DOI:
10.1039/D3FO00496A
(Paper)
Food Funct., 2023,
14, 7222-7239
Ginsenoside-Rg1 synergized with voluntary running exercise protects against glial activation and dysregulation of neuronal plasticity in depression†
Received
6th February 2023
, Accepted 28th June 2023
First published on 29th June 2023
Abstract
Depression is a common psychological disease accompanied by mental disorders and somatic symptoms. However, the underlying mechanisms regarding the pathogenesis of depression are still not clear. Neuronal damage resulting from inflammation is considered to be one of the important risk factors for depression. Ginsenoside-Rg1, a sterol extract extracted from ginseng herbs, has been shown to have neuroprotective effects against neurodegenerative diseases. Moreover, running exercise, a simple behavioral therapy, has been recently shown to have antidepressant effects. However, whether these two synergized strategies are more efficient in depression treatment, especially the neural mechanisms underlying this practical and interesting treatment is unknown. In this study, we have shown that ginsenoside-Rg1 synergized with voluntary running exercise exerts more efficiency on suppressing neuroinflammation, up-regulating expression of neurotrophic factors, and synaptic-related proteins, ameliorating neuronal structural damages than that of ginsenoside-Rg1 or voluntary exercise alone, suggesting its better neuroprotective effects. More importantly, the antidepressant-like effect of this synergistic treatment was also significantly better than either of these two treatments. These results suggest that ginsenoside-Rg1, synergized with voluntary running, may have higher efficacy in the treatment of depression through anti-inflammation and the improvement of neuroplasticity. These findings may provide a new perspective for the development of a therapeutic strategy for depression.
1. Introduction
Major depressive disorder (MDD) is a very prevalent mental illness that severely impairs patients’ psychosocial performance and lowers the overall quality of their lives.1 However, the fundamental mechanisms that are involved in the pathophysiology of depression are still unknown. Depression is associated with structural and functional abnormalities in certain brain regions, such as the amygdala, hippocampus, and prefrontal cortex.2–4 Recent evidence has suggested that depression is accompanied by volume reduction and dysfunction of the frontal cortex and hippocampal area.5 Neuroinflammation is considered to contribute to a range of neurological disorders as the major risk factor for neuronal damage.6,7 Impairments in neuronal structure and function can be caused by enhanced neuroinflammatory response, which can then lead to neuroplasticity deficiencies.8 In the brain, microglial dysregulation or glia activation plays key roles in neuroprotection,9,10 neuroinflammation, and induction of depression-like behavior.11,12 According to the findings of earlier studies, patients who suffer from major depressive disorder have been shown to have elevated levels of certain pro-inflammatory factors, including interleukin-1 (IL-1), tumor necrosis factor-a (TNF-a), interleukin-6 (IL-6), and reactive oxygen species (ROS).13 These findings suggested the possibility of inflammatory response in the development of depressive disorders.14 Therefore, investigating the molecular mechanisms driving these inflammatory processes in the depressed phenotype is required for the development of targeted treatment in MDD.
Ginsenoside-Rg1 is a natural steroidal saponin extracted from the herb Panax ginseng, which is shown to have a wide range of neurotrophic and neuroprotective effects with little toxicity.15,16 We previously reported that ginsenoside-Rg1 could prevent depression-like behavior in rats by suppressing oxidative stress, neuroinflammation, synaptic deficits, and neuronal apoptosis.17–20 In addition, ginsenoside-Rg1 was found to prevent depression-like behavior in rats by modulating neuronal structural plasticity.21,22 Physical activity is currently reported to exert antidepressant effects, which suggests its potential to be a useful addition to pharmacotherapy and psychotherapy in depression.23–25 Running exercise, as a simple behavioral therapy, has been widely demonstrated to have antidepressant effects.24 It has been reported that running exercise may increase the level of brain-derived neurotrophic factor (BDNF),26 enhance hippocampal neurogenesis,27 and reduce inflammatory damage.28 However, whether ginsenoside-Rg1 synergized with exercise could exhibit more efficiency in depression treatment is mainly unknown.
Therefore, in the present study, we investigated whether this synergized strategy, which is practically carried out in life, is more efficient in depression treatment. Based on this, we utilized an LPS-induced depression animal model to explore whether a combination of drug treatment and exercise could better ameliorate depression-like behavior in rats and further elucidate the underlying mechanism of its antidepressant effects.
2. Materials and methods
2.1. Animals
The Experimental Animal Centre at Shandong University supplied male Wistar rats weighing 160–180 g (about six weeks). Only male rats were utilized in this study to rule out the influence of sex differences on depression. All experimental procedures were approved by the Ethics Committee of Shandong University (ECSBMSSDU2020-2-017) and strictly adhered to the International Guidelines for Animal Research established by the Council of International Medical Organizations. Rats lived in each cage setting with controlled temperature (22–24 °C) and light (12 h light/dark cycle) for one week before the experiment began. During the investigation, every effort was made to minimize animal distress and the number of animals used.
2.2. Depression animal model
As previously described, LPS (0.5 mg kg−1, Sigma, L-2880) was dissolved in sterile 0.9% saline (NaCl, 0.9%). Wistar rats were intraperitoneally injected daily with LPS for two weeks to induce depressive-like behavior.29 This dose was used to stimulate subclinical infection without inducing overt inflammation and other overt damage in the animals. Ginsenoside-Rg1 (National Institute for Drug and Biological Control (Beijing, China)) with >99% purity was dissolved in normal saline at a concentration of 10 mg mL−1 for experiments.18,19 In all experiments, saline, LPS, or ginsenoside Rg1 was injected intraperitoneally at a constant volume. Normal saline or ginsenosides were injected 30 min before LPS injection. All drugs were administered between 09:00 and 12:00 hours daily for two weeks. The control group was injected with an equal volume of saline. The running wheel was used for the voluntary running exercise in the experimental group for 1 h per day.
2.3. Experimental design
Rats were randomly divided into five groups (N = 20 per group): (1) non-LPS injection control group, (2) intraperitoneal LPS injection group, (3) running treatment group, followed by LPS injection, (4) ginsenoside Rg1 treatment group (40 mg kg−1), followed by LPS injection, (5) running exercise and ginsenoside co-treatment group, followed by LPS injection. After the last LPS injection, behavioral tests were performed. Twenty-four hours following behavioral testing, hippocampal and ventromedial prefrontal cortex tissues were obtained for further investigations.
2.4 Behavioral tests
On the second day of the last LPS injection, the following behavioral tests were performed by an observer who was blind to the treatment protocol. All behavioral tests were performed during the dark circadian period (19:00–24:00 for rats).
2.4.1 Sucrose preference test.
As reported previously, the sucrose preference test (SPT) was used to examine the anhedonia symptoms.21,30 Rats were caged individually during the adaption phase. They had access to 2 bottles of 1% sucrose solution for 24 h; then, one was replaced with tap water. In the test phase, rats were given free access to 100 mL of sucrose solution (1%, w/v) and 100 mL of tap water for 3 h following 24 h of food and water deprivation. Sucrose preference was defined as sucrose consumption/[water consumption + sucrose consumption] 100%.
2.4.2 Forced swimming test.
The forced swim test (FST) was used to measure behavioral despair in rats.31,32 On the first day, the rat was put in an 80 cm × 30 cm glass cylinder filled with 40 cm of 25 °C water for 15 min. After 24 h, each rat was placed in the cylinder for 5 min to record immobility and swimming time. Floating with only the head exposed was considered immobility time.
2.4.3 Open field test.
The open field test (OFT) was performed to evaluate the exploratory activity and anxiety-like behavior in rats as previously described.33,34 The open-field test consists of a wooden box (95 × 95 × 95 cm) with a black bottom and walls, and the bottom is divided equally into 25 blocks. Rats were placed individually in the center of the arena and allowed to explore for 5 min freely. A camera was on the apparatus to record the activity of the rats. Time spent in the central area and the total movement distance were analyzed using a video tracking system (SMARTv2.5, Spain). After each rat was tested, the arena surface was cleaned with 75% ethanol.
2.4.4 Elevated plus maze test.
The elevated plus maze test (EPT) test was performed as previously described to assess exploration of the novel environment and fear of cantilever opening, which represent anxiety-like behavior.35,36 The device is formed by two opposing open arms (30 cm length × 15 cm height) and two closed arms surrounding a central platform (5 cm × 5 cm) with four arms horizontally 50 cm above the ground. Rats were placed individually on a robust platform with their head facing open arms and free to explore the maze for 5 min. A camera was on the apparatus to record the activity of the rats. The time and number of entries into the open arms for each rat were conveyed by a video tracking system (SMARTv2.5, Spain) analysis.
2.4.5 Morris water maze.
The Morris water maze (MWM) test was performed as previously described to evaluate spatial memory and learning ability in an aqueous environment.33,37 The procedure of this test consisted of placing the rats individually in a glass cylinder (height: 80 cm, diameter: 30 cm) filled with 40 cm of water (25 °C) for 15 min on day 1. After 24 h, the rats were again individually placed in the cylinder for 5 min, and the immobility and swimming times of each rat were recorded. Immobility time was defined as only exposing the head above the water surface while floating.
2.5 Transmission electron microscopy (TEM)
Twenty-four hours after behavioral tests, six rats were anesthetized with phenobarbital (30 mg kg−1), and the brains were removed by decapitation. Then, the DG tissues (1 × 1 × 1 mm) were isolated from the brain on an ice plate. To minimize the number of rats in this study, the rest of the brain tissue was collected for western blot and PCR analysis. The samples were soaked in the 2.5% glutaraldehyde at 4 °C for 12 h.Tissue was fixed with 1% OsO4 in 0.1 M PBS (pH 7.4) for 1 h, then dehydrated with ethanol and infiltrated with acetone overnight. Tissues were implanted in resin, sliced into ultrathin slices (70 nm), and stained with 4% uranyl acetate and 0.5% lead citrate. Sections were TEM-analyzed (Philips Tecnai 20 U-Twin). At least 20 micrographs from each rat were evaluated using Image J. (NIH, Scion Corporation, Frederick, MD). Synapses per unit volume and presynaptic vesicles were matched as specified.38,39
2.6 Western blot
Twenty-four hours after behavioral tests, four rats were decapitated after anesthetized with phenobarbital (30 mg kg−1), and the brain was collected on an ice plate to prepare tissue homogenate. The hippocampus and ventromedial prefrontal cortex tissue were homogenized with protease and phosphatase inhibitors extracted protein. After centrifugation (20 min, 12
000 rpm, 4 °C), protein-rich lysates were gathered. The BCA assay kit measured protein concentrations (Beyotime). Electrophoretic ally resolved proteins (30 g) from each sample were repositioned to PVDF membranes. Membranes were treated with 5% non-fat milk at room temperature for 1 h and primary antibodies overnight at 4 °C. Primary antibodies were anti-IL-1β (catalog: ab254360; Abcam); anti-BDN (catalog: ab6201, Abcam); anti-CD45 (catalog: ab10558; Abcam); anti-CD11b (catalog: ab133357;Abcam); anti–PSD-95 (catalog: 3450; Cell Signaling Technologies); anti-SYT1 (catalog: 4558; Cell Signaling Technologies); anti-p-CREB (catalog: 9198; Cell Signaling Technologies); anti–β-actin (catalog: 4970; Cell Signaling Technologies); anti-GAPDH (catalog: 10494-1-AP; both Pro-teintech Group); The secondary antibodies were Peroxidase-conjugated goat anti-rabbit IgG (catalog: ZB-2301, Zhongshan Golden Bridge Biotechnology) and Peroxidase-conjugated goat anti-mouse (catalog: ZB-2305, Zhongshan Golden Bridge Biotechnology). Bands on the membranes were detected using the Enhanced Chemiluminescence kit (ECL, Thermo Fisher) and quantified using Image-J software. Intra-run normalization against the internal β-actin or antibody GAPDH control was performed for each sample.
2.7 Quantitative real-time PCR
Twenty-four hours after behavioral tests, six rats were decapitated after being anesthetized with phenobarbital (30 mg kg−1). The hippocampus and ventromedial prefrontal cortex tissue were isolated on ice, and total RNA was extracted using a kit (Aidlab Biotechnologies, Beijing, China). Nano Drop ND-1000 spectrophotometer was utilized to measure RNA purity and concentration (Nano Drop Thermo, Wilmington, DE). The All-in-OneTM miRNA First-Strand cDNA Synthesis Kit was used to reverse-transcribe RNA into cDNA (GeneCopoeia, Guangzhou, China). After reverse-transcribing into cDNA, PCR amplified total RNA using specified primers (ESI Table 1†). Bio-Rad IQ5 Real-Time PCR was utilized for quantitative PCR. 2Ct was used to define miRNA fold change. Each group was loaded with GAPDH.
2.8 Immunofluorescent staining and confocal microscopy
Twenty-four hours after behavioral tests, eight rats were anesthetized with phenobarbital (30 mg kg−1) and perfused with 4% paraformaldehyde (PFA). Brains were fixed in 4% PFA overnight and then in 30% sucrose for two days. 30 μm frozen coronal hippocampus and ventromedial prefrontal cortex slices were preserved at 20 °C. Primary antibodies were incubated overnight at 4 °C. Primary antibodies used were anti-BDNF (catalog: sc-546, Santa Cruz Biotechnology Inc.); anti-PSD-95 (catalog 3450; Cell Signaling Technologies); anti-SYT1 (catalog: 14558; Cell Signaling Technologies); anti-p-CREB (catalog: 9198; Cell Signaling Technologies); anti-ion-Iba-1 (catalog: 019-19741, Wako Pure Chemical Inc), anti-GFAP (catalog: 16825-1-AP, Proteintech) Secondary antibodies were alexa-405 (Abcam) and alexa-568 (Invitrogen). The slices were washed in PBS and counterstained with DAPI (C0060, Solarbio) for 5 min. Images were captured by laser scanning confocal microscope (LSM780, Carl Zeiss). Image-Pro Plus 6.0 program analyzed 4 to 6 photos per rat (Media Cybernetics). ImageJ was used to analyze fluorescence intensity. Using Image J, researchers counted Iba1 and GFAP-positive cells per 1 mm2. All experiments were blinded and stereological.
2.9 Golgi staining
After behavioral testing, six rats were anesthetized with sodium phenobarbital (30 mg kg−1) and then decapitated to collect the brains. Golgi staining was applied to examine alterations in neuronal dendritic spines. The brains were immersed in an impregnation solution (A/B = 1
:
1, 15 mL per rat) for two weeks. The coronal slides (100 μm) were transferred to gelatin-coated microscope slides and air-dried at room temperature. The slides were stained as directed and covered with Rahman gum for light microscopy. Neuronal apical dendrites were analyzed morphologically. From each group, 4 to 6 dendritic segments per neuron and five pyramidal neurons per rat were randomly picked and then counted the spines with Image-Pro Plus 6.0.
2.10 Statistics
GraphPad Prism version 8.0.1. (GraphPad Software, Inc., San Diego, CA) was used for statistical analyses. All data were expressed as the Means ± SEMs. To determine whether or not there was a statistically significant difference between the groups, a Student's t-test and one-way or two-way analysis of variance (ANOVA) followed by Tukey's multiple-comparison test was applied. We have chosen a value of P < 0.05 to denote statistical significance.
2.11 Study approval
All experimental procedures were approved by the Shandong University Animal Care and Use Committee (ECSBMSSDU-2018-2-056) and conform to the NIH Guide for the Care and Use of Laboratory Animals (National Academies Press, 2011).
3. Result
3.1 Ginsenoside-Rg1 synergistic voluntary wheel running treatment alleviated LPS-induced depressive-like behavior
The sucrose preference test showed that the percentage of sucrose consumption by each of the five groups was significantly different [F(4, 55) = 290.1, P < 0.0001] (Fig. 1B). Post hoc analysis showed that a two-week treatment with LPS resulted in a significant reduction in the percent of sucrose consumption in rats when compared to that of the control group (P < 0.0001). These kinds of reactions suggested that the rats, who had been induced by LPS, were suffering from anhedonia, a basic sign of depression. However, chronic pretreatment of voluntary exercise or ginsenoside-Rg1 for rats significantly increased the percentage of sucrose consumption in LPS-induced rats (P < 0.0001). More importantly, ginsenoside-Rg1 synergized by voluntary exercise induced higher sucrose consumption compared to that of voluntary exercise or ginsenoside-Rg1 treatment solely.
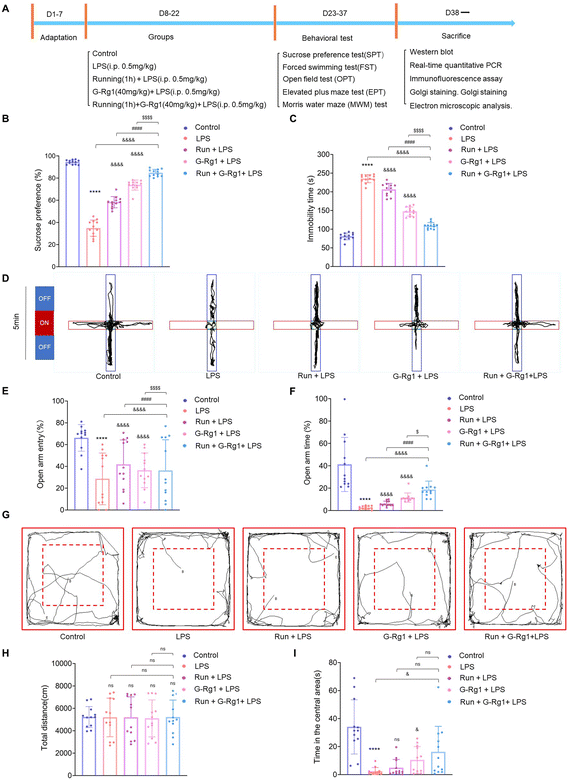 |
| Fig. 1 Ginsenoside-Rg1 synergized with voluntary running treatment ameliorated LPS-induced depressive-like behavior. (A) Experimental design: schematic figure of the treatment protocol. (B) The synergistic treatment prevented the decrease in sucrose consumption in LPS-rats in the SPT. (C) The synergistic treatment reversed the increase in immobility times in LPS-rats in the FST. (D–F) The synergistic treatment ameliorated the reduced open arm entry (OE) times and the reduced entrance number in open arm time (OT) in LPS-rats. (G–I) The synergistic treatment increased the central zone residence time and the total distance in the open field in LPS-rats. All values are presented as means ± SEM. N = 12 per group. A one-way analysis of variance (ANOVA) with the Tukey post-hoc correction is used for analysis. | |
Results of the forced swim test showed that there were significant differences among the five groups with regard to both immobility [F(4, 55) = 362.1, P < 0.0001] (Fig. 1C). Post hoc analysis showed that LPS-rats have considerably longer immobility periods than the normal control rats (P < 0.0001). Similarly, ginsenoside-Rg1 synergized with voluntary exercise significantly decreased the immobility times in rats than that of voluntary exercise or ginsenoside-Rg1-treated rats (P < 0.001).
The elevated plus maze (EPM) test was used to investigate the anxiety behavior of rats. The results revealed a statistically significant difference between open arm entry (OE) number [F(4, 55) = 5.484, P < 0.001] and open arm times (OT) [F(4, 55) = 21.00, P < 0.0001] (Fig. 1D–F). Post hoc analysis indicated that LPS treatment for 2 weeks significantly reduced the number of open arm entries (OE) and open arm times (OT) compared to non-LPS controls (P < 0.0001). In contrast, rats pretreated with ginsenoside-Rg1 synergized by voluntary exercise had a statistical increase in open arm entry (OE) (P < 0.0001) and open arm times (OT) compared with rats pretreated with voluntary exercise (P < 0.0001) or ginsenoside-Rg1 (P < 0.05) solely.
The open field test (OFT) is used to detect loco-motor activity and exploratory behavior of rats. The results showed that there was not a statistically significant difference in the total distance moved in the open field [F(4, 55) = 0.01041, P > 0.1], but there was a statistically significant difference in the residence time in the central region of the open field [F(4, 55) = 11.35, P < 0.0001] (Fig. 1G–I). LPS treatment for 2 weeks significantly reduced the central zone residence time compared to non-LPS controls (P < 0.0001). In contrast, rats that received pretreatment with ginsenoside-Rg1 synergized with exercise (P < 0.05) had a statistically significant increase in the central region residence time in the open field.
The Morris water maze (MWM) test were conducted to evaluate cognitive function, learning, and memory abilities (ESI Fig. 1A†). In the MWM test, there was no difference in the average swimming speed in the place navigation test from days 1 to 5 among each group [F(16, 275) = 1.612, P > 0.05, ESI Fig. 1B†], but the escape latency was significantly changed among the five groups [F(16, 275) = 2.390, P < 0.01, ESI Fig. 1C†]. Results showed that LPS rats significantly increased the escape latency as compared with that of the control group (P < 0.0001). However, the escape latency of rats that received synergistic treatment was significantly shortened (P < 0.0001). In the spatial probe test on day 6, the times and the number of crossings in the platform also changed significantly among the five groups [F(4, 44) = 98.56, P < 0.0001 and F(4, 44) = 17.15, P < 0.0001, ESI Fig. 1D and E†]. The number of times the LPS-induced rats crossed the platform was less than that of the control group (P < 0.0001), while rats receiving synergistic treatment significantly increased the number of times than that of rats subjected to running (P < 0.0001) or ginsenoside-Rg1 (P < 0.0001) solely. These findings demonstrated that the synergistic treatment markedly protected learning and memory loss in the LPS-induced rats.
3.2 Ginsenoside-Rg1 synergistic voluntary wheel running treatment reduced LPS-induced glial activation within the hippocampus and ventromedial prefrontal cortex (vmPFC)
The activation of glial cells was employed to evaluate the potential involvement of neuroinflammatory responses in animal depression models. The number of GFAP-positive astroglia in the hippocampus (Fig. 2A) and vmPFC area varied significantly between the five groups (Fig. 3A). Results showed that the LPS induced an extraordinary activation of astroglia cell responses within the hippocampus (Fig. 2C and D) (P < 0.0001 for both) and vmPFC (Fig. 3C and D) (P < 0.0001 for both) of rats; moreover, cellular protuberance extension and hypertrophy were also observed in the hippocampus (ESI Fig. 2A†) and vmPFC regions (ESI Fig. 3A†). Howerever, these changes were significantly attenuated by ginsenoside-Rg1 synergized with running treatment. These results indicate that the synergistic treatment can more significantly inhibit astroglia activation.
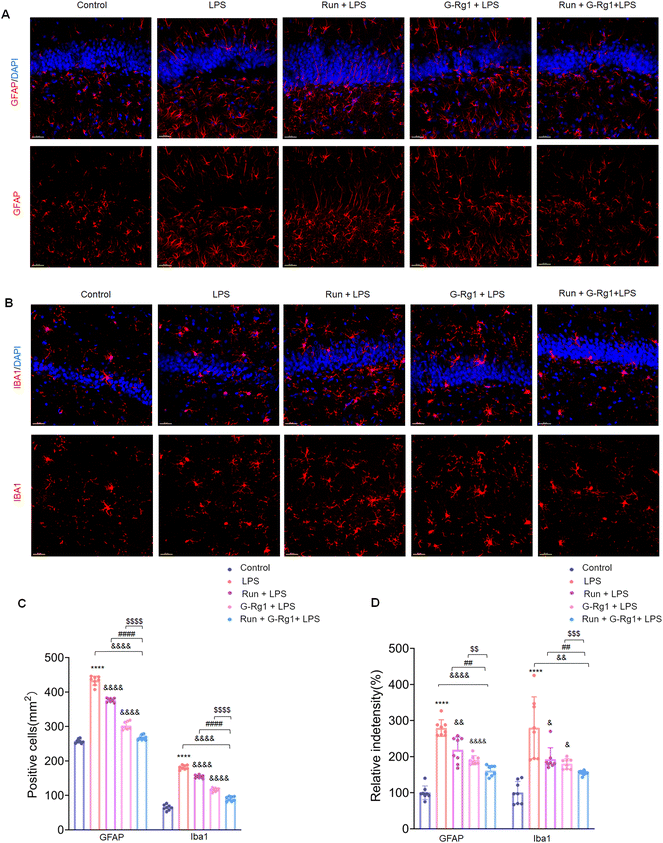 |
| Fig. 2 Ginsenoside-Rg1 synergistic voluntary wheel running treatment reduced LPS-induced glial activation. (A) Immunofluorescence shows GFAP-positive astrocytes within the hippocampus. Nuclei (blue) are stained with DAPI. Scale bar is 50 μm. (B) Immunofluorescence shows Iba-1-positive microglial cells within the hippocampus. Nuclei (blue) are stained with DAPI. Scale bar is 50 μm. (C) Bar graph illustrating the mean number of glial cells within the hippocampus of rats. (D) Bar graph illustrating the mean fluorescence intensity of glial cells within the hippocampus. All values are presented as means ± SEM. N = 8 per group. A one-way analysis of variance (ANOVA) with the Tukey post-hoc correction used for analysis. | |
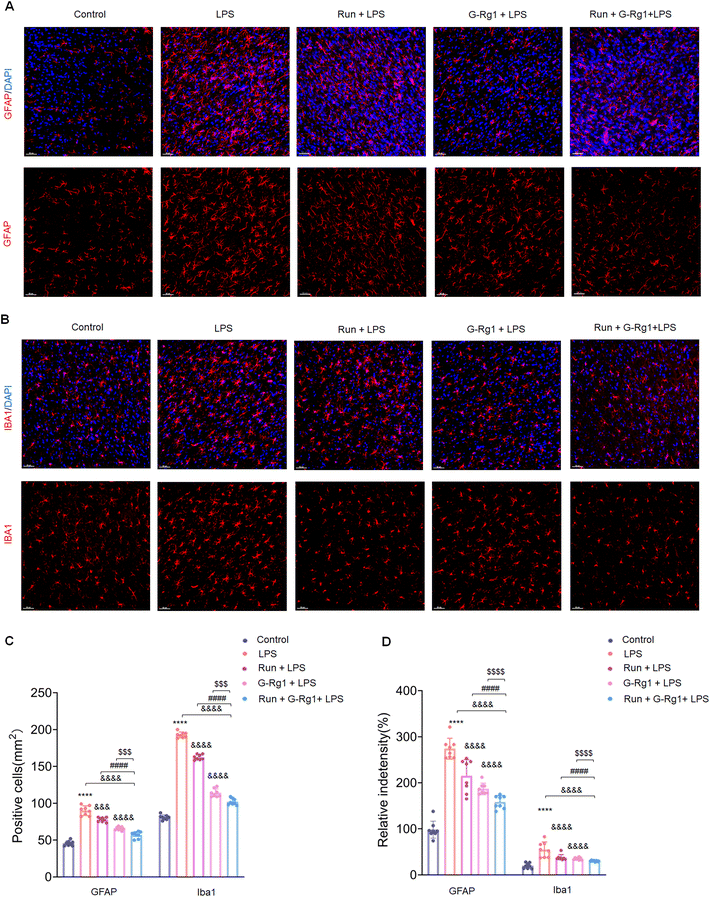 |
| Fig. 3 Ginsenoside-Rg1 synergistic voluntary wheel running treatment reduced LPS-induced glial activation. (A) Immunofluorescence shows GFAP-positive astrocytes within the vmPFC region of rats. Nuclei (blue) are stained with DAPI. Scale bar is 50 μm. (B) Immunofluorescence shows Iba-1-positive microglial cells within the vmPFC region of rats. Nuclei (blue) are stained with DAPI. Scale bar is 50 μm. (C) Bar graph illustrating the mean number of glial cells within the vmPFC region of rats. (D) Bar graph illustrating the fluorescence intensity of glial cells within the vmPFC region of rats. All values are presented as means ± SEM. N = 8 per group. A one-way analysis of variance (ANOVA) with the Tukey post-hoc correction was used for analysis. | |
Microglial activation is vital for neuroinflammatory responses. Here, ANOVA exhibited that the numeral of Iba-1positive microglia within the hippocampus (Fig. 2B) and vmPFC (Fig. 3B) significantly increased among the five groups. Results showed that LPS significantly raised the number of activated microglia within the hippocampus (Fig. 2C and D) (P < 0.0001 for both) and vmPFC (Fig. 3C and D) (P < 0.0001 for both). Moreover, 2-week treatment with LPS caused ramified process retraction and soma augmentation of microglia in the hippocampus (ESI Fig. 2B†) and vmPFC (ESI Fig. 3B†). These alterations in microglia cell number and morphology were reduced by treatment with ginsenoside-Rg1 or voluntary wheel running. However, the synergistic treatment reversed these changes considerably (P < 0.01).
3.3 Ginsenoside-Rg1 synergistic voluntary wheel running treatment reduced LPS-induced inflammatory cytokine expression within the hippocampus and vmPFC
Neuroinflammation is regarded as a crucial risk factor in the etiology of depression. Two weeks of treatment with LPS caused overexpression of CD11b (P < 0.05), CD45(P < 0.05), and IL-1β (P < 0.001) proteins within the hippocampus (Fig. 4A) and vmPFC (Fig. 4B) as compared with the control group, effects which were reduced by treatment with ginsenoside-Rg1 synergized by voluntary running more significantly. In addition, mRNA expressions of several essential pro-inflammatory cytokines, including IL-1β, IL-6, and IFN-γ were all increased within the hippocampus (Fig. 4C) and ventromedial prefrontal cortex (Fig. 4D). Consistently, the synergistic treatment also reduced the overexpression of these cytokines more obviously than pretreatment with ginsenoside-Rg1or running solely.
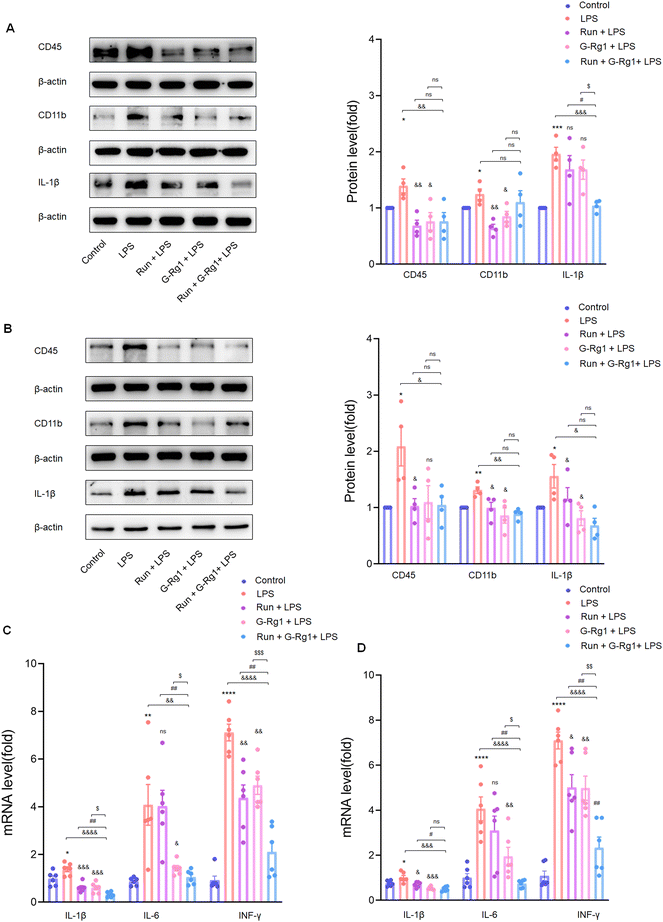 |
| Fig. 4 Ginsenoside-Rg1 synergistic voluntary wheel running treatment reduced LPS-induced inflammatory cytokine expression. (A and B) Western blot analysis shows protein levels of CD45, CD11b, and IL-1β within the hippocampus and vmPFC regions of rats. Normalized intensity bands are presented as the means ± SEM. N = 4 per group. (C and D) PCR assays of mRNA expression levels of IL-1β, IL-6, and IFN-γ within each group. N = 6 per group. A one-way analysis of variance (ANOVA) with the Tukey post-hoc correction was used for analysis. | |
3.4 Ginsenoside-Rg1 synergistic voluntary wheel running treatment up-regulated the production of BDNF protein in the hippocampus and vmPFC
To determine the potential role of neurotrophic factors in the antidepressant effects of ginsenoside-Rg1 or voluntary wheel running, the phosphorylation levels of CREB were first measured. Immunofluorescence analysis revealed that the LPS-induced reduction of CREB phosphorylation levels in the hippocampus (Fig. 5A and C) (P < 0.0001) and vmPFC regions (Fig. 6A and C) (P < 0.0001). The western blot study provided additional support for these findings within the hippocampus (Fig. 5D) and vmPFC (Fig. 6D). However, the synergized treatment significantly improved the CREB signaling pathway than pretreatment with ginsenoside-Rg1 or running solely (P < 0.0001).
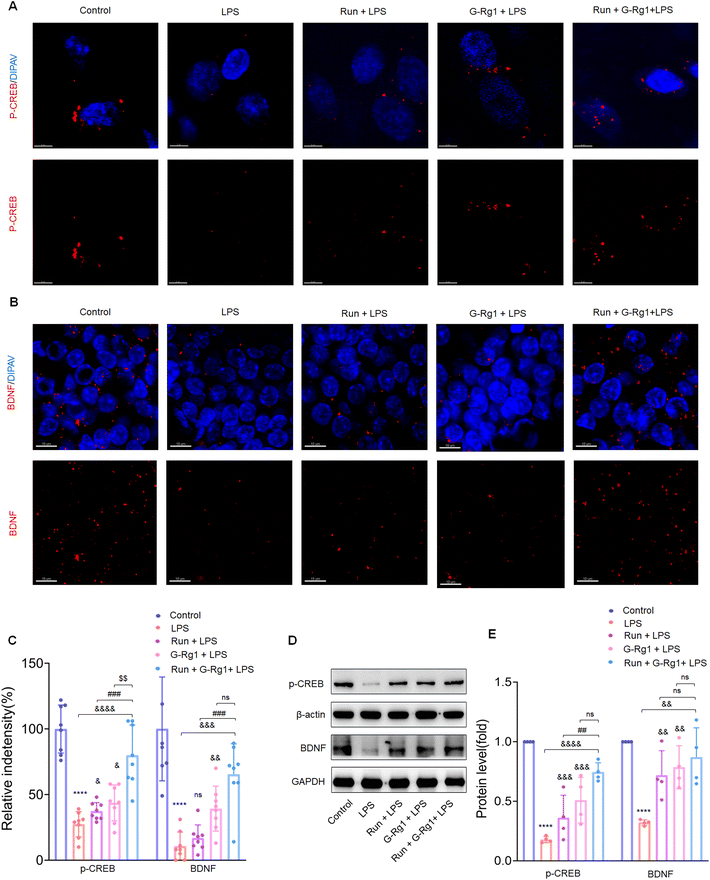 |
| Fig. 5 Ginsenoside-Rg1 synergistic voluntary wheel running treatment up-regulated the expression of brain-derived neurotrophic factor. (A and B) Immunofluorescence shows phosphorylated-CREB and BDNF within the hippocampus. (C) Histogram shows the fluorescence intensities in the hippocampus neurons of each group. Nuclei (blue) are stained with DAPI. Scale bars are 5 μm and 10 μm. N = 8 per group. (D) Western blot analysis of phosphorylation levels of p-CREB and protein expression levels of BDNF within the hippocampus. (E) Normalized intensity bands are presented as means ± SEM. N = 4 per group. A one-way analysis of variance (ANOVA) with the Tukey post-hoc correction was used for analysis. | |
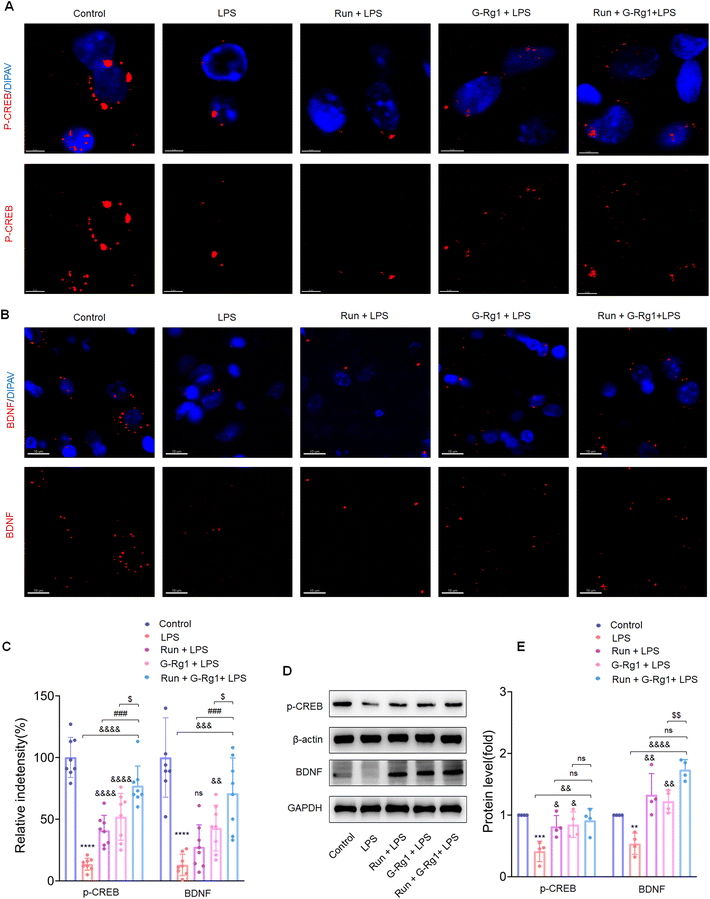 |
| Fig. 6 Ginsenoside-Rg1 synergistic voluntary wheel running treatment up-regulated the expression of brain-derived neurotrophic factor. (A and B) Immunofluorescence shows phosphorylated-CREB and BDNF within the vmPFC region of rats. (C) Histogram shows the fluorescence intensities in the vmPFC neurons of each group. Nuclei (blue) are stained with DAPI. Scale bars are 5 μm and 10 μm. N = 8 per group. (D) Western blot analysis of phosphorylation levels of p-CREB and protein expression levels of BDNF within the vmPFC region of rats. (E) Normalized intensity bands are presented as means ± SEM. N = 4 per group. A one-way analysis of variance (ANOVA) with the Tukey post-hoc correction was used for analysis. | |
In addition, LPS also decreased the BDNF expression within the hippocampus (Fig. 5B and C) (P < 0.0001) and vmPFC (Fig. 6B and C) (P < 0.0001). Moreover, western blot assays of BDNF protein levels also supported the above findings (Fig. 5D and 6D) (P < 0.01), while the synergized treatment recreates more significant effects on the BDNF expression than either ginsenoside Rg1 or voluntary running treatment.
3.5 Ginsenoside-Rg1 synergized voluntary wheel running treatment up-regulates synaptic-related proteins in the hippocampus and vmPFC
Synaptic-related proteins were considered involved in neuronal synaptogenesis. The immunofluorescence assays indicated that synaptophysin and PSD-95 levels were all significantly reduced in the hippocampus (Fig. 7A and B) (P < 0.0001) and vmPFC (Fig. 8A and B) (P < 0.0001) after 2-week treatment with LPS. In contrast, the synergized treatment up-regulated synapse-related proteins significantly compared to the ginsenoside-Rg1 or voluntary running treatment solely (Fig. 7C and Fig. 8C). Western blot assays showed a similar tendency of PSD-95 and synaptophysin expression (Fig. 7D and Fig. 8D) (p < 0.05). These findings further confirm the synergistic treatment could improve the LPS-induced reduction in synapse-related protein expression more significantly.
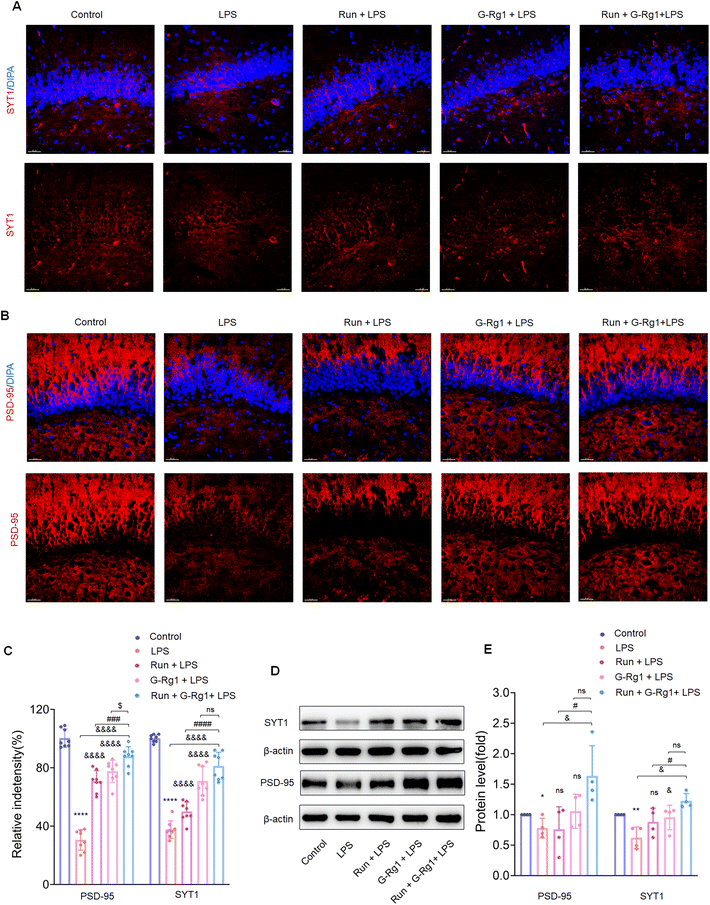 |
| Fig. 7 Ginsenoside-Rg1 synergized voluntary wheel running treatment up-regulates synaptic-related proteins expression. (A and B) Immunofluorescence shows SYT1 and PSD-95 within the hippocampus. (C) Histogram shows the fluorescence intensities in the hippocampus neurons of each group. Nuclei (blue) are stained with DAPI. Scale bar is 30 μm. N = 8 per group. (D) Western blot analysis of protein levels of SYT1 and PSD-95 within the hippocampus. (E) Normalized intensity bands are presented as means ± SEM. N = 4 per group. A one-way analysis of variance (ANOVA) with the Tukey post-hoc correction was used for analysis. | |
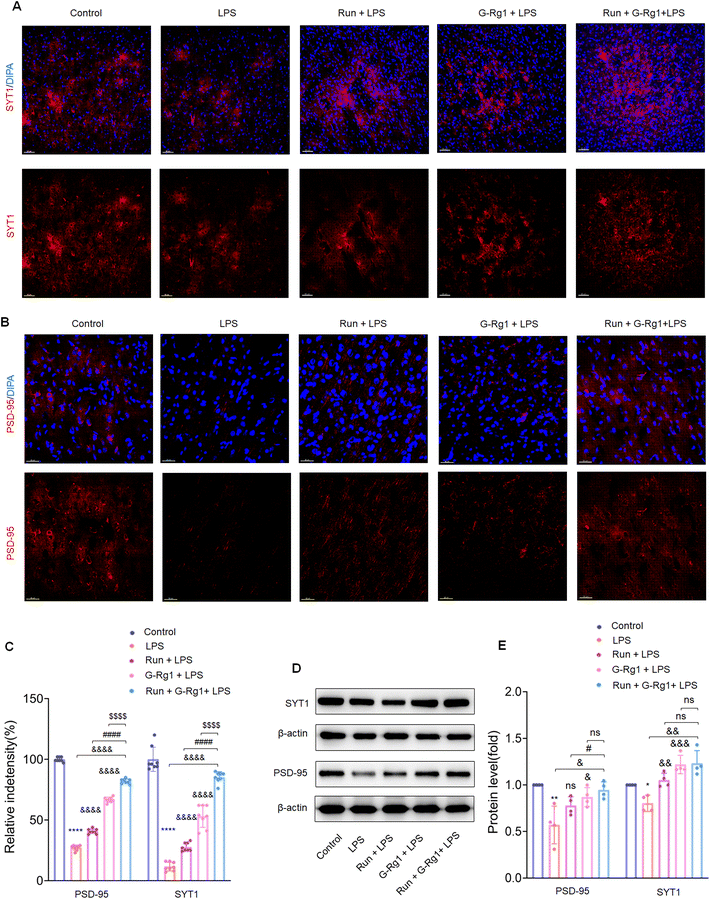 |
| Fig. 8 Ginsenoside-Rg1 synergized voluntary wheel running treatment up-regulates synaptic-related protein expression. (A and B) Immunofluorescence shows SYT1 and PSD-95 within the hippocampus. (C) Histogram shows the fluorescence intensities in the hippocampus neurons of each group. Nuclei (blue) are stained with DAPI. Scale bar is 30 μm. N = 8 per group. (D) Western blot analysis of SYT1 and PSD-95 levels within the hippocampus. (E) Normalized intensity bands are presented as the means ± SEM. A one-way analysis of variance (ANOVA) with the Tukey post-hoc correction was used for analysis. | |
3.6 Ginsenoside-Rg1 synergized voluntary wheel running treatment ameliorated LPS-induced alterations in hippocampal synaptic structure
Next, we investigated the synaptic ultrastructure of hippocampal neurons utilizing transmission electron microscopy. ANOVA analysis revealed a significant change among the five groups in the synaptic density and the vesicle number per unit volume within the hippocampal neurons (Fig. 9A and B). As demonstrated in Fig. 9A, a 2-week LPS treatment significantly decreased the number of hippocampus synapses and vesicles (P < 0.0001), while the regular synergized treatment extensively ameliorated this synaptic loss (Fig. 9C and D) (P < 0.05).
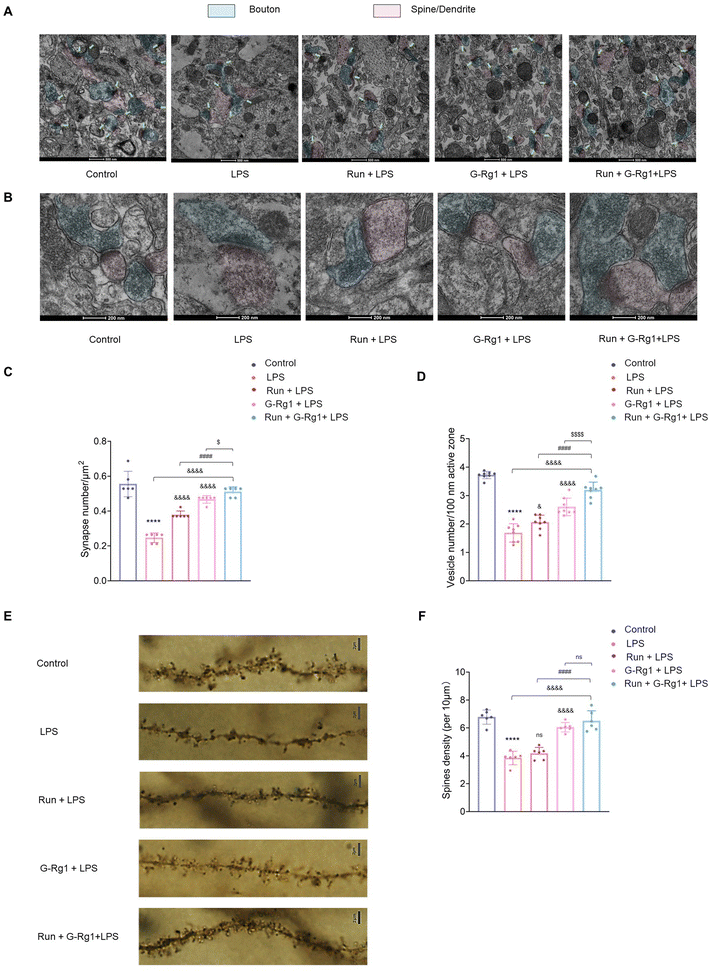 |
| Fig. 9 Ginsenoside-Rg1 synergized voluntary wheel running treatment attenuated LPS-induced changes in synaptic structure. (A) Representative electron micrograph of hippocampus neurons in rats from each group. Arrows indicate spine synapses. (B) Representative TEM images of docked vesicles. Scale bar: 200 nm. (C) Quantification of synapse number. (D) Quantification of synaptic vesicles number. (E) Representative Golgi-stained images of hippocampus neurons in rats from each group. Scale bar: 2 μm. (F) Quantification of mushroom spines in neurons. All values are presented as means ± SEM. N = 6 per group. A one-way analysis of variance (ANOVA) with the Tukey post-hoc correction used for analysis. | |
In addition, we also used the Golgi staining assay to evaluate dendritic spine densities. Results demonstrated that the thickness of dendritic spines was significantly distinct among the five groups (Fig. 9E and F). LPS treatment resulted in a decline in the density of dendritic spines compared to that of the control group (P < 0.0001), which was improved by the synergized treatment more significantly (P < 0.0001). These morphological outcomes indicate that the neuroprotective capability of this synergized treatment on the hippocampal neuronal structure may be one of the essential automatic bases for its antidepressant-like impact.
4. Discussion
Depression is a multifaceted and complex illness with a high morbidity and mortality rate, as well as a significant probability of recurrence and repercussions.40,41 However, classic antidepressants have many side effects, and 40% of people do not respond to them.42 In this study, we demonstrated that LPS could elicit depression-like behavior in animals and that ginsenoside-Rg1 or voluntary wheel running have antidepressant effects in rat models of depression. More importantly, we found that the synergistic treatment with ginsenoside-Rg1 and voluntary running demonstrate a better antidepressant-like effect than either of them alone. This synergistic treatment exerts antidepressant-like effects by inhibiting the activation of glial cells, suppressing the production of inflammatory factors, increasing the level of p-CREB and BDNF expression, and ameliorating the structural impairment on dendritic spines and synapses. In conclusion, our study suggests that neuroinflammation and structural damage of neurons may play a critical role in the development of depression-like behavior, while the synergistic treatment could ameliorate these neuronal changes through its stronger neuroprotective potential, showing a more potent antidepressant-like effect.
In the present study, LPS treatment in rats dramatically increased immobility durations in the FST and decreased the percentage of sucrose consumption in the SPT, two major markers of behavioral despair and anhedonia, which are core symptoms of depression.43 In addition, our experimental findings revealed that LPS-treated rats had significantly reduced central area residence time in the OFT, as well as significantly reduced time spent in the open arm of EPM and number of entries into the open arm, which indicated that the rats showed anxiety-like behavior. Morris water maze37,44 was used to evaluate spatial learning and memory and cognitive function in rats. The results demonstrated that the learning and memory capacities of rats treated with LPS were considerably impaired. More meaningfully, our results showed that although treatment with ginsenoside-Rg1 or voluntary running may alleviate these depressive-like behavior in rats, the synergized treatment showed more significant effects than either of them alone. Therefore, these results provided evidence that synergized treatment is more significant for depression treatment.
Neuroinflammation is considered crucial to the pathophysiology of depression, similar to numerous neurological diseases.45–47 Microglia are immune cells that permanently dwell in the brain usually respond to a wide variety of neuropathological stimuli, such as anxiety, physical trauma, and infectious diseases.48 Microglial density and morphological changes multiply fast once they have been activated, followed by the release of pro-inflammatory cytokines such as IL-1β, IL-6, tumor necrosis factor-α, and interferon-γ.49,50 The inflammatory cytokines could cause neuronal damage in the brain and might be therapeutic targets for depression treatment.49,51,52 In addition, the activation of astrocytes could result in the production of other neurotoxic substances, including cytokines. For this reason, suppressing neuroinflammation is becoming increasingly important in developing therapeutic therapies for brain injury.52 Our previous study showed that inhibiting COX2/PGE2 mediated neuroinflammatory pathway by celecoxib ameliorates depression-like behavior via suppressing glial activation and neuronal deterioration in rats.53 In the present study, our findings demonstrate that the presence of depressive-like symptoms is related to enhanced microglial and astrocyte activation throughout the hippocampus and the vmPFC. However, ginsenoside-Rg1 treatment inhibited inflammatory cytokine levels and glial activation, similar to our previous reports.17,18,21 It has been previously reported that physical activity and exercise reduce neuroinflammation and exert neuroprotective effects in neurological diseases.54–56 In this report, we also found that voluntary wheel running reduced the LPS-induced inflammation in the hippocampus and vmPFC region. More importantly, in the current study, we first demonstrated that ginsenoside-Rg1 synergized with voluntary running treatment showed better effects on attenuating glial activation. These results proved that this synergized treatment exerts a more significant antidepressant effect via suppressing neuroinflammatory responses.
Neuroinflammation usually results in structural and functional injury and thus impairs the synaptic transmission. We then evaluated the levels of synaptic plasticity-related proteins, p-CREB and BDNF, within the hippocampus and vmPFC in order to discover whether modulation of neuroplasticity is involved in the antidepressant-like effects of this synergized treatment. Although our previous study has shown that ginsenoside-Rg1 can rescue the decrease of BDNF and p-CREB protein levels induced by LPS treatment,17,36 and other studies showed that exercise could increase p-CREB and BDNF expression.50,57,58 The present study showed that the synergized treatment improved BDNF and p-CREB protein expression more significantly in LPS-rats than either ginsenoside-Rg1 or voluntary wheel running, indicating that this synergized treatment exerts more significant therapeutic effects through its neuroprotective capacity based on the p-CREB/BDNF signaling pathways.
In addition, accumulating research indicates that alterations in synaptic function are frequently regulated with a variety of structurally related proteins within the synapse.59,60 Synaptophysin is the principal presynaptic synaptic vesicle protein involved in vesicle fusion and synaptic transmission.35 PSD-95, a primary scaffold protein in excitatory postsynaptic density, is an essential element involved in synaptic plasticity. Therefore, reduced synaptic proteins may cause ultrastructural alterations, such as decreased synaptic density in the LPS-induced depression model. Most significantly, present results showed that the synergized treatment prevented the decrease in synaptophysin and PSD-95 expression, thereby improving structural and functional plasticity changes associated with depression-like behavior.
Previous studies showed that aberrant neuronal structure could produce synaptic transmission anomalies and behavioral changes.61,62 In the present study, terminal spine density assessment by Golgi staining assay showed that LPS induced insufficient terminal dendrite spine within hippocampal neurons and a failure of synapses. The synergistic treatment improved the LPS-induced ultrastructural alterations in hippocampal neurons, including increased synapses, PSD thickness, and surface density. Moreover, the synergistic treatment more significantly ameliorated depressive-like behavior in these rats. In summary, it is reasonable to suggest that the underlying antidepressant mechanism of this synergized treatment may be attributed to its neuroprotective capacity to modulate neuronal structural plasticity and demonstrate better therapeutic effects.
5. Conclusion
In conclusion, these results of the study suggest that synergistic pretreatment with ginsenoside-Rg1 and voluntary running can more effectively improve LPS-induced depression-like behavior via better neuroprotective effects than either of the treatments alone. In light of these findings, the therapeutic modality of pharmacological synergistic exercise has the potential to become a new therapeutic modality for the treatment of depression.
Author contributions
S. Y. Y. and S. C. contributed to the study design. W. W. and L. W. (Lihong Wang) performed the biochemical analysis and immunohistochemistry and confocal imaging analysis. W. W. and L. W. (Liyan Wang) performed TEM experiments. Y. L.,T. L. and C. W. performed depression model and behavioral tests. X. C. and W. W. performed Golgi staining experiments. S. Y. Y. and W. W. wrote the first draft. All author approved the final version of the manuscript for publication.
Abbreviations
ANOVA | Analysis of variance |
BDNF | Brain-derived neurotrophic factor |
ELISA | Enzyme linked immunosorbent assay |
EPM | Elevated plus maze |
FST | Forced swimming test |
IL-1 | Interleukin-1 |
IL-6 | Interleukin-6 |
LPS | Lipopolysaccharide |
MDD | Major depression disorder |
MWM | Morris water maze |
OFT | Open field test |
PSD | Post-synaptic density |
ROS | Reactive oxygen species |
SPT | Sucrose preference test |
TEM | Transmission electron microscopy |
TNF-a | Tumor necrosis factor-a |
WHO | World health organization |
Ethics approval and consent to participate
The Ethics Committee at Shandong University Animal Care and Use Committee (Jinan, China) approved the protocols of this study. All experiments were conducted in accordance with the National Institutes of Health Guide for the Care and Use of Laboratory Animals (National Research Council, 1996).
Consent for publication
All authors agreed to publish.
Data availability
The data that support the findings of this study are available from the corresponding author upon reasonable request.
Conflicts of interest
The authors declare that they have no known competing financial interests or personal relationships that could have appeared to influence the work reported in this paper.
Acknowledgements
This study was supported by the National Natural Science Foundation of China (NSFC82271566 and NSFC82070847). We thank the staff of the electron microscope experimental center of medical morphology in the basic medical school for technical assistance.
References
- G. S. Malhi and J. J. Mann, Depression, Lancet, 2018, 392, 2299–2312 CrossRef PubMed.
- J. Hiser and M. Koenigs, The Multifaceted Role of the Ventromedial Prefrontal Cortex in Emotion, Decision Making, Social Cognition, and Psychopathology, Biol. Psychiatry, 2018, 83, 638–647 CrossRef PubMed.
- C. Jiang, W. J. Lin, B. Labonte, C. A. Tamminga, G. Turecki, E. J. Nestler, S. J. Russo and S. R. Salton, VGF and its C-terminal peptide TLQP-62 in ventromedial prefrontal cortex regulate depression-related behaviors and the response to ketamine, Neuropsychopharmacology, 2019, 44, 971–981 CrossRef CAS PubMed.
- W. C. Drevets, J. L. Price and M. L. Furey, Brain structural and functional abnormalities in mood disorders: implications for neurocircuitry models of depression, Brain Struct. Funct., 2008, 213, 93–118 CrossRef PubMed.
- E. L. Belleau, M. T. Treadway and D. A. Pizzagalli, The Impact of Stress and Major Depressive Disorder on Hippocampal and Medial Prefrontal Cortex Morphology, Biol. Psychiatry, 2019, 85, 443–453 CrossRef PubMed.
- M. S. Tan, J. T. Yu, T. Jiang, X. C. Zhu and L. Tan, The NLRP3 inflammasome in Alzheimer's disease, Mol. Neurobiol., 2013, 48, 875–882 CrossRef CAS PubMed.
- N. Zhang, X. Zhang, X. Liu, H. Wang, J. Xue, J. Yu, N. Kang and X. Wang, Chrysophanol inhibits NALP3 inflammasome activation and ameliorates cerebral ischemia/reperfusion in mice, Mediators Inflammation, 2014, 2014, 370530 Search PubMed.
- F. Calabrese, A. C. Rossetti, G. Racagni, P. Gass, M. A. Riva and R. Molteni, Brain-derived neurotrophic factor: a bridge between inflammation and neuroplasticity, Front. Cell. Neurosci., 2014, 8, 430 Search PubMed.
- F. Vasile, E. Dossi and N. Rouach, Human astrocytes: structure and functions in the healthy brain, Brain Struct. Funct., 2017, 222, 2017–2029 CrossRef CAS PubMed.
- M. E. Tremblay, B. Stevens, A. Sierra, H. Wake, A. Bessis and A. Nimmerjahn, The role of microglia in the healthy brain, J. Neurosci., 2011, 31, 16064–16069 CrossRef CAS PubMed.
- R. Yirmiya, N. Rimmerman and R. Reshef, Depression as a microglial disease, Trends Neurosci., 2015, 38, 637–658 CrossRef CAS PubMed.
- L. Leng, K. Zhuang, Z. Liu, C. Huang, Y. Gao, G. Chen, H. Lin, Y. Hu, D. Wu, M. Shi, W. Xie, H. Sun, Z. Shao, H. Li, K. Zhang, W. Mo, T. Y. Huang, M. Xue, Z. Yuan, X. Zhang, G. Bu, H. Xu, Q. Xu and J. Zhang, Menin Deficiency Leads to Depressive-like Behaviors in Mice by Modulating Astrocyte-Mediated Neuroinflammation, Neuron, 2018, 100, 551–563 CrossRef CAS PubMed.
- O. J. Schiepers, M. C. Wichers and M. Maes, Cytokines and major depression, Prog. Neuropsychopharmacol. Biol. Psychiatry, 2005, 29, 201–217 CrossRef CAS PubMed.
- J. K. Kiecolt-Glaser, H. M. Derry and C. P. Fagundes, Inflammation: depression fans the flames and feasts on the heat, Am. J. Psychiatry, 2015, 172, 1075–1091 CrossRef PubMed.
- B. Jiang, Z. Xiong, J. Yang, W. Wang, Y. Wang, Z. L. Hu, F. Wang and J. G. Chen, Antidepressant-like effects of ginsenoside Rg1 are due to activation of the BDNF signalling pathway and neurogenesis in the hippocampus, Br. J. Pharmacol., 2012, 166, 1872–1887 CrossRef CAS PubMed.
- C. Jin, Z. Z. Wang, H. Zhou, Y. X. Lou, J. Chen, W. Zuo, M. T. Tian, Z. Q. Wang, G. H. Du, I. Kawahata, T. Yamakuni, Y. Zhang, N. H. Chen and D. S. Zhang, Ginsenoside Rg1-induced antidepressant effects involve the protection of astrocyte gap junctions within the prefrontal cortex, Prog. Neuropsychopharmacol. Biol. Psychiatry, 2017, 75, 183–191 CrossRef CAS PubMed.
- Z. Liu, Y. Qi, Z. Cheng, X. Zhu, C. Fan and S. Y. Yu, The effects of ginsenoside Rg1 on chronic stress induced depression-like behaviors, BDNF expression and the phosphorylation of PKA and CREB in rats, Neuroscience, 2016, 322, 358–369 CrossRef CAS PubMed.
- C. Fan, Q. Song, P. Wang, Y. Li, M. Yang and S. Y. Yu, Neuroprotective Effects of Ginsenoside-Rg1 Against Depression-Like Behaviors via Suppressing Glial Activation, Synaptic Deficits, and Neuronal Apoptosis in Rats, Front. Immunol., 2018, 9, 2889 CrossRef CAS PubMed.
- X. Zhu, R. Gao, Z. Liu, Z. Cheng, Y. Qi, C. Fan and S. Y. Yu, Ginsenoside Rg1 reverses stress-induced depression-like behaviours and brain-derived neurotrophic factor expression within the prefrontal cortex, Eur. J. Neurosci., 2016, 44, 1878–1885 CrossRef PubMed.
- Y. Li, L. Wang, P. Wang, C. Fan, P. Zhang, J. Shen and S. Y. Yu, Ginsenoside-Rg1 Rescues Stress-Induced Depression-Like Behaviors via Suppression of Oxidative Stress and Neural Inflammation in Rats, Oxid. Med. Cell. Longevity, 2020, 2020, 2325391 Search PubMed.
- H. Yu, C. Fan, L. Yang, S. Yu, Q. Song, P. Wang and X. Mao, Ginsenoside Rg1 Prevents Chronic Stress-Induced Depression-Like Behaviors and Neuronal Structural Plasticity in Rats, Cell. Physiol. Biochem., 2018, 48, 2470–2482 CrossRef CAS PubMed.
- C. Fan, X. Zhu, Q. Song, P. Wang, Z. Liu and S. Y. Yu, MiR-134 modulates chronic stress-induced structural plasticity and depression-like behaviors via downregulation of Limk1/cofilin signaling in rats, Neuropharmacology, 2018, 131, 364–376 CrossRef CAS PubMed.
- T. Josefsson, M. Lindwall and T. Archer, Physical exercise intervention in depressive disorders: meta-analysis and systematic review, Scand. J. Med. Sci. Sports, 2014, 24, 259–272 CrossRef CAS PubMed.
- K. M. C. Dallagnol, A. P. Remor, R. A. da Silva, R. D. Prediger, A. Latini and A. S. Aguiar Jr., Running for REST: Physical activity attenuates neuroinflammation in the hippocampus of aged mice, Brain, Behav., Immun., 2017, 61, 31–35 CrossRef CAS PubMed.
- C. J. Caspersen, K. E. Powell and G. M. Christenson, Physical-Activity, Exercise, and Physical-Fitness - Definitions and Distinctions for Health-Related Research, Public Health Rep., 1985, 100, 126–131 CAS.
- M. P. Cunha, A. Oliveira, F. L. Pazini, D. G. Machado, L. E. Bettio, J. Budni, A. S. Aguiar Jr., D. F. Martins, A. R. Santos and A. L. Rodrigues, The antidepressant-like effect of physical activity on a voluntary running wheel, Med. Sci. Sports Exercise, 2013, 45, 851–859 CrossRef CAS PubMed.
- C. Knochel, V. Oertel-Knochel, L. O'Dwyer, D. Prvulovic, G. Alves, B. Kollmann and H. Hampel, Cognitive and behavioural effects of physical exercise in psychiatric patients, Prog. Neurobiol., 2012, 96, 46–68 CrossRef PubMed.
- C. Bridle, K. Spanjers, S. Patel, N. M. Atherton and S. E. Lamb, Effect of exercise on depression severity in older people: systematic review and meta-analysis of randomised controlled trials, Br. J. Psychiatry, 2012, 201, 180–185 CrossRef PubMed.
- P. Wang, Y. B. Feng, L. Wang, Y. Li, C. Fan, Q. Song and S. Y. Yu, Interleukin-6: Its role and mechanisms in rescuing depression-like behaviors in rat models of depression, Brain, Behav., Immun., 2019, 82, 106–121 CrossRef CAS PubMed.
- Y. Li, C. Fan, L. Wang, T. Lan, R. Gao, W. Wang and S. Y. Yu, MicroRNA-26a-3p rescues depression-like behaviors in male rats via preventing hippocampal neuronal anomalies, J. Clin. Invest., 2021, 131(16), e148853 CrossRef CAS PubMed.
- C. H. Duman, L. Schlesinger, M. Kodama, D. S. Russell and R. S. Duman, A role for MAP kinase signaling in behavioral models of depression and antidepressant treatment, Biol. Psychiatry, 2007, 61, 661–670 CrossRef CAS PubMed.
- R. D. Porsolt, M. Le Pichon and M. Jalfre, Depression: a new animal model sensitive to antidepressant treatments, Nature, 1977, 266, 730–732 CrossRef CAS PubMed.
- H. Li, Y. Xiang, Z. Zhu, W. Wang, Z. Jiang, M. Zhao, S. Cheng, F. Pan, D. Liu, R. C. M. Ho and C. S. H. Ho, Rifaximin-mediated gut microbiota regulation modulates the function of microglia and protects against CUMS-induced depression-like behaviors in adolescent rat, J. Neuroinflammation, 2021, 18, 254 CrossRef CAS PubMed.
- W. Wang, R. Wang, Z. Jiang, H. Li, Z. Zhu, A. Khalid, D. Liu and F. Pan, Inhibiting Brd4 alleviated PTSD-like behaviors and fear memory through regulating immediate early genes expression and neuroinflammation in rats, J. Neurochem., 2021, 158, 912–927 CrossRef CAS PubMed.
- J. Xu, R. Wang, Y. Liu, D. Liu, H. Jiang and F. Pan, FKBP5 and specific microRNAs via glucocorticoid receptor in the basolateral amygdala involved in the susceptibility to depressive disorder in early adolescent stressed rats, J. Psychiatr. Res., 2017, 95, 102–113 CrossRef PubMed.
- L. Hritcu and L. D. Gorgan, Intranigral lipopolysaccharide induced anxiety and depression by altered BDNF mRNA expression in rat hippocampus, Prog. Neuropsychopharmacol. Biol. Psychiatry, 2014, 51, 126–132 CrossRef CAS PubMed.
- T. Lan, Y. Li, C. Fan, L. Wang, W. Wang, S. Chen and S. Y. Yu, MicroRNA-204–5p reduction in rat hippocampus contributes to stress-induced pathology via targeting RGS12 signaling pathway, J. Neuroinflammation, 2021, 18, 243 CrossRef CAS PubMed.
- B. Minnich, H. Leeb, E. W. Bernroider and A. Lametschwandtner, Three-dimensional morphometry in scanning electron microscopy: a technique for accurate dimensional and angular measurements of microstructures using stereopaired digitized images and digital image analysis, J. Microsc., 1999, 195, 23–33 CrossRef CAS PubMed.
- J. H. Lee, J. Y. Kim, S. Noh, H. Lee, S. Y. Lee, J. Y. Mun, H. Park and W. S. Chung, Astrocytes phagocytose adult hippocampal synapses for circuit homeostasis, Nature, 2021, 590, 612–617 CrossRef CAS PubMed.
- C. B. Nemeroff, The State of Our Understanding of the Pathophysiology and Optimal Treatment of Depression: Glass Half Full or Half Empty?, Am. J. Psychiatry, 2020, 177, 671–685 CrossRef PubMed.
- M. Ii Timberlake and Y. Dwivedi, Linking unfolded protein response to inflammation and depression: potential pathologic and therapeutic implications, Mol. Psychiatry, 2019, 24, 987–994 CrossRef PubMed.
- M. E. Fox and M. K. Lobo, The molecular and cellular mechanisms of depression: a focus on reward circuitry, Mol. Psychiatry, 2019, 24, 1798–1815 CrossRef PubMed.
- C. Fan, Y. Li, T. Lan, W. Wang, X. Mao and S. Y. Yu, Prophylactic treatment of curcumin in a rat model of depression by attenuating hippocampal synaptic loss, Food Funct., 2021, 12, 11202–11213 RSC.
- C. V. Vorhees and M. T. Williams, Morris water maze: procedures for assessing spatial and related forms of learning and memory, Nat. Protoc., 2006, 1, 848–858 CrossRef PubMed.
- E. Beurel, M. Toups and C. B. Nemeroff, The Bidirectional Relationship of Depression and Inflammation: Double Trouble, Neuron, 2020, 107, 234–256 CrossRef CAS PubMed.
- H. D. Liu, W. Li, Z. R. Chen, Y. C. Hu, D. D. Zhang, W. Shen, M. L. Zhou, L. Zhu and C.
H. Hang, Expression of the NLRP3 inflammasome in cerebral cortex after traumatic brain injury in a rat model, Neurochem. Res., 2013, 38, 2072–2083 CrossRef CAS PubMed.
- J. Hannestad, N. DellaGioia and M. Bloch, The effect of antidepressant medication treatment on serum levels of inflammatory cytokines: a meta-analysis, Neuropsychopharmacology, 2011, 36, 2452–2459 CrossRef CAS PubMed.
- P. Cao, C. Chen, A. Liu, Q. Shan, X. Zhu, C. Jia, X. Peng, M. Zhang, Z. Farzinpour, W. Zhou, H. Wang, J. N. Zhou, X. Song, L. Wang, W. Tao, C. Zheng, Y. Zhang, Y. Q. Ding, Y. Jin, L. Xu and Z. Zhang, Early-life inflammation promotes depressive symptoms in adolescence via microglial engulfment of dendritic spines, Neuron, 2021, 109, 2573–2589 CrossRef CAS PubMed.
- J. Y. Lee, S. R. Kang and T. Y. Yune, Fluoxetine prevents oligodendrocyte cell death by inhibiting microglia activation after spinal cord injury, J. Neurotrauma, 2015, 32, 633–644 CrossRef PubMed.
- E. S. Wohleb, T. Franklin, M. Iwata and R. S. Duman, Integrating neuroimmune systems in the neurobiology of depression, Nat. Rev. Neurosci., 2016, 17, 497–511 CrossRef CAS PubMed.
- M. Li, C. Li, H. Yu, X. Cai, X. Shen, X. Sun, J. Wang, Y. Zhang and C. Wang, Lentivirus-mediated interleukin-1beta (IL-1beta) knock-down in the hippocampus alleviates lipopolysaccharide (LPS)-induced memory deficits and anxiety- and depression-like behaviors in mice, J. Neuroinflammation, 2017, 14, 190 CrossRef PubMed.
- M. V. Sofroniew and H. V. Vinters, Astrocytes: biology and pathology, Acta Neuropathol., 2010, 119, 7–35 CrossRef PubMed.
- Q. Song, C. Fan, P. Wang, Y. Li, M. Yang and S. Y. Yu, Hippocampal CA1 βCaMKII mediates neuroinflammatory responses via COX-2/PGE2 signaling pathways in depression, J. Neuroinflammation, 2018, 15, 338 CrossRef CAS PubMed.
- S. Kvam, C. L. Kleppe, I. H. Nordhus and A. Hovland, Exercise as a treatment for depression: A meta-analysis, J. Affect. Disord., 2016, 202, 67–86 CrossRef PubMed.
- L. J. Spielman, J. P. Little and A. Klegeris, Physical activity and exercise attenuate neuroinflammation in neurological diseases, Brain Res. Bull., 2016, 125, 19–29 CrossRef CAS PubMed.
- T. Tuon, S. S. Valvassori, G. C. Dal Pont, C. S. Paganini, B. G. Pozzi, T. F. Luciano, P. S. Souza, J. Quevedo, C. T. Souza and R. A. Pinho, Physical training prevents depressive symptoms and a decrease in brain-derived neurotrophic factor in Parkinson's disease, Brain Res. Bull., 2014, 108, 106–112 CrossRef CAS PubMed.
- A. M. Sohroforouzani, S. Shakerian, M. Ghanbarzadeh and H. Alaei, Effect of forced treadmill exercise on stimulation of BDNF expression, depression symptoms, tactile memory and working memory in LPS-treated rats, Behav. Brain Res., 2022, 418, 113645 CrossRef CAS PubMed.
- S. S. Zhang, L. Zhu, Y. Peng, L. Zhang, F. L. Chao, L. Jiang, Q. Xiao, X. Liang, J. Tang, H. Yang, Q. He, Y. J. Guo, C. N. Zhou and Y. Tang, Long-term running exercise improves cognitive function and promotes microglial glucose metabolism and morphological plasticity in the hippocampus of APP/PS1 mice, J. Neuroinflammation, 2022, 19, 34 CrossRef CAS PubMed.
- C. A. Stockmeier, G. J. Mahajan, L. C. Konick, J. C. Overholser, G. J. Jurjus, H. Y. Meltzer, H. B. Uylings, L. Friedman and G. Rajkowska, Cellular changes in the postmortem hippocampus in major depression, Biol. Psychiatry, 2004, 56, 640–650 CrossRef PubMed.
- A. Vyas, R. Mitra, B. S. Shankaranarayana Rao and S. Chattarji, Chronic stress induces contrasting patterns of dendritic remodeling in hippocampal and amygdaloid neurons, J. Neurosci., 2002, 22, 6810–6818 CrossRef CAS PubMed.
- L. Zhang, J. Luo, M. Zhang, W. Yao, X. Ma and S. Y. Yu, Effects of curcumin on chronic, unpredictable, mild, stress-induced depressive-like behaviour and structural plasticity in the lateral amygdala of rats, Int. J. Neuropsychopharmacol., 2014, 17, 793–806 CrossRef CAS PubMed.
- J. Luo, L. Zhang, N. Ning, H. Jiang and S. Y. Yu, Neotrofin reverses the effects of chronic unpredictable mild stress on behavior via regulating BDNF, PSD-95 and synaptophysin expression in rat, Behav. Brain Res., 2013, 253, 48–53 CrossRef CAS PubMed.
|
This journal is © The Royal Society of Chemistry 2023 |