DOI:
10.1039/D3FO01116J
(Paper)
Food Funct., 2023,
14, 4824-4835
Cocoa flavanol supplementation preserves early and late radial artery function after transradial catheterization†
Received
22nd March 2023
, Accepted 25th April 2023
First published on 25th April 2023
Abstract
Background: The transradial approach for coronary angiography is associated with fewer complications and preferred over the femoral approach. Injury to the radial artery (RA) endothelium elicits intimal hyperplasia, possibly resulting in total occlusion and limb functional decline. Flavanols are known to improve endothelial function. Effects on arterial remodeling after mechanical injury are unknown. Objective: To investigate the effects of cocoa flavanols on (a) intimal hyperplasia and (b) endothelial functional recovery after mechanical vascular wall injury through transradial coronary angiography (TCA). Methods: Primary endpoint in this double-blind, randomized, controlled trial was RA intima-media thickness (IMT) after 6 months follow-up (FU). Secondary endpoints were RA flow-mediated vasodilation (FMD) and fractional diameter change (Fdc). Further luminal diameter and circulating endothelial microparticles (EMP) were assessed. Thirty-six male patients undergoing elective TCA were included. Flavanol or matched placebo supplementation started 7 days prior TCA (cocoa flavanol 1000 mg day−1) for 14 days. Four measurements spanned three periods over 6-moths-FU. Results: TCA induced sustained intimal hyperplasia in the placebo-, but not in the flavanol-group (IMT 0.44 ± 0.01 vs. 0.37 ± 0.01 mm, p = 0.01). FMD decreased after TCA in both groups, but recovered to baseline after 6 months in the flavanol group only. Fdc acutely decreased, EMPs increased in the placebo-, not in the flavanol -group. Luminal diameter remained unchanged in both groups. Conclusion: Peri-interventional cocoa flavanol supplementation prevents long-term intima media thickening and endothelial dysfunction 6 months after TCA opening the perspective for dietary interventions to mitigate endothelial cell damage and intimal hyperplasia after mechanical injury.
Introduction
The radial artery (RA) is the widely preferred access site for coronary angiography and percutaneous coronary interventions (PCI) for both diagnostic and therapeutic management of coronary artery disease (CAD).1 Compared to the femoral access, the transradial access (TRA) is associated with decreased bleeding risk, vascular site complications, a significant reduction in peri-procedural mortality and major adverse cardiovascular events (MACE) in acute coronary syndrome (ACS) procedures.2,3 The American Heart Association (AHA) therefore recommend the TRA as first line approach for acute or stable ischemic heart disease.4 This can also provide reductions in economic burden and costs, patient's comfort and increases post-procedural safety.1 However, there are concerns that the TRA may damage the arterial wall and induce intimal hyperplasia following the late onset of complications like post-procedural radial artery occlusion (RAO).5 Damage of the vessel wall and chronic hyperplasia limit the usability of the RA as a potential bypass graft or dialysis shunt vessel in the future and may induce a reduction in endothelial cell regeneration and function, which can affect cardiovascular health.
In our previous work, we already demonstrated that radial access for TCA leads to an acute impairment in vascular and endothelial function as measured by a decrease in flow-mediated-vasodilation (FMD) of the radial and brachial artery.6 Furthermore, we demonstrated that the functional capacity of the endothelium is impaired in smokers,7 which is associated with reduced nitric oxide synthase (NOS)-activity and reduced vascular repair capabilities.8 Maintaining endothelial health is of crucial importance and several mechanisms to optimize endothelial function have been addressed,9 one of them being the dietary supplementation of flavanols, a subfamily of flavonoids that are found in various food groups like berries, chocolate, and wine, for example. Flavanols are thought to increase endothelial NO synthase (eNOS) activity and nitric oxide (NO) bioavailability.10,11 Our previous data suggest that (−)-epicatechin via it circulating structurally related metabolites may be the major bioactive component of the flavanol capsule in this study (see ESI Table 3†).12 (−)-Epicatechin is associated with a prolonged and augmented NO synthesis that may be mediated by the increase of L-arginine conversion to L-citrulline and NO.13,14 It was further shown to improve endothelial function, integrity,15,16 and to decrease blood pressure and arterial stiffness.17–19 The methylxanthines in cocoa may contribute to the biological activities of cocoa via enhancing the bioavailability.20 In addition, our research suggests that procyanidins may interact with cholesterol absorption leading to lowering of plasma cholesterol levels.12
Flavanols also modulate the adhesion and migration of monocytes to the vascular endothelium and increase the levels of circulating angiogenic cells (CAC).21
Therefore, we hypothesize that peri-interventional cocoa flavanol supplementation mitigates EC dysfunction associated with TCA, preserves EC integrity as assessed by microparticle release, and thus prevents long-term intima-media hyperplasia.
Materials and methods
Recruitment and ethics
We identified 60 consecutive male patients, who were scheduled for elective TCA in the cardiology department of the Heinrich Heine University Hospital Düsseldorf. After the screening process, 16 patients did not meet the inclusion criteria and 3 men refused to participate in the study (Fig. 2).
Inclusion criteria were the indication for an elective TCA by a cardiologist and a non-pathologic Allen's test, indicative of sufficient collateral blood supply to the hand and forearm on the intervention site and male gender to exclude sex-specific effects. For TCA interventions a 6F-sheath was used and patients were heparinized by 5000 I.E. of heparin. Exclusion criteria were prior TCA on the site of the currently planned approach, inflammation (C-reactive protein (CRP) >2.0 mg dl−1), known malignancies, heart rhythm other than sinus rhythm, symptomatic heart failure (New York Heart Association functional class III or IV), terminal renal failure (eGFR < 30 ml min−1), and female gender. No distinction was made concerning known CAD or prior coronary angiography. If CAD was already known, patients were on standard optimal medical therapy including a statin, beta-blocker, ACE-inhibitor/ARB, Aspirin, and/or P2Y12 inhibition. Ethical approval was obtained from the ethics committee of the Heinrich Heine University Düsseldorf (No. 3574) and registered at clinical trials (NCT03488823). All participants gave informed signed consent for the examination of medical records and use of the data. The data that support the findings of this study are available from the corresponding author, upon reasonable request.
Study protocol
Examinations took place at four-time points including 6 months of FU (Fig. 1). Baseline assessment was performed one week before TCA (−7 d) and included baseline characteristics, blood work, and medication history as well as ultrasound measures. Primary endpoint was defined as intima-media thickness (IMT) during FU. Secondary endpoints were radial artery endothelial function measured by flow-mediated vasodilation (FMD) and fractional diameter change (Fdc). Tertiary endpoint were luminal and external arterial diameter. Quaternary endpoint was circulating levels of endothelial microparticles (EMP), acquired at three visits. As an intra-individual control, we acquired FMD of the contralateral arm, where no local intervention or manipulation occurred. Participants were randomized after assessment to receive either 500 mg (2 capsules) of cocoa flavanols or 2 capsules of matched placebo control supplementation twice-daily (total of 4 capsules per day) for one week prior to elective TCA to achieve a stable increase in endothelial function even after excretion of structurally related cocoa flavanol metabolites as demonstrated in our previous work and using a similar dose.16,22 The composition of the flavanol capsule is listed in ESI Table 3.† Placebo capsules were filled with microcrystalline cellulose and matched for theobromine and caffeine content. Thus, flavanols and (−)-epicatechin were absent in placebo capsules. Overall appearance and weight of placebo capsule were not distinguishable from those of active capsules. Supplementation was continued for 14 days in total. Participants were instructed to continue intake for 7 days after TCA to ensure stable effects on endothelial function during the healing process. Participants were advised to consume study capsule with breakfast and with dinner. The morning before TCA subject's bilateral radial arteries were examined the second time (−2 h) to document the effect of the dietary intervention and directly after compression release on the radial artery after TCA (+2 h) for the third time. Blood was drawn at time points −7 d, −2 h before TCA, and +2 h after TCA to determine plasma levels of circulating endothelial microparticles (EMP). After 6 months, patients were called and examined again (+6 M) to assess the long-term arterial remodeling (primary and tertiary outcome) and late functional recovery. To evaluate the changes between the four time points, three periods were defined: period 1 = pretreatment prior to TCA, flavanol effect from −7 d to −2 h; period 2 = acute effects of TCA, flavanol effect from −7 d to +2 h, and period 3 = long-term effects of TCA, flavanol effect from 7 d to +6 M.
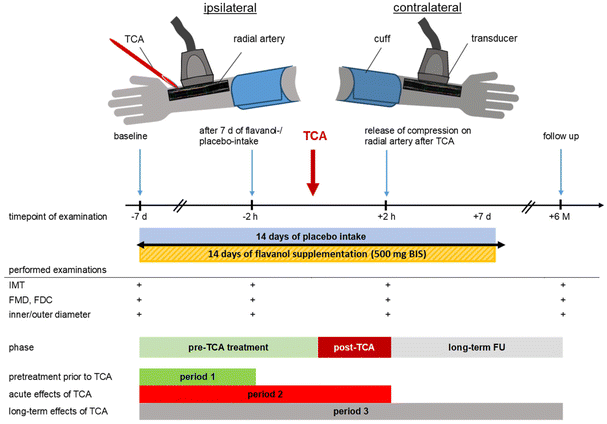 |
| Fig. 1 Study protocol. The vascular assessment was performed at baseline (−7 d), after one week of flavanol-/placebo-supplementation (−2 h), after complete release of the compression device after TCA (+2 h), and at follow-up (+6 M). Flavanols or placebo were administrated seven days prior and seven days after TCA, respectively. Primary outcome was intima-media thickness (IMT). Secondary outcomes were flow-mediated vasodilation (FMD), flow-mediated vasodilation on the contralateral arm of sheath induction (FMDcontra), and fractional diameter change (Fdc). Tertiary outcomes were the outer diameter (OD) and inner diameter (ID) of the investigated vessel (d = days, h = hours, M = months). Period 1 “green bars” = pretreatment prior to TCA = flavanol effect from −7 d to −2 h; period 2 “red bars” = acute effects of TCA = from −7 d to +2 h; period 3 “grey bars” = long-term effects of TCA = from −7 d to +6 M. | |
Ultrasound assessment of IMT, arterial diameters, FMD, and Fdc
FMD was measured as previously described23 at both, intervention arm and contralateral arm. Briefly, vessel diameter and flow velocity of the RA were measured using a 12 MHz transducer (Vivid I, GE) and automatic edge-detection software (Brachial Analyzer, Medical Imaging Applications, Iowa City, Iowa) yielding standard deviations of mean differences between repeated measurements of less than an absolute 1.0%. Reactive hyperemia was induced by 5 min of lower arm occlusion with a sphygmomanometer cuff inflated to 200 mmHg. Immediately after cuff deflation, and 20, 40, 60, and 80 s later, RA diameter was assessed, and FMD was calculated as maximal relative diameter gain relative to baseline. External diameters reflected the media adventitia interface, also called external elastic membrane to the opposite side (EEM diameter). Inner/luminal arterial diameters were calculated as diastolic external EEM diameter minus 2*IMT. IMT was measured with automatic contour detection software between the intimal and adventitial layers at identical sites used for the RA-FMD measurements. Local arterial stiffness was determined at the site of RA-FMD measurements as a fractional diameter change (Fdc) during one heart cycle and calculated as diametersystolic − diameterdiastolic/diameterdiastolic. Thus, an increased arterial stiffness is reflected by a decrease in Fdc.
Blood collection, preparation, and microparticle characterization by flow cytometry
The methods have been already described.24,25 Citrated blood (6 ml) was drawn from the cubital vein contralateral to intervention arm and processed within 2 h. Platelet-rich plasma was obtained by centrifugation of whole blood at 300g over 15 min at room temperature. Platelet-free plasma was obtained by 2 successive centrifugations of platelet-rich plasma at 10
000g for 5 min at room temperature. Briefly, samples were incubated for 30 min with fluorochrome-labeled antibodies or matching isotype controls and analyzed in a Canto II flow cytometer (Beckton Dickinson, Heidelberg, Germany). Microbead standards (1.0 μm) were used to define MPs as <1 μm in diameter. The EMP subpopulations were defined as CD31+/CD41−, CD62e+, or CD144+ events. The total number of EMP was quantified using flow-count calibrator beads (Beckman Coulter; 20 ml).
Statistical analysis
Data are presented as means ± standard error of the mean (SEM). Statistical analyses were performed by using Fisher's exact test (case controls) and unpaired t-tests for detecting differences between the groups. Repeated measurements were calculated as ANCOVA with baseline values as covariates with the Bonferroni post hoc test. Significance was assumed if p was <0.05. Analyses were performed by GraphPad Prism version 9 and SPSS statistics, version 24 (IBM, Armonk, USA).
Results
Baseline characteristics
Patient characteristics and procedural specifications are shown in Table 1. No significant differences in age, presence of known CAD, blood pressure, heart rate, smoking history or status, blood cell count, cholesterol, CRP, estimated glomerular filtration rate (eGFR), or medication history were detected between groups.
Table 1 Descriptive statistics of patient's characteristics. Mean, standard error of the mean (SEM), and statistical significance of differences (p) are reported for flavanol and placebo
|
Placebo (n = 18) |
Flavanol (n = 18) |
|
|
Mean |
SEM |
Mean |
SEM |
p
|
Age |
63.4 |
1.7 |
63.1 |
1.7 |
0.908 |
Weight (kg) |
90.9 |
3.8 |
81.8 |
2.4 |
0.053 |
Height (cm) |
178 |
2 |
176 |
2 |
0.487 |
BMI (kg m−2) |
28.6 |
1.0 |
26.3 |
0.7 |
0.083 |
Active smoker (%) |
11.1 |
|
11.1 |
|
1 |
Former smoker (%) |
77.8 |
|
83.3 |
|
0.99 |
|
Heart rate ( min−1) |
66 |
2 |
68 |
3 |
0.577 |
Blood pressure, systolic (mmHg) |
146 |
4 |
145 |
4 |
0.824 |
Blood pressure, diastolic (mmHg) |
82 |
2 |
85 |
2 |
0.369 |
Hypertension (%) |
89 |
|
100 |
|
0.49 |
Diabetes mellitus (%) |
39 |
|
11 |
|
0.402 |
CAD (%) |
44 |
|
50 |
|
0.999 |
|
eGFR (ml min−1) |
83 |
3 |
73 |
5 |
0.131 |
Urea (mg dl−1) |
31 |
2 |
32 |
2 |
0.695 |
Uric acid (mg dl−1) |
6.1 |
0.4 |
6.0 |
0.3 |
0.881 |
Total cholesterol (mg dl−1) |
176 |
9 |
202 |
10 |
0.073 |
Triglycerides (mg dl−1) |
179 |
38 |
212 |
32 |
0.527 |
HDL-C (mg dl−1) |
45 |
3 |
47 |
3 |
0.626 |
LDL-C (mg dl−1) |
118 |
10 |
148 |
11 |
0.053 |
C-reactive protein (mg dl−1) |
0.1 |
0.0 |
0.1 |
0.1 |
0.845 |
WBC (×1000) |
6.3 |
0.3 |
6.4 |
0.4 |
0.828 |
Hemoglobin(g dl−1) |
14.7 |
0.2 |
14.6 |
0.3 |
0.961 |
Flavanols prevent long-term media hyperplasia after TCA (period 3)
After randomization, no significant differences were detected between groups regarding IMT, inner or outer arterial diameter (see Table 1). In short-term follow-up (FU) + 2 h after TCA, no changes occurred in structural parameters IMT, inner diameter (ID), or outer diameter (OD). After 6 months of FU, in the placebo group RA-IMT increased significantly with a delta at +6 M of 0.074 mm [95% CI 0.061; 0.088] (ESI Table 1†). In the group taking flavanols no significant increase in IMT was detected with a delta to baseline at +6 M of 0.013 mm [95% CI −0.001; 0.026] (Fig. 3). Further, we did not find RA occlusion in any of our patients in the short- and long-term FU. In the contralateral arm, no changes in radial artery structure were observed in both groups. These data support the notion that dietary flavanol supplementation immediately before and after TCA prevents specifically at the site of injury structural remodeling of the injured radial artery.
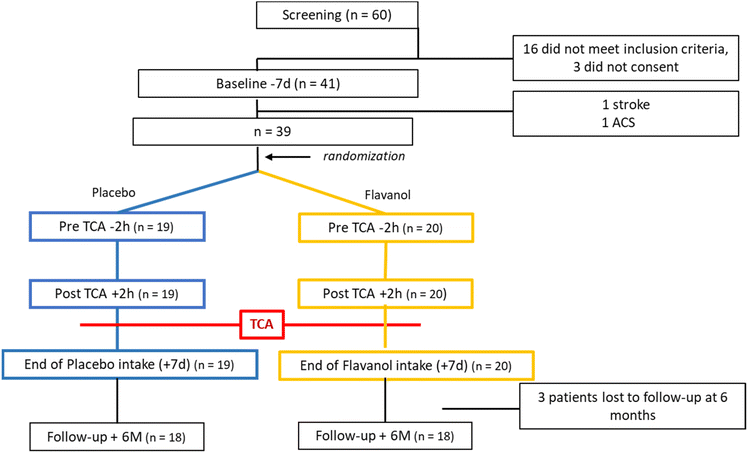 |
| Fig. 2 CONSORT diagram. After screening 60 potential participants, 36 complete datasets were included in the analysis (TCA = transradial coronary angiography, ACS = acute coronary syndrome). | |
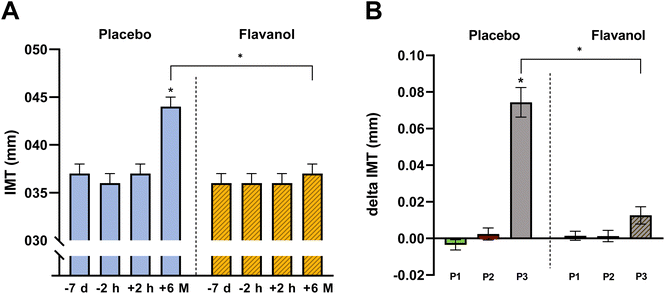 |
| Fig. 3 Flavanol supplementation reduces long-term IMT hyperplasia after TCA. (A) Absolute measures at different time points and (B) calculated deltas of absolute measures of IMT within three different periods relative to TCA. Supplementation of flavanols −7 d to +7 d. Long-term effect of the flavanol supplementation (−7 d to +6 M), p < 0.001; repeated measurements 2-way-(time × intervention) ANCOVA with baseline values as covariates with Bonferroni's post hoc test. Values are reported as mean ± SEM; (d = days; h = hours, m = months; P = period; period 1 “green bars” = pretreatment prior to TCA = flavanol effect from −7 d to −2 h; period 2 “red bars” = acute effects of TCA = flavanol effect from −7 d to +2 h; period 3 “grey bars” = long-term effects of TCA, flavanol effect from −7 d to +6 M). | |
Acute effects of cocoa flavanols on vascular function and compliance prior to TCA (period 1)
After randomization, no significant differences were detected between groups regarding FMD, contralateral FMD, Fdc (as a surrogate for arterial stiffness), or IMT (ESI Table 1†). One week of flavanol supplementation increased FMD on the site of later sheath insertion (9.2 ± 0.3% to 11.2 ± 0.5%) with a delta to baseline of 2.0% [95% CI 1.2; 2.7] and contralateral RA (8.7 ± 0.3% to 10.9 ± 0.4%) with a delta to baseline of 2.2% [95% CI 1.6; 2.9] (Fig. 4). Shortly after TCA FMD decreased back to baseline (+0.2 [95% CI −0.5; 0.9]). In the placebo group, it remained unchanged (see Table 2). Also, Fdc increased during the pre-TCA flavanol supplementation week (1.8 ± 0.3% to 3.2 ± 0.3%, delta to baseline 1.3% [95% CI 1.6; 2.9]), while it remained unchanged in placebo (2.2 ± 0.3% vs. 2.0 ± 0.3%) with a delta to baseline −0.1% [95% CI −0.9; 0.6]. The decrease in brachial artery blood pressure after one week of flavanol supplementation was not significant compared to placebo. Taken together these data corroborate previous findings from our and other groups that dietary flavanols improve endothelial function systemically and most important that the chosen dose of flavanols was bioactive.
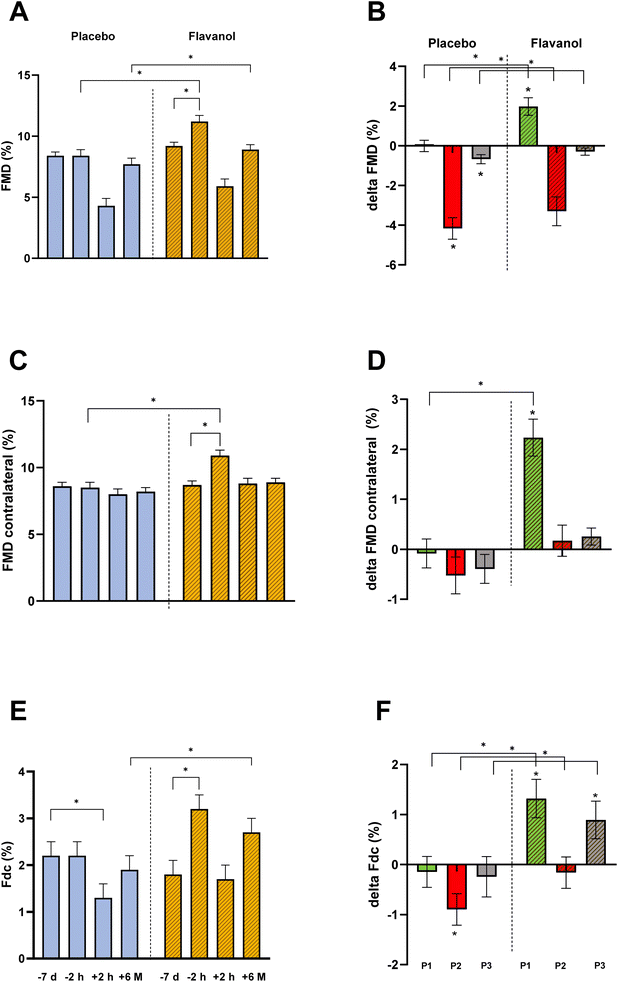 |
| Fig. 4 Flavanol supplementation improves endothelial function and facilitates functional recovery 6 months after TCA. Absolute measures and calculated deltas to baseline at −7 d for FMD on intervention arm (A and B), contralateral arm relative to TCA (C and D), and Fdc (E and F). Pretreatment effect (−7 d to −2 h), p < 0.05; acute effect (−7 d vs. +2 h), p < 0.001; repeated measurements 2-way- (time × intervention) ANCOVA with baseline values as covariates with Bonferroni's post hoc test. Values are reported as mean ± SEM. FMD, flow-mediated vasodilation, Fdc, fractional diameter change; (d = days; h = hours, m = months; P = period, period 1 “green bars” = pretreatment prior to TCA = flavanol effect from −7 d to −2 h; period 2 “red bars” = acute effects of TCA = flavanol effect from −7 d to +2 h; period 3 “grey bars” = long-term effects of TCA, flavanol effect from −7 d to +6 M). | |
Table 2 Differences in vascular structural and functional parameters calculated versus baseline. Values are reported as mean difference versus baseline with a 95% confidence interval. FMD, flow-mediated vasodilation; Fdc, fractional diameter change; FMD contralateral, flow-mediated vasodilation at the contralateral arm of sheath induction; inner diameter, intraluminal diameter; outer diameter, the diameter of the vessel including intima and media; repeated measurements 2-way-(time × intervention) ANCOVA with baseline values as covariates with Bonferroni's post hoc test, *p < 0.01; delta between periods (flavanol vs. placebo) are reported as absolute values; (period 1 “green bars” = pretreatment prior to TCA = flavanol effect from −7 d to −2 h; period 2 “red bars” = acute effects of TCA = flavanol effect from −7 d to +2 h; period 3 “grey bars” = long-term effects of TCA, flavanol effect from −7 d to +6 M)
TCA acutely decreases endothelial function, flavanols preserve long-term recovery
To test whether or not the beneficial long-term effects of flavanols on IMT 6 months after TCA are mediated through functional changes in the endothelium, we measured site specifically flow-mediated dilation and radial artery compliance immediately after TCA (period 2) and after long-term FU (period 3). TCA induced a rapid decrease of RA-FMD in cocoa flavanol (11.2 ± 0.5% vs. 5.9 ± 0.6%, p < 0.001) and placebo (8.4 ± 0.5% vs. 4.3 ± 0.6%, p < 0.001). FMD decreased with a delta of −3.3% compared to baseline [95% CI −4.6; −2.0] in flavanol and −4.2% [95% CI −5.5; −2.9] in placebo. Furthermore, Fdc decreased in both groups pre-TCA to post-TCA (3.2 ± 1.29% to 1.7 ± 1.38% and 2.0 ± 1.17% to 1.3 ± 0.88%). Post-TCA assessed Fdc was not different from baseline at −7 d when subjects ingested flavanol supplements (delta −0.2% [95% CI −0.8; 0.5]. In the placebo group, the decrease was significant with a delta of −0.9% compared to the initial measurement [95% CI −1.5; −0.3]. No significant changes were seen for IMT on the intervention arm or contralateral arm immediately post-TCA (Table 2).
After a 6-month FU (period 3), FMD had recovered to baseline (−7 d) in the cocoa flavanol group (delta +6 M −0.3 [−0.7; 0.1]). In the placebo group, FMD remained impaired with a delta to baseline of −0.7% (95% CI [−1.1; −0.3]). On the control arm, no significant long-term changes occur. These results unequivocally demonstrate that cocoa flavanols site-specifically limits endothelial dysfunction associated with TCA induced damage of radial artery endothelium.
Short-term effects of TCA on endothelial integrity
Cocoa flavanols improve not only vasodilatory function but also the integrity of vascular endothelium. Here we demonstrate that flavanols prevents an increase of levels in circulating EMP (see Table 3) as compared to the placebo group, which implies that cocoa flavanols limits site specific sequel of endothelial injury associated with the TCA procedure. Two hours after TCA endothelial microparticles increased in placebo group with a delta to baseline of 81 events per microliter (Ev per μl) [95% CI 48; 114] for CD31+/41−, 89 Ev per μl [95% CI 48; 130] for CD144+ and 69 Ev per μl [95% CI 14;125] for CD62e+ respectively. In the flavanol group, none of the EMP subgroups increased after TCA (ESI Table 2†).
Table 3 Differences in endothelial microparticle concentrations calculated versus baseline. Values are reported as mean difference versus baseline with a 95% confidence interval; repeated measurements 2-way-(time × intervention) ANCOVA with baseline values as covariates with Bonferroni's post hoc test, *p < 0.05; delta between periods (flavanol vs. placebo) are reported as absolute values; (period 1 “green bars” = pretreatment prior to TCA = flavanol effect from −7 d to −2 h; period 2 “red bars” = acute effects of TCA = flavanol effect from −7 d to +2 h)
Discussion
Our study provides evidence for the first time that cocoa flavanol supplementation
(i) mitigates EC dysfunction caused by TCA-induced injury directly and 6 months after transradial cardiac catheterization,
(ii) limits acute endothelial damage as evidenced by reduced microparticle release,
(iii) and thus, prevents intimal hyperplasia of the radial artery after 6 months of FU.
These findings clearly demonstrate, that cocoa flavanol supplementation preserves endothelial function after mechanical injury. Furthermore, our results encourage for testing the efficacy of dietary interventions during cardiac catheterization to preserve radial artery function.
Long-term structural remodeling following TCA
Radial artery injury through TCA induced significant vessel wall thickening in the placebo group after 6 months. These structural changes were prevented via flavanol supplementation. Though we did not detect significant luminal diameter changes in both groups, thickening of the intima-media complex is associated with both reduced elasticity and functional capacity.5 In a previous study, we showed a more pronounced IMT thickening in smokers, supposedly propagated through smooth muscle cell proliferation initiating atherosclerosis.7 Contrary, cocoa flavanol supplementation appears as a potential preventive strategy for injury-induced IMT thickening and reduced vascular remodeling, both of which represent risk factors for subsequent radial artery damage and occlusion.
Acute vascular complications occurring during and after TCA range from transient bleeding complications to vessel functional impairments to total RAO.26 Three risk factor categories are proposed for vascular complications: (1) pre-procedural, non-modifiable including age, female sex, diabetes, or previous RA-access; (2) peri-procedural, modifiable risk factors like insufficient anticoagulation, repeated punctures, sheath-to-artery ratio, or number of used catheters; and (3) post-procedural, modifiable parameters like occlusive or prolonged hemostasis.27 For subclinical, non-occlusive vascular remodeling following TCA, data is scarce. Prevention of vasospasms by intra-arterial or subcutaneous nitroglycerine28,29 has been proven effective in the mitigation of RAO. Short-term RAO is often induced through thrombosis following inadequate or prolonged compression duration (see ref. 27 for a review). Considering the 5.2% occlusion rate after transradial coronary angiography in the ARTEMIS trial,30 there is a need for improvement in peri-interventional management of vascular function and prevention of short- and long-term vascular complications.
Acute and long-term functional impairment after vascular injury
In line with previous observations,24,31 TCA induced significant damage to the vessel wall with an acute decrease in EC function as measured by FMD. The acute and chronic functional impairment is reversible after 6 months in the group supplementing cocoa flavanols for two weeks (1 before and 1 following TCA). In the placebo group, FMD remained impaired in long-term FU, indicative of persistently impaired EC function, which can be associated with reduced nitric oxide (NO) bioavailability.32 This observation is in line with previous studies31,33 describing a decrease in FMD following TCA in short- and mid-term FU. After TCA-induced vascular injury, CAC and nearby endothelial cells migrate to the point of injury where they propagate re-endothelialization processes and reduce the development of further damage, which is supported by NOS activity as it is induced by flavanol supplementation.34 Compared to baseline, Fdc significantly decreased after TCA in the control group, but not in the flavanol group and cocoa flavanols significantly improved Fdc after 7 days. The effect persisted after mechanical injury and in 6-month follow-up assessments. Fdc is a marker for arterial stiffness and local physicomechanical properties.35 Thereby, it serves as an adjunct to complement FMD and IMT as markers for endothelial function and vascular remodeling. Long-lasting improvements in Fdc arise from short-term improvements in endothelial function and vascular repair, which in turn curbs intima-media thickening.16
The improvements in FMD and Fdc are in line with previous publications suggesting a beneficial functional effect of flavanol intervention on short-term endothelial function and arterial stiffness15,36 and extends the findings to chronic effects on arterial structural adaptation to injury and maintenance. Dietary flavanols, especially (−)-epicatechin as the major monomeric flavan-3-ol contained in cocoa and the main ingredient in our study supplement, received a health claim by the European Food and Safety Authority (EFSA) in 2012. It is considered beneficial in maintaining FMD.37 The amount of (−)-epicatechin as a reliable marker of total flavanol content in commercially available chocolate products shows large variation. To achieve comparable amounts to the flavanols used in the present supplement, approximately 240–900 g of dark chocolate would be required.38–40 Cocoa flavanols have been shown to improve CVD risk biomarkers, including FMD and blood pressure, in individuals of different CVD risk categories ranging from healthy to established CVD.16 Potential mechanisms include modulation of the inflammatory response through NF-κB, TNFα, and IL-1β cascades.41 In the recent COSMOS trial, a 27% reduction in CV mortality was observed in a large cohort of older adults.42
Flavanols are potent inductors of NO bioavailability43 potentially through reduced NO inactivation by inhibition of NADPH oxidase, reducing oxidative damage and activating eNOS, thus increasing vasomotor function.44 Here we demonstrate that supplementation of flavan-3-ols before TCA, improves basal endothelial function and likely its regenerative capacity prior to mechanical damage. Circulating levels of EMP as markers of endothelial damage and injury were lower in the cocoa flavanol than in the control group (Fig. 5). The effect was significant for all three subpopulations (CD31+/41−, CD144+, CD62e+). CD144+ represents vascular endothelial cell cadherin (VE-cadherin), a marker for mechanical endothelial injury and endothelial cell damage.45 PROCAM+ (CD31+/41−) is a vascular cell adhesion and signaling molecule with increased vesicularization in response to endothelial damage. In patients with coronary artery disease, we had previously shown that flavan-3-ol-related improvements in FMD and circulating NO species were associated with marked decreases in circulating EMP, suggesting that flavan-3-ols improve endothelial integrity in patients with CVD45,46 without prior mechanical injury. In this trial, we confirm the previously observed decrease in EMP through one week of cocoa flavanol supplementation. Additionally, cocoa flavanols mitigates EMP release caused by mechanical injury measured two hours after TCA. Remote effects on contralateral FMD transmitted via circulating EMP could not be detected implying a site-specific beneficial effect of flavanols on mechanically injured endothelium.
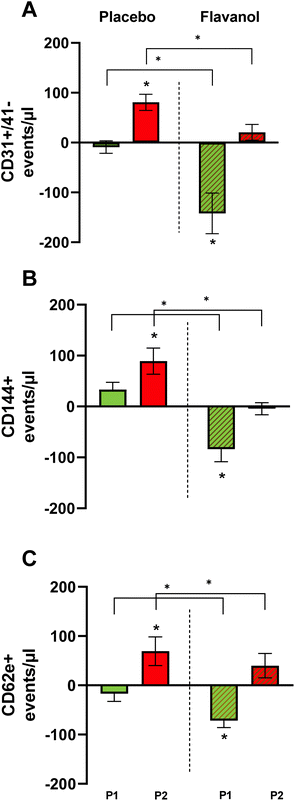 |
| Fig. 5 Flavanols preserve endothelial integrity and reduce structural damage after TCA. Circulating levels of endothelial microparticles CD31+/41− (A), CD144+ (B), and CD62e+ (C) as the difference to baseline measurement. *p < 0.001; repeated measurements 2-way-(time × intervention) ANOVA with baseline values as covariates with Bonferroni's post hoc test; (P = period; period 1 “green bars” = pretreatment prior to TCA = flavanol effect from −7 d to −2 h; period 2 “red bars” = acute effects of TCA = flavanol effect from −7 d to +2 h). | |
Preservation of RA integrity and function is critically important to prevent limb dysfunction and preserve access sites for future therapeutic interventions. In particular, high-risk patients are prone to severe aggravation of pre-existing endothelial dysfunction following TCA. Also, these subsets of patients with diabetes mellitus, hyperlipidemia, smoking history, and arterial hypertension exhibit a higher likelihood that functional radial arteries will be necessary for either coronary artery bypass grafting or dialysis shunts or as repetitive radial access sites for coronary angiography.
The flavanol amount of 1000 mg twice a day (containing around 160 mg (−)-epicatechin) represents a multiple of the average flavanol intake in the German general population.47 Our previous results have shown that this amount is approximately required to achieve maximal increase in FMD (ED50 approx. 226 mg cocoa flavanols with 31 mg (−)-epicatechin20) when given as a single dose and when given daily for at least one week leads to a ‘chronic’ increase in FMD that persists even after structurally related metabolites have been excreted.16,22 A Standard-European-Diet would not supply similar amounts of total flavanols, specifically (−)-epicatechin (daily average European (−)-epicatechin intake: 13 mg (ref. 48)), as supplemented in our study.49 If such flavanol-rich diet provides similar benefit on vascular function and repair remains unknown.
Limitations
A few limitations of our proof-of-concept study are worth mentioning. First, we did not measure total cholesterol levels at 6-months-FU, which may have an influence on the long-term effects.49 Second, we waived to measure neither plasma or urine levels of flavanols, i.e. (−)-epicatechin, (+)-catechin, or valerolactone nor did we assess the background diet with a food questionaries.10 Third, we did not measure the effect cocoa flavanols on microvascular endothelial function e.g. by laser Doppler imaging. However, the analysis of the contralateral radial artery served as control for interindividual variability of the biological activity18 of the flavanols as every individual in the flavanol group revealed an increment in FMD in the expected range. Imbalances in baseline characteristics between groups, especially for LDL-cholesterol, weight and diabetes mellitus (Table 1), while close to statistical significance, and due to chance in our randomized trial and likely attributable to the relatively small sample size of our study cohort.
Conclusion
Peri-interventional cocoa flavan-3-ol supplementation prevents arterial wall intima-media hyperplasia, protects the endothelium from mechanical damage and preserves endothelial function long-term, suggesting an improved healing process after mechanical vascular injury. Larger randomized controlled trials including other patient populations, geographic and cultural regions are necessary to assess the generalizability of our data, and to illustrate the huge potential of dietary interventions prior to TCA on vascular function. This may help to transfer the approach of flavanol supplementation before elective TCA into daily clinical practice.
Clinical perspectives
What is known?
Vascular injury induces a sustained endothelial dysfunction and long-term negative vascular remodeling. Several cardiovascular risk factors like smoking aggravate the response to damage. Coca flavanol supplementation improves short-term vascular function in healthy, non-injured arteries.
What is new?
Supplementation of cocoa flavanols facilitates regain of arterial function, and protects the endothelium after mechanical injury. One week prior to and after transradial coronary angiography prevents endothelial dysfunction, negative vascular remodeling, and intima-media thickening at 6 months after transradial catheterization.
What is next?
Future studies are necessary to test the preventive approach of peri-interventional cocoa flavanol supplementation at a larger scale and evaluate the beneficial effect of dietary interventions on short- and long-term radial artery function.
Abbreviations
CAC | Circulating angiogenic cells |
CAD | Coronary artery disease |
EC | Endothelial cell |
EMP | Endothelial microparticles |
eNOS | Endothelial nitric oxide synthase |
eGFR | Estimated glomerular filtration rate |
Ev/μl | Events per microliter |
Fdc | Fractional diameter change |
FMD | Flow-mediated vasodilation |
FU | Follow-up |
IMT | Intima-media thickness |
NO | Nitric-oxide |
NOS | Nitric oxide synthase |
OD | Outer diameter |
ID | Inner diameter |
PCI | Percutaneous coronary interventions |
RA | Radial artery |
RAO | Radial artery occlusion |
TCA | Transradial coronary angiography |
TRA | Transradial access |
Author contributions
Conceptualization: Christian Heiss, Malte Kelm and Ralf Erkens; data curation: Michael Gröne, Miriam Schillings and Dragos Duse; investigation: Michael Gröne, Miriam Schillings, Nicolas Kramser and Roberto Sansone; validation: Christian Heiss, Christian Jung, Malte Kelm and Ralf Erkens; writing – original draft: Michael Gröne, Dragos Duse and Ralf Erkens; writing – review & editing: Christine Quast, Christian Heiss, Roberto Sansone, Christian Jung, Malte Kelm and Ralf Erkens; supervision: Christian Heiss, Christian Jung and Malte Kelm.
Disclosures
Cocoa flavanol and placebo capsules were donated by Mars, Inc., USA.
Conflicts of interest
There are no conflicts to declare.
References
- A. A. Kolkailah, R. S. Alreshq, A. M. Muhammed, M. E. Zahran, M. Anas El-Wegoud and A. F. Nabhan, Transradial versus transfemoral approach for diagnostic coronary angiography and percutaneous coronary intervention in people with coronary artery disease, Cochrane Database Syst. Rev., 2018, 4, Cd012318 Search PubMed.
- A. J. Chase, E. B. Fretz, W. P. Warburton, W. P. Klinke, R. G. Carere, D. Pi, B. Berry and J. D. Hilton, Association of the arterial access site at angioplasty with transfusion and mortality: the M.O.R.T.A.L study (Mortality benefit Of Reduced Transfusion after percutaneous coronary intervention via the Arm or Leg), Heart, 2008, 94, 1019–1025 CrossRef CAS PubMed.
- P. J. Mason, B. Shah, J. E. Tamis-Holland, J. A. Bittl, M. G. Cohen, J. Safirstein, D. E. Drachman, J. A. Valle, D. Rhodes and I. C. Gilchrist, An Update on Radial Artery Access and Best Practices for Transradial Coronary Angiography and Intervention in Acute Coronary Syndrome: A Scientific Statement From the American Heart Association, Circ.: Cardiovasc. Interventions, 2018, 11, e000035 Search PubMed.
- J. S. Lawton, J. E. Tamis-Holland, S. Bangalore, E. R. Bates, T. M. Beckie, J. M. Bischoff, J. A. Bittl, M. G. Cohen, J. M. DiMaio, C. W. Don, S. E. Fremes, M. F. Gaudino, Z. D. Goldberger, M. C. Grant, J. B. Jaswal, P. A. Kurlansky, R. Mehran, T. S. Metkus Jr., L. C. Nnacheta, S. V. Rao, F. W. Sellke, G. Sharma, C. M. Yong and B. A. Zwischenberger, 2021 ACC/AHA/SCAI Guideline for Coronary Artery Revascularization: Executive Summary: A Report of the American College of Cardiology/American Heart Association Joint Committee on Clinical Practice Guidelines, Circulation, 2022, 145, e4–e17 CrossRef PubMed.
- M. Rashid, C. S. Kwok, S. Pancholy, S. Chugh, S. A. Kedev, I. Bernat, K. Ratib, A. Large, D. Fraser, J. Nolan and M. A. Mamas, Radial Artery Occlusion After Transradial Interventions: A Systematic Review and Meta-Analysis, J. Am. Heart Assoc., 2016, 5(1), e002686 CrossRef PubMed.
- C. Heiss, J. Balzer, T. Hauffe, S. Hamada, E. Stegemann, T. Koeppel, M. W. Merx, T. Rassaf, M. Kelm and T. Lauer, Vascular dysfunction of brachial artery after transradial access for coronary catheterization: impact of smoking and catheter changes, JACC Cardiovasc. Interventions, 2009, 2, 1067–1073 CrossRef PubMed.
- R. Sansone, E. Stegemann, G. Ozaslan, D. Schuler, M. Lukosz, A. Rodriguez-Mateos, T. Lauer, R. Westenfeld, M. Kelm and C. Heiss, Early and late response-to-injury in patients undergoing transradial coronary angiography: arterial remodeling in smokers, Am. J. Cardiovasc. Dis., 2014, 4, 47–57 Search PubMed.
- C. Heiss, A. Rodriguez-Mateos and M. Kelm, Central role of eNOS in the maintenance of endothelial homeostasis, Antioxid. Redox Signal., 2015, 22, 1230–1242 CrossRef CAS PubMed.
- R. Erkens, M. Totzeck, A. Brum, D. Duse, H. E. Bøtker, T. Rassaf and M. Kelm, Endothelium-dependent remote signaling in ischemia and reperfusion: alterations in the cardiometabolic continuum, Free Radical Biol. Med., 2021, 165, 265–281, DOI:10.1016/j.freeradbiomed.2021.01.040.
- A. Rodriguez-Mateos, N. Toro-Funes, T. Cifuentes-Gomez, M. Cortese-Krott, C. Heiss and J. P. Spencer, Uptake and metabolism of (−)-epicatechin in endothelial cells, Arch. Biochem. Biophys., 2014, 559, 17–23 CrossRef CAS PubMed.
- H. Schroeter, C. Heiss, J. Balzer, P. Kleinbongard, C. L. Keen, N. K. Hollenberg, H. Sies, C. Kwik-Uribe, H. H. Schmitz and M. Kelm, (−)-Epicatechin mediates beneficial effects of flavanol-rich cocoa on vascular function in humans, Proc. Natl. Acad. Sci. U. S. A., 2006, 103, 1024–1029 CrossRef CAS PubMed.
- A. Rodriguez-Mateos, T. Weber, S. S. Skene, J. I. Ottaviani, A. Crozier, M. Kelm, H. Schroeter and C. Heiss, Assessing the respective contributions of dietary flavanol monomers and procyanidins in mediating cardiovascular effects in humans: randomized, controlled, double-masked intervention trial, Am. J. Clin. Nutr., 2018, 108(6), 1229–1237, DOI:10.1093/ajcn/nqy229.
- L. Decroix, C. Tonoli, D. D. Soares, A. Descat, M. J. Drittij-Reijnders, A. R. Weseler, A. Bast, W. Stahl, E. Heyman and R. Meeusen, Acute cocoa Flavanols intake has minimal effects on exercise-induced oxidative stress and nitric oxide production in healthy cyclists: a randomized controlled trial, J. Int. Soc. Sports Nutr., 2017, 14, 28 CrossRef PubMed.
- J. A. Lovegrove, A. Stainer and D. A. Hobbs, Role of flavonoids and nitrates in cardiovascular health, Proc. Nutr. Soc., 2017, 1–13, DOI:10.1017/s0029665116002871.
- M. Gröne, R. Sansone, P. Höffken, P. Horn, A. Rodriguez-Mateos, H. Schroeter, M. Kelm and C. Heiss, Cocoa Flavanols Improve Endothelial Functional Integrity in Healthy Young and Elderly Subjects, J. Agric. Food Chem., 2020, 68, 1871–1876 CrossRef PubMed.
- R. Sansone, A. Rodriguez-Mateos, J. Heuel, D. Falk, D. Schuler, R. Wagstaff, G. G. Kuhnle, J. P. Spencer, H. Schroeter, M. W. Merx, M. Kelm and C. Heiss, Cocoa flavanol intake improves endothelial function and Framingham Risk Score in healthy men and women: a randomised, controlled, double-masked trial: the Flaviola Health Study, Br. J. Nutr., 2015, 114, 1246–1255 CrossRef CAS PubMed.
- C. Heiss, R. Sansone, H. Karimi, M. Krabbe, D. Schuler, A. Rodriguez-Mateos, T. Kraemer, M. M. Cortese-Krott, G. G. Kuhnle, J. P. Spencer, H. Schroeter, M. W. Merx and M. Kelm, Impact of cocoa flavanol intake on age-dependent vascular stiffness in healthy men: a randomized, controlled, double-masked trial, Age, 2015, 37, 9794 CrossRef PubMed.
- M. Bapir, P. Campagnolo, A. Rodriguez-Mateos, S. S. Skene and C. Heiss, Assessing Variability in Vascular Response to Cocoa With Personal Devices: A Series of Double-Blind Randomized Crossover n-of-1 Trials, Front. Nutr., 2022, 9, 886597 CrossRef PubMed.
- M. Bapir, G. R. Untracht, D. Cooke, J. H. McVey, S. S. Skene, P. Campagnolo, M. B. Whyte, N. Dikaios, A. Rodriguez-Mateos, D. D. Sampson, D. M. Sampson and C. Heiss, Cocoa flavanol consumption improves lower extremity endothelial function in healthy individuals and people with type 2 diabetes, Food Funct., 2022, 13, 10439–10448 RSC.
- R. Sansone, J. I. Ottaviani, A. Rodriguez-Mateos, Y. Heinen, D. Noske, J. P. Spencer, A. Crozier, M. W. Merx, M. Kelm, H. Schroeter and C. Heiss, Methylxanthines enhance the effects of cocoa flavanols on cardiovascular function: randomized, double-masked controlled studies, Am. J. Clin. Nutr., 2017, 105, 352–360 CrossRef CAS PubMed.
- D. Milenkovic, A. Rodriguez-Mateos, M. Lucosz, G. Istas, K. Declerck, R. Sansone, R. Deenen, K. Köhrer, K. F. Corral-Jara, J. Altschmied, J. Haendeler, M. Kelm, W. V. Berghe and C. Heiss, Flavanol Consumption in Healthy Men Preserves Integrity of Immunological-Endothelial Barrier Cell Functions: Nutri(epi)genomic Analysis, Mol. Nutr. Food Res., 2022, e2100991, DOI:10.1002/mnfr.202100991.
- C. Heiss, D. Finis, P. Kleinbongard, A. Hoffmann, T. Rassaf, M. Kelm and H. Sies, Sustained increase in flow-mediated dilation after daily intake of high-flavanol cocoa drink over 1 week, J. Cardiovasc. Pharmacol., 2007, 49, 74–80 CrossRef CAS PubMed.
- C. Heiss, A. Rodriguez-Mateos, M. Bapir, S. S. Skene, H. Sies and M. Kelm, Flow-mediated dilation reference values for evaluation of endothelial function and cardiovascular health, Cardiovasc. Res., 2023, 119(1), 283–293, DOI:10.1093/cvr/cvac095.
- P. Horn, N. Amabile, F. S. Angeli, R. Sansone, B. Stegemann, M. Kelm, M. L. Springer, Y. Yeghiazarians, H. Schroeter and C. Heiss, Dietary flavanol intervention lowers the levels of endothelial microparticles in coronary artery disease patients, Br. J. Nutr., 2014, 111, 1245–1252 CrossRef CAS PubMed.
- P. Horn, M. M. Cortese-Krott, N. Amabile, C. Hundsdorfer, K. D. Kroncke, M. Kelm and C. Heiss, Circulating microparticles carry a functional endothelial nitric oxide synthase that is decreased in patients with endothelial dysfunction, J. Am. Heart Assoc., 2012, 2, e003764 CrossRef PubMed.
- E. M. Coghill, T. Johnson, R. E. Morris, I. L. Megson and S. J. Leslie, Radial artery access site complications during cardiac procedures, clinical implications and potential solutions: The role of nitric oxide, World J. Cardiol., 2020, 12, 26–34 CrossRef PubMed.
- I. Bernat, A. Aminian, S. Pancholy, M. Mamas, M. Gaudino, J. Nolan, I. C. Gilchrist, S. Saito, G. N. Hahalis, A. Ziakas, Y. Louvard, G. Montalescot, G. A. Sgueglia, M. A. H. van Leeuwen, A. M. Babunashvili, M. Valgimigli, S. V. Rao and O. F. Bertrand, Best Practices for the Prevention of Radial Artery Occlusion After Transradial Diagnostic Angiography and Intervention: An International Consensus Paper, JACC Cardiovasc. Interventions, 2019, 12, 2235–2246 CrossRef PubMed.
- Y. Chen, Z. Ke, J. Xiao, M. Lin, X. Huang, C. Yan, S. Ye and X. Tan, Subcutaneous Injection of Nitroglycerin at the Radial Artery Puncture Site Reduces the Risk of Early Radial Artery Occlusion After Transradial Coronary Catheterization: A Randomized, Placebo-Controlled Clinical Trial, Circ.: Cardiovasc. Interventions, 2018, 11, e006571 CAS.
- S. Dharma, S. Kedev, T. Patel, F. Kiemeneij and I. C. Gilchrist, A novel approach to reduce radial artery occlusion after transradial catheterization: postprocedural/prehemostasis intra-arterial nitroglycerin, Catheter. Cardiovasc. Interventions, 2015, 85, 818–825 CrossRef PubMed.
- G. Hahalis, K. Aznaouridis, G. Tsigkas, P. Davlouros, I. Xanthopoulou, N. Koutsogiannis, I. Koniari, M. Leopoulou, O. Costerousse, D. Tousoulis and O. F. Bertrand, Radial Artery and Ulnar Artery Occlusions Following Coronary Procedures and the Impact of Anticoagulation: ARTEMIS (Radial and Ulnar ARTEry Occlusion Meta-AnalysIS) Systematic Review and Meta-Analysis, J. Am. Heart Assoc., 2017, 6(8), e005430 CrossRef PubMed.
- A. Buturak, B. M. Tekturk, A. Degirmencioglu, S. Ulus, O. Surgit, C. Ariturk, E. Zencirci and S. Gorgulu, Transradial catheterization may decrease the radial artery luminal diameter and impair the vasodilatation response in the access site at late term: an observational study, Heart Vessels, 2016, 31, 482–489 CrossRef PubMed.
- R. Erkens, M. Totzeck, A. Brum, D. Duse, H. E. Bøtker, T. Rassaf and M. Kelm, Endothelium-dependent remote signaling in ischemia and reperfusion: Alterations in the cardiometabolic continuum, Free Radicals Biol. Med., 2021, 165, 265–281 CrossRef CAS PubMed.
- Z. Yan, Y. Zhou, Y. Zhao, Z. Zhou, S. Yang and Z. Wang, Impact of transradial coronary procedures on radial artery
function, Angiology, 2014, 65, 104–107 CrossRef PubMed.
- C. Heiss, A. Schanz, N. Amabile, S. Jahn, Q. Chen, M. L. Wong, T. Rassaf, Y. Heinen, M. Cortese-Krott, W. Grossman, Y. Yeghiazarians and M. L. Springer, Nitric oxide synthase expression and functional response to nitric oxide are both important modulators of circulating angiogenic cell response to angiogenic stimuli, Arterioscler., Thromb., Vasc. Biol., 2010, 30, 2212–2218 CrossRef CAS PubMed.
- J. Balzer, M. Boos, T. Rassaf, C. Heiss, M. Preik, S. Matern, F.-C. Schoebel, M. Kelm and T. Lauer, “One-Stop-Shop” ultrasound diagnosis of functional, structural and physicomechanical properties of the brachial artery, VASA. Zeitschrift für Gefässkrankheiten – J. Vasc. Dis., 2007, 36, 100–106 CrossRef CAS PubMed.
- J. P. Garcia, A. Santana, D. L. Baruqui and N. Suraci, The Cardiovascular effects of chocolate, Rev. Cardiovasc. Med., 2018, 19, 123–127 CrossRef PubMed.
- N. Efsa, Panel on Dietetic Products and Allergies, Scientific Opinion on the modification of the authorisation of a health claim related to cocoa flavanols and maintenance of normal endothelium-dependent vasodilation pursuant to Article 13(5) of Regulation (EC) No 1924/2006 following a request in accordance with Article 19 of Regulation (EC) No 1924/2006, EFSA J., 2014, 12, 3654 Search PubMed.
- S. Langer, L. J. Marshall, A. J. Day and M. R. Morgan, Flavanols and methylxanthines in commercially available dark chocolate: a study of the correlation with nonfat cocoa solids, J. Agric. Food Chem., 2011, 59, 8435–8441 CrossRef CAS PubMed.
- J. Vlachojannis, P. Erne, B. Zimmermann and S. Chrubasik-Hausmann, The Impact of Cocoa Flavanols on Cardiovascular Health, Phytother. Res., 2016, 30, 1641–1657 CrossRef CAS PubMed.
- I. Amoah, J. J. Lim, E. O. Osei, M. Arthur, P. Tawiah, I. N. Oduro, M. S. Aduama-Larbi, S. T. Lowor and E. Rush, Effect of Cocoa Beverage and Dark Chocolate Consumption on Blood Pressure in Those with Normal and Elevated Blood Pressure: A Systematic Review and Meta-Analysis, Foods, 2022, 11(13), 1962 CrossRef CAS PubMed.
- D. Milenkovic, A. Rodriguez-Mateos, M. Lucosz, G. Istas, K. Declerck, R. Sansone, R. Deenen, K. Köhrer, K. F. Corral-Jara, J. Altschmied, J. Haendeler, M. Kelm, W. V. Berghe and C. Heiss, Flavanol Consumption in Healthy Men Preserves Integrity of Immunological-Endothelial Barrier Cell Functions: Nutri(epi)genomic Analysis, Mol. Nutr. Food Res., 2022, 66, e2100991 CrossRef PubMed.
- H. D. Sesso, J. E. Manson, A. K. Aragaki, P. M. Rist, L. G. Johnson, G. Friedenberg, T. Copeland, A. Clar, S. Mora, M. V. Moorthy, A. Sarkissian, W. R. Carrick and G. L. Anderson, Effect of cocoa flavanol supplementation for the prevention of cardiovascular disease events: the COcoa Supplement and Multivitamin Outcomes Study (COSMOS) randomized clinical trial, Am. J. Clin. Nutr., 2022, 115, 1490–1500 CrossRef PubMed.
- C. Heiss, C. L. Keen and M. Kelm, Flavanols and cardiovascular disease prevention, Eur. Heart J., 2010, 31, 2583–2592 CrossRef CAS PubMed.
- J. Davignon and P. Ganz, Role of endothelial dysfunction in atherosclerosis, Circulation, 2004, 109, III27–32 Search PubMed.
- R. Sansone, M. Baaken, P. Horn, D. Schuler, R. Westenfeld, N. Amabile, M. Kelm and C. Heiss, Release of endothelial microparticles in patients with arterial hypertension, hypertensive emergencies and catheter-related injury, Atherosclerosis, 2018, 273, 67–74 CrossRef CAS PubMed.
- C. Heiss, S. Jahn, M. Taylor, W. M. Real, F. S. Angeli, M. L. Wong, N. Amabile, M. Prasad, T. Rassaf, J. I. Ottaviani, S. Mihardja, C. L. Keen, M. L. Springer, A. Boyle, W. Grossman, S. A. Glantz, H. Schroeter and Y. Yeghiazarians, Improvement of endothelial function with dietary flavanols is associated with mobilization of circulating angiogenic cells in patients with coronary artery disease, J. Am. Coll. Cardiol., 2010, 56, 218–224 CrossRef CAS PubMed.
- A. Vogiatzoglou, T. Heuer, A. A. Mulligan, M. A. Lentjes, R. N. Luben and G. G. Kuhnle, Estimated dietary intakes and sources of flavanols in the German population (German National Nutrition Survey II), Eur. J. Nutr., 2014, 53, 635–643 CrossRef CAS PubMed.
- A. Vogiatzoglou, A. A. Mulligan, R. N. Luben, M. A. Lentjes, C. Heiss, M. Kelm, M. W. Merx, J. P. Spencer, H. Schroeter and G. G. Kuhnle, Assessment of the dietary intake of total flavan-3-ols, monomeric flavan-3-ols, proanthocyanidins and theaflavins in the European Union, Br. J. Nutr., 2013, 1–11, DOI:10.1017/S0007114513003930.
- A. Rodriguez-Mateos, T. Weber, S. S. Skene, J. I. Ottaviani, A. Crozier, M. Kelm, H. Schroeter and C. Heiss, Assessing the respective contributions of dietary flavanol monomers and procyanidins in mediating cardiovascular effects in humans: randomized, controlled, double-masked intervention trial, Am. J. Clin. Nutr., 2018, 108, 1229–1237 CrossRef PubMed.
|
This journal is © The Royal Society of Chemistry 2023 |
Click here to see how this site uses Cookies. View our privacy policy here.