DOI:
10.1039/D2FO03627D
(Paper)
Food Funct., 2023,
14, 4722-4733
A minor metabolite from Curcuma longa effective against metabolic syndrome: results from a randomized, double-blind, placebo-controlled clinical study†
Received
24th November 2022
, Accepted 6th April 2023
First published on 14th April 2023
Abstract
Metabolic syndrome (MetS) is characterized by the presence of at least three interrelated risk factors, including central obesity, hypertension, elevated serum triglycerides, low serum high-density lipoproteins, and insulin resistance. Abdominal obesity is considered a predominant risk factor. Lifestyle changes with medications to lower cholesterol, blood sugar, and hypertension are the general treatment approaches. Functional foods and bioactive food ingredients represent versatile tools for addressing different aspects of MetS. In a randomized placebo-controlled clinical study, we evaluated the effect of Calebin A, a minor bioactive phytochemical from Curcuma longa, on metabolic syndrome in obese adults (N = 100), and 94 individuals completed the study (N = 47 in both groups). They were subjected to Calebin A supplementation for 90 days, which resulted in a statistically significant reduction in their body weight, waist circumference, body mass index, low-density lipoprotein-cholesterol, and triglyceride levels compared to those with the placebo. A small but significant increase in high-density lipoprotein-cholesterol levels was also observed in these individuals. Furthermore, Calebin A showed a positive effect on adipokines by reducing circulating leptin levels. Finally, C-reactive protein levels were significantly reduced in Calebin A-supplemented individuals, suggesting a beneficial impact on managing MetS-induced inflammation. Blood glucose levels, insulin resistance, and blood pressure levels were not affected by Calebin A. In conclusion, Calebin A may be an effective supplement for managing abdominal obesity, dyslipidemia, and systemic inflammation in individuals with metabolic syndrome. This study was prospectively registered on the Clinical Trial Registry of India (CTRI) with the registration number CTRI/2021/09/036495. https://ctri.nic.in/Clinicaltrials/advancesearchmain.php
Introduction
Metabolic syndrome (MetS) represents a cluster of metabolic abnormalities, including abdominal obesity, hyperglycemia, glucose intolerance, arterial hypertension, and dyslipidemia, which greatly increase the risk for cardiovascular disease or type 2 diabetes.1 A recent study by the National Health and Nutrition Examination Survey (NHANES) reported an overall prevalence of MetS at 34.7%, with a significant increase among young adults in the age group of 20–39 years, a leading public health concern.2,3 The underlying pathophysiology of MetS and its multifaceted origin are not yet fully understood.4 The most widely accepted hypothesis suggests insulin resistance/hyperinsulinemia and central obesity as the main pathogenic mechanisms.5,6 However, other factors, such as oxidative stress and low-grade chronic inflammation, have also been proposed to be involved in the development and/or progression of the syndrome.7,8
MetS is usually managed by lifestyle changes, along with pharmacological treatment4 using statins, anti-diabetic and anti-hypertensive drugs, often in combination.1 An effective natural remedy could represent a valuable tool in the fight against MetS. The use of functional foods and natural bioactive compounds could exert beneficial effects on the various components of MetS like body weight, blood pressure, glucose metabolism, endothelial damage, and lipid profile, apart from the favorable effects on the inflammatory state and oxidative stress.
The rhizomes of the Curcuma longa plant are a rich source of numerous phytochemicals.9–11 Curcuminoids and turmerones are among the well-known researched materials. The minor components have hardly merited any serious scientific studies. The increasing use of turmeric powder in food and supplement products prompts one to study the physiological effects of the minor components. Calebin A (feruloylmethyl ferulate) is a phytochemical present in trace amounts in Curcuma species (Curcuma longa/caesia) and belongs to a family of ferulate esters, rightfully called calebenoids. While curcuminoids occur to the extent of 4–8% in Curcuma longa, Calebin A occurs in extremely minute quantities, <0.01% w/w, excluding the possibility of the plant being an economical source for this active phytochemical. The calebenoids seem to arise from a biological Bayer–Villiger type of transformation of curcuminoids.12 Endophytic fungi producing Bayer–Villiger monooxygenase were found to bio-transform curcumin to Calebin A.13,14 Though Calebin A is structurally related to curcumin, it lacks the beta-diketone group at 1,3-carbon, which is unique to curcumin's structure.15 Calebin A shows higher chemical stability in acidic and basic media in contrast to the noted instability of curcumin at higher pH values while retaining several physiological properties with curcumin.16
Calebin A exhibits a variety of pharmacological activities. Hence, it could be a potential compound in managing various human ailments such as metabolic disorders, obesity, arthritis, low bone density, and cancer.16 Lai et al. (2015) demonstrated that Calebin A prevents high-fat-diet-induced obesity, inhibits adipocyte differentiation, and ameliorates hepatic steatosis, by suppressing the expression of peroxisome proliferator-activated receptor (PPAR), fatty acid synthase and activated acetyl-CoA carboxylase.17 In the animal model of diet-induced obesity, Calebin A supplementation decreased weight gain, and visceral fat accumulation, which was suggested to be mediated by the activation of AMP-activated protein kinase in adipocytes and the liver.17 Furthermore, Calebin A inhibited osteoclastogenesis,18 protected cells from β-amyloid toxicity,19 and prevented fatty liver disease by inhibiting adipogenesis.20 Preclinical safety and toxicological studies have elaborated on the safety of Calebin A in animals as per the regulatory requirement.21
Although several preclinical studies describe the pharmacological uses of Calebin A, human clinical evaluation to translate the effects from animal studies to bedside is lacking. In the present study, we evaluated the effect of Calebin A supplementation on metabolic parameters associated with MetS in a randomized clinical study.
Materials and methods
Materials
The interventional materials for the study were supplied by Sami-Sabinsa Group Limited. Since Calebin A occurs only in trace amounts in the turmeric rhizome, it was chemically synthesized with >99% purity. The test product was a capsule containing 25 mg of Calebin A (CurCousin®) with 3 mg of piperine (BioPerine®), a safe food component known for increasing the bioavailability of phytoactives.22,23 Microcrystalline cellulose capsules were used as a placebo. Both capsules were identical in weight and appearance.
Calebin A
Calebin A was analyzed by reverse phase HPLC using a Thermo BDS Hypersil C18 column having a particle size of 5 μ, an internal diameter of 4.6 mm and a length of 250 mm. The mobile phase used was a mixture of acetonitrile and water in a ratio of 1
:
1 (v
:
v) with a flow rate of 1.0 mL min−1. Standard and sample solutions were prepared in acetonitrile
:
phosphate buffer with pH 4.0 in a ratio of 1
:
1.25 (v
:
v) at a concentration of 0.025 mg mL−1 and 20 μL was injected into the HPLC. A Calebin A peak was detected at 340 nm and elutes were obtained at about 4.9 min. The spectral properties of Calebin A were in accordance with earlier reports.13,19 A typical HPLC chromatogram of Calebin A along with its structural similarity with curcumin are depicted in Fig. 1.
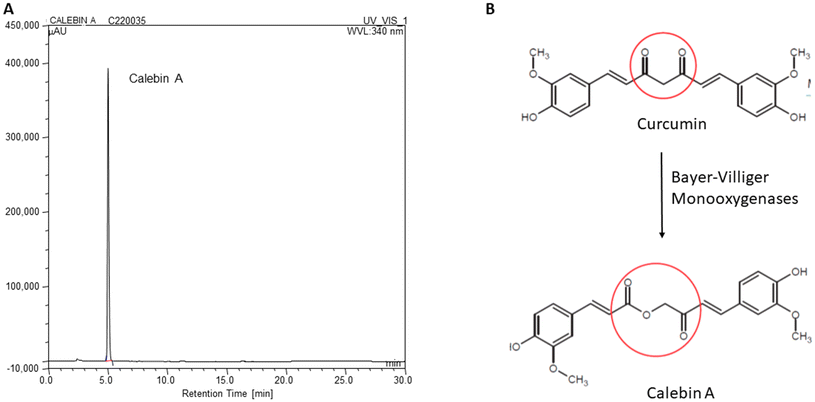 |
| Fig. 1 Structure and HPLC profile of Calebin A. A: The difference in the structures of Calebin A and Curcumin, B: HPLC profile of Calebin A used for the study. | |
Study design and ethics
The study was conducted as a multi-centric, randomized, double-blind, placebo-controlled clinical trial from April 2021 to August 2022 at three sites, Apollo First Med Hospitals in Chennai, Santosh Hospital in Bangalore, and Vijaya Specialty Hospital in Nellore. The study was carried out by following the approved ethical guidelines of the Central Drugs Standard Control Organization (September 2019), ICMR Guidelines 2017, Schedule Y (Drug & Cosmetic Act, India 2014), the World Medical Association Declaration of Helsinki, Fortaleza, 2013 & the ICH (Step V), and “Guidance on Good Clinical Practice” (GCP). The entire study protocol was approved by the ethics committee of Apollo First Med Hospitals in Chennai, Santosh Hospital in Bangalore, and Vijaya Specialty Hospital in Nellore in Southern India, and no changes were made to the study design after recruitment had commenced. Written informed consent was obtained from all the participants at the time of enrolment, and the study was registered prospectively with the registration number CTRI/2021/09/036495.
Sample size
Male and female obese subjects (N = 100) in the age group of 30 to 65 years (both inclusive) who met all the inclusion criteria were enrolled in this study (Fig. 2). The sample size was calculated based on the earlier research publication.24 Using these inputs with 80% power and alpha = 0.05 significance level and assuming a correlation of 0.2, the required sample size was calculated to be at least 60 participants. Allowing a 20% dropout, the sample size was estimated at 72 participants. Therefore, we recruited 100 participants in a 1
:
1 ratio between two treatment groups (N = 50 per treatment group).
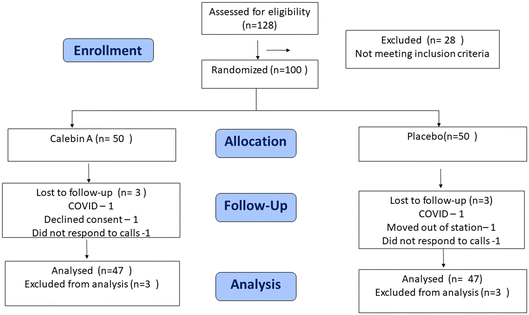 |
| Fig. 2 CONSORT flow diagram. | |
Inclusion and exclusion criteria
Overweight individuals in the age group of 30 to 65 years (both inclusive), with a waist circumference of ≥90 cm (men) or ≥80 cm (women) and the presence of any two or more of the following conditions according to the International Diabetes Federation (IDF) were included in this study: (a) fasting blood glucose of ≥5.6 mmol L−1 (100 mg dL−1) or diagnosed diabetes; (b) high-density lipoprotein cholesterol (HDL-C) of <1.0 mmol L−1 (40 mg dL−1) in men, <1.3 mmol L−1 (50 mg dL−1) in women; (c) blood triglycerides of >1.7 mmol L−1 (150 mg dL−1); (d) blood pressure of >130/85 mmHg. All the participants enrolled in the study had a BMI of ≥28 kg m−2 and ≤35 kg m−2 and were willing to take up regular physical activity. Exclusion criteria included the presence of any genetic syndromes associated with obesity (Cushing's syndrome, Turner's syndrome, and Prader-Willi's syndrome) based on the medical records of participants screened by the principal investigator, excessive alcohol use, pregnancy, thyroid diseases, hepatic/cardiac/pulmonary/psychiatric diseases, malignancies and presence of chronic inflammatory conditions, history of coagulopathies, cardiovascular diseases, congestive heart failure, pancreatitis, lactic acidosis, hepatomegaly with steatosis, motor weakness, peripheral sensory neuropathy, psychiatric disorder, uncontrolled bronchial asthma and/or Chronic Obstructive Pulmonary Disorder [COPD] were excluded from the study. Participants with a fasting blood glucose of ≥150 mg dL−1 and HbA1c of >7%, hypertension with a blood pressure of >160/100 mmHg or undergone weight loss (−5%) in the last three months were also excluded from the study. Details are given in the ESI section.†
Randomization and blinding
One hundred participants meeting the eligibility criteria were enrolled in the study. The subjects were randomized using a predetermined randomization schedule generated using computer-based randomization software STATA15, prepared by a statistician, independent of the sponsoring organization and not involved in the conduct or reporting of the study. An alpha code was generated for both the active and placebo to improve the blindness of the study and concealment of allocations. Block randomization (only one block) was followed wherein the subjects were randomized to receive either a placebo or an investigational product. The randomization codes were kept strictly confidential and were accessible only to authorized persons on an emergency basis as per the standard operating procedures of the sponsor until the time of unblinding. The study was double-blinded, wherein, the study groups, investigators, and other staff were blinded to the group assignment. The principal investigator assigned the subjects to two different groups using the alpha codes. The treatment codes were kept in a sealed envelope, which was not opened until study completion or in case of an emergency, i.e., a serious adverse event.
Intervention
The participants received either a Calebin A capsule (containing 25 mg of Calebin A and 3 mg of piperine) or a placebo twice daily, 30 minutes after breakfast and dinner, for 90 days (Fig. 2). Participants were advised to adhere to their usual lifestyle, continue to consume their regular diet but avoid junk foods and fried items. They were advised to undertake physical activity of walking for 30 minutes daily for five days a week, which was monitored through a patient record diary. After every 30 days, they were asked to visit the study site to assess outcome measures. All the participants were telephonically followed up 15 days after the final visit for overall health and any adverse events.
Outcome measures and endpoints
During every visit, all the participants underwent a medical and physical examination. The primary endpoints were to measure the mean change in body weight and fasting blood sugar (FBS) between the baseline (day 0) and the final visit (day 90). Secondary endpoints were to assess the efficacy of Calebin A through the change in anthropometric measurements (waist and hip circumferences), body mass index (BMI), blood pressure, lipid profile, serum biomarkers (Leptin, Adiponectin, and Insulin), glycosylated hemoglobin (HbA1c), and high sensitive C-reactive protein (hs-CRP), and vital signs (body temperature, pulse rate, and blood pressure). Safety was monitored throughout the study by recording any adverse events and by any change in vital parameters, biochemical, hematological, and urinary markers. Body weight measurement of the participants were taken in a surgical gown without footwears and other external apparel, using a weighing balance from the same manufacturer and model at each site. The waist circumference was measured by placing the measuring tape horizontally around the waist just above the hip bone. The details are provided in the ESI section.† The height was measured using a stadiometer.
Biochemical and biomarker evaluation
The serum biochemical parameters were estimated in the SRL Diagnostics lab (Gurugram India), using a COBAS 8000 biochemical analyzer. Biomarkers leptin and Adiponectin levels were estimated by the enzyme linked immunosorbent assay (ELISA), using commercial kits (Diagnostics Biochem, Canada, as per the manufacturer's instructions. The levels of HbA1c were measured by iron exchange HPLC, liver/renal enzymes by spectrophotometry, Hs-CRP by nephelometry, the thyroid profile by competitive electrochemiluminescence and urine analysis was carried out using an integrated automated system. The details of lab evaluations are given in the ESI section.† Insulin resistance was calculated using the fasting glucose (mg dL−1) and insulin levels (μU mL−1) according to the homeostasis model assessment (HOMA-IR) method: glucose × insulin/405. The HOMA-β-cell function (HOMA-β) was calculated using the following formula: 360 × fasting insulin (μU mL−1)/(fasting glucose (mg dL−1) − 63).25 The Apolipoprotein B level (ApoB100) was calculated using the formula: [ApoB100 = −33.12 + 0.675 × LDL + 11.95 × ln(tg)].26
Safety measurements
The safety data were monitored throughout the course of the study by the principal investigator at every visit by physical examination and incidence of unfavorable changes in health. The changes in the pulse rate, body temperature, clinical biochemical parameters including, the liver profile, renal profile, thyroid profile, hematology, urine analysis and electrolyte levels were monitored from the baseline to the end of the study.
Statistical analysis
The statistical analysis was conducted using the STATA Software version 15.0 on the study population who completed the study. For continuous variables, the normality of distribution was tested by a one-sample Shapiro–Wilk normality test. The paired t-test or Wilcoxon Signed Rank Test was used to compare quantitative variables within the group and the independent T-test or Mann–Whitney U test between the groups based on their normal distribution. Categorical variables were compared using Chi-square tests. Repeated measure analysis of variance (ANOVA) was used to compare the parameters that were monitored every visit. The change in efficacy parameters from the baseline to the end of the study was compared between the treatment groups to assess the treatment effect. The level of statistical significance is defined as p < 0.05.
Results
Demographic details at the baseline of the study
A total of 94 participants (N = 47, each in placebo and Calebin A groups) completed the study and were included in the data analysis. Three participants from each group were lost to follow-up (Fig. 2). The baseline variables, including the gender distribution, body weight, blood pressure, and fasting sugar, were comparable between the Calebin A and placebo groups (Table 1). The average age was 45.25 ± 8.14 in Calebin A and 41.27 ± 7.11 in placebo (P = 0.02). All the participants were Indians, and none had a smoking or alcohol abuse history. All the participants were overweight, with an average body weight of 87.53 ± 8.32 kgs and a BMI of 31.18 ± 1.75 kg m−2. The waist circumferences of the participants were more than 94 cm in males and ≥80 cm in females. The mean triglyceride levels were 167.4 ± 63.6 mg dL−1 and fasting blood sugar level was 104.5± 15.6 mg dL−1. All the participants satisfied at least two conditions of metabolic syndrome apart from central obesity, conforming to the IDF definition of MetS. All the participants were advised to walk for 30 minutes five days a week during a study period of 90 days.
Table 1 Demographic details
Parameters |
Calebin A (N = 47) |
Placebo (N = 47) |
Overall (N = 94) |
P value |
Demographic values were compared between the two groups at the baseline. The quantitative variables were compared by independent T-tests, while the chi-square test was used to compare categorical variables. None of the parameters, except age (P = 0.02), were significantly different between the groups. WC: waist circumference, BMI: body mass index, SBP: systolic blood pressure, and DBP: diastolic blood pressure. |
Age (years) |
45.25 ± 8.14 |
41.27 ± 7.11 |
43.26 ± 7.86 |
0.02 |
Gender, n (%)
|
|
|
|
|
Male |
30 (63.82%) |
31 (65.95%) |
61 (64.89%) |
0.83 |
Female |
17 (36.17%) |
16 (34.04%) |
33 (35.10%) |
|
WC (Cm)
|
|
|
|
|
Male |
98.10 ± 7.03 |
100.53 ± 8.92 |
99.21 ± 8.03 |
0.23 |
Female |
93.88 ± 11.84 |
94.38 ± 9.21 |
94.12 ± 10.48 |
0.57 |
Total |
96.57± 9.17 |
98.57 ± 9.22 |
97.42 ± 9.24 |
0.26 |
Weight (kg) |
87.51 ± 7.79 |
87.52 ± 8.91 |
87.53 ± 8.32 |
0.72 |
BMI (kg m−2) |
30.79 ± 1.71 |
31.02 ± 1.78 |
31.18 ± 1.75 |
0.36 |
Height (cm) |
167.05 ± 6.52 |
167.76 ± 6.39 |
167.40 ± 6.43 |
0.63 |
SBP mmHg |
136.68 ± 7.61 |
137.72 ± 7.06 |
137.20 ± 7.32 |
0.65 |
DBP mmHg |
85.87 ± 5.99 |
84.85 ± 8.48 |
85.36 ± 5.71 |
0.66 |
Pulse rate |
82.34 ± 6.78 |
82.78 ± 5.78 |
82.56 ± 6.57 |
0.51 |
Triglycerides mg dL−1 |
167.91 ± 77.22 |
166.89 ± 47.21 |
167.4± 63.65 |
0.96 |
FBS mg dL−1 |
108.10 ± 16.34 |
105.37 ± 14.77 |
104.5 ± 15.6 |
0.62 |
Effect of Calebin A on anthropometric parameters and blood pressure
Consumption of Calebin A for 90 days significantly reduced anthropometric characteristics. The mean waist circumference was reduced by 3.75 cm in individuals consuming Calebin A, which was significantly better than those consuming placebo. The body weight of the participants in the placebo group was reduced by 0.94 kg compared to 5.32 kg in the Calebin A group (P < 0.001). Other parameters like the BMI (1.88 kg m−2 compared to 0.29 kg m−2 in placebo) and hip circumference (4.02 cm vs. 0.77 cm) also reduced significantly in Calebin A-supplemented participants, suggesting its profound effect on overall weight management (Table 2). Weight loss, reduction in the waist circumference, and related parameters were observed after a month of supplementation, which gradually decreased further in three months (Fig. 3). The systolic blood pressure was reduced in the placebo and Calebin A groups, but the difference was insignificant.
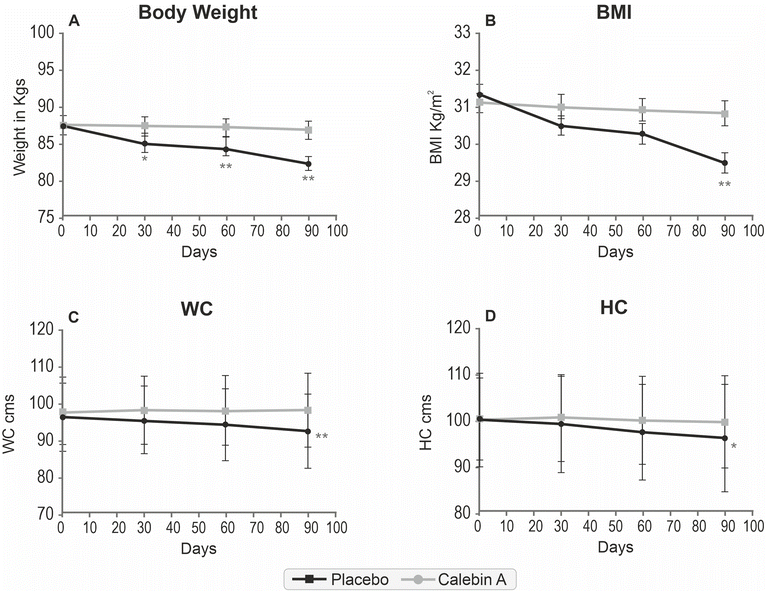 |
| Fig. 3 Reduction in body weight and related parameters over time by Calebin A. (A) Reduction in (B) body weight, body mass index (BMI) (C) waist circumference (WC), and (D) hip circumference (HC) with time is depicted in the graphs. One-way repeated measures ANOVA with Dunnett's post-hoc test was used to compare the changes within the group from the baseline. *P < 0.05 and **P < 0.01 compared to the baseline. | |
Table 2 Effect of Calebin A on anthropometric parameters and blood pressure and blood pressure
Parameters |
Group |
Day 0 |
Day 90 |
Mean diff. |
p-Value |
The values related to the body weight, body mass index (BMI), waist circumference (WC) and hip circumference (HC), systolic blood pressure (SBP), and diastolic blood pressure (DBP) from the baseline to the end of the study are represented as mean ± SD. The change in values after 90 days of supplementation were compared within the groups by paired T test (*) and between the group by independent (unpaired) T test (#), the mean difference between Calebin A and placebo were compared by the Mann Whitney test. |
Body weight (kg) |
Placebo |
87.52 ± 8.91 |
86.82 ± 8.59* |
−0.94 |
<0.001 |
Calebin A |
87.55 ± 7.79 |
82.24 ± 6.74***# |
−5.32 |
BMI (kg m−2) |
Placebo |
31.08 ± 1.71 |
30.79 ± 1.91 |
−0.29 |
<0.001 |
Calebin A |
31.33 ± 1.79 |
29.44 ± 2.30***# |
−1.88 |
Waist circumference (cm) |
Placebo |
98.28 ± 9.33 |
98.53 ± 10.23 |
0.26 |
<0.001 |
Calebin A |
96.57 ± 9.17 |
92.82 ± 10.09*** |
−3.75 |
Hip circumference (cm) |
Placebo |
100.43 ± 9.18 |
99.66 ± 10.11** |
−0.77 |
0.016 |
Calebin A |
100.0 ± 10.25 |
95.98 ± 11.66***# |
−4.02 |
SBP mmHg |
Placebo |
137.72 ± 7.06 |
130.12 ± 8.76** |
−5.30 |
0.41 |
Calebin A |
136.68 ± 7.61 |
131.38 ± 7.69** |
−7.60 |
DBP mmHg |
Placebo |
84.85 ± 8.48 |
83.48 ± 7.04 |
−1.36 |
0.44 |
Calebin A |
85.87 ± 5.99 |
82.74 ± 6.64* |
−3.13 |
Effect of Calebin A on lipid and sugar profiles
Calebin A supplementation showed a positive effect on lipid levels. Total cholesterol (−13.65 mg dL−1), low-density lipoprotein cholesterol (LDL-C) (−9.53 mg dL−1), very low-density lipoprotein cholesterol (VLDL-C) (−1.75 mg dL−1), and triglyceride (−11.51 mg dL−1) levels reduced significantly, and this difference was significantly better than that of the placebo group. The high-density lipoprotein cholesterol (HDL-C) level increased to 2.65 mg dL−1 in the Calebin A group, while it decreased (0.51 mg dL−1) in the placebo group. We calculated the ApoB100 levels based on the LDL-C and TG levels as they are known to be a better predictor of cardiometabolic risks.27 The calculated ApoB100 levels reduced by −6.16 in the Calebin A group compared to −2.81 in the placebo group, which was a significant difference. Fasting blood sugar and HbA1c levels were not impacted by Calebin A. A mild reduction was observed in the placebo and Calebin A groups, which was not statistically significant (Table 3).
Table 3 Effect of Calebin A on lipid and sugar profiles
Parameters |
Group |
Day 0 |
Day 90 |
Mean difference |
p-Value |
The values are represented as median and interquartile range. The change in the levels of the parameters after 90 days of supplementation were compared. The changes in values after 90 days of supplementation were compared within the groups (*) by the Wilcoxon signed rank test. The mean difference between Calebin A and placebo were compared by the Mann Whitney test. TC; total cholesterol, LDL: low-density lipoprotein, VLDL: very low-density lipoprotein, HDL: high-density lipoprotein, TG: triglycerides, Apo B: apolipoprotein B, FBS: fasting blood sugar, HbA1c; glycosylated hemoglobin. HOMA-IR, homeostatic model assessment for insulin resistance, and HOMA-β, homeostatic model assessment of beta cells. |
TC mg dL−1 |
Placebo |
155.0 (146.0, 195.0) |
167.0 (144.0, 191.0) |
−4.57 |
0.01 |
Calebin A |
159.0 (145.0, 207.0) |
153.0 (138.0, 187.0)** |
−13.65 |
LDL-C mg dL−1 |
Placebo |
91.0 (973.0, 125.00) |
99.0 (68.0, 116.0) |
−4.45 |
0.006 |
Calebin A |
95.0 (72.0, 135.0) |
79.0 (67.0, 116.0)** |
−9.53 |
VLDL-C mg dL−1 |
Placebo |
33.0 (25.1, 36.9) |
30.0 (24.5, 34.5) |
−0.51 |
0.001 |
Calebin A |
33.7 (27.9, 36.0) |
31.0 (26.0, 33.0)* |
−1.75 |
HDL-C mg dL−1 |
Placebo |
42.0 (38.0, 45.0) |
42.0 (36.0, 47.0) |
−0.51 |
<0.001 |
Calebin A |
40.0 (37.0, 42.0) |
44.5 (40.0, 48.0)* |
+2.65 |
TG mg dL−1 |
Placebo |
169.0 (9116.0, 184.0) |
153.0 (123.0, 181.0) |
1.47 |
0.002 |
Calebin A |
169.0 (139.0, 177.0) |
153.0 (114.0, 165.0)* |
−11.51 |
ApoB 100 mg dL−1 |
Placebo |
84.21 (77.17, 112.8) |
89.25 (72.89, 108.8) |
−2.81 |
0.02 |
Calebin A |
90.02 (75.98, 122.0) |
80.70 (69.93, 103.60) |
−6.16 |
FBS mg dL−1 |
Placebo |
104.0 (98.0, 117.0) |
102.0 (96.0, 106.5) |
−2.93 |
0.14 |
Calebin A |
105.0 (100.5, 109.0) |
103.0 (95.0, 108.0) |
−5.62 |
HbA1c% |
Placebo |
6.1 (5.7, 6.3) |
5.9 (5.5, 6.1) |
−0.14 |
0.87 |
Calebin A |
6.1 (5.6, 6.4) |
6.0 (5.5, 6.1) |
−0.16 |
HOMA-IR |
Placebo |
3.34 (2.55, 5.58) |
43.01 (2.15, 4.79) |
0.18 |
0.59 |
Calebin A |
4.14 (2.53, 6.20) |
3.88 (2.55, 5.53) |
−0.12 |
HOMA-β |
Placebo |
44.66 (36.82, 71.55) |
40.79 (32.06, 60.0) |
2.68 |
0.23 |
Calebin A |
52.65 (36.05, 86.59) |
60.74 (37.29, 76.29) |
3.18 |
Effect of Calebin A on serum biomarkers
The levels of leptin reduced significantly in Calebin A-supplemented individuals by 9.21 ng ml−1. The placebo did not have any effect on leptin, showing an increase in the mean levels (0.68 ng ml−1) at the end of the treatment period. Adiponectin was observed to increase in both groups, but was not significant. The difference in the adiponectin increase was higher in the Calebin A group (0.57 μg ml−1vs. 0.05 μg ml−1), which was significantly better. The levels of the C-reactive protein, an acute phase protein, and a marker for inflammation were lowered significantly by Calebin A (2.9 μg ml−1) compared to the placebo (0.4 μg ml−1), suggesting an anti-inflammatory effect of Calebin A (Fig. 4).
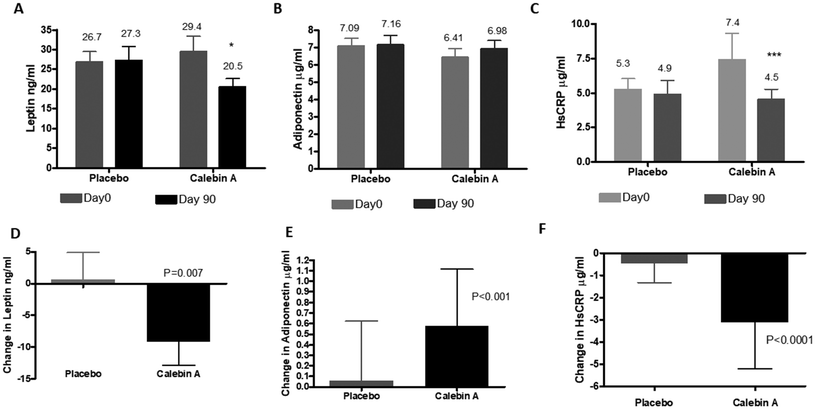 |
| Fig. 4 Effect of Calebin A on serum biomarkers. Circulating levels of A: leptin, B: adiponectin, and C: high sensitivity C-reactive protein at the baseline and after 90 days of supplementation. The change in their levels in 90 days is represented in D–F. The change in values from the baseline to 90 days of supplementation was compared within the groups by the Wilcoxon signed rank test (*P < 0.05 and ***P < 0.01). The mean difference between Calebin A and placebo were compared by the Mann Whitney test. | |
Safety outcomes
Two participants, one from Calebin A and one from the placebo group reported diarrhea during the study. The events were resolved without any medications and were not related to the medications of this study (Table 4). Calebin A and placebo supplementation were continued without a break and no participant discontinued the study due to any adverse events. The clinical safety parameters did not show any significant changes from the baseline to 90 days. The hematological parameters, liver and renal function test, and urine analysis were in the normal range in both Calebin A and the placebo groups at the end of the study (ESI Tables 1–3†).
Table 4 List of adverse events and their resolution
Participant no. |
Gender-age |
Group |
Event term |
Start date |
End date |
Outcome |
V03-036 |
F-51 |
Calebin A |
Diarrhea |
13/6/22 |
14/6/22 |
Resolved |
V03-040 |
F-44 |
Placebo |
Diarrhea |
16/6/22 |
17/6/22 |
Resolved |
Discussion
The present study evaluated the effect of Calebin A supplements on metabolic syndrome in obese individuals. The results showed a significant reduction in body weight, waist circumference, BMI, total and LDL-cholesterol, triglyceride levels and a small but significant increase in HDL-C in individuals consuming Calebin A compared to placebo at the end of 90 days. Leptin and hs-CRP levels were decreased by Calebin A, suggesting a beneficial effect on managing MetS-induced inflammation. Surprisingly, blood glucose levels, insulin resistance, and blood pressure levels were not affected by Calebin A supplementation.
Calebin A decreased the expression of PPAR γ, acetyl CoA carboxylase (ACC) and fatty acid synthase, resulting in reducing lipid accumulation in pre-adipocyte cell lines in vitro. In animal models of diet-induced obesity, Calebin A was found to reduce weight accumulation and improve hepatic steatosis by activating AMPK, a key metabolic regulator responsible for modulating cellular metabolism, mitochondrial biogenesis, fatty acid oxidation and loss of adipose weight.20,28 The activation of AMPK downregulates ACC, resulting in the reduction of malonyl CoA and increase in carnitine palmitoyltransferase-1 (CPT-1), further resulting in reduced triglyceride accumulation through increased fatty acid oxidation.29 Extending this observation, a recent study demonstrated that Calebin A enriched the microbiota of high-fat diet-fed mice with Akkermansia, Butyricicoccus, and Ruminiclostridium in association with weight reduction, blood sugar and white adipocyte content reduction and induced thermogenesis in mice.30 The anti-obesity effect of Calebin A could be translated in this clinical study, wherein we observed a 6% reduction in the body weight and BMI and close to a 4% reduction in the waist circumference in the absence of any caloric restriction. In an earlier clinical study, a Curcuma caesia extract containing Calebin A was shown to have similar effects.24
Obesity and overweight are associated with dyslipidemia, which is generally associated with increased triglycerides and free fatty acids and decreased HDL-C.31 LDL-C, VLDL-C and ApoB100 are also increased in these individuals due to the hepatic overproduction of ApoB100-containing lipoproteins.32,33 We observed a reduction in triglyceride levels by 6.9%, and an increase in HDL levels by 6.08% in Calebin A-treated individuals. We also observed a decrease in LDL and VLDL cholesterol, suggesting an overall positive effect on the lipid profile. These results agree with the preclinical and clinical studies wherein lipid levels were positively modulated by Calebin A.20,24 The possible mechanism of the lipid lowering effect of Calebin A could be due to an enhancement in fatty acid oxidation as observed in preclinical studies.20 In addition, we observed a 6.34% decrease in the calculated ApoB100 levels, which directly reflects the number of atherogenic particles, including LDL, VLDL, and intermediate-density lipoproteins.34 ApoB100 has been found to be a better predictor of CVD risks than LDL-C in several epidemiological studies.35,36
Insulin resistance and progressive pancreatic β-cell dysfunction are considered the two primary features in the pathogenesis of type 2 diabetes. The homeostasis model assessment (HOMA), a mathematical calculation derived from fasting glucose and insulin levels, is a validated clinical and epidemiological tool for estimating insulin resistance and β-cell function.37,38 We could not find a difference between Calebin A and the placebo in reducing the fasting blood sugar or HbA1c levels in serum. A very mild reduction in insulin resistance and an improvement in β-cell functions were observed with Calebin A intake, which was not significant. In animal studies, Calebin A was found to reduce blood glucose levels.20,30In vitro and in silico studies have shown an inhibitory effect of Calebin A on dipeptidyl peptidase-IV (DPP-IV) enzyme activity, suggesting a possible effect of diabetes.39 AMPK activation is also known to have a positive influence on diabetes. The participants enrolled in the present study had very mild prediabetic conditions, unlike the high fat-induced animal model, where the blood glucose levels increased significantly compared to control. It may be valuable to target individuals with type 2 diabetes with higher blood sugar and HbA1c levels in future studies to clearly understand the effect of Calebin A on controlling blood sugar levels.
An increase in free fatty acids plays a critical role in the pathogenesis of inflammation in metabolic syndrome individuals.40,41 Inflammation in the adipose tissue, pancreas, kidneys, and liver is also associated with metabolic diseases.42,43 Increased plasma concentrations of C-reactive proteins (CRP), tumor necrosis factor (TNF)-α, IL-1, and IL-6 have been reported in individuals with metabolic diseases.43 As a marker of inflammation, the CRP, was found to be higher among subjects with MetS and more recently has been characterized as an independent predictor of future cardiovascular events in MetS patients.44 The CRP concentrations were higher among subjects with MetS in the National Health and Nutrition Examination Survey.45 The hs-CRP levels were positively correlated with waist circumference, BMI, blood pressure, insulin resistance, lipid and glucose levels.46 Calebin A has been shown to have anti-inflammatory activity by targeting the NF-κb signaling pathway.47 We observed a significant reduction in hs-CRP levels in individuals consuming Calebin A, confirming the in vitro observation. The significant decrease in hs-CRP is likely to induce additional benefits for managing metabolic syndrome.
Leptin and adiponectin are other biomarkers associated with MetS.48 As members of the adipokine family of cytokines secreted from the adipose tissue, adiponectin and leptin carry out antagonistic functions in the body. Leptin supports appetite regulation by inducing satiety and maintaining fat storage in the body, enhances glucose utilization, prevents insulin hypersecretion, and is involved in fat metabolism.49–51 Leptin levels are increased in individuals with MetS and is considered a predictive marker.52–54 We observed a significant decrease in circulating leptin levels in the Calebin A group compared to the placebo group, suggesting a correlation between a reduction in visceral adiposity and leptin in the MetS patients participating in the study.
Adiponectin is involved in energy metabolism and exhibits anti-inflammatory effects, including its role as an insulin sensitizer.55 Low adiponectin levels were found in obese individuals and are associated with insulin resistance, diabetes, and cardiovascular disease.56,57 In our study, the increase in adiponectin levels did not reach significance with Calebin A supplementation, although, the difference in the levels from the baseline to the end of the study was significantly higher compared to placebo.
The possible mechanisms of Calebin A could be mediated by increased fatty acid oxidation, reflecting a positive effect on the lipid profile. Other mechanisms could be mediated through its effect on inflammation and leptin levels. Leptin in its normal physiological role is involved in energy regulation, and satiety.49 Paradoxically, a state of leptin resistance and increased levels of circulating leptin were observed in overweight/obese individuals, reflecting a state of the leptin resistance.58 Several mechanisms have been proposed for leptin resistance, while the presence of high levels of leptin could also trigger a resistance,59 thus, reducing leptin levels could be a possible mechanism of weight reduction. A recent study showed that a targeted anti-inflammatory therapy had a positive effect on weight reduction and metabolic dysfunction.60 Calebin A is a known antioxidant and has shown anti-inflammatory activity in cell lines and animal studies.18,20,30,47,61 The observed effect of Calebin A in this study could be a cumulative impact on multiple pathways involved in metabolic dysfunction.
In summary, Calebin A could reduce visceral obesity, dyslipidemia, and inflammation. Visceral fat is central to the development of MetS. The free fatty acids from adipocytes are packed into LDL and VLDL, and lower HDL levels. Dysfunctional HDL, in turn, can increase inflammation and reduce AMPK and nitric oxide levels, causing vasoconstriction and hypertension. By influencing these functions, Calebin A may benefit obese/overweight individuals with MetS.
Calebin A can be combined with herbal extracts with proven effects on metabolic syndrome especially diabetic parameters to increase the benefits of a formulation in Met S. The Emblica officinalis extract, grape seed extract,62,63 and curcuminoids64 are known to have antioxidant and anti-inflammatory properties due to the polyphenol content and may have a synergistic effect on different aspects of metabolic dysregulation. Some limitations of the study are that all the participants were in the early stages of MetS and were from the southern part of India. The participants were not given a caloric restriction, although they were asked to undertake 30 minutes of physical exercise five days a week.
Conclusion
In conclusion, Calebin A could be a promising supplement to manage body weight, dyslipidemia and mild inflammation associated with metabolic dysfunction. Hs-CRP is increasingly recognized as the important inflammatory parameter to be controlled and Calebin A does have a mitigating effect on this marker. In the present study, Calebin A supplementation did not show any effect on blood glucose levels and associated parameters. Future studies with a longer duration and a higher dosage of Calebin A on overweight individuals with type 2 diabetes would be helpful in elucidating its effects on hyperglycemia which is an important parameter for metabolic syndrome.
Abbreviation
AE | Adverse event |
Apo B100 | Apolipoprotein B |
BMI | Body mass index |
CVD | Cardiovascular disease |
DBP | Diastolic blood pressure |
FBS | Fasting blood sugar |
GRAS | Generally regarded as safe |
HbA1c | Glycosylated hemoglobin |
HC | Hip circumference |
HDL | High-density lipoprotein |
HOMA-β | Homeostasis model assessment – β-cell function |
HOMA-IR | Homeostasis model assessment of insulin resistance |
hs-CRP | High-sensitivity C-reactive protein |
LDL | Low-density lipoprotein |
MetS | Metabolic syndrome |
NF-κB | Nuclear factor kappa B |
SBP | Systolic blood pressure |
TC | Total cholesterol |
TG | Triglycerides |
VLDL | Very-low-density lipoprotein |
WC | Waist circumference |
Ethics approval
The study was approved by the Institutional Ethics Committee of Apollo First Med Hospitals, Chennai; Santosh Hospital, Bangalore and Vijaya Specialty Hospital, Nellore.
Patient consent statement
All participants signed an informed written consent to participate in the study.
Conflicts of interest
MM, KN and LM are affiliated with Sami-Sabinsa Group Limited. TVD, SS and SVKR do not have any conflict of interest to declare. Sabinsa Corporation owns the trademark CurCousin®.
Acknowledgements
The authors would like to acknowledge the entire clinical research team from the Sami-Sabinsa group, Apollo First Med Hospitals, Chennai; Santosh Hospital, Bangalore; and Vijaya Specialty Hospital, Nellore. The authors thank the statistician Mr Kamal Kammili, M/s. Sanjeevani, Bio Services Pvt. Ltd, who independently analyzed the data.
References
- F. F. Lillich, J. D. Imig and E. Proschak, Multi-Target Approaches in Metabolic Syndrome, Front. Pharmacol., 2020, 11, 554961 CrossRef CAS PubMed.
- G. Hirode and R. J. Wong, Trends in the Prevalence of Metabolic Syndrome in the United States, 2011-2016, J. Am. Med. Assoc., 2020, 323, 2526–2528 CrossRef PubMed.
- J. Scholze, E. Alegria, C. Ferri, S. Langham, W. Stevens, D. Jeffries and K. Uhl-Hochgraeber, Epidemiological and economic burden of metabolic syndrome and its consequences in patients with hypertension in Germany, Spain and Italy; a prevalence-based model, BMC Public Health, 2010, 10, 529 CrossRef PubMed.
- P. Perez-Martinez, D. P. Mikhailidis, V. G. Athyros, M. Bullo, P. Couture, M. I. Covas, L. de Koning, J. Delgado-Lista, A. Diaz-Lopez, C. A. Drevon, R. Estruch, K. Esposito, M. Fito, M. Garaulet, D. Giugliano, A. Garcia-Rios, N. Katsiki, G. Kolovou, B. Lamarche, M. I. Maiorino, G. Mena-Sanchez, A. Munoz-Garach, D. Nikolic, J. M. Ordovas, F. Perez-Jimenez, M. Rizzo, J. Salas-Salvado, H. Schroder, F. J. Tinahones, R. de la Torre, B. van Ommen, S. Wopereis, E. Ros and J. Lopez-Miranda, Lifestyle recommendations for the prevention and management of metabolic syndrome: an international panel recommendation, Nutr. Rev., 2017, 75, 307–326 CrossRef PubMed.
- D. B. Carr, K. M. Utzschneider, R. L. Hull, K. Kodama, B. M. Retzlaff, J. D. Brunzell, J. B. Shofer, B. E. Fish, R. H. Knopp and S. E. Kahn, Intra-abdominal fat is a major determinant of the National Cholesterol Education Program Adult Treatment Panel III criteria for the metabolic syndrome, Diabetes, 2004, 53, 2087–2094 CrossRef CAS PubMed.
- S. Guo, Insulin signaling, resistance, and the metabolic syndrome: insights from mouse models into disease mechanisms, J. Endocrinol., 2014, 220, T1–T23 CAS.
- E. McCracken, M. Monaghan and S. Sreenivasan, Pathophysiology of the metabolic syndrome, Clin. Dermatol., 2018, 36, 14–20 CrossRef PubMed.
- C. R. Kahn, G. Wang and K. Y. Lee, Altered adipose tissue and adipocyte function in the pathogenesis of metabolic syndrome, J. Clin. Invest., 2019, 129, 3990–4000 CrossRef PubMed.
- S. Fuloria, J. Mehta, A. Chandel, M. Sekar, N. N. I. M. Rani, M. Y. Begum, V. Subramaniyan, K. Chidambaram, L. Thangavelu, R. Nordin, Y. S. Wu, K. V. Sathasivam, P. T. Lum, D. U. Meenakshi, V. Kumarasamy, A. K. Azad and N. K. Fuloria, A Comprehensive Review on the Therapeutic Potential of Curcuma longa Linn. in Relation to its Major Active Constituent Curcumin, Front. Pharmacol., 2022, 13, 820806 CrossRef CAS PubMed.
- S. Li, W. Yuan, G.-R. Deng, P. Wang, P. Yang and B. B. Aggarwal, Chemical Composition and Product Quality Control of Turmeric (Curcuma longa L.), Pharmaceutical Crops., 2011, 2, 28–54 CrossRef CAS.
- Y. Zhou, M. Xie, Y. Song, W. Wang, H. Zhao, Y. Tian, Y. Wang, S. Bai, Y. Zhao, X. Chen and G. She, Two Traditional Chinese Medicines Curcumae Radix and Curcumae Rhizoma: An Ethnopharmacology, Phytochemistry, and Pharmacology Review, Evidence-Based Complementary Altern. Med., 2016, 2016, 4973128 Search PubMed.
- H. Leisch, K. Morley and P. C. Lau, Baeyer-Villiger monooxygenases: more than just green chemistry, Chem. Rev., 2011, 111, 4165–4222 CrossRef CAS PubMed.
- A. Majeed, K. Nagabhushanam, M. Majeed, S. M. Thomas and N. Thajuddin, An expeditious, green and protecting-group-free synthesis of a potent secondary metabolite calebin-a and its analogues, SynOpen, 2017, 1, 0125–0128 CrossRef CAS.
- A. Majeed, M. Majeed, N. Thajuddin, S. Arumugam, F. Ali, K. Beede, S. J. Adams and M. Gnanamani, Bioconversion of curcumin into calebin-A by the endophytic fungus Ovatospora brasiliensis EPE-10 MTCC 25236 associated with Curcuma caesia, AMB Express, 2019, 9, 1–13 CrossRef CAS PubMed.
- S. Goenka, K. Nagabhushanam, M. Majeed and S. R. Simon, Calebin-A, a Curcuminoid analog inhibits α-MSH-induced melanogenesis in B16F10 mouse melanoma cells, Cosmetics, 2019, 6, 51 CrossRef CAS.
- A. Brockmueller, A.-L. Mueller, A. B. Kunnumakkara, B. B. Aggarwal and M. Shakibaei, Multifunctionality of Calebin A in inflammation, chronic diseases and cancer, Front. Oncol., 2022, 12, 962066 CrossRef PubMed.
- C. S. Lai, S. N. Liao, M. L. Tsai, N. Kalyanam, M. Majeed, A. Majeed, C. T. Ho and M. H. Pan, Calebin–A inhibits adipogenesis and hepatic steatosis in high–fat diet–induced obesity via activation of AMPK signaling, Mol. Nutr. Food Res., 2015, 59, 1883–1895 CrossRef CAS PubMed.
- A. K. Tyagi, S. Prasad, M. Majeed and B. B. Aggarwal, Calebin A downregulates osteoclastogenesis through suppression of RANKL signalling, Arch. Biochem. Biophys., 2016, 593, 80–89 CrossRef CAS PubMed.
- S. Y. Park and D. S. Kim, Discovery of natural products from Curcuma longa that protect cells from beta-amyloid insult: a drug discovery effort against Alzheimer's disease, J. Nat. Prod., 2002, 65, 1227–1231 CrossRef CAS PubMed.
- C. S. Lai, S. N. Liao, M. L. Tsai, N. Kalyanam, M. Majeed, A. Majeed, C. T. Ho and M. H. Pan, Calebin-A inhibits adipogenesis and hepatic steatosis in high-fat diet-induced obesity via activation of AMPK signaling, Mol. Nutr. Food Res., 2015, 59, 1883–1895 CrossRef CAS PubMed.
- M. Majeed, K. Nagabhushanam, S. Natarajan, S. Bani, A. Pandey and S. K. Karri, Investigation of repeated dose (90 day) oral toxicity, reproductive/developmental toxicity and mutagenic potential of ‘Calebin A′, Toxicol. Rep., 2015, 2, 580–589 CrossRef CAS PubMed.
- I.-U. Haq, M. Imran, M. Nadeem, T. Tufail, T. A. Gondal and M. S. Mubarak, Piperine: A review of its biological effects, Phytother. Res., 2021, 35, 680–700 CrossRef CAS PubMed.
- D. J. McClements and H. Xiao, Excipient foods: designing food matrices that improve the oral bioavailability of pharmaceuticals and nutraceuticals, Food Funct., 2014, 5, 1320–1333 RSC.
- M. Muhammed, M. Anju, P. Anjali, P. S. Lad and K. K. Vuppala, Efficacy and Tolerability of a Novel Formulation for Weight mangement in Obese subjects : A Randomized, Double Blind, Placebo Controlled, Int. J. Ayurveda Pharma Res., 2016, 4, 10–17 CrossRef.
- H. Imano, A. Kitamura, K. Yamagishi, M. Kiyama, T. Ohira, R. Cui, M. Umesawa, T. Okada and H. Iso, Abstract P455: Insulin Resistance, Secretion and Risk of Incident Coronary Heart Disease in Non-diabetic Japanese Population: The Circulatory Risk in Communities Study, Circulation, 2014, 129, AP455–AP455 Search PubMed.
- D.-S. Cho, S. Woo, S. Kim, C. D. Byrne, J.-H. Kong and K.-C. Sung, Estimation of plasma apolipoprotein B concentration using routinely measured lipid biochemical tests in apparently healthy Asian adults, Cardiovasc. Diabetol., 2012, 11, 55 CrossRef CAS PubMed.
- J. D. Brunzell, M. Davidson, C. D. Furberg, R. B. Goldberg, B. V. Howard, J. H. Stein and J. L. Witztum, Lipoprotein Management in Patients With Cardiometabolic Risk: Consensus Conference Report From the American Diabetes Association and the American College of Cardiology Foundation, J. Am. Coll. Cardiol., 2008, 51, 1512–1524 CrossRef PubMed.
- Y.
C. Long and J. R. Zierath, AMP-activated protein kinase signaling in metabolic regulation, J. Clin. Invest., 2006, 116, 1776–1783 CrossRef CAS PubMed.
- R. A. Srivastava, S. L. Pinkosky, S. Filippov, J. C. Hanselman, C. T. Cramer and R. S. Newton, AMP-activated protein kinase: an emerging drug target to regulate imbalances in lipid and carbohydrate metabolism to treat cardio-metabolic diseases, J. Lipid Res., 2012, 53, 2490–2514 CrossRef CAS PubMed.
- P.-S. Lee, Y.-Y. Lu, K. Nagabhushanam, C.-T. Ho, H.-C. Mei and M.-H. Pan, Calebin-A prevents HFD-induced obesity in mice by promoting thermogenesis and modulating gut microbiota, J. Tradit. Complementary Med., 2022, 13(2), 119–127 CrossRef PubMed.
- B. Klop, J. W. Elte and M. C. Cabezas, Dyslipidemia in obesity: mechanisms and potential targets, Nutrients, 2013, 5, 1218–1240 CrossRef CAS PubMed.
- R. Franssen, H. Monajemi, E. S. Stroes and J. J. Kastelein, Obesity and dyslipidemia, Med. Clin. North Am., 2011, 95, 893–902 CrossRef CAS PubMed.
- H. Wang and D. Q. Peng, New insights into the mechanism of low high-density lipoprotein cholesterol in obesity, Lipids Health Dis., 2011, 10, 176 CrossRef CAS PubMed.
- M. P. Hermans, F. M. Sacks, S. A. Ahn and M. F. Rousseau, Non-HDL-cholesterol as valid surrogate to apolipoprotein B100 measurement in diabetes: Discriminant Ratio and unbiased equivalence, Cardiovasc. Diabetol., 2011, 10, 20 CrossRef CAS PubMed.
- J. E. R. v. Lennep, H. T. Westerveld, H. W. O. R. v. Lennep, A. H. Zwinderman, D. W. Erkelens and E. E. v. d. Wall, Apolipoprotein Concentrations During Treatment and Recurrent Coronary Artery Disease Events, Arterioscler., Thromb., Vasc. Biol., 2000, 20, 2408–2413 CrossRef PubMed.
- G. Walldius, I. Jungner, I. Holme, A. H. Aastveit, W. Kolar and E. Steiner, High apolipoprotein B, low apolipoprotein A-I, and improvement in the prediction of fatal myocardial infarction (AMORIS study): a prospective study, Lancet, 2001, 358, 2026–2033 CrossRef CAS PubMed.
- D. R. Matthews, J. P. Hosker, A. S. Rudenski, B. A. Naylor, D. F. Treacher and R. C. Turner, Homeostasis model assessment: insulin resistance and beta-cell function from fasting plasma glucose and insulin concentrations in man, Diabetologia, 1985, 28, 412–419 CrossRef CAS PubMed.
- T. M. Wallace, J. C. Levy and D. R. Matthews, Use and abuse of HOMA modeling, Diabetes Care, 2004, 27, 1487–1495 CrossRef PubMed.
- N. S. S. Chalichem, S. Jupudi, V. R. Yasam and D. Basavan, Dipeptidyl peptidase-IV inhibitory action of Calebin A: An in silico and in vitro analysis, J. Ayurveda Integr. Med., 2021, 12, 663–672 CrossRef CAS PubMed.
- K. G. Alberti, P. Zimmet, J. Shaw and I. D. F. E. T. F. C. Group, The metabolic syndrome–a new worldwide definition, Lancet, 2005, 366, 1059–1062 CrossRef PubMed.
- J. M. Forbes and M. E. Cooper, Mechanisms of diabetic complications, Physiol. Rev., 2013, 93, 137–188 CrossRef CAS PubMed.
- S. Bernardi, A. Marcuzzi, E. Piscianz, A. Tommasini and B. Fabris, The Complex Interplay between Lipids, Immune System and Interleukins in Cardio-Metabolic Diseases, Int. J. Mol. Sci., 2018, 19(12), 4058 CrossRef PubMed.
- P. Dandona, A. Aljada, A. Chaudhuri, P. Mohanty and R. Garg, Metabolic syndrome: a comprehensive perspective based on interactions between obesity, diabetes, and inflammation, Circulation, 2005, 111, 1448–1454 CrossRef PubMed.
- S. Devaraj, U. Singh and I. Jialal, Human C-reactive protein and the metabolic syndrome, Curr. Opin. Lipidol., 2009, 20, 182–189 CrossRef CAS PubMed.
- E. S. Ford, U. A. Ajani and A. H. Mokdad, The Metabolic Syndrome and Concentrations of C-Reactive Protein Among U.S. Youth, Diabetes Care, 2005, 28, 878–881 CrossRef PubMed.
- A. Festa, R. D'Agostino, G. Howard, L. Mykkänen, R. P. Tracy and S. M. Haffner, Chronic Subclinical Inflammation as Part of the Insulin Resistance Syndrome, Circulation, 2000, 102, 42–47 CrossRef CAS PubMed.
- B. B. Aggarwal, W. Yuan, S. Li and S. C. Gupta, Curcumin-free turmeric exhibits anti-inflammatory and anticancer activities: Identification of novel components of turmeric, Mol. Nutr. Food Res., 2013, 57, 1529–1542 CrossRef CAS PubMed.
- K. W. Lee and D. Shin, Prospective Associations of Serum Adiponectin, Leptin, and Leptin-Adiponectin Ratio with Incidence of Metabolic Syndrome: The Korean Genome and Epidemiology Study, Int. J. Environ. Res. Public Health, 2020, 17(9), 3287 CrossRef CAS PubMed.
- J. Friedman, 20 years of leptin: leptin at 20: an overview, J. Endocrinol., 2014, 223, T1–T8 CAS.
- S. C. Woods and D. A. D'Alessio, Central Control of Body Weight and Appetite, J. Clin. Endocrinol. Metab., 2008, 93, s37–s50 CrossRef CAS PubMed.
- R. B. S. Harris, Direct and indirect effects of leptin on adipocyte metabolism, Biochim. Biophys. Acta, Mol. Basis Dis., 2014, 1842, 414–423 CrossRef CAS PubMed.
- I.-C. Huang, C.-C. Chang, B.-G. Hsu, C.-J. Lee and J.-H. Wang, Association of hyperleptinemia with peripheral arterial disease in hypertensive patients, Tzu Chi Med. J., 2017, 29, 148–153 CrossRef PubMed.
- N. Ramakrishanan, T. Denna, S. Devaraj, B. Adams-Huet and I. Jialal, Exploratory lipidomics in patients with nascent Metabolic Syndrome, J. Diabetes Its Complications, 2018, 32, 791–794 CrossRef PubMed.
- A. A. Ghadge and A. A. Khaire, Leptin as a predictive marker for metabolic syndrome, Cytokine, 2019, 121, 154735 CrossRef CAS PubMed.
- T. Kadowaki, T. Yamauchi, N. Kubota, K. Hara, K. Ueki and K. Tobe, Adiponectin and adiponectin receptors in insulin resistance, diabetes, and the metabolic syndrome, J. Clin. Invest., 2006, 116, 1784–1792 CrossRef CAS PubMed.
- C. Weyer, T. Funahashi, S. Tanaka, K. Hotta, Y. Matsuzawa, R. E. Pratley and P. A. Tataranni, Hypoadiponectinemia in obesity and type 2 diabetes: close association with insulin resistance and hyperinsulinemia, J. Clin. Endocrinol. Metab., 2001, 86, 1930–1935 CrossRef CAS PubMed.
- J. A. Im, S. H. Kim, J. W. Lee, J. Y. Shim, H. R. Lee and D. C. Lee, Association between hypoadiponectinemia and cardiovascular risk factors in nonobese healthy adults, Metabolism, 2006, 55, 1546–1550 CrossRef CAS PubMed.
- R. V. Considine, M. K. Sinha, M. L. Heiman, A. Kriauciunas, T. W. Stephens, M. R. Nyce, J. P. Ohannesian, C. C. Marco, L. J. McKee and T. L. Bauer,
et al., Serum immunoreactive-leptin concentrations in normal-weight and obese humans, N. Engl. J. Med., 1996, 334, 292–295 CrossRef CAS PubMed.
- A. G. Izquierdo, A. B. Crujeiras, F. F. Casanueva and M. C. Carreira, Leptin, Obesity, and Leptin Resistance: Where Are We 25 Years Later?, Nutrients, 2019, 11(11), 2704 CrossRef CAS PubMed.
- S. Prabhu, H. Deng, T.-W. L. Cross, S. H. Shahoei, C. J. Konopka, N. Gonzalez Medina, C. C. Applegate, M. A. Wallig, L. W. Dobrucki, E. R. Nelson, A. M. Smith and K. S. Swanson, Nanocarriers targeting adipose macrophages increase glucocorticoid anti-inflammatory potency to ameliorate metabolic dysfunction, Biomater. Sci., 2021, 9, 506–518 RSC.
- Y. Li, S. Li, Y. Han, J. Liu, J. Zhang, F. Li, Y. Wang, X. Liu and L. Yao, Calebin-A induces apoptosis and modulates MAPK family activity in drug resistant human gastric cancer cells, Eur. J. Pharmacol., 2008, 591, 252–258 CrossRef CAS PubMed.
- S. Foshati, F. Nouripour, E. Sadeghi and R. Amani, The effect of grape (Vitis vinifera) seed extract supplementation on flow-mediated
dilation, blood pressure, and heart rate: A systematic review and meta-analysis of controlled trials with duration- and dose-response analysis, Pharmacol. Res., 2022, 175, 105905 CrossRef PubMed.
- S. Foshati, M. H. Rouhani and R. Amani, The effect of grape seed extract supplementation on oxidative stress and inflammation: A systematic review and meta-analysis of controlled trials, Int. J. Clin. Pract., 2021, 75, e14469 CAS.
- Y. Panahi, N. Khalili, E. Sahebi, S. Namazi, M. S. Karimian, M. Majeed and A. Sahebkar, Antioxidant effects of curcuminoids in patients with type 2 diabetes mellitus: a randomized controlled trial, Inflammopharmacology, 2017, 25, 25–31 CrossRef CAS PubMed.
Footnote |
† Electronic supplementary information (ESI) available: Methods: Sample size calculations and details of anthropometric measurements. Table 1: Liver and kidney function tests. Table 2: Hematological parameters for active and placebo groups. Table 3: Urine analysis for active and placebo groups. See DOI: https://doi.org/10.1039/d2fo03627d |
|
This journal is © The Royal Society of Chemistry 2023 |
Click here to see how this site uses Cookies. View our privacy policy here.