DOI:
10.1039/D2FO04111A
(Paper)
Food Funct., 2023,
14, 7615-7630
Comparison of the laxative effects of Korean Gochujang containing different microbiota on loperamide-induced constipation in ICR mice†
Received
30th December 2022
, Accepted 1st June 2023
First published on 3rd August 2023
Abstract
The prevalence of constipation, one of the common gastrointestinal (GI) diseases, has been gradually increasing. Gochujang, a traditional Korean fermented paste, has various microbiota and exerts diverse health beneficial effects. However, the ameliorative effect of Gochujang on constipation is unexplored. Seven-week-old ICR mice were divided into five groups: the normal group, the loperamide (LOP) group, the LOP + mosapride citrate (3 mg per kg BW, MOSA) treated group, the LOP + BMG Gochujang (2 g per kg BW) group, and the LOP + VMG Gochujang (2 g per kg BW) group. Gochujang alleviated constipation by increasing defecation frequency and water content in feces by reducing AQP3 mRNA expression. Additionally, Gochujang increased GI transit time and excitatory neurotransmitter levels and decreased inhibitory neurotransmitter levels. Moreover, Gochujang reduced mitogen-activated protein kinase (MAPK) activation and increased the c-Kit/SCF signaling pathway, suggesting that Gochujang regulates the enteric nervous system (ENS). Interestingly, BMG and VMG differently influenced the gut microbiota composition. Both Gochujang groups significantly decreased the Bacteroidetes and Firmicutes ratio compared to the LOP group. However, among Firmicutes genera, Acetatifactor was only reduced in BMG, and VMG only decreased Caproiciproducens and Acutalibacter. In summary, Gochujang effectively alleviated LOP-induced constipation outcomes regardless of their different microbial communities by ameliorating GI motility and changing the gut microbiota composition.
1. Introduction
Constipation is characterized by unsatisfactory or infrequent defecation and is one of the common gastrointestinal (GI) diseases affecting approximately 20% of the adult population.1 The incidence of constipation causes inconveniences and pain both physically and mentally to the patients. The general medications for constipation include osmotic laxatives (e.g., lactulose and polyethylene glycol), stimulant laxatives (e.g., bisacodyl and senna), an ileal bile acid transport inhibitor (e.g., elobixibat), and serotonergic agents (e.g., mosapride citrate and prucalopride).2 However, long-term intake of these drugs is associated with severe side effects such as myocardial infarction, artery contraction, coronary spasms, and the development of drug resistance.3,4 Hence, the significance of nutritional and/or diet therapeutic approaches for constipation has been emphasized to lessen the adverse effects of pharmacological agents.5
The gut microbiota is the community of microorganisms that live in the GI tract of vertebrates and is significantly influenced by diet. Recent studies have shown that the gut microbiota is strongly related to constipation.6,7 The community of the gut microbiota in constipation patients differs from that in healthy subjects;8 for example, constipation patients have lower levels of Bifidobacterium, Lactobacillus, and Bacteroides compared to healthy individuals. Additionally, the gut microbiota plays significant roles in GI motility and the neuroendocrine system.9 Germ-free rats restored normal levels of intestinal transit time after intestinal colonization with Lactobacillus and Bifidobacterium.10 Moreover, the gut microbiota directly and/or indirectly alters gut microbiota-derived metabolite production and GI neuroendocrine factors.11,12
The traditional Korean fermented foods, including Cheonggukjang, Doenjang, and Gochujang, are made based on meju, a naturally fermented soybean functioning as a starter of fermentation.13,14Gochujang is a primary spicy sauce in Korean cuisine and it is a mixture of meju and red pepper powder.15 Recent studies have demonstrated that the intake of fermented soybean foods alters the gut microbiota composition since its microorganisms act as probiotics and prebiotics.16 Indeed, Jeong et al. reported that the consumption of Cheonggukjang increased the relative abundances of Lactobacillales and Bifidobacteriales and decreased harmful bacteria.16,17 Interestingly, the meju microbiota is influenced by various factors, including weather conditions, provinces, and manufacturers, altering the microbiota composition of Gochujang subsequently.13,18,19 Although the general microbial composition of Gochujang and its health beneficial functions have been studied well,14,20–23 the dissimilar physiological effects of Gochujang based on its distinct microbial communities have not been fully investigated. Therefore, the aims of this study were: (1) to study the constipation ameliorative effects of two different types of Gochujangs in aspects of their microbial communities; (2) to investigate their underlying molecular mechanisms, and (3) to measure the distinct effects of two different types of Gochujangs on the gut microbiota composition.
2. Materials and methods
2.1. NGS analysis
Bacterial analysis is conducted by next-generation sequencing (NGS) analysis. Bacterial genomic DNA was extracted using a Fast DNA Spin kit (MP Bio Laboratories, USA). The primer set pairs were 27F (5′-CCTATCCCCTGTGTGCCTTGGCAGTC (adapter) – TCAG (key)-AC linker-GAGTTTGATCMTGGCTCAG-3′) and 518R (5′-CCATCTCATCCC TGCGTGTCTCCGAC (adapter)-TCAG (key)-Barcode-AC linker-WTTA CCGCGGCTGCTGG-3′). A PCR was conducted on 16S rDNA genes. The PCR cycle was performed as follows: 94 °C for 5 min, 94 °C for 30 s, 60 °C for 45 s, and 72 °C for 90 s × 10 cycles. 94 °C for 30 s, 55 °C for 45 s, and 72 °C for 90 s × 20 cycles.
Ez Taxon database PKSSU 4.0 was used as a database for taxonomic analysis in NGS analysis. Also, Jensen–Shannon was used as an algorithm for beta-diversity analysis. LEfSe (linear discriminant analysis effect size) analysis was used for the comparison of taxonomic biomarker discovery between different Gochujang types.
2.2. The selection of Gochujang
The Microbial Institute for Fermentation Industry (Sunchang-gun, Jeollabuk-do, Korea) collected 29 kinds of Gochujangs and selected and provided two Gochujang types after screening through NGS analysis and quality inspection (ESI Fig. S1, S2 and Table S1†). In general, Gochujang is made by mixing glutinous rice powder, fermented soybean powder, salt, and water with pepper powder as the main ingredient, then putting it in an earthenware, sealing the lid, and fermenting it in a cool place. During the manufacturing process, the ratio, temperature, and humidity of the ingredients vary depending on the region and the manufacturer.
2.3. Experimental protocols for animal studies
All animal-related protocols were endorsed by the Animal Ethics Committee of Jeonbuk National University (CBNU 2020-089). Seven-week-old ICR mice were purchased from DooYeol Biotech (Seoul, Korea). Upon arrival, mice were housed in a light-controlled room (12 h) at 23 ± 2 °C with humidity regulated at 55 ± 5%. They were fed an AIN-93 laboratory diet and allowed to drink water unrestrictedly. Following a one-week adaptation period, mice were divided into five groups (n = 10): the non-constipation group (ND group), the LOP + vehicle treated group (LOP group), the LOP + mosapride citrate treated group (MOSA group), the LOP + BMG Gochujang group (BMG group), and the LOP + VMG Gochujang group (VMG group). LOP-induced mild constipation groups such as LOP, MOSA, BMG, and VMG groups were orally administered LOP (Sigma-Aldrich, MO, USA) in 0.9% sodium chloride at 5 mg per kg body weight (BW), twice a day for 14 days. The MOSA group received oral administration of mosapride citrate solution (3 mg per kg BW). BMG and VMG groups were also orally administered the individual Gochujang solutions at 2 g per kg BW. Distilled water was used for dissolving the samples and each mouse was orally administered 200 μl of it for 14 days, once a day. The ND and LOP groups received oral administration of the same amount of distilled water. ICR mice were sacrificed after 12 h of overnight fasting and tissue samples were collected and stored at −72 °C until further assay.
2.4. Analysis of body weight, feed intake, and water consumption
The body weight, water consumption volume, and the feed intake of experimental mice were measured daily throughout the experimental period. The water consumption and feed intake were calculated for each cage. All measurements were performed three times for accuracy.
2.5. Measurement of urine volume and feces parameters
All ICR mice were kept in metabolic cages to collect pure urine and feces during the experimental period without contamination on experimental days 0 and 10. The urine and feces excreted from each cage were collected at 10:00 A.M. The urine volume was measured using a cylinder. The feces weight of one cage was weighed using an electric balance. Its water content was calculated using the following formula:
The wet weight denotes the weight of natural feces collected from ICR mice and the dry weight represents the weight after drying at 70 °C for 24 h on the first and tenth days. All measurements were executed three times per sample. For each group, the change in day 10 compared to day 0 was calculated and the statistically significant difference was compared between groups.
2.6. Gastrointestinal transit
On the sacrifice day, gastrointestinal transit experiments were performed after overnight fasting. 5% carbon powder was dissolved in distilled water. At 30 min before mice were sacrificed, it was orally administered to the mice to measure the gastrointestinal transit, which was calculated as:
2.7. Histological analysis
Colon tissue was fixed in 10% formalin for 48 h and hematoxylin and eosin (H&E) staining was performed at the Korean Pathology Technical Center (KP&T, Cheongju-si, Korea). The samples were analyzed by optical microscopy (DM2500, Leica, Germany) at the Center for University-wide Research Facilities (CURF) of Jeonbuk National University. The Image-J program (US National Institutes of Health, Bethesda, MD, USA) was used to measure the length of the muscle, mucosa layer, and flat luminal surface thickness.
2.8. Enzyme-linked immunosorbent assay (ELISA) analysis
To measure acetylcholinesterase (AChE, Cat# ab138871, Abcam, Cambridge, UK), motilin (MTL, Cat# KTE70927, Abbkine, Inc., China), and gastrin (GAS, Cat# EIA-GAS, RayBiotech, Norcross, GA, USA), experimental mouse colon tissue was homogenized and analyzed via commercial ELISA kits according to the manufacturer's instructions. A microplate reader (MRX II, Dynex Technologies, Chantilly, VA, USA) was used to determine absorbance.
2.9. Quantitative real-time PCR (qRT-PCR) analysis
Real time-PCR (Applied Biosystems, Waltham, MA, USA) with the SYBR Green qPCR Mix (Toyobo, Osaka, Japan) was used to analyze the mRNA expressions of genes in colon tissue. The QIAGEN RNAeasy mini kit (QIAGEN GmbH, Hilden, Germany) was used to extract the total RNA. After determining the purity of RNA, the Prime Script RT Master Mix (Takara, Kyoto, Japan) was used to synthesize cDNA. Table 1 shows the primers used in RT-PCR.
Table 1 List of primers used for qRT-PCR
Gene |
Primers |
Sequence (5′ → 3′) |
AQP3, aquaporin 3; BDNF, brain-derived neurotrophic factor; GDNF, glial cell line-derived neurotrophic factor; NOS, nitric oxide synthase; TRPV1, transient receptor potential cation channel subfamily V member 1; SCF, stem cell factor; mAChR M2, muscarinic acetylcholine receptors M2; mAChR M3, muscarinic acetylcholine receptors M3. |
AQP3 |
Forward |
GCCAAGGTAGGATAGCAAATAA |
Reverse |
TTGAAAACTTGGTCCCTTGC |
BDNF |
Forward |
CAATCGCTTCATCTTAGGAGT |
Reverse |
TAAACGGCACAAAACAATC |
GDNF |
Forward |
GACGCTTGGTGGTTGATTCTG |
Reverse |
GTTTCTGAGGGCACGAAGGAG |
NOS |
Forward |
TCAGCGGTGATAGGATAAAGCA |
Reverse |
CGCTGTGCTAAGTAGCCCTCG |
TRPV1 |
Forward |
TCTCGTGGAGCCCTTGAACCG |
Reverse |
CCGATAGTAAGCAGCCGTGGT |
c-Kit |
Forward |
CCGACGCAACTTCCTTATGAT |
Reverse |
TCAGGACCTTCAGTTCCGACA |
SCF |
Forward |
ATAGTGGATGACCTCGTGTTA |
Reverse |
GAATCTTTCTCGGGACCTAAT |
mAChR M2 |
Forward |
GCGGATCCTGTGGCCAACCAAGAC |
Reverse |
CGAATTCACGATTTTGCGGGCTA |
mAChR M3 |
Forward |
AAGGCACGAAACGGTCATCT |
Reverse |
GCAAACCTCTTAGCCAGCGT |
2.10. Western blotting analysis
Immunoblot analysis was executed to discover the relative protein expression of MAPK and c-Kit/SCF signaling pathways in the colon mucosa. Phosphor ERK1/2 (#9101), ERK1/2 (#9102), phosphor JNK (#9251), JNK (#9252), phosphor p38 (#9211), p38 (#9212), c-Kit (#3392), and SCF (#2273) were obtained from Cell Signaling Technology (Beverly, MA, USA) and β-actin (#sc-47778) was purchased from Santa Cruz Biotechnology Inc. (Dallas, TX, USA). Colon tissue was put in and homogenized in the lysis buffer. The supernatant was measured for the protein concentration after the centrifugation. All the samples were matched to an equal protein concentration, mixed with 5× protein buffer, and heated to 95 °C. Each sample was electrophoresed on SDS-polyacrylamide gels and transferred to PVDF membranes (Merck, Massachusetts, U.S.A.) followed by blotting with antibodies.
2.11. Statistical analysis
Statistical significance was evaluated using one-way ANOVA in SPSS version 17.0 (SPSS Inc., Chicago, IL, USA), followed by Tukey's post hoc test. The data are presented as means ± standard deviation (SD). *, p < 0.05, **, p < 0.01, and ***, p < 0.001 were considered as statistically significant.
3. Results
3.1. The different microorganisms in BMG and VMG
To compare microorganisms in two different types of Gochujangs, NGS analyses were performed. The microorganisms of BMG were organized into 9 orders. The most abundant order level in the total microorganism community of BMG was Bacillales (99.64%), followed by Lactobacillales (0.16%) (Fig. 1A). In the case of VMG, it was classified into 17 orders, and the representative orders of VMG included Oscillatoriales (66.93%), Enterobacterales (13.53%), and Lactobacillales (8.29%) (Fig. 1B). Table 2 shows the distributions of beneficial and pernicious microorganisms in BMG and VMG. BMG (92.79%) has a relatively higher number of beneficial microorganisms than VMG (2.42%). Meanwhile, VMG (12.52%) displays a relatively higher number of harmful microorganisms compared to BMG (0.06%). Then, we further scrutinized the microorganisms in both BMG and VMG at the species level. Bacillus subtilis (92.77%) was the most dominant bacteria in BMG, whereas Aerosakkonema funiforme (66.93%) was the most dominant bacterial species in VMG (ESI Tables S2 and S3†).
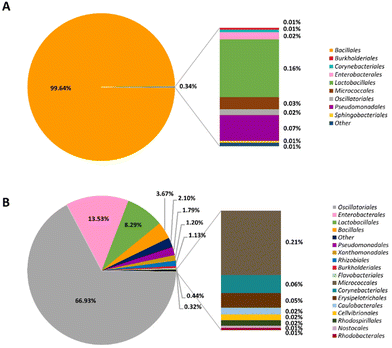 |
| Fig. 1 The relative abundances of microorganisms in BMG and VMG (n = 1 Gochujang). (A) Micro-organisms of BMG at the order level and (B) microorganisms of VMG at the order level. BMG, loperamide + BMG Gochujang (2 g per kg bw) and VMG, loperamide + VMG Gochujang (2 g per kg bw). | |
Table 2 The relative abundances of beneficial and pernicious microorganisms in BMG and VMG at the species level
Type of Gochujang |
Beneficial or pernicious |
Order |
Species |
Percentage (%) |
Each
|
Total
|
The relative abundances of beneficial and pernicious microorganisms of BMG and VMG (n = 1 Gochujang). |
BMG |
Beneficial |
Bacillales
|
Bacillus subtilis
|
92.77 |
92.79 |
Bacillus coagulans
|
0.01 |
Lactobacillales
|
Leuconostoc mesenteroides
|
0.01 |
Pernicious |
Enterobacterales
|
Proteus mirabilis
|
0.01 |
0.06 |
Enterobacter cloacae
|
0.01 |
Pseudomonadales
|
Acinetobacter baumannii
|
0.04 |
VMG |
Beneficial |
Lactobacillales
|
Leuconostoc mesenteroides
|
0.41 |
2.42 |
Weissella confusa
|
0.21 |
Lactococcus lactis
|
0.07 |
Lactobacillus sakei
|
0.07 |
Lactobacillus plantarum
|
0.01 |
Lactobacillus brevis
|
0.01 |
Bacillales
|
Bacillus subtilis
|
1.63 |
Bacillus velezensis
|
0.01 |
Pernicious |
Enterobacterales
|
Enterobacter cloacae
|
12.38 |
12.52 |
Cronobacter sakazakii
|
0.11 |
Proteus mirabilis
|
0.01 |
Pseudomonadales
|
Acinetobacter baumannii
|
0.02 |
Next, α-diversity measurements, including OTUs, ACE, CHAO, Shannon, Simpson, phylogenetic diversity (Pd) and Good's coverage of library indices were analyzed to evaluate the diversity and abundance of microorganisms in BMG and VMG. Overall, the levels of diversity and abundance indicators were higher in VMG compared to BMG (Table 3). Also, in the screening stage for selecting Gochujang, it was confirmed that BMG and VMG have different microbial community structures through the β-diversity and LEfSe analysis (ESI Fig. S2† and Table 4). Through the PCoA graph analyzed during the Gochujang screening, it was visually confirmed that BMG and VMG have different microbial community structures (ESI Fig. S2†). In addition, the LDA score was calculated by comparing the relative taxonomic composition between BMG and VMG, and 30 kinds of microorganisms that caused the difference in microbial communities between them were detected. Bacillus, Bacillaceae, Staphylococcaceae, Staphylococcus, and Staphylococcus saprophyticus were sequentially confirmed to be microorganisms that greatly affect the difference in the microbial communities between BMG and VMG (Table 4).
Table 3 α-Diversity indices for BMG and VMG
|
OTUs |
ACE |
Chao1 |
Shannon |
Simpson |
Phylogenetic diversity |
Good's coverage of library |
α-Diversity indices for the microbiome samples from BMG and VMG (n = 1 Gochujang). OTUs, operational taxonomic units; ACE, abundance-based coverage estimators. |
BMG |
158 |
201.42 |
193.05 |
1.36 |
0.35 |
301 |
99.96 |
VMG |
710 |
830.7 |
801.08 |
3.92 |
0.05 |
1041 |
99.53 |
Table 4 Biomarker discovery of BMG and VMG (n = 1 Gochujang)
Taxon name |
Taxon rank |
LDA effect size |
p-Value |
Relative abundance |
BMG |
VMG |
Bacillus
|
Genus |
5.544 |
0.025 |
82.141 |
14.827 |
Bacillaceae
|
Family |
5.479 |
0.025 |
82.172 |
16.131 |
Staphylococcaceae
|
Family |
5.416 |
0.025 |
0.304 |
50.143 |
Staphylococcus
|
Genus |
5.412 |
0.025 |
0.302 |
49.854 |
Staphylococcus saprophyticus
|
Species |
5.393 |
0.025 |
0.040 |
48.060 |
Bacillus licheniformis
|
Species |
5.364 |
0.025 |
47.476 |
1.161 |
Bacilli
|
Class |
5.138 |
0.025 |
99.786 |
78.064 |
Firmicutes
|
Phylum |
5.113 |
0.025 |
99.791 |
78.201 |
Bacillus subtilis
|
Species |
4.939 |
0.025 |
30.117 |
12.127 |
Proteobacteria
|
Phylum |
4.935 |
0.025 |
0.020 |
16.627 |
Lactobacillus
|
Genus |
4.923 |
0.025 |
17.271 |
0.811 |
Lactobacillus rennini
|
Species |
4.915 |
0.025 |
17.257 |
0.067 |
Lactobacillaceae
|
Family |
4.900 |
0.025 |
17.275 |
1.670 |
Gammaproteobacteria
|
Class |
4.839 |
0.025 |
0.011 |
14.172 |
Bacillales
|
Order |
4.822 |
0.025 |
82.491 |
66.488 |
Enterobacterales
|
Order |
4.751 |
0.025 |
0.004 |
10.683 |
Enterococcaceae
|
Family |
4.470 |
0.025 |
0.015 |
6.052 |
Lactobacillales
|
Order |
4.408 |
0.025 |
17.295 |
11.574 |
Enterobacteriaceae
|
Family |
4.384 |
0.025 |
0.002 |
4.914 |
Tetragenococcus halophilus
|
Species |
4.327 |
0.025 |
0.002 |
4.208 |
Tetragenococcus
|
Genus |
4.297 |
0.025 |
0.002 |
4.208 |
Leuconostocaceae
|
Family |
4.263 |
0.025 |
0.004 |
3.521 |
Bacillus thermoamylovorans
|
Species |
4.222 |
0.025 |
3.630 |
0.286 |
Actinobacteria
|
Phylum |
4.165 |
0.025 |
0.181 |
3.018 |
Actinobacteria_c
|
Class |
4.158 |
0.025 |
0.179 |
3.009 |
Erwiniaceae
|
Family |
4.105 |
0.025 |
0.002 |
2.557 |
Enterobacteriaceae
|
Species |
4.094 |
0.025 |
0.000 |
2.424 |
Enterobacteriaceae_g
|
Genus |
4.091 |
0.025 |
0.000 |
2.424 |
Pantoea
|
Genus |
4.037 |
0.025 |
0.002 |
2.112 |
In summary, BMG is a Gochujang that is dominant with more beneficial bacteria and has less diversity than VMG, and VMG is a Gochujang that has more pernicious bacteria than beneficial bacteria but has a greater variety of species than BMG.
In the nutritional aspects, VMG had higher total carbohydrate and sugars than BMG, resulting in higher calories than BMG. The sodium concentration of VMG was higher than that of BMG (ESI Table S4†)
3.2. Effects of BMG and VMG on body weight, food intake, water consumption, urine output, and fecal excretion parameters
There were no statistically marked differences in the changes in body weight, food intake, water consumption, and urine excretion among all groups (Table 5), suggesting that none of the treatments have been toxic.
Table 5 Measurement of body weight, feed intake, and urine output in experimental mice
Contents |
ND |
Loperamide (5 mg per kg BW) |
LOP
|
MOSA
|
BMG
|
VMG
|
Initial body weight (g) |
32.54 ± 2.02 |
32.98 ± 2.08 |
33.38 ± 2.12 |
33.19 ± 1.52 |
33.14 ± 1.62 |
Final body weight (g) |
34.51 ± 1.01 |
34.00 ± 2.08 |
35.10 ± 3.24 |
33.91 ± 2.95 |
34.43 ± 0.91 |
Feeding behavior |
Food intake (g day−1) |
4.25 ± 0.45 |
3.99 ± 0.21 |
3.87 ± 0.18 |
4.51 ± 1.20 |
4.6 ± 0.28 |
Water consumption (mL day−1) |
7.41 ± 2.65 |
5.03 ± 1.43 |
8.17 ± 9.29 |
5.61 ± 1.57 |
5.57 ± 1.7 |
Urine volume (mL day−1) |
0.9 ± 0.2 |
1.08 ± 0.28 |
1.12 ± 0.1 |
0.9 ± 0.18 |
1.34 ± 0.33 |
Next, the fecal parameters, including fecal weight, feces number and fecal water content were assessed to determine the fecal excretion stimulatory effects of BMG and VMG on LOP-induced constipation. As shown in Fig. 2A, the weight of fecal pellets was significantly reduced in the LOP group compared to that in the ND group. Compared to the LOP group, the fecal weights of the BMG and VMG groups were significantly increased by 107.9% and 106.98%, respectively. Also, the number of fecal pellets in the LOP group was markedly reduced compared to that in the ND group. Compared with the LOP group, the number of fecal pellets was significantly increased in the BMG (123.37%) and VMG (115.4%) groups (Fig. 2B). Additionally, the water contents of feces were measured on days 1 (baseline) and 10. In all LOP-treated groups, the fecal water contents were reduced compared to the baseline, and the LOP group had the largest reduction, but the BMG and VMG groups showed a significant restoration of the fecal water content (Fig. 2C). Compared with the ND group, the fecal water content of the LOP group on day 10 was significantly decreased by 87.38% (Fig. 2D). Moreover, there were significant upregulations of the water contents in the BMG (117.28%) and VMG (108.91%) groups compared to the LOP group.
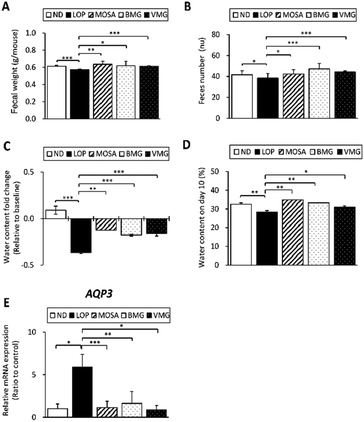 |
| Fig. 2 Effects of BMG and VMG on fecal excretion parameters in ICR mice with LOP-induced constipation. (A) Fecal weight, (B) fecal number, (C) fecal water content change compared to the baseline, (D) fecal water content on day 10, and (E) AQP3 mRNA expression in the colon. These parameters were measured at the same time during the experimental period. Seven mice per group were investigated in triplicate for fecal parameter analysis. ND, non-constipation group, LOP, loperamide (5 mg per kg BW) + vehicle-treated group, MOSA, loperamide (5 mg per kg BW) + mosapride citrate (3 mg per kg BW) group, BMG, loperamide (5 mg per kg BW) + BMG Gochujang (2 g per kg BW) group, and VMG, loperamide (5 mg per kg BW) + VMG Gochujang (2 g per kg BW) group. Data are expressed as the mean ± SD (n = 7 mice per group). *, p < 0.05, **, p < 0.01 and ***, p < 0.001 show significant differences between different groups. Abbreviation: ICR, Institute of Cancer Research; LOP, loperamide; AQP3, aquaporin 3. | |
AQP3, an important regulator of water transfer in the colon, is inversely associated with water reabsorption.24 The AQP3 mRNA expression was analyzed to identify whether the changes in the fecal water content are related to the AQP3 level. Consistent with the above observations, the mRNA expression of AQP3 was markedly increased in the LOP group compared to that in the ND group (Fig. 2E). In addition, compared to the LOP group, the BMG and VMG groups had a significant reduction in AQP3 mRNA expression. These findings suggest that administration of BMG and VMG could mitigate LOP-induced constipation by improving the fecal water content and AQP3 mRNA expression.
3.3 Effects of BMG and VMG on the GI motility and intestinal length
To investigate whether the defecation stimulatory effects of BMG and VMG result from the changes in GI motility and intestinal length, we tested the GI motility through the charcoal meal transit experiment and measured the length of the intestine (Fig. 3A). Compared with the ND group, the propulsion of the charcoal meal was significantly decreased by 7.46% in the LOP group (Fig. 3B). However, these alterations were markedly reverted by the administration of BMG (23.51%) and VMG (16%) similar to the MOSA group. Additionally, the LOP group had a significantly shorter length of the intestine compared to the ND group, while the BMG and VMG groups had a significantly longer intestine length than the LOP group (Fig. 3C). These observations suggest that BMG and VMG exert defecation stimulatory effects by elevating intestinal motility.
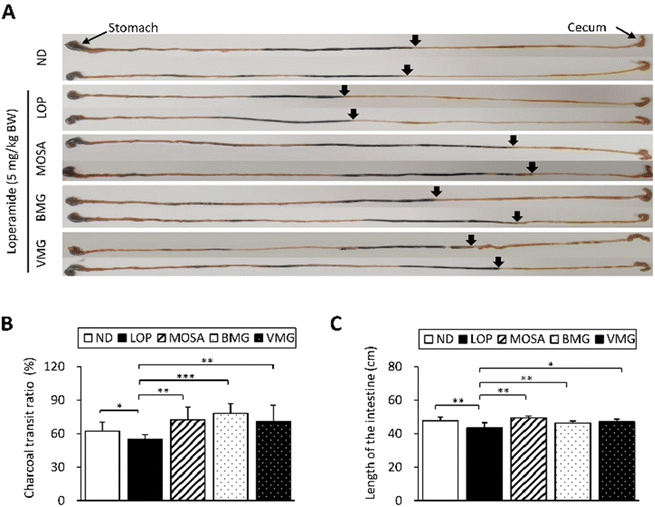 |
| Fig. 3 Effects of BMG and VMG on the GI transit ratio in ICR mice with LOP-induced constipation. (A) Representative actual image showing the charcoal meal transit in the intestine. The total intestinal tract was excised from mice of each subset group treated with 5% charcoal meal powder. After 30 min of administration with charcoal, the length of charcoal from the stomach to the intestine was measured. The arrows indicate the portion of the charcoal meal. (B) Transit ratio of the charcoal meal was calculated using the total length of the intestine and the distance of the charcoal meal. (C) Length of the small intestine. ND, non-constipation group, LOP, loperamide (5 mg per kg BW) + vehicle-treated group, MOSA, loperamide (5 mg per kg BW) + mosapride citrate (3 mg per kg BW) group, BMG, loperamide (5 mg per kg BW) + BMG Gochujang (2 g per kg BW) group, and VMG, loperamide (5 mg per kg BW) + VMG Gochujang (2 g per kg BW) group. Data are expressed as the mean ± SD (n = 7 mice per group). *, p < 0.05, **, p < 0.01 and ***, p < 0.001 show significant differences between different groups. Abbreviations: GI, gastrointestinal; ICR, Institute of Cancer Research; LOP, loperamide. | |
3.4. Effects of BMG and VMG on the GI hormones and ENS-related factors
The secretion of GI hormones and neurotransmitters regulates the enteric nervous system (ENS), modulating the gut motility.25 The levels of MTL and GAS are negatively associated with the severity of constipation.26 As BMG and VMG administration enhances the gut motility, GI hormones and ENS-associated parameters were analyzed. The concentrations of GI hormones were strongly decreased in the LOP group compared with those in the ND group (MTL, 87.34%, GAS, 48.50%, and AChE, 75.36%) (Fig. 4A–C). Compared to the LOP group, the BMG group had significantly increased levels of MTL (115.06%), GAS (170.05%), and AChE (130.54%); furthermore, the VMG group showed elevated levels of these hormones (MTL, 111.18%, GAS, 141.39%, and AChE, 120.67%) (Fig. 4A–C).
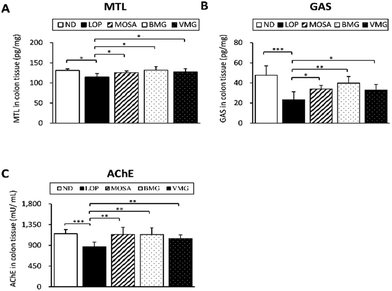 |
| Fig. 4 Effects of BMG and VMG on GI hormones in ICR mice with LOP-induced constipation. The concentrations of (A) MTL, (B) GAS, and (C) AChE were measured in the colon homogenate by ELISA. Data are expressed as the mean ± SD (n = 7 mice per group). *, p < 0.05, **, p < 0.01 and ***, p < 0.001 show significant differences between different groups. Abbreviations: GI, gastrointestinal; ICR, Institute of Cancer Research; LOP, loperamide; AChE, acetylcholinesterase; MTL, motilin; GAS, gastrin. ND, non-constipation group, LOP, loperamide (5 mg per kg BW) + vehicle-treated group, MOSA, loperamide (5 mg per kg BW) + mosapride citrate (3 mg per kg BW) group, BMG, loperamide (5 mg per kg BW) + BMG Gochujang (2 g per kg BW) group, and VMG, loperamide (5 mg per kg BW) + VMG Gochujang (2 g per kg BW) group. | |
Neurotransmission is controlled through the interaction of excitatory and inhibitory neurotransmitters.25 There are pivotal ENS-associated proteins playing roles in gut motility, including brain-derived neurotrophic factor (BDNF), glial cell line-derived neurotrophic factor (GDNF), nitric oxide synthase (NOS), and transient receptor potential cation channel subfamily V member 1 (TRPV1).27 Compared to the ND group, the mRNA expressions of BDNF and GDNF were markedly decreased in the LOP group, whereas those in BMG and VMG groups showed an increased tendency compared to the LOP group with and/or without statistical significances (Fig. 5A and B). Moreover, the mRNA expressions of NOS and TRPV1 were increased by LOP administration, while both BMG and VMG groups showed significantly decreased mRNA expressions of NOS and TRPV1 (Fig. 5C and D).
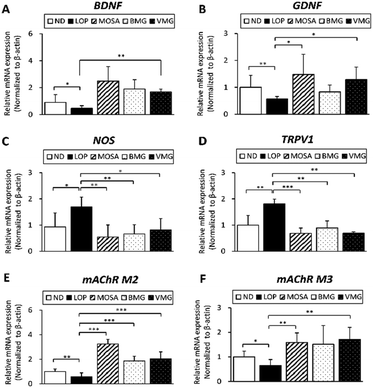 |
| Fig. 5 Effects of BMG and VMG on the mRNA expression levels of enteric nerve-related factor in the colon tissue of ICR mice with LOP-induced constipation. (A) BDNF, (B) GDNF, (C) NOS, (D) TRPV1. (E) mAChR M2, and (F) mAChR M3. ND, non-constipation group, LOP, loperamide (5 mg per kg BW) + vehicle-treated group, MOSA, loperamide (5 mg per kg BW) + mosapride citrate (3 mg per kg BW) group, BMG, loperamide (5 mg per kg BW) + BMG Gochujang (2 g per kg BW) group, and VMG, loperamide (5 mg per kg BW) + VMG Gochujang (2 g per kg BW) group. Data are expressed as the mean ± SD (n = 7 mice per group). *, p < 0.05, **, p < 0.01 and ***, p < 0.001 show significant differences between different groups. Abbreviations: ICR, Institute of Cancer Research; LOP, loperamide; BDNF, brain-derived neurotrophic factor; GDNF, glial cell line-derived neurotrophic factor; NOS, nitric oxide synthase; TRPV1, transient receptor potential cation channel subfamily V member 1. mAChR M2, muscarinic acetylcholine receptors M2; mAChR M3, muscarinic acetylcholine receptors M3. | |
The muscarinic acetylcholine (Ach) receptors (mAChRs), including M2 and M3, also play a major role in GI motility by mediating contractions.28 The mAChR M2 maintains rhythmic motor activities and the mAChR M3 plays a modulatory role in the GI tract.29 LOP treatment significantly reduced the mRNA levels of mAChR M2 and M3, while the administration of BMG and VMG elevated the mRNA expressions of these receptors with and/or without a statistical significance (Fig. 5E and F). In conclusion, these results demonstrate that BMG and VMG have anti-constipation effects by improving the release of GI hormones and abnormalities of the ENS.
3.5. Effects of BMG and VMG on the signaling pathways related to GI motility
Interstitial cells of Cajal (ICCs) control GI motility as pacemaker cells by mediating intestinal electrical coupling between smooth muscles and nerve terminals,30 and their development, maturation, maintenance, and function are modulated by c-Kit/SCF signaling pathways.31
Compared to the ND group, the mRNA level of c-Kit showed a decreased tendency in the LOP group, whereas the administration of BMG and VMG significantly upregulated the c-Kit mRNA level compared to the LOP group (Fig. 6A). Additionally, the LOP group showed a significant reduction in SCF mRNA expression relative to the ND group, while both BMG and VMG groups showed an increased tendency of SCF mRNA levels with and/or without a statistical significance compared to the LOP group (Fig. 6B). Consistent with the mRNA expression, the protein levels of c-Kit and SCF were also strongly increased by BMG and VMG administration compared to the LOP group (Fig. 6C).
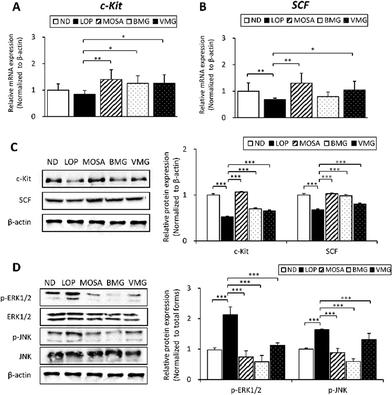 |
| Fig. 6 Effects of BMG and VMG on the signalling pathways related to gut motility in the colon tissue of ICR mice with LOP-induced constipation. (A) The mRNA expression level of c-Kit, (B) mRNA expression level of SCF, (C) western blot of c-Kit and SCF, and the quantification bar graph (D) western blot of MAPK pathways and the quantification bar graph. ND, non-constipation group, LOP, loperamide (5 mg per kg BW) + vehicle-treated group, MOSA, loperamide (5 mg per kg BW) + mosapride citrate (3 mg per kg BW) group, BMG, loperamide (5 mg per kg BW) + BMG Gochujang (2 g per kg BW) group, and VMG, loperamide (5 mg per kg BW) + VMG Gochujang (2 g per kg BW) group. Data are expressed as the mean ± SD (n = 7 mice per group). *, p < 0.05, **, p < 0.01 and ***, p < 0.001 show significant differences between different groups. Abbreviations: ICR, Institute of Cancer Research; LOP, loperamide; SCF, stem cell factor. MAPK, mitogen-activated protein kinase; p-ERK1/2, phospho-extracellular signal-regulated protein kinase; ERK1/2, extracellular signal-regulated protein kinase; p-JNK, phospho-c-Jun N-terminal kinase; JNK, c-Jun N-terminal kinase. | |
The MAPK pathway is another critical signaling pathway for GI motility in normal intestinal smooth muscle.32 Compared to the ND group, phosphorylation of ERK and JNK was markedly higher in the LOP group, while BMG and VMG groups had significantly reduced levels of these proteins compared to the LOP group (Fig. 6D). Although phosphorylation of p38 did not differ between the ND and LOP groups, the BMG and VMG groups showed a significant reduction in p38 phosphorylation compared to the LOP group (ESI Fig. S3†). Taken together, these observations indicate that BMG and VMG alter the activation of c-Kit/SCF and MAPK signaling pathways, leading to improvements in GI motility in LOP-induced constipation.
3.6. Effects of BMG and VMG on the histopathological structures of the transverse colon
In addition to the beneficial effects of BMG and VMG on GI motility via the secretions of GI hormones and neurotransmitters, and the signaling pathways, the influences of both types of Gochujangs on the colon structure were also investigated (Fig. 7A). Compared with the ND group, the LOP group displayed a significant reduction in the muscle thickness, mucosa layer thickness, and flat luminal surface thickness (Fig. 7B–D). However, these parameters of the BMG and VMG groups were dramatically increased compared with those of the LOP group. Taken together, these observations imply that BMG and VMG administration ameliorates histopathological damage to the colon tissue in LOP-induced constipation.
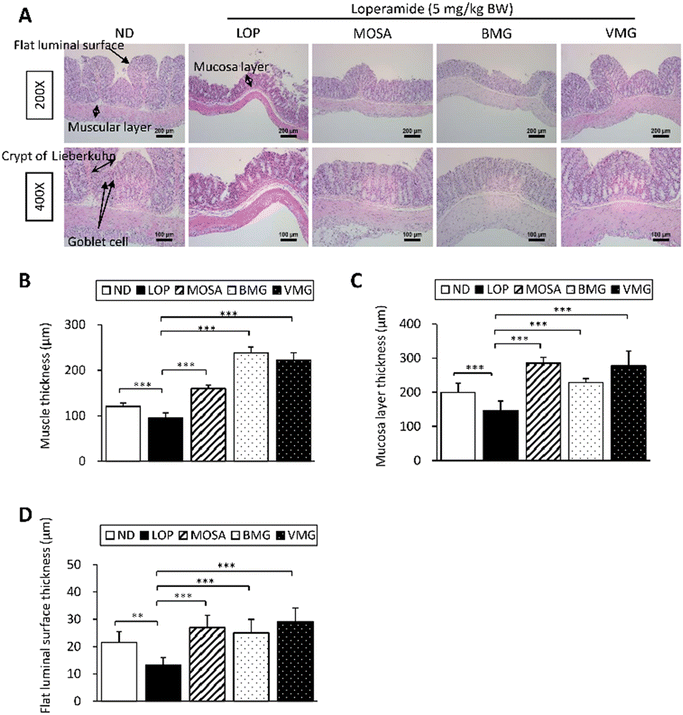 |
| Fig. 7 Histopathological structures of the transverse colon in ICR mice with LOP-induced constipation. (A) The transverse colon by H&E staining was observed at 200× (up column) and 400× (down column) magnification using a light microscope. (B) Muscular layer thickness, (C) mucosa layer thickness, and (D) flat luminal surface thickness. Histopathological parameters were measured using the Leica Application Suite. Data are expressed as the mean ± SD (n = 7 mice per group). *, p < 0.05, **, p < 0.01 and ***, p < 0.001 show significant differences between different groups. Abbreviations: ICR, Institute of Cancer Research; LOP, loperamide; H&E, hematoxylin and eosin. | |
3.7. Effects of BMG and VMG on gut microbial diversity and composition
The 16S rRNA gene sequence metagenomic analysis was used to evaluate the alteration of the intestinal microbiome profile among groups. The VMG group was different from the LOP group and similar to the MOSA group, suggesting that BMG can change the composition of the intestinal microbiota (Fig. 8A). Moreover, the VMG group had significantly lower ACE, CHAO, and Pd indices compared to the LOP group, whereas the other parameters did not have any significant differences among the groups (Fig. 8B), indicating that VMG affects the distribution and diversity of the gut microbiota while BMG does not.
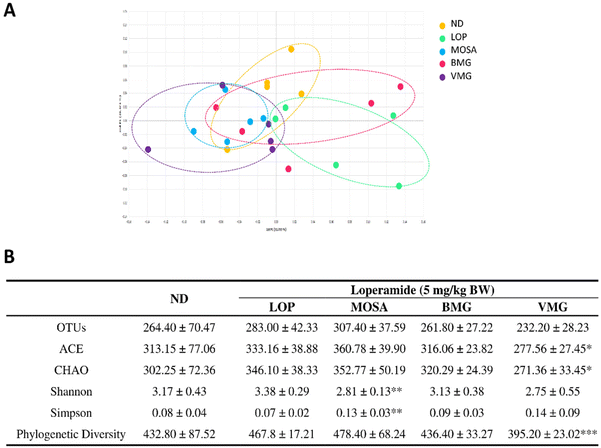 |
| Fig. 8 Effects of BMG and VMG on the richness and diversity indices of the fecal microbiota of ICR mice with LOP-induced constipation. (A) A principal co-ordinate analysis of the gut microbiomes after the induction of constipation. (B) α-Diversity indices after the induction of constipation, ND, non-constipation group, LOP, loperamide (5 mg per kg BW) + vehicle-treated group, MOSA, loperamide (5 mg per kg BW) + mosapride citrate (3 mg per kg BW) group, BMG, loperamide (5 mg per kg BW) + BMG Gochujang (2 g per kg BW) group, and VMG, loperamide (5 mg per kg BW) + VMG Gochujang (2 g per kg BW) group. Data are expressed as the mean ± SD (n = 5 mice per group). *, p < 0.05, **, p < 0.01 and ***, p < 0.001 show significant differences between different groups. Abbreviations: ICR, Institute of Cancer Research; LOP, loperamide; OTUs, operational taxonomic units; ACE, abundance-based coverage estimators. | |
At the phylum level, the fecal microbiota of all groups was dominated by Bacteroidetes, Firmicutes, Verrucomicrobia, and Proteobacteria (Fig. 9A). Compared to the ND group, the LOP group showed downregulated levels of Bacteroidetes and Verrucomicrobia and upregulated levels of Firmicutes and Proteobacteria (Fig. 9A and B). Compared to the LOP group, both BMG and VMG groups strongly reversed these trends of the LOP group. Therefore, the ratio of Firmicutes/Bacteroidetes of the LOP group was significantly higher compared with that of the ND group, while the BMG and VMG groups had a markedly lower ratio of Firmicutes/Bacteroidetes than the LOP group (Fig. 9C). At the genus level, compared with the ND group, the LOP group showed reduced levels of Akkermansia, Parabacteroides, and Alistipes and elevated levels of Pseudoflavonifractor and Oscillibacter (Fig. 10A and B). Both the BMG and VMG groups restored these tendencies in the LOP group. In summary, BMG and VMG mitigate LOP-induced constipation via alterations in gut microbiota diversity and compositions.
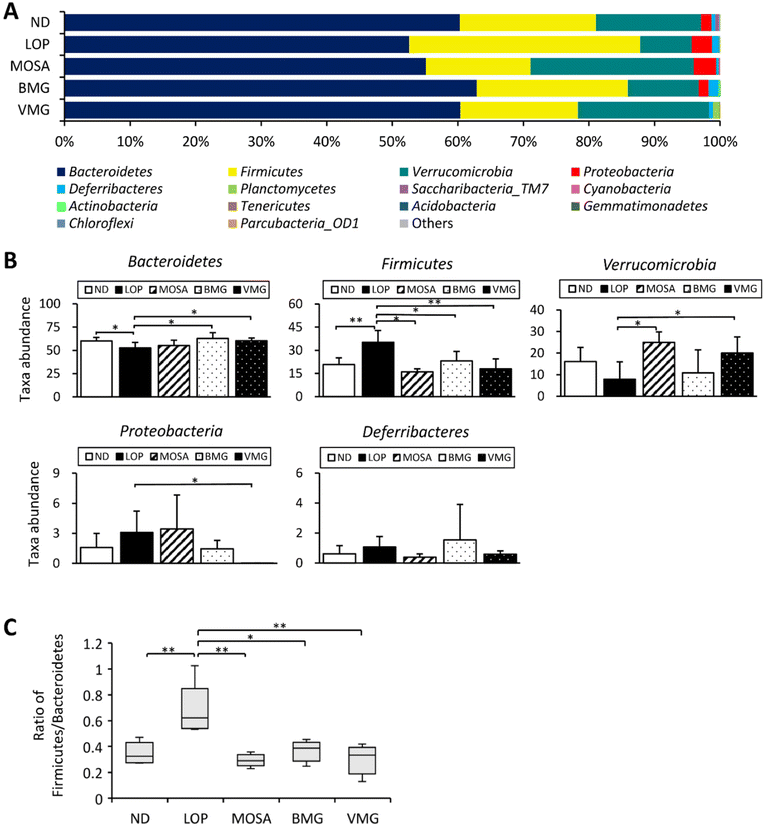 |
| Fig. 9 Effects of BMG and VMG on the gut microbiota at the phylum level of ICR mice with LOP-induced constipation. (A) Phylum level graph, (B) top 5 phyla with significant differences at the genus level, and (C) ratio of Firmicutes and Bacteroidetes. ND, non-constipation group, LOP, loperamide (5 mg per kg BW) + vehicle-treated group, MOSA, loperamide (5 mg per kg BW) + mosapride citrate (3 mg per kg BW) group, BMG, loperamide (5 mg per kg BW) + BMG Gochujang (2 g per kg BW) group, and VMG, loperamide (5 mg per kg BW) + VMG Gochujang (2 g per kg BW) group. Data are expressed as the mean ± SD (n = 5 mice per group). *, p < 0.05, **, p < 0.01 and ***, p < 0.001 show significant differences between different groups. Abbreviations: ICR, Institute of Cancer Research; LOP, loperamide. | |
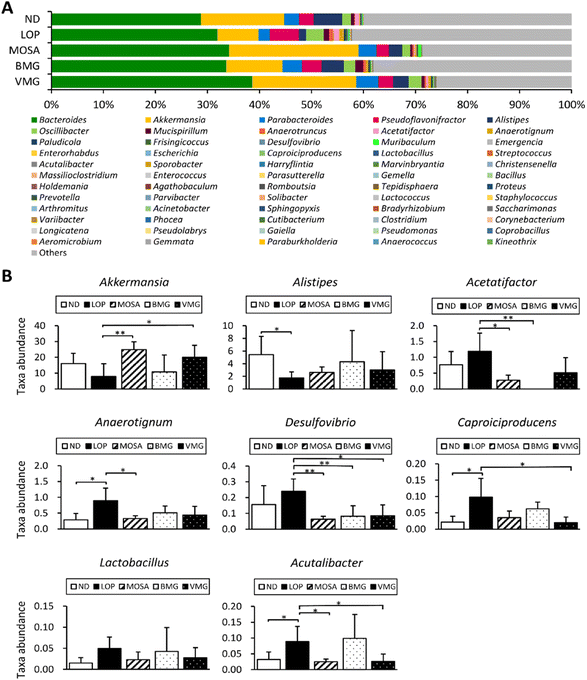 |
| Fig. 10 Effects of BMG and VMG on the gut microbiota at the genus level of ICR mice with LOP-induced constipation. (A) Genus level graph and (B) genera with significant differences at the genus level. ND, non-constipation group, LOP, loperamide (5 mg per kg BW) + vehicle-treated group, MOSA, loperamide (5 mg per kg BW) + mosapride citrate (3 mg per kg BW) group, BMG, loperamide (5 mg per kg BW) + BMG Gochujang (2 g per kg BW) group, and VMG, loperamide (5 mg per kg BW) + VMG Gochujang (2 g per kg BW) group. Data are expressed as the mean ± SD (n = 5 mice per group). *, p < 0.05, **, p < 0.01 and ***, p < 0.001 show significant differences between different groups. Abbreviations: ICR, Institute of Cancer Research; LOP, loperamide. | |
3.8. Correlation between physicochemical indices and the gut microbiota
To understand the correlations between the physicochemical indices of constipation and 42 genera of the gut microbiota, Spearman's correlation analysis was performed (Fig. 11). The GI transit ratio was negatively correlated with Oscillibacter, Marvinbryantia, and Enterococcus. AQP3 was positively correlated with Bacteroides and Paludicola, but inversely correlated with Parabacteroides and Enterococcus. GAS and mAChR M3 were positively and negatively correlated with Pseudoflavonifractor, Acetatifactor, Desulfovibrio, and Enterohabdus, respectively. Additionally, GAS and Lactococcus showed a negative correlation. In addition, Lactococcus has a positive correlation with TRPV1 and c-Kit. Acutalibacter, Harryflintia, and Massilioclostridium were inversely correlated with TRPV1, while Oscillibacter, Desulfovibrio, and Muribaculum had a negative correlation with c-Kit. Lastly, mAChR M3 was positively correlated with Akkermansia and Parabacteroides. These observations indicate that those changes in the gut microbiota composition are associated with constipation and that they are ameliorated by BMG and VMG consumption.
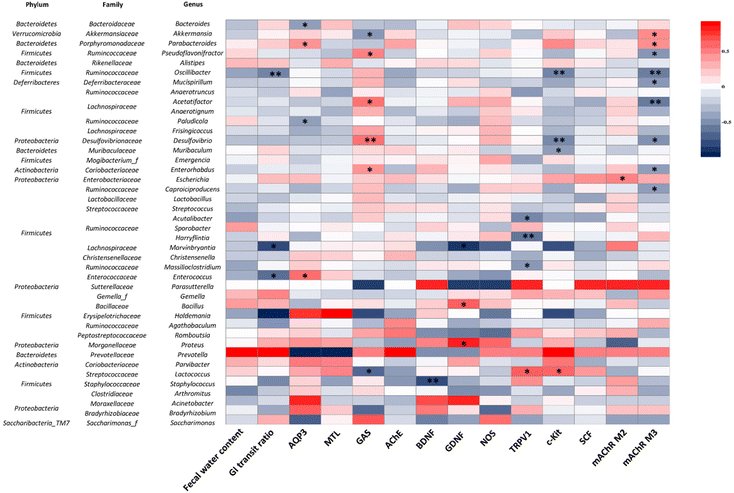 |
| Fig. 11 Spearman correlations between the communities of the gut microbiota and the physicochemical indices at the genus level (n = 5 mice per group). Abbreviations: GI, gastrointestinal; AQP3, aquaporin 3; MTL, motilin; GAS, gastrin; AChE, acetylcholinesterase; BDNF, brain-derived neurotrophic factor; GDNF, glial cell line-derived neurotrophic factor; NOS, nitric oxide synthase; TRPV1, transient receptor potential cation channel subfamily V member 1; SCF, stem cell factor; mAChR, muscarinic acetylcholine receptor. Significant differences of correlations are presented by *, p < 0.05, **, p < 0.01 and ***, p < 0.001. | |
4. Discussion
The pharmacological treatments of constipation are less applied due to the possibility of adverse effects, such as myocardial infarction.3,4 Thus, food and/or diet interventions (e.g., traditional foods and herbal medications) have been recommended to prevent and/or treat constipation.33Gochujang is a representative fermented hot pepper paste of Korea containing multiple bacterial species.14 The composition of its microbial communities is highly dependent on environmental factors (e.g., weather and province) and the physicochemical properties of raw materials (e.g., meju).34,35 However, the distinct physiological effects of Gochujang based on its different microbial composition are less explored. Here, we found that two different types of Gochujangs, BMG and VMG, ameliorate LOP-induced constipation by improving water reabsorption, GI motility, GI hormone and neurotransmitter release, aberration of the ENS and the related signaling pathways, and by changing the gut microbiota composition.
The interruptions of water reabsorption and water contents in stool reduce GI motility, leading to the development of constipation consequently, because water has a laxative effect.36 AQP3 is highly expressed at the apical membrane of colon mucosal epithelial cells,24 and it has been highlighted as a new therapeutic target for constipation due to its roles in water transport in the colon.37 Both BMG and VMG increased fecal weight, the frequency of defecation, water contents in stools, and decreased AQP3 mRNA expression in the colon in LOP-induced constipation mice. A previous study demonstrated that B. subtilis, isolated from Korean fermented soybean foods (doenjang and kimchi), has a high adaptability to the GI environment and prevents constipation by modulating the GI transit ratio.38 BMG has a higher level of B. subtilis than VMG, suggesting that the above defecation stimulatory effects of BMG might be associated with its high level of B. subtilis, but VMG might have different mechanisms such as the alterations of metabolite production (e.g., short chain fatty acid and lactate) and the intestinal pH.39,40 Therefore, future studies are essentially required to investigate the production of different metabolites based on the microbial composition of Gochujang to connect the detailed functions of Gochujang's microbial communities and its health beneficial outcomes.
A balance of contraction and relaxation of the intestinal muscle layer by excitatory and inhibitory stimulations is critical to maintaining normal GI motility.41 GI movements are regulated by GI hormones, neurotransmitters, and the ENS. The administration of BMG and VMG significantly enhanced the release of GI hormones (MTL, GAS, and AChE) and the mRNA expressions of excitatory neurotransmitters (BDNF and GDNF) in LOP-induced constipation mice. Moreover, the mRNA levels of inhibitory neurotransmitters, NOS and TRPV1 were suppressed by BMG and VMG consumption. Additionally, BMG and VMG significantly elevated the mRNA expressions of mAChR M2 and M3 acting on water absorption through the regulation of Ach action. The administration of probiotics such as Lactobacillus acidophilus LA11-Onlly restored MTL and GAS levels in LOP-induced constipation mice.42 Additionally, the oral administration of Lactobacillus plantarum YS2 reversed not only TRPV1 and NOS protein levels, but also serum Ach levels in activated carbon-induced constipation mice.43 In the future, the exact involvement of each Gochujang's microbiota in the regulation of GI hormone and neurotransmitter release should be established.
c-Kit/SCF and MAPK signaling pathways play significant roles in intestinal GI movements. The c-Kit/SCF pathway controls the maturation and development of ICCs, pacemaker cells of intestinal motility.30,31 According to Shahi et al., the c-Kit/SCF pathway promotes the expression of the 5-hydroxytryptamine 4 receptor (5-HT4) of ICCs by elevating the sensitivity of ICCs to GI hormones.44,45 BMG and VMG significantly elevated c-Kit/SCF signaling-associated mRNA and protein levels in LOP-induced constipation mice, suggesting the possibility that BMG and VMG could directly play roles in the development and maturation of ICCs. The MAPK pathway manages intestinal smooth muscle contractions.32 An earlier study found that Cheonggukjang, another traditional Korean soybean fermented food, elicits anti-constipation effects by downregulating MAPK signaling pathways in LOP-induced constipation mice.46 Consistently, the present study found that BMG and VMG suppressed MAPK signaling pathways in LOP-induced constipation mice. Isoflavones, such as daidzein, have anti-inflammation effects through MAPK signaling pathway modulation,47 and the main ingredient in Gochujang is soybean. Therefore, the subsequent studies should explore the possible and/or precise compounds, including isoflavones in Gochujang to better understand their functions in ICC maintenance and GI motility-associated signaling pathways.
The gut microbiota is pivotal to maintaining normal colon health and GI movement; therefore, dysbiosis of intestinal flora causes chronic GI diseases, including constipation.48 For example, constipation patients have a reduced level of Alistipes and an increased Firmicutes to Bacteroidetes ratio and Desulfovibrio level.49–51 As fermented foods contain large and diverse microbiomes, consumption of fermented foods (e.g., kimchi, yogurt, and cheese) could alter the gut microbiota composition and alleviate GI diseases, such as constipation.52 BMG and VMG markedly upregulated the levels of Bacteroidetes, Verrucomicrobia, Akkermansia, Parabacteroides, and Alistipes, but significantly lowered the levels of Firmicutes, Proteobacteria, Pseudoflavonifractor and Oscillibacter in LOP-induced constipation mice. Moreover, the previous changes in physicochemical parameters in this study had a significant correlation with those gut microbiota composition alterations (Fig. 11). Intriguingly, even though BMG and VMG showed similar outcomes in the alterations of the gut microbiota and other previous physicochemical indicators, only the VMG group had a lower α-diversity (ACE and CHAO) and phylogenetic diversity than the LOP group, implying that the microbial diversity in the intestinal microbiota does not directly reflect the characteristics of food's microbial communities.
The present study demonstrated that the consumption of Gochujang elicits anti-constipation outcomes regardless of its different microbial communities by improving GI motility and altering the gut microbiota composition. Shortly, Gochujang enhances GI motility via (1) the elevations of water contents and reabsorption, (2) the enhancements of GI hormone secretion, neurotransmitter release, and ENS abnormalities, and (3) the alterations of GI motility associated signaling pathways. The limitations of the current study are that (1) only two kinds of Gochujangs are used for this study and (2) the experimental period was only two weeks. As described above, the gut microbiota of Gochujang differs based on diverse factors, including weather, nutrition etc. Although the two types of Gochujangs had different effects on the gut microbiota of mice due to the difference in the microbial composition, they may also be due to nutritional differences, which are minor elements. Therefore, upcoming research is needed to utilize more of the various Gochujang types in long-term experimental design and human feeding studies. Nevertheless, the current study is still meaningful as it investigated the constipation mitigative effect of Gochujang and its detailed underlying molecular mechanisms in addition to the gut microbial composition changes.
5. Conclusions
In conclusion, it was discovered that Gochujang can relieve LOP-induced constipation in ICR mice, despite the different microbiota. BMG and VMG improve fecal excretion by increasing feces weight, water content and GI transition through GI hormones and various neurotransmitters. It was found that the constipation relief effect of Gochujang leads through the c-Kit/SCF, MAPK, and mAChR signaling pathways. The mice's gut microbiota was dominated by Bacteroidetes, Firmicutes, and Verrucomicrobiota. BMG and VMG increase in Bacteroidetes, decrease in Firmicutes (at the phylum level), and decrease in Desulfovibrio (at the genus level). However, significantly reduced Acetatifactor was shown only in BMG, but Akkermansia, Caproiciproducens, and Acutalibacter showed significant differences in the VMG group.
Author contributions
NYK performed the experiments and wrote the manuscript draft. KAK conducted part of the experiments. HJY and SJJ experimented and analysed the NGS data. All authors analysed the data. AH corrected and revised the article. AH and YSC were the principal investigators.
Conflicts of interest
There are no conflicts to declare.
Acknowledgements
This work was supported by the “Functional identification of Korean traditional soybean products (safety monitoring) project” under the Ministry of Agriculture, Food and Rural Affairs and partly by Korea Agro-Fisheries and Food trade corporation in 2022.
References
- L. R. Schiller, Chronic constipation: new insights, better outcomes?, Lancet Gastroenterol Hepatol., 2019, 4, 873–882 CrossRef PubMed.
- Q. Zhao, Y.-Y. Chen, D.-Q. Xu, S.-J. Yue, R.-J. Fu, J. Yang, L.-M. Xing and Y.-P. Tang, Action Mode of Gut Motility, Fluid and Electrolyte Transport in Chronic Constipation, Front. Pharmacol., 2021, 1763 Search PubMed.
- A. E. Bharucha, J. H. Pemberton and G. R. Locke, American Gastroenterological Association technical review on constipation, Gastroenterology, 2013, 144, 218–238 CrossRef.
- A. Lembo and M. Camilleri, Chronic constipation, N. Engl. J. Med., 2003, 349, 1360–1368 CrossRef CAS.
- J. E. Kim, J. W. Park, M. J. Kang, H. J. Choi, S. J. Bae, Y. S. Choi, Y. J. Lee, H. S. Lee, J. T. Hong and D. Y. Hwang, Anti-inflammatory response and muscarinic cholinergic regulation during the laxative effect of Asparagus cochinchinensis in loperamide-induced constipation of SD rats, Int. J. Mol. Sci., 2019, 20, 946 CrossRef CAS PubMed.
- D. Vandeputte, G. Falony, S. Vieira-Silva, R. Y. Tito, M. Joossens and J. Raes, Stool consistency is strongly associated with gut microbiota richness and composition, enterotypes and bacterial growth rates, Gut, 2016, 65, 57–62 CrossRef CAS PubMed.
- E. M. Quigley and R. C. Spiller, Constipation and the microbiome: lumen versus mucosa!, Gastroenterology, 2016, 150, 300–303 CrossRef PubMed.
- I. Khalif, E. Quigley, E. Konovitch and I. Maximova, Alterations in the colonic flora and intestinal permeability and evidence of immune activation in chronic constipation, Dig. Liver Dis., 2005, 37, 838–849 CrossRef CAS.
- G. Barbara, V. Stanghellini, G. Brandi, C. Cremon, G. Di Nardo, R. De Giorgio and R. Corinaldesi, Interactions between commensal bacteria and gut sensorimotor function in health and disease, Am. J. Gastroenterol., 2005, 100, 2560–2568 CrossRef CAS.
- E. Husebye, P. M. Hellström, F. Sundler, J. Chen and T. Midtvedt, Influence of microbial species on small intestinal myoelectric activity and transit in germ-free rats, Am. J. Physiol.: Gastrointest. Liver Physiol., 2001, 280, G368–G380 CrossRef CAS.
- A. Uribe, M. Alam, O. Johansson, T. Midtvedt and E. Theodorsson, Microflora modulates endocrine cells in the gastrointestinal mucosa of the rat, Gastroenterology, 1994, 107, 1259–1269 CrossRef CAS.
- E. M. Quigley, Microflora modulation of motility, J. Neurogastroenterol. Motil., 2011, 17, 140 CrossRef.
- D. Shin and D. Jeong, Korean traditional fermented soybean products: Jang, J. Ethnic Foods, 2015, 2, 2–7 CrossRef.
- S.-J. Jang, Y.-J. Kim, J.-M. Park and Y.-S. Park, Analysis of microflora in gochujang, Korean traditional fermented food, Food Sci. Biotechnol., 2011, 20, 1435–1440 CrossRef.
- S. Ramalingam, A. Bahuguna, S. Lim, A.-R. Joe, J.-S. Lee, S.-Y. Kim and M. Kim, Physicochemical, Microbial, and Volatile Compound Characteristics of Gochujang, Fermented Red Pepper Paste, Produced by Traditional Cottage Industries, Foods, 2022, 11, 375 CrossRef CAS PubMed.
- D.-Y. Jeong, M. S. Ryu, H.-J. Yang and S. Park, γ-PGA-Rich Chungkookjang, Short-term fermented soybeans: Prevents memory impairment by modulating brain insulin sensitivity, neuro-inflammation, and the gut–microbiome–brain axis, Foods, 2021, 10, 221 CrossRef CAS PubMed.
- D. Y. Jeong, J. W. Daily, G. H. Lee, M. S. Ryu, H.-J. Yang, S.-Y. Jeong, J. Y. Qiu, T. Zhang and S. Park, Short-term fermented soybeans with Bacillus amyloliquefaciens potentiated insulin secretion capacity and improved gut microbiome diversity and intestinal integrity to alleviate Asian type 2 diabetic symptoms, J. Agric. Food Chem., 2020, 68, 13168–13178 CrossRef CAS PubMed.
- S. H. Cho, H. S. Park, S. W. Jo, E. J. Yim, H. Y. Yang, G. S. Ha, E. J. Kim, S. J. Yang and D. Y. Jeong, Comparison of microbial community profiling on traditional fermented soybean products (Deonjang, Gochujang) produced in Jeonbuk, Jeonnam, and Jeju province area, Korean J. Microbiol. Biotechnol., 2017, 53, 39–48 CrossRef.
- H.-E. Kim, S.-Y. Han and Y.-S. Kim, Quality characteristics of gochujang meju prepared with different fermentation tools and inoculation time of Aspergillus oryzae, Food Sci. Biotechnol., 2010, 19, 1579–1585 CrossRef.
- G. R. Shin, S. Lee, E. S. Jang, H. W. Shin, B. S. Moon and C. H. Lee, Metabolomics reveal that amino acids are the main contributors to antioxidant activity in wheat and rice gochujangs (Korean fermented red pepper paste), Food Res. Int., 2016, 87, 10–17 CrossRef.
- I.-S. Ahn, M.-S. Do, S.-O. Kim, H.-S. Jung, Y.-I. Kim, H.-J. Kim and K.-Y. Park, Antiobesity effect of Kochujang (Korean fermented red pepper paste) extract in 3T3-L1 adipocytes, J. Med. Food, 2006, 9, 15–21 CrossRef.
- K.-Y. Park, K.-R. Kong, K.-O. Jung and S.-H. Rhee, Inhibitory effects of Kochujang extracts on the tumor formation and lung metastasis in mice, Prev. Nutr. Food Sci., 2001, 6, 187–191 Search PubMed.
- P. Mahoro, H.-J. Moon, H.-J. Yang, K.-A. Kim and Y.-S. Cha, Protective Effect of Gochujang on Inflammation in a DSS-Induced Colitis Rat Model, Foods, 2021, 10, 1072 CrossRef CAS PubMed.
- C. Silberstein, A. Kierbel, G. Amodeo, E. Zotta, F. Bigi, D. Berkowski and C. Ibarra, Functional characterization and localization of AQP3 in the human colon, Braz. J. Med. Biol. Res., 1999, 32, 1303–1313 CrossRef CAS PubMed.
- M. Camilleri, A. C. Ford, G. M. Mawe, P. G. Dinning, S. S. Rao, W. D. Chey, M. Simrén, A. Lembo, T. M. Young-Fadok and L. Chang, Chronic constipation, Nat. Rev. Dis. Primers, 2017, 3, 17095 CrossRef.
- Y. Qian, J. Zhang, X. Zhou, R. Yi, J. Mu, X. Long, Y. Pan, X. Zhao and W. Liu, Lactobacillus plantarum CQPC11 Isolated from Sichuan Pickled Cabbages Antagonizes d-galactose-Induced Oxidation and Aging in Mice, Molecules, 2018, 23, 3026 CrossRef.
- J. Yin, Y. Liang, D. Wang, Z. Yan, H. Yin, D. Wu and Q. Su, Naringenin induces laxative effects by upregulating the expression levels of c-Kit and SCF, as well as those of aquaporin 3 in mice with loperamide-induced constipation, Int. J. Mol. Med., 2018, 41(2), 649–658 CAS.
- F. J. Ehlert, R. S. Ostrom and G. W. Sawyer, subtypes of the muscarinic receptor in smooth muscle, Life Sci., 1997, 61, 1729–1740 CrossRef CAS.
- Y. Tanahashi, N. Waki, T. Unno, H. Matsuyama, S. Iino, T. Kitazawa, M. Yamada and S. Komori, Roles of M2 and M3 muscarinic receptors in the generation of rhythmic motor activity in mouse small intestine, Neurogastroenterol. Motil., 2013, 25, e687–e697 CAS.
- T. Komuro, Structure and organization of interstitial cells of Cajal in the gastrointestinal tract, J. Physiol., 2006, 576, 653–658 CrossRef CAS.
- Y. Y. Tan, Z. L. Ji, G. Zhao, J. R. Jiang, D. Wang and J. M. Wang, Decreased SCF/c-kit signaling pathway contributes to loss of interstitial cells of Cajal in gallstone disease, Int. J. Clin. Exp. Med., 2014, 7, 4099–4106 Search PubMed.
- E. Ihara, H. Akiho, K. Nakamura, S. R. Turner and J. A. MacDonald, MAPKs represent novel therapeutic targets for gastrointestinal motility disorders, World J. Gastrointest. Pathophysiol., 2011, 2, 19 CrossRef.
- C. Cirillo and R. Capasso, Constipation and botanical medicines: an overview, Phytother. Res., 2015, 29, 1488–1493 CrossRef.
- S.-Y. Baek, N.-Y. Gil, M.-H. Han, H.-Y. Kang, H.-Y. Lee, H.-S. Yoon, J. Lee, Y.-E. Song, S.-K. Lee, J.-A. Ryu, H.-Y. Kim, S.-H. Yeo and S.-Y. Kim, Relationship between exterior environment and quality characteristics of the traditional Gochujang produced in 2018 by eight regions in Korea, Korean J. Food Preserv., 2019, 26, 745–755 CrossRef.
- M.-H. Ryu, J. Zhang, T. Toth, D. Khokhani, B. A. Geddes, F. Mus, A. Garcia-Costas, J. W. Peters, P. S. Poole, J.-M. Ané and C. A. Voigt, Control of nitrogen fixation in bacteria that associate with cereals, Nat. Microbiol., 2020, 5, 314–330 CrossRef CAS PubMed.
- L. Schiller, The therapy of constipation, Aliment. Pharmacol. Ther., 2001, 15, 749–763 CrossRef CAS PubMed.
- N. Ikarashi, R. Kon and K. Sugiyama, Aquaporins in the Colon as a New Therapeutic Target in Diarrhea and Constipation, Int. J. Mol. Sci., 2016, 17, 1172 CrossRef.
- B. J. Kim, J.-H. Hong, Y. S. Jeong and H. K. Jung, Evaluation of two Bacillus subtilis strains isolated from Korean fermented food as probiotics against loperamide-induced constipation in mice, J. Korean Soc. Appl. Biol. Chem., 2014, 57, 797–806 CrossRef.
- X. Ge, W. Zhao, C. Ding, H. Tian, L. Xu, H. Wang, L. Ni, J. Jiang, J. Gong, W. Zhu, M. Zhu and N. Li, Potential role of fecal microbiota from patients with slow transit constipation in the regulation of gastrointestinal motility, Sci. Rep., 2017, 7, 441 CrossRef.
- J. G. LeBlanc, F. Chain, R. Martín, L. G. Bermúdez-Humarán, S. Courau and P. Langella, Beneficial effects on host energy metabolism of short-chain fatty acids and vitamins produced by commensal and probiotic bacteria, Microb. Cell Fact., 2017, 16, 79 CrossRef PubMed.
- M. Camilleri, A. C. Ford, G. M. Mawe, P. G. Dinning, S. S. Rao, W. D. Chey, M. Simrén, A. Lembo, T. M. Young-Fadok and L. Chang, Chronic constipation, Nat. Rev. Dis. Primers, 2017, 3, 17095 CrossRef PubMed.
- Y. He, L. Zhu, J. Chen, X. Tang, M. Pan, W. Yuan and H. Wang, Efficacy of Probiotic Compounds in Relieving Constipation and Their Colonization in Gut Microbiota, Molecules, 2022, 27, 666 CrossRef CAS.
- X. Zhao, Y. Qian, G. Li, R. Yi, K.-Y. Park and J.-L. Song, Lactobacillus plantarum YS2 (yak yogurt Lactobacillus) exhibited an activity to attenuate activated carbon-induced constipation in male Kunming mice, J. Dairy Sci., 2019, 102, 26–36 CrossRef CAS.
- Y. Chai, Y. Huang, H. Tang, X. Tu, J. He, T. Wang, Q. Zhang, F. Xiong, D. Li and Z. Qiu, Role of stem cell growth factor/c-Kit in the pathogenesis of irritable bowel syndrome (Review), Exp. Ther. Med., 2017, 13, 1187–1193 CrossRef CAS PubMed.
- P. K. Shahi, S. Choi, D. C. Zuo, C. H. Yeum, P. J. Yoon, J. Lee, Y. D. Kim, C. G. Park, M. Y. Kim and H. R. Shin, 5-hydroxytryptamine generates tonic inward currents on pacemaker activity of interstitial cells of cajal from mouse small intestine, Korean J. Physiol. Pharmacol., 2011, 15, 129–135 CrossRef CAS PubMed.
- H.-R. Kim, I.-S. Park, S.-B. Park, H.-J. Yang, D.-Y. Jeong and S.-Y. Kim, Comparison of laxative effects of fermented soybeans (Cheonggukjang) containing toxins and biogenic amines against loperamide-induced constipation mouse model, Nutr. Res. Pract., 2022, 16(4), 435–449 CrossRef CAS.
- G. Yu, Y. Liu, W. Ou, J. Dai, Q. Ai, W. Zhang, K. Mai and Y. Zhang, The protective role of daidzein in intestinal health of turbot (Scophthalmus maximus L.) fed soybean meal-based diets, Sci. Rep., 2021, 11, 3352 CrossRef CAS PubMed.
- J. Qin, R. Li, J. Raes, M. Arumugam, K. S. Burgdorf, C. Manichanh, T. Nielsen, N. Pons, F. Levenez, T. Yamada, D. R. Mende, J. Li, J. Xu, S. Li, D. Li, J. Cao, B. Wang, H. Liang, H. Zheng, Y. Xie, J. Tap, P. Lepage, M. Bertalan, J. M. Batto, T. Hansen, D. Le Paslier, A. Linneberg, H. B. Nielsen, E. Pelletier, P. Renault, T. Sicheritz-Ponten, K. Turner, H. Zhu, C. Yu, S. Li, M. Jian, Y. Zhou, Y. Li, X. Zhang, S. Li, N. Qin, H. Yang, J. Wang, S. Brunak, J. Doré, F. Guarner, K. Kristiansen, O. Pedersen, J. Parkhill, J. Weissenbach, P. Bork, S. D. Ehrlich and J. Wang, A human gut microbial gene catalogue established by metagenomic sequencing, Nature, 2010, 464, 59–65 CrossRef CAS.
- T. G. de Meij, E. F. de Groot, A. Eck, A. E. Budding, C. F. Kneepkens, M. A. Benninga, A. A. van Bodegraven and P. H. Savelkoul, Characterization of microbiota in children with chronic functional constipation, PLoS One, 2016, 11, e0164731 CrossRef PubMed.
- M. A. Pourhoseingholi, S. A. Kaboli, A. Pourhoseingholi, B. Moghimi-Dehkordi, A. Safaee, B. K. Mansoori, M. Habibi and M. R. Zali, Obesity and functional constipation; a community-based study in Iran, J. Gastrointestin Liver Dis., 2009, 18, 151–155 Search PubMed.
- J. Jalanka, G. Major, K. Murray, G. Singh, A. Nowak, C. Kurtz, I. Silos-Santiago, J. M. Johnston, W. M. de Vos and R. Spiller, The effect of psyllium husk on intestinal microbiota in constipated patients and healthy controls, Int. J. Mol. Sci., 2019, 20, 433 CrossRef PubMed.
- E. Mani-López, E. Palou and A. López-Malo, Probiotic viability and storage stability of yogurts and fermented milks prepared with several mixtures of lactic acid bacteria, J. Dairy Sci., 2014, 97, 2578–2590 CrossRef PubMed.
|
This journal is © The Royal Society of Chemistry 2023 |
Click here to see how this site uses Cookies. View our privacy policy here.