Dietary flavanol intervention lowers the levels of endothelial microparticles in coronary artery disease patients, Br. J. Nutr., 2014, 111, 1245–1252 CrossRef CAS PubMed.
- A. Rodriguez-Mateos, T. Weber, S. S. Skene, J. I. Ottaviani, A. Crozier, M. Kelm, H. Schroeter and C. Heiss, Assessing the respective contributions of dietary flavanol monomers and procyanidins in mediating cardiovascular effects in humans: randomized, controlled, double-masked intervention trial, Am. J. Clin. Nutr., 2018, 108, 1229–1237 CrossRef PubMed.
- H. Schroeter, C. Heiss, J. Balzer, P. Kleinbongard, C. L. Keen, N. K. Hollenberg, H. Sies, C. Kwik-Uribe, H. H. Schmitz and M. Kelm, (-)-Epicatechin mediates beneficial effects of flavanol-rich cocoa on vascular function in humans, Proc. Natl. Acad. Sci. U. S. A., 2006, 103, 1024–1029 CrossRef CAS PubMed.
- R. Sansone, J. I. Ottaviani, A. Rodriguez-Mateos, Y. Heinen, D. Noske, J. P. Spencer, A. Crozier, M. W. Merx, M. Kelm, H. Schroeter and C. Heiss, Methylxanthines enhance the effects of cocoa flavanols on cardiovascular function: randomized, double-masked controlled studies, Am. J. Clin. Nutr., 2017, 105, 352–360 CrossRef CAS PubMed.
- M. Martin and S. Ramos, Impact of cocoa flavanols on human health, Food Chem. Toxicol., 2021, 151, 112121 CrossRef CAS PubMed.
- C. Heiss, A. Dejam, P. Kleinbongard, T. Schewe, H. Sies and M. Kelm, Vascular effects of cocoa rich in flavan-3-ols, J. Am. Med. Assoc., 2003, 290, 1030–1031 CrossRef PubMed.
- K. Ried, T. R. Sullivan, P. Fakler, O. R. Frank and N. P. Stocks, Effect of cocoa on blood pressure, Cochrane Database Syst. Rev., 2012, Cd008893, DOI:10.1002/14651858.CD008893.pub2.
- H. Holm, M. Magnusson, A. Jujić, E. Bozec and N. Girerd, How to calculate ventricular-arterial coupling?, Eur. J. Heart Failure, 2022, 24, 600–602 CrossRef PubMed.
- P. Zamani, D. Rawat, P. Shiva-Kumar, S. Geraci, R. Bhuva, P. Konda, P. T. Doulias, H. Ischiropoulos, R. R. Townsend, K. B. Margulies, T. P. Cappola, D. C. Poole and J. A. Chirinos, Effect of inorganic nitrate on exercise capacity in heart failure with preserved ejection fraction, Circulation, 2015, 131, 371–380 CrossRef CAS PubMed ; discussion 380.
- J. D. Roberts, M. G. Roberts, M. D. Tarpey, J. C. Weekes and C. H. Thomas, The effect of a decaffeinated green tea extract formula on fat oxidation, body composition and exercise performance, J. Int. Soc. Sports Nutr., 2015, 12, 1 CrossRef PubMed.
- N. Ota, S. Soga and A. Shimotoyodome, Daily consumption of tea catechins improves aerobic capacity in healthy male adults: a randomized double-blind, placebo-controlled, crossover trial, Biosci., Biotechnol., Biochem., 2016, 80, 2412–2417 CrossRef CAS PubMed.
- R. K. Patel, J. Brouner and O. Spendiff, Dark chocolate supplementation reduces the oxygen cost of moderate intensity cycling, J. Int. Soc. Sports Nutr., 2015, 12, 47 CrossRef PubMed.
- K. Shaw, J. Singh, L. Sirant, J. P. Neary and P. D. Chilibeck, Effect of Dark Chocolate Supplementation on Tissue Oxygenation, Metabolism, and Performance in Trained Cyclists at Altitude, Int. J. Sport Nutr. Exercise Metab., 2020, 1–7, DOI:10.1123/ijsnem.2020-0051.
- R. Sansone, A. Rodriguez-Mateos, J. Heuel, D. Falk, D. Schuler, R. Wagstaff, G. G. Kuhnle, J. P. Spencer, H. Schroeter, M. W. Merx, M. Kelm and C. Heiss, Cocoa flavanol intake improves endothelial function and Framingham Risk Score in healthy men and women: a randomised, controlled, double-masked trial: the Flaviola Health Study, Br. J. Nutr., 2015, 114, 1246–1255 CrossRef CAS PubMed.
- M. Gröne, M. Schillings, D. Duse, N. Kramser, C. Quast, C. Heiss, R. Sansone, C. Jung, M. Kelm and R. Erkens, Cocoa flavanol supplementation preserves early and late radial artery function after transradial catheterization, Food Funct., 2023, 14, 4824–4835 RSC.
- C. Heiss, J. Balzer, T. Hauffe, S. Hamada, E. Stegemann, T. Koeppel, M. W. Merx, T. Rassaf, M. Kelm and T. Lauer, Vascular dysfunction of brachial artery after transradial access for coronary catheterization: impact of smoking and catheter changes, JACC Cardiovasc. Interv., 2009, 2, 1067–1073 CrossRef PubMed.
- R. Sansone, E. Stegemann, G. Ozaslan, D. Schuler, M. Lukosz, A. Rodriguez-Mateos, T. Lauer, R. Westenfeld, M. Kelm and C. Heiss, Early and late response-to-injury in patients undergoing transradial coronary angiography: arterial remodeling in smokers, Am. J. Cardiovasc. Dis., 2014, 4, 47–57 Search PubMed.
- R. M. Ross, J. N. Murthy, I. D. Wollak and A. S. Jackson, The six minute walk test accurately estimates mean peak oxygen uptake, BMC Pulm. Med., 2010, 10, 31 CrossRef PubMed.
- J. Balzer, T. Rassaf, C. Heiss, P. Kleinbongard, T. Lauer, M. Merx, N. Heussen, H. B. Gross, C. L. Keen, H. Schroeter and M. Kelm, Sustained benefits in vascular function through flavanol-containing cocoa in medicated diabetic patients a double-masked, randomized, controlled trial, J. Am. Coll. Cardiol., 2008, 51, 2141–2149 CrossRef CAS PubMed.
- E. Kasikcioglu, H. Oflaz, H. A. Kasikcioglu, A. Kayserilioglu, S. Umman and M. Meric, Endothelial flow-mediated dilatation and exercise capacity in highly trained endurance athletes, Tohoku J. Exp. Med., 2005, 205, 45–51 CrossRef PubMed.
- Constitution of the World Health Organization, Am. J. Public Health., 1946, 36, 1315–1323 CrossRef PubMed.
- J. A. Chirinos, P. Segers, T. Hughes and R. Townsend, Large-Artery Stiffness in Health and Disease: JACC State-of-the-Art Review, J. Am. Coll. Cardiol., 2019, 74, 1237–1263 CrossRef PubMed.
- F. L. J. Visseren, F. Mach, Y. M. Smulders, D. Carballo, K. C. Koskinas, M. Bäck, A. Benetos, A. Biffi, J. M. Boavida, D. Capodanno, B. Cosyns, C. Crawford, C. H. Davos, I. Desormais, E. Di Angelantonio, O. H. Franco, S. Halvorsen, F. D. R. Hobbs, M. Hollander, E. A. Jankowska, M. Michal, S. Sacco, N. Sattar, L. Tokgozoglu, S. Tonstad, K. P. Tsioufis, I. van Dis, I. C. van Gelder, C. Wanner and B. Williams, 2021 ESC Guidelines on cardiovascular disease prevention in clinical practice, Eur. Heart J., 2021, 42, 3227–3337 CrossRef PubMed.
- B. Williams, G. Mancia, W. Spiering, E. Agabiti Rosei, M. Azizi, M. Burnier, D. L. Clement, A. Coca, G. de Simone, A. Dominiczak, T. Kahan, F. Mahfoud, J. Redon, L. Ruilope, A. Zanchetti, M. Kerins, S. E. Kjeldsen, R. Kreutz, S. Laurent, G. Y. H. Lip, R. McManus, K. Narkiewicz, F. Ruschitzka, R. E. Schmieder, E. Shlyakhto, C. Tsioufis, V. Aboyans and I. Desormais, 2018 ESC/ESH Guidelines for the management of arterial hypertension, Eur. Heart J., 2018, 39, 3021–3104 CrossRef PubMed.
- F. Mach, C. Baigent, A. L. Catapano, K. C. Koskinas, M. Casula, L. Badimon, M. J. Chapman, G. G. De Backer, V. Delgado, B. A. Ference, I. M. Graham, A. Halliday, U. Landmesser, B. Mihaylova, T. R. Pedersen, G. Riccardi, D. J. Richter, M. S. Sabatine, M.-R. Taskinen, L. Tokgozoglu, O. Wiklund, S. Windecker, V. Aboyans, C. Baigent, J.-P. Collet, V. Dean, V. Delgado, D. Fitzsimons, C. P. Gale, D. Grobbee, S. Halvorsen, G. Hindricks, B. Iung, P. Jüni, H. A. Katus, U. Landmesser, C. Leclercq, M. Lettino, B. S. Lewis, B. Merkely, C. Mueller, S. Petersen, A. S. Petronio, D. J. Richter, M. Roffi, E. Shlyakhto, I. A. Simpson, M. Sousa-Uva, R. M. Touyz, D. Nibouche, P. H. Zelveian, P. Siostrzonek, R. Najafov, P. van de Borne, B. Pojskic, A. Postadzhiyan, L. Kypris, J. Špinar, M. L. Larsen, H. S. Eldin, M. Viigimaa, T. E. Strandberg, J. Ferrières, R. Agladze, U. Laufs, L. Rallidis, L. Bajnok, T. Gudjónsson, V. Maher, Y. Henkin, M. M. Gulizia, A. Mussagaliyeva, G. Bajraktari, A. Kerimkulova, G. Latkovskis, O. Hamoui, R. Slapikas, L. Visser, P. Dingli, V. Ivanov, A. Boskovic, M. Nazzi, F. Visseren, I. Mitevska, K. Retterstøl, P. Jankowski, R. Fontes-Carvalho, D. Gaita, M. Ezhov, M. Foscoli, V. Giga, D. Pella, Z. Fras, L. Perez de Isla, E. Hagström, R. Lehmann, L. Abid, O. Ozdogan, O. Mitchenko and R. S. Patel, 2019 ESC/EAS guidelines for the management of dyslipidaemias: Lipid modification to reduce cardiovascular risk, Atherosclerosis, 2019, 290, 140–205 CrossRef PubMed.
- C. Heiss, R. Sansone, H. Karimi, M. Krabbe, D. Schuler, A. Rodriguez-Mateos, T. Kraemer, M. M. Cortese-Krott, G. G. Kuhnle, J. P. Spencer, H. Schroeter, M. W. Merx and M. Kelm, Impact of cocoa flavanol intake on age-dependent vascular stiffness in healthy men: a randomized, controlled, double-masked trial, Age, 2015, 37, 9794 CrossRef PubMed.
- D. M. Lloyd-Jones, C. E. Lewis, P. J. Schreiner, J. M. Shikany, S. Sidney and J. P. Reis, The Coronary Artery Risk Development In Young Adults (CARDIA) Study: JACC Focus Seminar 8/8, J. Am. Coll. Cardiol., 2021, 78, 260–277 CrossRef PubMed.
- R. Dhingra and R. S. Vasan, Age as a risk factor, Med. Clin. North Am., 2012, 96, 87–91 CrossRef PubMed.
- B. J. North and D. A. Sinclair, The intersection between aging and cardiovascular disease, Circ. Res., 2012, 110, 1097–1108 CrossRef CAS PubMed.
- M. Han, R. Qie, X. Shi, Y. Yang, J. Lu, F. Hu, M. Zhang, Z. Zhang, D. Hu and Y. Zhao, Cardiorespiratory fitness and mortality from all causes, cardiovascular disease and cancer: dose-response meta-analysis of cohort studies, Br. J. Sports Med., 2022, 56, 733–739 CrossRef PubMed.
- D. Lazzeroni, A. Villatore, G. Souryal, G. Pili and G. Peretto, The Aging Heart: A Molecular and Clinical Challenge, Int. J. Mol. Sci., 2022, 23(24), 16033 CrossRef CAS PubMed.
- D. D. Christou and D. R. Seals, Decreased maximal heart rate with aging is related to reduced {beta}-adrenergic responsiveness but is largely explained by a reduction in intrinsic heart rate, J. Appl. Physiol., 2008, 105, 24–29 CrossRef PubMed.
- E. J. Howden, S. Sarma, J. S. Lawley, M. Opondo, W. Cornwell, D. Stoller, M. A. Urey, B. Adams-Huet and B. D. Levine, Reversing the Cardiac Effects of Sedentary Aging in Middle Age-A Randomized Controlled Trial: Implications For Heart Failure Prevention, Circulation, 2018, 137, 1549–1560 CrossRef CAS PubMed.
- H. E. Molmen, U. Wisloff, I. L. Aamot, A. Stoylen and C. B. Ingul, Aerobic interval training compensates age related decline in cardiac function, Scand. Cardiovasc. J., 2012, 46, 163–171 CrossRef PubMed.
- W. Bouaziz, T. Vogel, E. Schmitt, G. Kaltenbach, B. Geny and P. O. Lang, Health benefits of aerobic training programs in adults aged 70 and over: a systematic review, Arch. Gerontol. Geriatr., 2017, 69, 110–127 CrossRef PubMed.
- G. Huang, R. Wang, P. Chen, S. C. Huang, J. E. Donnelly and J. P. Mehlferber, Dose-response relationship of cardiorespiratory fitness adaptation to controlled endurance training in sedentary older adults, Eur. J. Prev. Cardiol., 2016, 23, 518–529 CrossRef PubMed.
- J. M. Letnes, I. Berglund, K. E. Johnson, H. Dalen, B. M. Nes, S. Lydersen, H. Viken, E. Hassel, S. Steinshamn, E. K. Vesterbekkmo, A. Støylen, L. S. Reitlo, N. Zisko, F. H. Bækkerud, A. R. Tari, J. E. Ingebrigtsen, S. B. Sandbakk, T. Carlsen, S. A. Anderssen, M. A. F. Singh, J. S. Coombes, J. L. Helbostad, Ø. Rognmo, U. Wisløff and D. Stensvold, Effect of 5 years of exercise training on the cardiovascular risk profile of older adults: the Generation 100 randomized trial, Eur. Heart J., 2022, 43, 2065–2075 CrossRef PubMed.
- K. Neukam, W. Stahl, H. Tronnier, H. Sies and U. Heinrich, Consumption of flavanol-rich cocoa acutely increases microcirculation in human skin, Eur. J. Nutr., 2007, 46, 53–56 CrossRef CAS PubMed.
- M. Yildiz, A. A. Oktay, M. H. Stewart, R. V. Milani, H. O. Ventura and C. J. Lavie, Left ventricular hypertrophy and hypertension, Prog. Cardiovasc. Dis., 2020, 63, 10–21 CrossRef PubMed.
- J. M. Kim and H. J. Heo, The roles of catechins in regulation of systemic inflammation, Food Sci. Biotechnol., 2022, 31, 957–970 CrossRef CAS PubMed.
- S. S. Franklin, W. t. Gustin, N. D. Wong, M. G. Larson, M. A. Weber, W. B. Kannel and D. Levy, Hemodynamic patterns of age-related changes in blood pressure. The Framingham Heart Study, Circulation, 1997, 96, 308–315 CrossRef CAS PubMed.
- M. A. Horn and A. W. Trafford, Aging and the cardiac collagen matrix: Novel mediators of fibrotic remodelling, J. Mol. Cell. Cardiol., 2016, 93, 175–185 CrossRef CAS PubMed.
- C. Heiss, S. Jahn, M. Taylor, W. M. Real, F. S. Angeli, M. L. Wong, N. Amabile, M. Prasad, T. Rassaf, J. I. Ottaviani, S. Mihardja, C. L. Keen, M. L. Springer, A. Boyle, W. Grossman, S. A. Glantz, H. Schroeter and Y. Yeghiazarians, Improvement of endothelial function with dietary flavanols is associated with mobilization of circulating angiogenic
cells in patients with coronary artery disease, J. Am. Coll. Cardiol., 2010, 56, 218–224 CrossRef CAS PubMed.
- M. Gröne, R. Sansone, P. Höffken, P. Horn, A. Rodriguez-Mateos, H. Schroeter, M. Kelm and C. Heiss, Cocoa Flavanols Improve Endothelial Functional Integrity in Healthy Young and Elderly Subjects, J. Agric. Food Chem., 2020, 68, 1871–1876 CrossRef PubMed.
- K. Wolhuter, S. M. Y. Kong, C. P. Stanley and J. C. Kovacic, The Role of Oxidants in Percutaneous Coronary Intervention-Induced Endothelial Dysfunction: Can We Harness Redox Signaling to Improve Clinical Outcomes?, Antioxid. Redox Signaling, 2023, 38, 1022–1040 CrossRef CAS PubMed.
- H. D. Sesso, J. E. Manson, A. K. Aragaki, P. M. Rist, L. G. Johnson, G. Friedenberg, T. Copeland, A. Clar, S. Mora, M. V. Moorthy, A. Sarkissian, W. R. Carrick and G. L. Anderson, Effect of cocoa flavanol supplementation for the prevention of cardiovascular disease events: the COcoa Supplement and Multivitamin Outcomes Study (COSMOS) randomized clinical trial, Am. J. Clin. Nutr., 2022, 115, 1490–1500 CrossRef PubMed.
- J. I. Ottaviani, M. Balz, J. Kimball, J. L. Ensunsa, R. Fong, T. Y. Momma, C. Kwik-Uribe, H. Schroeter and C. L. Keen, Safety and efficacy of cocoa flavanol intake in healthy adults: a randomized, controlled, double-masked trial, Am. J. Clin. Nutr., 2015, 102, 1425–1435 CrossRef CAS PubMed.
- S. Langer, L. J. Marshall, A. J. Day and M. R. Morgan, Flavanols and methylxanthines in commercially available dark chocolate: a study of the correlation with nonfat cocoa solids, J. Agric. Food Chem., 2011, 59, 8435–8441 CrossRef CAS PubMed.
- J. Vlachojannis, P. Erne, B. Zimmermann and S. Chrubasik-Hausmann, The Impact of Cocoa Flavanols on Cardiovascular Health, Phytother. Res., 2016, 30, 1641–1657 CrossRef CAS PubMed.
- I. Amoah, J. J. Lim, E. O. Osei, M. Arthur, P. Tawiah, I. N. Oduro, M. S. Aduama-Larbi, S. T. Lowor and E. Rush, Effect of Cocoa Beverage and Dark Chocolate Consumption on Blood Pressure in Those with Normal and Elevated Blood Pressure: A Systematic Review and Meta-Analysis, Foods, 2022, 11(13), 1962 CrossRef CAS PubMed.
|
This journal is © The Royal Society of Chemistry 2023 |