DOI:
10.1039/D3FO01599H
(Paper)
Food Funct., 2023,
14, 8217-8228
Impact of polyphenol oxidase on the bioavailability of flavan-3-ols in fruit smoothies: a controlled, single blinded, cross-over study†
Received
20th April 2023
, Accepted 8th August 2023
First published on 24th August 2023
Abstract
Flavan-3-ols are bioactive compounds found in a variety of fruits and vegetables (F&V) that have been linked to positive health benefits. Increasing habitual flavan-3-ol intake is challenged by the generally low consumption of F&V. While smoothies are a commonly endorsed, consumer-accepted means to increase the daily intake of these important foods, fruits used for smoothie preparation can have a high polyphenol oxidase (PPO) activity and thus potentially affect the content and bioavailability of flavan-3-ols. To assess whether or not consuming freshly prepared smoothies made with different PPO-containing fruit impacts the bioavailability of the flavan-3-ols, a controlled, single blinded and cross-over study was conducted in healthy men (n = 8) who consumed a flavan-3-ol-containing banana-based smoothie (high-PPO drink), a flavan-3-ol-containing mixed berry smoothie (low-PPO drink) and flavan-3-ols in a capsule format (control). The peak plasma concentration (Cmax) of flavan-3-ol metabolites after capsule intake was 680 ± 78 nmol L−1, which was similar to the levels detected after the intake of the low PPO drink. In contrast, the intake of the high PPO drink resulted in a Cmax of 96 ± 47 nmol L−1, 84% lower than that obtained after capsule intake. In a subsequent study (n = 11), flavan-3-ols were co-ingested with a high-PPO banana drink but contact prior to intake was prevented. In this context, plasma flavan-3-ol levels were still reduced, suggesting an effect possibly related to post-ingestion PPO activity degrading flavan-3-ols in the stomach. There was a substantial range in the PPO activity detected in 18 different fruits, vegetables and plant-derived dietary products. In conclusion, bioavailability of flavan-3-ols, and most likely other dietary polyphenol bioactives, can be reduced substantially by the co-ingestion of high PPO-containing products, the implications of which are of importance for dietary advice and food preparation both at home and in industrial settings.
1. Introduction
Flavan-3-ols, including the monomers (−)-epicatechin and (+)-catechin, and their related oligomeric derivatives, the procyanidins, are a group of plant-derived, dietary bioactives within the wider group of polyphenols. A growing body of evidence supports the notion that increasing habitual flavan-3-ol intake results in improvements in health, notably for cardiovascular health and cognitive function,1,2 with the Academy of Nutrition and Dietetics recently issuing a recommendation of a 400–600 mg daily consumption of flavan-3-ols for cardiometabolic protection.3 Dietary sources of flavan-3-ols include teas, cocoa-based products and a variety of fruits including apple, pear, berries, peaches and grapes.4–6 In this context, eating more flavan-3-ol-containing fruits would represent a valid means to increase intake while observing current dietary guidelines.7 However, the overall consumption of fruit and vegetables is below recommended levels despite a continuing effort to promote their intake.7,8 Smoothies are often promoted by various nutrition and health-focused organizations7,9,10 and are popular among consumers as a convenient approach to consuming the needed daily servings of fruit and vegetables and their derived nutrients.9,11,12 However, blending of fruits and vegetables to produce smoothies may influence the content of flavan-3-ols, and other polyphenols, due to the reaction with polyphenol oxidase (PPO).13–16 PPO has a well-documented role in enzymatic browning of fruit, vegetables, and grain products. This occurs to varying degrees during harvesting, transportation, storage, as well as food processing and preparation, affecting not only the sensory characteristics of foods but also their nutritional quality.13,17–19 Substantial efforts have been directed to developing novel methods for fruit and vegetable processing that mitigate the effects of PPO.13,17,20,21 However, while most improve sensory traits,22,23 little is known about the impact of PPO activity in freshly prepared smoothies on dietary bioactives, such as flavan-3-ols. Obtaining such information is important as it has implications for dietary advice, as well as considerations on how foods are processed and prepared.
After ingestion, flavan-3-ols are rapidly absorbed and extensively metabolized.24–26 Recent studies have shown that the intake of (−)-epicatechin results in more than 20 different metabolites appearing in the circulation relatively quickly after ingestion,27 with the glucuronidated, sulfated and methylated structurally-related (−)-epicatechin metabolites (SREMs) directly linked to the beneficial effects mediated by flavan-3-ol intake.28,29 Other flavan-3-ol metabolites include the gut microbiota-derived 5-(3′,4′-dihydroxyphenyl)-γ-valerolactone metabolites (γ-VLMs). There are many reports on the absorption, distribution, metabolism and excretion (ADME) of flavan-3-ols after the intake of isolated flavan-3-ols and the ingestion of simple flavan-3-ol-containing foods and beverages.27,30–32 However, little is known about the ADME of flavan-3-ols when consumed in a blended matrix such as a fruit smoothie. This work describes a dietary intervention study investigating how consuming smoothies made with different PPO-containing fruit impacts the bioavailability of the flavan-3-ols.
2. Methods and methods
2.1. Chemicals
Authentic chemically synthesized SREMs and γ-VLMs were provided by Mars, Inc. (McLean, VA, USA). HPLC grade water, methanol and acetonitrile were purchased from Fisher (Pittsburgh, PA, USA). (−)-Epicatechin, PPO inhibitors and other reagents for PPO activity assessment were purchased from Sigma-Aldrich (St Louis, MO, USA).
2.2. Subjects
Healthy male adults between 25 and 60 years of age were recruited by public advertisement in the city of Davis and surrounding areas (California, USA). Exclusion criteria included a body mass index (BMI) higher than 30 kg m−2, blood pressure (BP) higher than 140/90 mmHg, allergies to peanut or cocoa, avoidance of caffeinated food products and beverages, a history of CVD, stroke, renal, hepatic, or thyroid disease, gastrointestinal tract disorders, previous gastrointestinal surgery (except appendectomy), the current intake of herbal-, plant- or botanicals-containing dietary supplements, persons following a vegan/vegetarian diet, and those adhering to an uncommon diet or a weight loss program. To determine eligibility, participants were asked to complete health and lifestyle questionnaires, have their height, weight, and in-office BP determined, and to provide a blood sample for complete blood count, liver panel, lipid panel and metabolic panel assessments. Enrolled participants commenced the study protocol between 1–3 weeks after eligibility was determined. To control for flavan-3-ols from the diet, participants were asked to follow a low flavan-3-ol and procyanidin diet on the day before, and during the study days. All volunteers were instructed on how to follow the prescribed diet, receiving a list of foods low in flavan-3-ols and procyanidins (ESI Fig. 1†). Volunteers were asked to restrain from consuming alcohol, cocoa-based products, coffee or other caffeine-containing beverages on the day prior to and during the study visits. Volunteers were asked to fast for 12 h before each study day (water ad libitum).
2.3. Test foods and beverages
For study part 1, volunteers were asked to consume two different fruit smoothies with different PPO activities. The smoothies tested included: (i) a banana smoothie (serving: 417 mL), with a natural high PPO activity (8926 ± 389 IU mL−1), and (ii) a mixed berry smoothie (serving: 590 mL) with a natural low PPO activity (96 ± 5 IU mL−1). To standardize (−)-epicatechin content and minimize the different contributions of (−)-epicatechin from the fruits used in smoothie preparation, an (−)-epicatechin-containing standardized cocoa extract was added to each smoothie recipe in known amounts. In addition to smoothies, a control intervention consisting of the same (−)-epicatechin-containing standardized cocoa extract in capsule form was consumed with cow milk (1% fat). The banana smoothie was prepared with 177 g of ripe, frozen bananas and 240 g of almond milk. The mixed berry smoothie was prepared with 50 g each of strawberries, blueberries, blackberries and raspberries, 120 g of almond milk, 70 g of water, 105 g of crushed ice, and 95 g of yogurt. The contribution of berries to (−)-epicatechin content was estimated to be <20 mg based on USDA database for flavonoids.5 The content of macronutrients in the food matrices tested as well as the amount of (−)-epicatechin added is detailed in Table 1. Quantification of flavanols was done according to definition and method described by AOAC2020.05.33 Quantification of (−)-epicatechin was done according to AOAC2013.04.34 To maintain consistency with smoothie preparation and reduce to a minimum natural variations in fruit composition and maturity, each smoothie type was freshly prepared on two independent study days and consumed by volunteers within 1 h of preparation. This resulted in the administration of test material in a non-randomized manner in study part 1. In addition to these test materials, other flavan-3-ol-containing test foods were evaluated as part of study part 1, aiming at assessing the influence of a broad range of food matrixes on the bioavailability of flavan-3-ols. The characteristics of these additional test foods are shown in ESI.†
Table 1 Composition of macronutrients of food matrices tested and amount of flavan-3-ols, including (−)-epicatechin, added to fruit smoothies. Nutritional content was obtained from labels and from USDA Food Data Central
|
Capsule + milka |
Banana smoothie |
Mixed berry smoothie |
Nutritional composition of milk, 1% fat consumed with capsules. Capsules did not contribute to nutrient content. DP: degree of polymerization.
|
Energy (kcal) |
145 |
243 |
203 |
Protein (g) |
11 |
4 |
13 |
Fat (g) |
3 |
4 |
2 |
Carbohydrate (g) |
17 |
52 |
36 |
Fiber (g) |
0 |
7 |
9 |
Sugar (g) |
0 |
29 |
19 |
Flavan-3-ols added (mg) |
541 |
638 |
484 |
(−)-Epicatechin (mg) |
75 |
88 |
68 |
(+)-Epicatechin (mg) |
b.l.d. |
b.l.d. |
b.l.d. |
(−)-Catechin (mg) |
13 |
17 |
9 |
(+)-Catechin (mg) |
3 |
3 |
3 |
DP2 (mg) |
91 |
106 |
75 |
DP3 (mg) |
99 |
116 |
91 |
DP4 (mg) |
86 |
101 |
78 |
DP5 (mg) |
74 |
87 |
67 |
DP6 (mg) |
58 |
69 |
53 |
DP7 (mg) |
42 |
51 |
40 |
For study 2, test drinks resembled the composition of the banana-based smoothie used in study part 1 but prevented the exposure of the flavan-3-ol (−)-epicatechin with banana PPO prior to intake. These drinks were (i) a flavan-3-ol drink and a banana blend drink (FD + BD) that were prepared separately but consumed simultaneously by alternating sips from each drink; and (ii) a flavan-3-ol drink (FD) without any banana that served as a control. FD + BD were prepared by mixing 120 g of almond milk to which was added (−)-epicatechin in a standardized cocoa extract, and separately 177 g of ripe, frozen bananas with 120 g of almond milk. FD was prepared with 240 mL of almond milk and (−)-epicatechin from a standardized cocoa extract. Both drinks provided 88 mg (303 μmol) of (−)-epicatechin.
The standardized cocoa extract used in this study (Cocoapro™ cocoa extract) as a source of (−)-epicatechin was provided by Mars, Inc. (VA, USA). All foods and beverages, including bananas, berries, almond milk and yogurt were obtained from a local grocery store and products were stored according to manufacturer directions.
2.4. Study design
Studies were conducted at the Ragle Human Nutrition Research Center at the University of California, Davis. Study part 1 consisted of a cross-over, single-masked (researchers analyzing data), controlled, and single acute intake study, in the course of which participants were asked to consume the two different flavan-3-ol-containing smoothies and the flavan-3-ol-containing capsule on different days.
One hour before arrival at the research facility, participants were asked to consume 250 mL of water to control for equal levels of hydration. Upon arrival, volunteers were asked to provide a blood sample (baseline, 0 h) and immediately after were given the test materials that were consumed in less than 5 min. Additional blood samples were taken at 1 h, 2 h, 3 h, 4 h, 5 h and 6 h after test material ingestion. Two hours after intake of test materials, water was available for volunteers ad libitum. Between 4–5 h after intake, volunteers had a flavan-3-ol-free lunch. Study days were separated by a minimum of 6 days in which volunteers followed their habitual diet, thus eliminating any potential carry over effect from test material intake. The minimum sample size required to determine a change in SREM plasma levels after the intake of the test products versus control (flavan-3-ol capsules) was determined by paired t-test (n = 6, α = 0.05, power = 0.8), imputing an SD of 17% and a mean difference of a 25% in the concentration of SREMs in plasma.35Participants were assigned to different sequences to receive test materials. The initial recruitment started in May 2016, and the study was conducted from May to July 2016.
Study 2 consisted of a randomized, a cross-over, single-masked (researchers analyzing data), controlled and single acute intake study, during which participants were asked to consume a flavan-3-ol-containing drink with and without the simultaneous intake of a drink with blended banana. For the simultaneous intake of a flavan-3-ol-containing test drink and a blended banana drink (FD + BD), participants consumed the drink in 4 rounds, each round consisting of one sip from the flavan-3-ol-containing drink and one sip from the blended banana drink. In this manner, flavan-3-ols would not come into contact with banana-PPO before intake. The other procedures followed by volunteers during study part 2 were the same as those followed during study part 1, with the additional collection of urine samples from 12 h prior to and 0–24 h after test drink intake. Participants were randomly assigned to different sequences receiving FD and (FD + BD) interventions in alternate order. Interventions were randomly assigned to volunteers according to a computer-generated allocation list created by JLE, and recruitment and allocation of participants was conducted by JK. The minimum sample size required to determine a change in SREM plasma levels after the intake of the test products was determined by paired t-test (n = 8, α = 0.05, power = 0.8), imputing an SD of 17% and a mean difference of a 20% change in the concentration of SREMs in plasma.35 The recruitment for study 2 started in June 2017 and the study was completed within 6 weeks.
2.5. Research ethics
The study protocol was approved by the Institutional Review Board of the University of California, Davis. All subjects gave their written informed consent to participate. The trial was registered at clinicaltrials.gov as NCT03526094.
2.6. Quantification of flavan-3-ol metabolites in plasma and urine
Blood samples were collected using EDTA as anticoagulant to assess the SREMs in plasma over 6 h post-intake (primary readout of the study) and the gut microbiota-derived γ-VLMs (γ-VLM; secondary readout of the study). Plasma was obtained by whole blood centrifugation at 1800g for 15 min at 4 °C, separated into aliquots, and spiked with ascorbic acid (final concentration: 1 mg ml−1). Plasma samples were stored at −80 °C until analyzed. Briefly, plasma samples (300 μL) were spiked with 30 μl of 100 μmol L−1 4-nitrophenyl sulfate and transferred to a 2.5 mL Phree Phospholipid Removal cartridge and collection plate combination for protein precipitation and phospholipid removal (Phenomenex, Torrance, CA, USA). Samples were added with 1.0 mL of acetonitrile and then with 0.3 mL of methanol. After each solvent addition, samples were filtered via centrifugation at 400g for 15 min at 4 °C. The resulting samples were transferred to a 2.0 ml tube and volume was reduced to approximately 50 μL using a Speedvac®. Samples were then mixed with 100 μL of 100 μmol L−1 3-methyl hippuric acid, as an internal standard, and analyzed by UPLC-MS within 24 h.
Urine samples were collected to assess the amount of SREMs and γ-VLMs excreted over 24 h (secondary readouts of the study part 2). Briefly, 50 μL of urine was loaded onto an Impact Protein Precipitation cartridge (Phenomenex) along with 50 μL of 100 μmol L−1 3-methyl hippuric acid. The mixture was centrifuged at 500g for 15 min at 4 °C. The resulting sample was collected and stored in a 600 μL vial at −80 °C prior to analysis.
Samples were run on a Waters Acquity UPLC (Waters, Milford, MA, USA) with an autosampler cooled at 5 °C. The injection volume was 25 μL. Chromatography was carried out at 25 °C using a 100 × 2.1 mm Kinetex C18 1.7 μm, reversed phase column with a krudcatcher (Phenomenex). The mobile phase, pumped at a flow rate of 0.5 mL min−1, was (A) 5 mmol L−1 ammonium formate (pH 2.9); and (B) acetonitrile/methanol (90
:
10, v/v). The gradient started with 5% B from 0–2.0 min, ramped to 17.5% from 2.0–3.5 min, and then to 22.5% from 3.5–6.0 min. Detection was achieved with a Waters Quattro Micromass spectrometer fitted with an electrospray interface (ESI; Waters). MS analysis was carried out in negative ionization mode by multiple reaction monitoring (ESI Table 1†). Data processing was performed using Masslynx software (Waters). MS conditions were set by tuning with the corresponding compound. Source and desolvation temperature were set at 150 °C and 500 °C, respectively, while desolvation and cone gas were set at 900 L h−1 and 30 L h−1, respectively. Capillary, extractor, and lens voltage were set at 4 kV, 5 V, and 2 V, respectively. The identification and quantification of the compounds were based on co-elution with authentic standards. The accuracy and precision of the methods was >90% and <10% for all metabolites quantified, respectively (ESI Table 2†).
2.7. Quantification of (−)-epicatechin in banana smoothie
The banana smoothie was prepared as described above with water replacing the almond milk, and aliquots of 0.4 mL were incubated for 0, 5, 10, 15, 30 and 60 min at room temperature (n = 6). Incubation was concluded by addition of 1.4 mL ice cold 0.5% acetic acid in acetone. After 10 min of incubation on ice, samples were centrifuged (16
000g for 15 min at 4 °C) and the supernatant collected. The extraction was repeated on the pellet and the supernatants were combined, diluted 50% with 0.5% acetic acid in water prior to analysis by HPLC according to Ottaviani et al.36
2.8. Quantification of PPO activity and the (−)-epicatechin content of a banana smoothie after incubation in simulated gastric digestion conditions
To determine the change in PPO activity and (−)-epicatechin content in the smoothie under simulated gastric digestion conditions, 0.5 ml aliquots of banana smoothie were incubated in the presence of 2000 IU ml−1 of pepsin and 38.5 mmol L−1 NaCl at pH 3 adjusted with concentrated HCl (final volume of reaction, 1 mL). After incubation for 2 h at 37 °C (n = 5), samples were assayed to determine (−)-epicatechin content and PPO activity. The content of (−)-epicatechin in banana smoothie was determined as described above. PPO activity was measured according to the method of Liu et al.37 with some modifications, including the use of (−)-epicatechin as a substrate. Briefly, 20 μL of banana smoothie were added to 1.5 mL 200 mmol L−1 sodium phosphate solution (pH 7.4). A 1 mL aliquot of 3 mmol L−1 (−)-epicatechin (in 5% ethanol/95% water) was added to initiate the reaction, in a final reaction volume of 3 mL (i.e. 100 mmol L−1 sodium phosphate, 1 mmol L−1 (−)-epicatechin). Absorbance at wavelength 440 nm was measured continuously for 1 h at room temperature. Activity was determined from the slope of the plot of absorbance vs. incubation time and was calculated as 1 unit = absorbance of 0.001. Final values are expressed as units per ml of banana smoothie.
2.9. Polyphenol oxidase (PPO) activity in common fruits and vegetables used in smoothie preparation
Fruit and vegetable homogenates were prepared from three individual fruits or vegetables (bananas, apples, pears, pineapples, mangos, cucumbers), or three bunches (parsley, kale, wheatgrass, beets/beet greens) or three punnets (strawberries and blueberries) that were purchased from local grocery stores. The produce was first rinsed using local tap water and patted dry, and then peeled, pitted, hulled and/or chopped into smaller pieces. Following preparation, the products were vacuum sealed in freezer bags, and frozen at −20 °C for at least 12 hours prior to homogenization. Homogenates of each product were prepared by mixing frozen portions with bottled drinking water at a ratio of 1.4 g water per g frozen fruit or vegetable in a kitchen blender. The products were blended until smooth, separated in aliquots and stored at −80 °C until assay. Natural cocoa powder (Mars Cocoapro™, Mars Inc., USA) and Spirulina (Natural Elements US, Phoenix, AZ, USA) were prepared in deionized water on the day of assay.
PPO activity was measured as described above with slight modifications. In this assay, the reaction was carried out at 25 °C in a shaking water bath. At six different time points (time points varied according to PPO activity), 0.4 mL of the reaction mixture was removed and added to 0.4 ml of acetone and placed on ice. The mixture was centrifuged at 13
000g, at 4 °C for 15 min and the supernatant read spectrophotometrically at 440 nm. These steps were introduced to reduce the interference of detritus resulting from homogenized fruits and vegetables with absorbance determination. Activity values are expressed as units per 100 g fruit (fresh weight). Each fruit/vegetable homogenate was run in triplicate. Limit of detection was determined as three times the rate of autooxidation of (−)-epicatechin in reaction media without any fruit/vegetable homogenate.
2.10. Statistical analysis
Data are presented as mean values ± SEM. Normality was assessed using Kolmogorov–Smirnov test. Pharmacokinetic parameters were calculated by Non-compartmental Analysis using Microsoft Excel (Microsoft, Redmond, WA, USA) and included peak plasma concentration of SREMs (Cmax), time to reach Cmax (Tmax), apparent half-life of SREMs in plasma (AT1/2) and area-under-the-curve described by the concentration of SREMs in plasma over 6 h after intake (AUC0–6 h). We tested for differences in pharmacokinetic parameters obtained in part 1 of the study using a linear mixed model, with treatment (test materials) as fixed effect and subject and sequence in which test materials were received as random effects. This statistical analysis was selected to account for the non-randomized design in study part 1 in favor of maintaining the homogeneity in the fruits used for smoothie preparation. Adjustments for multiple comparison were not done. These analyses were conducted using R38 version 4.2.2, lme4 package39 and lmerTest.40 In study part 2, pharmacokinetic parameters were analyzed via a paired t-test. These statistical analyses were computed with SigmaStat 3.5 (Systat Software Inc., San Jose, CA, USA). P-Values of 0.05 or less were considered statistically significant.
3. Results
3.1. Study population
For study part 1, we assessed 8 volunteers for eligibility, and 8 volunteers were enrolled. The intervention with the flavan-3-ol-containing capsule was completed by 8 volunteers and the intervention with the two smoothies tested was completed by 6 volunteers each (ESI Fig. 2†). For study part 2, we assessed 11 volunteers for eligibility, and all were enrolled and completed the study with every intervention (ESI† Fig. 2†). Characteristics of the study population are presented in Table 2. Baseline measurements of plasma samples taken before the consumption of the test drinks on each study day demonstrated low or no detectable levels of flavan-3-ol metabolites (Fig. 1), indicating adherence with the low-flavan-3-ol diet as set forth in the study protocol. No adverse events were reported after the intake of these test materials.
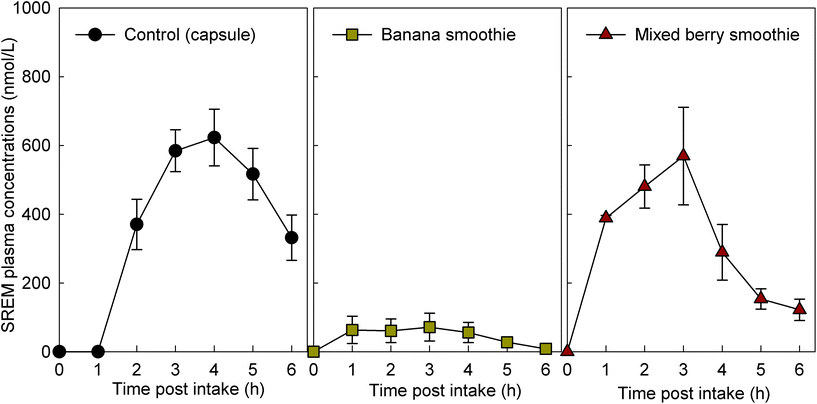 |
| Fig. 1 Concentration of structurally related (−)-epicatechin metabolites (SREMs) in plasma after the intake of flavan-3-ols in capsule format (n = 8) and flavan-3-ols mixed in different fruit smoothies (n = 6). Data are expressed as means ± SEM. | |
Table 2 Subject characteristics
Parameters |
Part 1 |
Part 2 |
Males (n) |
8 |
11 |
Age (years) |
30 ± 8 |
30 ± 7 |
Weight (kg) |
81 ± 8 |
78 ± 10 |
BMI (kg m−2) |
24 ± 2 |
28 ± 12 |
Systolic blood pressure (mmHg) |
119 ± 12 |
116 ± 9 |
Diastolic blood pressure (mmHg) |
74 ± 10 |
70 ± 8 |
Heart rate (bpm) |
66 ± 4 |
61 ± 10 |
Total cholesterol (mg dL−1) |
165 ± 46 |
174 ± 36 |
Glucose (mg dL−1) |
94 ± 3 |
90 ± 4 |
Creatinine (mg dL−1) |
0.90 ± 0.08 |
0.93 ± 15 |
Bilirubin (mg dL−1) |
1.1 ± 0.3 |
1.0 ± 0.2 |
White blood cell count (103 mm−3) |
5.3 ± 1.3 |
5.5 ± 1.3 |
Red blood cell count (106 mm−3) |
4.8 ± 0.2 |
5.1 ± 0.3 |
Hematocrit (%) |
44 ± 2 |
44 ± 1 |
3.2. Concentration of flavan-3-ol metabolites after the intake of flavan-3-ol-containing fruit smoothies
The intake of all fruit smoothies and the control intervention mixed with the flavan-3-ol-containing extract significantly increased the levels of SREMs in plasma (Fig. 1). However, ingestion of banana smoothie yielded plasma SREM levels that were significantly lower to those reached after the intake of the other test materials (Fig. 1). Most notably, intake of banana smoothie yielded Cmax, and AUC0–6 h values for SREMs that, respectively, were 84 ± 9% and 81 ± 11% lower than those obtained after intake of flavan-3-ol capsules (Table 3). Other minor, but statistically significant differences in Tmax of SREMs between capsules and smoothies were detected. In this case, the Tmax of SREMs after capsule intake was 1–2 h later than the Tmax obtained after the intake of smoothies (Table 3). No significant differences in the levels of SREMs were observed after the intake of additional test foods added with flavan-3-ols (ESI Results†).
Table 3 Pharmacokinetic parameters determined for the sum of structurally related (−)-epicatechin metabolites after the intake of flavan-3-ols in capsule format and mixed in fruit smoothies. Data presented as mean values ± SEM and analyzed following a linear mixed model, with treatment (test material) as fixed effect and subject and sequence as random effects. P-Values correspond to comparison between smoothie and capsule (control)a
Test products |
C
max
|
T
max
|
AT1/2 |
AUC0–6 h |
(nmol L−1) |
p
|
(h) |
p
|
(h) |
p
|
(nmol L−1) |
p
|
Not determined, n.d.; peak plasma concentration, Cmax, time to reach Cmax, Tmax, apparent elimination half-life, AT1/2; 0–6 h plasma area under the curve, AUC0–6 h.
|
Capsule (n = 8) |
680 ± 78 |
|
3.6 ± 0.2 |
|
2.4 ± 0.4 |
|
2259 ± 279 |
|
Banana smoothie (n = 6) |
96 ± 47 |
4 × 10−05 |
2.3 ± 0.7 |
0.002 |
n.d. |
n.d. |
272 ± 159 |
4 × 10−05 |
Mixed berry smoothie (n = 6) |
659 ± 104 |
0.818 |
2.2 ± 0.4 |
8 × 10−04 |
1.5 ± 0.2 |
0.08 |
1941 ± 294 |
0.362 |
Regardless of the test material consumed, the main SREMs detected in the circulation were (−)-epicatechin-3′-sulfate, (−)-epicatechin-3′-glucuronide, 3′-methoxy-(−)-epicatechin-5-sulfate and 3′-methoxy-(−)-epicatechin-7-sulfate. No significant differences in the profile of SREMs were observed between capsule and fruit smoothies (ESI Fig. 3†). In addition to SREMs, the gut microbiota-derived flavan-3-ol catabolites γVLMs were also detected in plasma. The plasma concentrations of γVLMs 6 h after intake were 342 ± 97 nmol L−1 (capsule), 194 ± 96 nmol L−1 (banana smoothie) and 600 ± 169 nmol L−1 (mixed berry smoothie). The main γVLMs were 5-(4′-hydroxyphenyl)-γ-valerolactone-3′-sulfate, 5-(4′-hydroxyphenyl)-γ-valerolactone-3′-glucuronide and 5-(3′-hydroxyphenyl)-γ-valerolactone-4′-glucuronide. There were no significant differences in the concentration and profile of γVLMs obtained between capsule and fruit smoothie intake.
3.3. Effect of banana-PPO on the bioavailability of flavan-3-ol prior to smoothie intake
Given the significant differences in SREM concentrations obtained after the intake of the banana smoothie and the intake of the rest of the test materials (Fig. 1 and Table 3), the level of (−)-epicatechin after banana smoothie preparation was evaluated to determine if the intrinsic PPO activity of banana could indeed alter the levels and, therefore, the amount of (−)-epicatechin available for absorption and metabolism before the ingestion of the smoothie. The results obtained showed a rapid decline in (−)-epicatechin levels in the banana smoothie over 1 h after preparation, with a half-life of 9.8 ± 2.1 min at room temperature (k = 0.071 ± 0.015 min−1) as illustrated in Fig. 2. The direct role of PPO in the decay of (−)-epicatechin was confirmed by addition of 1 mmol L−1 of three PPO inhibitors, namely phenylthiourea (competitive inhibitor of catechol substrate41), K2S2O5 (competitive inhibitor of O2 and reducing agent42) and (H4N)2MoS4 (copper chelator), all of which significantly reduced the disappearance of (−)-epicatechin when added into the banana smoothie (insert Fig. 2). Given the time-dependent effect of PPO on (−)-epicatechin levels in the banana smoothie, we explored if the magnitude of Cmax of SREMs obtained after the intake of banana smoothie in study part 1 was dependent on the time between smoothie preparation and intake (ESI Fig. 4†). Indeed, the results obtained showed a lower Cmax with increasing time between smoothie preparation and intake.
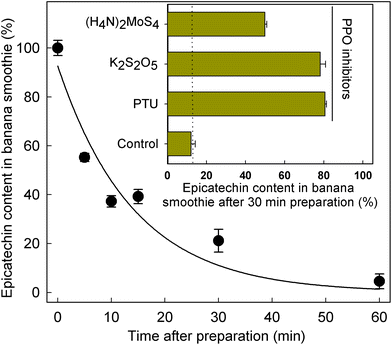 |
| Fig. 2 Decline in the amount of (−)-epicatechin in a blended banana drink mixed with flavan-3-ols after preparation. Data represent mean values ± SEM (n = 3). Insert: content of (−)-epicatechin in the blended banana drink prepared with flavan-3-ols 30 min after preparation when incubated in the absence and presence of 1 mmol L−1 concentrations of the polyphenol oxidase (PPO) inhibitors. Data are mean values ± SEM (n = 3) PTU: phenylthiourea. | |
3.4. Effect of banana-PPO on the bioavailability of flavan-3-ols after smoothie intake
To determine if banana-PPO could also affect the bioavailability of flavan-3-ols after ingestion, a subsequent study was conducted in which flavan-3-ols and banana-PPO were ingested as separated drinks but concomitantly (i.e., drink FD + BD), thus preventing any direct contact between flavan-3-ols and banana before ingestion. In addition, a flavan-3-ol drink (FD) without banana was included as a banana-PPO-free control. Concentration of SREMs in plasma after the intake of FD and FD + BD drink are shown in Fig. 3A. Consumption of the FD alone yielded a Cmax of 622 ± 115 nmol L−1 and an AUC0–6 h of 1702 ± 300 nmol L−1. In comparison, while the concomitant consumption of FD + BD did not significantly impact Cmax (429 ± 80 nmol L−1; p = n.s. vs. FD, paired-t test), there was a significant impact on AUC0–6 h values (988 ± 150 nmol L−1; p = 0.004 vs. FD, paired-t test) that were 37 ± 6% lower than those obtained after ingestion of FD alone. Reinforcing this observation, the amount of SREMs and γVLMs excreted in 0–24 h urine after FD + BD intake were, respectively, 41 ± 4% and 38 ± 6% lower than those obtained after FD intake (Fig. 3B). No differences in the profile of SREMs and γVLM in plasma and urine were detected between FD and FD + BD intake (ESI Fig. 5 and 6†).
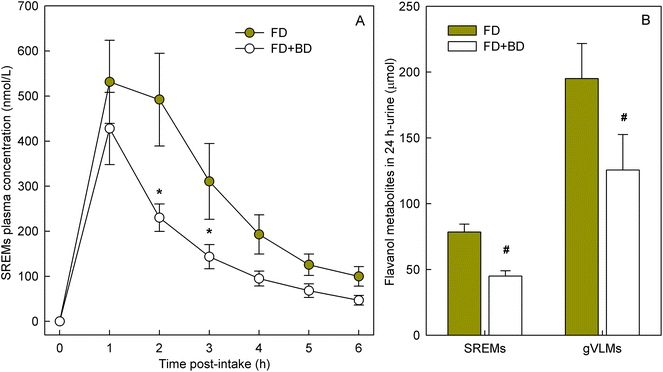 |
| Fig. 3 (A) Concentration of structurally related (−)-epicatechin metabolites (SREM) in plasma after the intake of a flavanol drink (FD) and a flavanol drink consumed together with a blended banana drink (FD + BD). Data are mean values ± SEM (n = 11). * p < 0.05 vs. control (FD); ANOVA-repeated measures; Bonferroni post hoc. (B) Amount of SREMs and 5-(3′,4′-dihydroxyphenyl)-γ-valerolactone metabolites (γVLM) excreted in urine 0–24 h after the intake of FD and FD + BD drinks. Data are mean ± SEM (n = 11). #Significant reduction after intake of FD + BD compared to FD drink, P < 0.05; paired t-test. | |
To evaluate if banana-PPO could react with (−)-epicatechin after intake during gastric digestion, banana smoothie was incubated in the presence of pepsin at pH 3 for 2 h at 37 °C to resemble gastric digestion conditions in vitro. The results obtained showed that PPO activity in banana smoothie was still present after the incubation under simulated gastric digestion conditions, maintaining 68 ± 5% of PPO activity present in the banana smoothie before ingestion (Fig. 4A). Similarly, (−)-epicatechin content was assessed before and after simulated gastric digestion conditions. The results obtained showed a significant decrease in (−)-epicatechin content in the banana smoothie, which was equivalent to a reduction of 28 ± 3% of the initial amount of (−)-epicatechin in the smoothie (Fig. 4B).
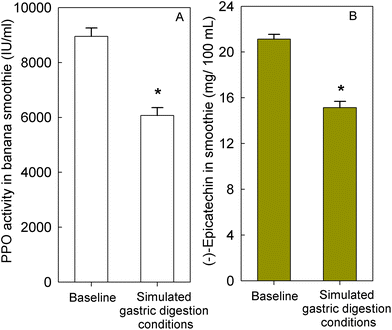 |
| Fig. 4 (A) Polyphenol oxidase (PPO) activity and (B) (−)-epicatechin in banana smoothie before and after incubation under simulated gastric digestion conditions. Simulated gastric digestion conditions consisted of an incubation at pH 3 in the presence of 2000 IU mL−1 of pepsin for 2 h at 37 °C. PPO activity was measured at pH 7.4 as described in the Materials and methods section. Data are mean ± SEM (n = 5). *P < 0.001 vs. baseline; t-test. | |
3.5. Polyphenol oxidase activity in plant-derived dietary products
Since PPO occurs widely in fruits and vegetables,13 the in vitro activity of the enzyme in different fresh fruit and other plant-derived ingredients used for smoothie preparation was investigated using (−)-epicatechin as the substrate. The results obtained are presented in Table 4. Given that some of the fruits and plant-derived ingredients tested are also important sources of flavan-3-ols in the diet, the expected amount of (−)-epicatechin provided by these food items is also reported (Table 4). In addition to banana, leaves of beetroot and pome fruits were the foods with the highest PPO activity, and all had the ability to use (−)-epicatechin as an enzyme substrate.
Table 4 Polyphenol oxidase (PPO) activity and (−)-epicatechin content of selected fruits and vegetables with relevance for smoothie preparation. PPO activity expressed as mean ± SEM (n = 3). Epicatechin content was obtained from the USDA database for the flavonoid content of selected foods, release 3.2 (2015)5 and data are expressed as means values (minimum and maximum)a
Products |
PPO activity (KU/100 g of edible portion) |
(−)-Epicatechin (mg per 100 g of edible portion) |
Below level of detection, b.l.d; not available, n/a.
|
Banana |
3258 ± 71 |
0.2 (0–0.07) |
Beet greens |
1594 ± 24 |
n/a |
Apple (red delicious) |
570 ± 27 |
9.83 (0.8–15.92) |
Pear |
147 ± 4 |
3.76 (0.1–17.74) |
Beets |
94 ± 5 |
0 (0–0) |
Peach |
41 ± 2 |
2.34 (0–6.92) |
Avocado |
24 ± 5 |
0.37 (0–1.11) |
Strawberry |
18 ± 1 |
1.56 (1.11–2.89) |
Wheatgrass |
15 ± 1 |
n/a |
Blueberry, highbush |
12 ± 1 |
0.62 (0–3.29) |
Cucumber |
10 ± 1 |
0 (0–0) |
Parsley |
6 ± 1 |
n/a |
Mango |
6 ± 1 |
0 (0–0) |
Cocoa powder, unsweetened |
b.l.d. |
196 (45–158) |
Orange |
b.l.d. |
0 (0–0) |
Pineapple |
b.l.d. |
0 (0–0) |
Kale |
b.l.d. |
n/a |
Spirulina (powder) |
b.l.d. |
n/a |
4. Discussion
The results obtained showed that (i) the intake of flavan-3-ols from a high PPO banana smoothie, but not a low PPO mixed berry smoothie, significantly reduced the levels of flavan-3-ol metabolites in circulation (Fig. 1); (ii) after banana smoothie preparation, flavan-3-ol levels rapidly declined in vitro, and the decrease was prevented by PPO inhibition (Fig. 2); and (iii) while preventing direct PPO contact in the food preparation did lessen some of the flavan-3-ol loss, it was insufficient to fully prevent the loss of flavan-3-ols when co-ingested (Fig. 3). Taken together, these results show the direct role of PPO in foods influencing the systemic bioavailability of flavan-3-ols pre- and post-ingestion. Considering that PPO is widely distributed in fruits and vegetables commonly used for smoothie preparation and readily able to use a flavan-3-ol, such as (−)-epicatechin, as a substrate (Table 4), it is possible to extend this observation to suggest that drinks, and potentially other food preparations made with blended, pureed, or pulverized fruits and vegetables containing high PPO activity, could also limit the bioavailability of flavan-3-ols and, potentially, other polyphenolic bioactives.
The effects of PPO on polyphenol oxidation are well-documented and are often controlled during handling and industrial processing to prevent browning.13,17,20,21 Outside of these processes, the impact of PPO activity is generally not considered. In home preparation and “fresh-take-away” foods purchased by consumers, the impact of PPO activity on the nutritional quality of foods may be quite significant and, thus relevant to health. Overall, the findings in this study are in line with the extensive literature characterizing PPO activity, with the current data pointing at the specific interaction between PPO and flavan-3-ols prior to ingestion as the main driver for the low bioavailability of flavan-3-ols from banana smoothies. However, it is of note that in study part 2, preventing the mixture of banana and flavan-3-ols prior to intake also resulted in a reduction in flavan-3-ol bioavailability compared to the ingestion of flavan-3-ols without any banana (Fig. 3). Furthermore, PPO activity in the banana smoothie remained active under simulated gastric digestion conditions, which was sufficient to decrease the (−)-epicatechin content to an extent comparable to the difference in (−)-epicatechin bioavailability when consuming FD and FD + BD drinks (Fig. 4). Thus, it is possible that PPO in banana could still play a role in affecting flavan-3-ol bioavailability, even post-intake. Previous studies conducted in humans showed that acidity in the stomach rapidly changes from pH of 2 to pH of 5 after consumption of a meal, requiring more than 1 h to return to a pH of 2.43–45 Precisely, a pH range between pH 4–5 is compatible with banana PPO activity,15,16 for which PPO during early phases of gastric digestion could have even a higher activity than the one tested at pH 3 in this study (Fig. 4). In addition, PPO is known to be present in certain plant species in a less active form namely latent PPO that can be activated by proteolysis.46,47 Previous studies showed that PPO in banana is present in a latent form,16 and it is thus possible that, during the initial phases of digestion, it is activated by pepsin to increase PPO activity. While considering the activity of PPO after intake could be counterintuitive, there are other enzymes known to remain active post-intake. For instance, certain formulations of lactase, supplemented in cases of lactose intolerance, and myrosinase, the enzyme involved in the release of sulforaphane from glucosinolates in cruciferous vegetables, are also expected to remain active after ingestion.48–50 Taken together, reaction of PPO with flavan-3-ols in the stomach is feasible and indicates that PPO activity in a food can have an impact on flavan-3-ol stability/bioavailability during the initial stages of digestion.
The concentration and profile of SREMs detected after the ingestion of flavan-3-ol-containing smoothies are in agreement with those reported in other studies in which flavan-3-ols were administered either as isolated compounds or from other flavan-3-ol-containing foods.27,30–32,51 In addition to the differences in AUC and Cmax due to banana-PPO, a difference in Tmax was also observed between smoothies and flavan-3-ol capsules (control, Table 2). Rather than an effect mediated by fruit smoothies, this difference in Tmax can be explained by the intake of flavan-3-ols in a capsule format. Prior to absorption, additional time is required for flavan-3-ols to be released from capsules due to disintegration of the capsule shell and dissolution of flavan-3-ol extract, which results in delayed absorption and an increase in Tmax
52 compared to the time required when consuming materials in which flavan-3-ols are already in solution. In addition, variations in viscosity of smoothies could explain differences in the kinetics obtained with the different drinks tested in study part 1 and study part 2. One limitation of the study consisted in the addition of flavan-3-ols to fruit smoothies to standardized content of these bioactives. In this context, it is possible that the reactivity of PPO with added flavan-3-ols dissolved in the drink presents differences with flavan-3-ols intrinsically present in the fruit. However, homogenization of fruit as occurring during blending could certainly increase bioaccessibility of flavan-3-ols intrinsically present in the fruit similar to those of added flavan-3-ols. While this study focused on the effect of smoothies and PPO on (−)-epicatechin and its metabolites, it is very likely that PPO also affected the levels of other flavan-3-ols including procyanidins. Further studies will be needed to understand the effect of PPO on the rest of flavan-3-ols besides (−)-epicatechin. Finally, it is worth noting that this study was conducted in male participants, thus representing another limitation of the study. While there is limited information regarding sex-based differences in flavan-3-ol absorption and metabolism, it is possible that the findings presented in this work are also relevant for women as the major effects mediated by PPO took place prior intake.
The reactivity of flavan-3-ols with PPO is based on the catechol group in the B-ring, which is a feature widely found in other polyphenols,53 and the description of PPO reaction with various other polyphenols has been described.54 Thus, blending of fruit during smoothie preparation could affect not only flavan-3-ol content but also the stability and bioavailability of other dietary polyphenols with a similar chemical structure. The oxidation product of catechol-bearing polyphenols and PPO results in the formation of o-quinone, a highly reactive molecule. For instance, o-quinones are involved in the oxidative polymerization of flavan-3-ols that results in the formation of theaflavins and thearubigins as part of black tea processing.14 These products of PPO reaction with flavan-3-ols are thought to have a limited bioavailability.25 In addition, o-quinone has been shown to enter redox reactions with ascorbic acid and electrophilic additions with amino acids like cysteine.55,56 Thus, PPO and polyphenol reactions will also affect the content of nutrients beyond polyphenols. In contrast, smoothies prepared from fruits and vegetables with a low PPO content offer an effective means to circumvent PPO-mediated losses in flavan-3-ol and polyphenol content. This is apparent in the good retention of flavan-3-ols following the preparation and intake of a smoothie prepared with mixed berries with low intrinsic PPO (Table 4). While the results obtained in this study are applicable for freshly prepared smoothies, it also opens the question to what extent PPO reaction with flavan-3-ols and polyphenols occurs during processing of ready-to-drink, bottled smoothies, especially in view of the speed at which the loss of (−)-epicatechin occurred in the presence of high PPO activity (Fig. 2). PPO has been a central consideration when developing commercial processes for the manufacture of fruit- and vegetable-based products.13,17,20,21 However, given that fruits and vegetables are common ingredients of meals prepared at home, the influence of intrinsic PPO activity on even more moderate forms of food preparation, and the timing of consumption could have major implications for the bioavailability of nutrients and bioactives. The assessment of changes in flavan-3-ols and polyphenol content, in general, and subsequent impact on bioavailability, however, are not commonly considered. This is particularly relevant in the context of nutrition research, when considering current survey-based methods for diet assessment that may not capture key aspects about fruit preparation, particularly when assessing intake of flavan-3-ol and other polyphenolic bioactives.
As the interest in the health benefits of dietary flavan-3-ols and polyphenols grows, this study highlights that consideration needs to be given not only to the types of fruits and vegetables and plant-based products to recommend to increase intake, but also how they are prepared, stored, and consumed as part of a regular meal in order to maximize their potential to support health. Currently, there is a dearth of information on the topic.
Abbreviations
γ-VLMs | 5-(3′,4′-Dihydroxyphenyl)-γ-valerolactone metabolites |
ADME | Absorption, distribution, metabolism and excretion |
AUC0–6 h | Area under the curve described by the concentration of SREMs in plasma over 6 h after intake |
AUC0-inf | Area under the curve described by the concentration in plasma extrapolated to infinity |
BMI | Body mass index |
BP | Blood pressure |
BW | Body weight |
FD | Flavan-3-ol-containing drink |
FD + BD | Flavan-3-ol drink and a banana blend drink |
C
max
| Peak plasma concentration |
AT1/2 | Plasma apparent half-life |
T
max
| Time to reach Cmax |
SREMs | Structurally related (−)-epicatechin metabolites |
PPO | Polyphenol oxidase |
Author contributions
JO, AC, HS and CKU designed the study; JLE, JK and VM conducted dietary intervention studies; RF, JLE, GGCK and JO processed samples and analyzed data; JO, AC and CKU wrote the paper and had primary responsibility for final content. All authors have read and approved the final manuscript.
Conflicts of interest
JIO, HS and CKU are employed by Mars, Inc., a company with long-term research and commercial interests in flavan-3-ols and procyanidins. AC has consulted for Mars Inc. and other food companies and government agencies with an interest in health and nutrition, as well as in phytonutrients, including flavan-3-ols and procyanidins. GGCK has received an unrestricted research grant from Mars, Inc. JLE, RYF, JK and VM declare no conflict of interest.
Acknowledgements
We thank Elaine North, RND (University California Davis), for her assistance with conducting the study, and Avani Sanghvi (Mars, Inc.) for characterizing cocoa flavan-3-ols used in this study. The study was funded by an unrestricted grant from Mars, Inc.
References
- H. D. Sesso, J. E. Manson, A. K. Aragaki, P. M. Rist, L. G. Johnson, G. Friedenberg, T. Copeland, A. Clar, S. Mora, M. V. Moorthy, A. Sarkissian, W. R. Carrick, G. L. Anderson and COSMOS Research Group, Effect of cocoa flavanol supplementation for the prevention of cardiovascular disease events: the COcoa Supplement and Multivitamin Outcomes Study (COSMOS) randomized clinical trial, Am. J. Clin. Nutr., 2022, 115, 1490–1500 CrossRef PubMed.
- R. P. Sloan, M. Wall, L. K. Yeung, T. Feng, X. Feng, F. Provenzano, H. Schroeter, V. Lauriola, A. M. Brickman and S. A. Small, Insights into the role of diet and dietary flavanols in cognitive aging: results of a randomized controlled trial, Sci. Rep., 2021, 11, 3837 CrossRef CAS PubMed.
- K. M. Crowe-White, L. W. Evans, G. G. C. Kuhnle, D. Milenkovic, K. Stote, T. Wallace, D. Handu and K. E. Senkus, Flavan-3-ols and Cardiometabolic Health: a Guideline Recommendation by the Academy of Nutrition and Dietetics, Adv. Nutr., 2022, 13, 2070–2083 CrossRef PubMed.
-
S. Bhagwat and D. B. Haytowitz, USDA database for the proanthocyanidin content of selected foods, release 2, 2015 (accessed October 2022).
-
S. Bhagwat and D. B. Haytowitz, USDA database for the flavonoid content of selected foods. Release 3.2., 2015 (accessed October 2022).
- V. Neveu, J. Perez-Jimenez, F. Vos, V. Crespy, L. du Chaffaut, L. Mennen, C. Knox, R. Eisner, J. Cruz, D. Wishart and A. Scalbert, Phenol-Explorer: an online comprehensive database on polyphenol contents in foods, Database, 2010, 2010, bap024 CrossRef CAS PubMed.
-
USDA and HHS, 2020–2025 Dietary Guidelines for Americans, 9th edn, 2020 Search PubMed, Available at https://www.DietaryGuidelines.gov. (accessed October 2022).
- S. S. Casagrande, Y. Wang, C. Anderson and T. L. Gary, Have Americans increased their fruit and vegetable intake? The trends between 1988 and 2002, Am. J. Prev. Med., 2007, 32, 257–263 CrossRef PubMed.
- E. Derbyshire, Where are we with smoothies? A eview of the latest guidelines, nutritional gaps and evidence, J. Nutr. Food Sci., 2017, 7, 632 Search PubMed.
- U. National Health Service, 5 A Day portion sizes. Available at https://www.nhs.uk/live-well/eat-well/5-a-day/portion-sizes/, (accessed October 2022).
- C. Myers, D. McCartney, B. Desbrow, S. Khalesi and C. Irwin, Consumption of a smoothie or cereal-based breakfast: impact on thirst, hunger, appetite and subsequent dietary intake, Int. J. Food Sci. Nutr., 2021, 72, 123–133 CrossRef CAS PubMed.
- B. Y. Rollins, W. Stein, K. L. Keller and J. S. Savage, Preschoolers will drink their GREENS! Children accept, like, and drink novel smoothies containing dark green vegetables (DGVs), Appetite, 2021, 162, 105148 CrossRef PubMed.
- M. E. Almeida and J. N. Nogueira, The control of polyphenol oxidase activity in fruits and vegetables. A study of the interactions between the chemical compounds used and heat treatment, Plant Foods Hum. Nutr., 1995, 47, 245–256 CrossRef CAS PubMed.
-
E. Haslam, Practical polyphenolics: from structure to molecular recognition and physiological action, Cambridge University Press, Cambridge, UK, 1998 Search PubMed.
- C. P. Yang, S. Fujita, M. Ashrafuzzaman, N. Nakamura and N. Hayashi, Purification and characterization of polyphenol oxidase from banana (Musa sapientum L.) pulp, J. Agric. Food Chem., 2000, 48, 2732–2735 CrossRef CAS PubMed.
- M. M. Sojo, E. Nuñez-Delicado, F. García-Carmona and A. Sánchez-Ferrer, Monophenolase Activity of Latent Banana Pulp Polyphenol Oxidase, J. Agric. Food Chem., 2008, 46, 4931–4936 CrossRef.
- K. M. Moon, E. B. Kwon, B. Lee and C. Y. Kim, Recent Trends in Controlling the Enzymatic Browning of Fruit and Vegetable Products, Molecules, 2020, 25, 2754 CrossRef CAS PubMed.
- A. M. Mayer, Polyphenol oxidases in plants and fungi: going places? A review, Phytochemistry, 2006, 67, 2318–2331 CrossRef CAS PubMed.
- C. Queiroz, M. L. Mendes Lopes, E. Fialho and V. L. Valente-Mesquita, Polyphenol Oxidase: Characteristics and Mechanisms of Browning Control, Food Rev. Int., 2008, 24, 361–375 CrossRef CAS.
- S. Zolghadri, A. Bahrami, M. T. Hassan Khan, J. Munoz-Munoz, F. Garcia-Molina, F. Garcia-Canovas and A. A. Saboury, A comprehensive review on tyrosinase inhibitors, J. Enzyme Inhib. Med. Chem., 2019, 34, 279–309 CrossRef CAS PubMed.
- N. Hamdan, C. H. Lee, S. L. Wong, C. Fauzi, N. M. A. Zamri and T. H. Lee, Prevention of Enzymatic Browning by Natural Extracts and Genome-Editing: A Review on Recent Progress, Molecules, 2022, 27, 1101 CrossRef CAS PubMed.
- X. Fan, Chemical inhibition of polyphenol oxidase and cut surface browning of fresh-cut apples, Crit. Rev. Food Sci. Nutr., 2022, 1–15, DOI:10.1080/10408398.2022.2061413.
-
A. Jukanti, Polyphenol Oxidase(s): Importance in Food Industry, in Polyphenol Oxidases (PPO) in Plants, Springer Nature, Singapore, 2017, pp. 93–106 Search PubMed.
- G. Borges, J. I. Ottaviani, J. J. J. van der Hooft, H. Schroeter and A. Crozier, Absorption, metabolism, distribution and excretion of (-)-epicatechin: A review of recent findings, Mol. Aspects Med., 2018, 61, 18–30 CrossRef CAS PubMed.
- M. N. Clifford, J. J. van der Hooft and A. Crozier, Human studies on the absorption, distribution, metabolism, and excretion of tea polyphenols, Am. J. Clin. Nutr., 2013, 98, 1619S–1630S CrossRef CAS PubMed.
- D. Del Rio, A. Rodriguez-Mateos, J. P. Spencer, M. Tognolini, G. Borges and A. Crozier, Dietary (poly)phenolics in human health: structures, bioavailability, and evidence of protective effects against chronic diseases, Antioxid. Redox Signal., 2013, 18, 1818–1892 CrossRef CAS PubMed.
- J. I. Ottaviani, G. Borges, T. Y. Momma, J. P. Spencer, C. L. Keen, A. Crozier and H. Schroeter, The metabolome of [2-(14)C](-)-epicatechin in humans: implications for the assessment of efficacy, safety, and mechanisms of action of polyphenolic bioactives, Sci. Rep., 2016, 6, 29034 CrossRef CAS PubMed.
- H. Schroeter, C. Heiss, J. Balzer, P. Kleinbongard, C. L. Keen, N. K. Hollenberg, H. Sies, C. Kwik-Uribe, H. H. Schmitz and M. Kelm, (-)-Epicatechin mediates beneficial effects of flavanol-rich cocoa on vascular function in humans, Proc. Natl. Acad. Sci. U. S. A., 2006, 103, 1024–1029 CrossRef CAS PubMed.
- C. Heiss, H. Schroeter, J. Balzer, P. Kleinbongard, S. Matern, H. Sies and M. Kelm, Endothelial function, nitric oxide, and cocoa flavanols, J. Cardiovasc. Pharmacol., 2006, 47(Suppl 2), S128–S135 CrossRef CAS PubMed ; discussion S172–126.
- J. I. Ottaviani, T. Y. Momma, G. K. Kuhnle, C. L. Keen and H. Schroeter, Structurally related (-)-epicatechin metabolites in humans: Assessment using de novo chemically synthesized authentic standards, Free Radical Biol. Med., 2012, 52, 1403–1412 CrossRef CAS PubMed.
- A. Stalmach, W. Mullen, H. Steiling, G. Williamson, M. E. Lean and A. Crozier, Absorption, metabolism, and excretion of green tea flavan-3-ols in humans with an ileostomy, Mol. Nutr. Food Res., 2010, 54, 323–334 CrossRef CAS PubMed.
- W. J. Hollands, S. Voorspoels, G. Jacobs, K. Aaby, A. Meisland, R. Garcia-Villalba, F. Tomas-Barberan, M. K. Piskula, D. Mawson, I. Vovk, P. W. Needs and P. A. Kroon, Development, validation and evaluation of an analytical method for the determination of monomeric and oligomeric procyanidins in apple extracts, J. Chromatogr., A, 2017, 1495, 46–56 CrossRef CAS PubMed.
- U. Bussy, G. Hewitt, Y. Olanrewaju, B. R. May, N. Anderson, J. I. Ottaviani and C. Kwik-Uribe, Single-Laboratory Validation for the Determination of Cocoa Flavanols and Procyanidins (by Degree of Polymerization DP1–7) in Cocoa-Based Products by Hydrophilic Interaction Chromatography Coupled with Fluorescence Detection: First Action 2020.05, J. AOAC Int., 2021, 104, 413–421 CrossRef PubMed.
- P. R. Machonis, M. A. Jones, B. T. Schaneberg, C. L. Kwik-Uribe and D. Dowell, Method for the determination of catechin and epicatechin enantiomers in cocoa-based ingredients and products by high-performance liquid chromatography: First Action 2013.04, J. AOAC Int., 2014, 97, 506–509 CrossRef CAS PubMed.
- A. Rodriguez-Mateos, T. Cifuentes-Gomez, I. Gonzalez-Salvador, J. I. Ottaviani, H. Schroeter, M. Kelm, C. Heiss and J. P. Spencer, Influence of age on the absorption, metabolism, and excretion of cocoa flavanols in healthy subjects, Mol. Nutr. Food Res., 2015, 59, 1504–1512 CrossRef CAS PubMed.
- J. I. Ottaviani, T. Y. Momma, C. Heiss, C. Kwik-Uribe, H. Schroeter and C. L. Keen, The stereochemical configuration of flavanols influences the level and metabolism of flavanols in humans and their biological activity in vivo, Free Radical Biol. Med., 2011, 50, 237–244 CrossRef CAS PubMed.
- L. Liu, S. Cao, B. Xie, Z. Sun, X. Li and W. Miao, Characterization of polyphenol oxidase from litchi pericarp using (-)-epicatechin as substrate, J. Agric. Food Chem., 2007, 55, 7140–7143 CrossRef CAS PubMed.
-
R Core Team, R: A language and environment for statistical computing, R Foundation for Statistical Computing, Vienna, Austria, 2022, https://www.R-project.org/ Search PubMed.
- D. Bates, M. Mächler, B. Bolker and S. Walker, Fitting Linear Mixed-Effects Models Using lme4, J. Stat. Softw., 2015, 67, 1–48 Search PubMed.
- A. Kuznetsova, P. B. Brockhoff and R. H. B. Christensen, lmerTest Package: Tests in Linear Mixed Effects Models, J. Stat. Softw., 2017, 82, 1–26 Search PubMed.
- A. D. Ryazanova, A. A. Alekseev and I. A. Slepneva, The phenylthiourea is a competitive inhibitor of the enzymatic oxidation of DOPA by phenoloxidase, J. Enzyme Inhib. Med. Chem., 2012, 27, 78–83 CrossRef CAS PubMed.
- E. Valero, R. Varon and F. Garciacarmona, Kinetic-Study of the Effect of Metabisulfite on Polyphenol Oxidase, J. Agric. Food Chem., 1992, 40, 904–908 CrossRef CAS.
- J. R. Malagelada, G. F. Longstreth, W. H. Summerskill and V. L. Go, Measurement of gastric functions during digestion of ordinary solid meals in man, Gastroenterology, 1976, 70, 203–210 CrossRef CAS.
- L. Y. Rios, R. N. Bennett, S. A. Lazarus, C. Remesy, A. Scalbert and G. Williamson, Cocoa procyanidins are stable during gastric transit in humans, Am. J. Clin. Nutr., 2002, 76, 1106–1110 CrossRef CAS PubMed.
- V. Tyssandier, E. Reboul, J. F. Dumas, C. Bouteloup-Demange, M. Armand, J. Marcand, M. Sallas and P. Borel, Processing of vegetable-borne carotenoids in the human stomach and duodenum, Am. J. Physiol.: Gastrointest. Liver Physiol., 2003, 284, G913–G923 CrossRef CAS PubMed.
- M. L. Sullivan and J. L. Foster, Perennial peanut (Arachis glabrata Benth.) contains polyphenol oxidase (PPO) and PPO substrates that can reduce post-harvest proteolysis, J. Sci. Food Agric., 2013, 93, 2421–2428 CrossRef CAS PubMed.
- F. Gandia-Herrero, M. Jimenez-Atienzar, J. Cabanes, F. Garcia-Carmona and J. Escribano, Evidence for a common regulation in the activation of a polyphenol oxidase by trypsin and sodium dodecyl sulfate, Biol. Chem., 2005, 386, 601–607 CAS.
- J. W. Fahey, K. L. Wade, K. K. Stephenson, A. A. Panjwani, H. Liu, G. Cornblatt, B. S. Cornblatt, S. L. Ownby, E. Fuchs, W. D. Holtzclaw and L. J. Cheskin, Bioavailability of Sulforaphane Following Ingestion of Glucoraphanin-Rich Broccoli Sprout and Seed Extracts with Active Myrosinase: A Pilot Study of the Effects of Proton Pump Inhibitor Administration, Nutrients, 2019, 11, 1489 CrossRef CAS PubMed.
- G. Ianiro, S. Pecere, V. Giorgio, A. Gasbarrini and G. Cammarota, Digestive Enzyme Supplementation in Gastrointestinal Diseases, Curr. Drug Metab., 2016, 17, 187–193 CrossRef CAS PubMed.
- M. A. Prieto, C. J. Lopez and J. Simal-Gandara, Glucosinolates: Molecular structure, breakdown, genetic, bioavailability, properties and healthy and adverse effects, Adv. Food Nutr. Res., 2019, 90, 305–350 CAS.
- L. Actis-Goretta, A. Leveques, F. Giuffrida, F. Romanov-Michailidis, F. Viton, D. Barron, M. Duenas-Paton, S. Gonzalez-Manzano, C. Santos-Buelga, G. Williamson and F. Dionisi, Elucidation of (-)-epicatechin metabolites after ingestion of chocolate by healthy humans, Free Radical Biol. Med., 2012, 53, 787–795 CrossRef CAS PubMed.
- N. Glube, L. Moos and G. Duchateau, Capsule shell material impacts the in vitro disintegration and dissolution behaviour of a green tea extract, Results Pharma Sci., 2013, 3, 1–6 CrossRef PubMed.
- A. Schieber, Reactions of Quinones-Mechanisms, Structures, and Prospects for Food Research, J. Agric. Food Chem., 2018, 66, 13051–13055 CrossRef CAS PubMed.
- A. G. Matthew and H. A. Parpia, Food browning as a polyphenol reaction, Adv. Food Res., 1971, 19, 75–145 Search PubMed.
- S. Bittner, When quinones meet amino acids: chemical, physical and biological consequences, Amino Acids, 2006, 30, 205–224 CrossRef CAS PubMed.
- M. Friedman, Food browning and its prevention: An overview, J. Agric. Food Chem., 1996, 44, 631–653 CrossRef CAS.
|
This journal is © The Royal Society of Chemistry 2023 |
Click here to see how this site uses Cookies. View our privacy policy here.