DOI:
10.1039/D2FO03587A
(Paper)
Food Funct., 2023,
14, 5429-5441
Vacuum impregnation as a sustainable technology to obtain iron-fortified broad bean (Vicia faba) flours
Received
21st November 2022
, Accepted 18th April 2023
First published on 24th May 2023
Abstract
Iron-fortified broad bean flours were obtained by vacuum impregnation during soaking. The impact of vacuum impregnation and iron fortification on the hydration kinetics of broad beans, as well as the processing (soaking, autoclaving, and dehulling) on the iron-absorption inhibitors (phytic acid and tannins), iron content, iron bioaccessibility, and physicochemical and techno-functional properties of flours was investigated. Results showed that the use of vacuum impregnation during soaking reduced the broad beans’ soaking time by 77%, and using iron solution instead of water did not affect the hydration kinetics. After soaking, iron-fortified broad bean flours increased twice (without hull) or more (with hull) the iron and bioaccessible iron content regarding non-fortified flours. Cooking broad beans by autoclaving modified the tannin content, the iron content and its bioaccessible fraction, and the physicochemical and techno-functional properties of the flours. Autoclaving increased the water holding capacity and absorption rate, swelling capacity, bulk density, and particle size, while decreased the solubility index, whiteness index, emulsifying capacity, emulsion stability, and gelling capacity. Finally, dehulling did not practically affect the physicochemical and techno-functional properties of flours, but showed a decrease in iron content, although increased iron bioaccessibility was observed, occurred mainly due to the reduction in tannin concentrations. The results obtained in this study demonstrated that vacuum impregnation is a useful technology for obtaining iron-fortified broad bean flours with different physicochemical and techno-functional properties depending on the production process used.
1. Introduction
The broad bean is a nutrient-rich legume since it is high in protein, complex carbohydrates, and dietary fibre. It also contains antinutritional factors such as lectins, phytic acid, tannins, vicine, and convicine, which reduce its biological value,1 although these compounds can be reduced with simple treatments or bioprocessing techniques like dehulling, soaking, cooking, extrusion, germination, or fermentation.2
Dry broad beans can be consumed in different ways, either whole after soaking and cooking treatments or as flour after a milling process.3 The flour offers a versatile and highly nutritious source of protein and can be easily added to many food products such as pasta, crackers, mayonnaise, sausages, and meat analogues.4,5 To produce flour, the seeds can be directly milled or pretreated, for example by soaking or cooking, to improve their nutritional, physicochemical, and organoleptic properties.3 Soaking is one of the most common pretreatments because it shortens the cooking time and reduces the content of antinutritional factors through activation of endogenous enzymes or leaching into the soaking medium.1,3 Likewise, cooking is also widely used because inactivates thermolabile antinutritional factors, increases protein and starch digestibility, reduces the beany flavour, and changes the physicochemical and techno-functional properties of flours.3
Soaking is a time-consuming part of the processing, so it would be interesting to shorten it by using technologies such as ultrasounds, high hydrostatic pressures or vacuum impregnation.6,7 This latest technology is based on depressurisation and, later, the reestablishment of the atmospheric pressure of a solid product–solution system. During depressurisation, the air inside the product is expelled through the pores. When the atmospheric pressure is reestablished, the solution flows into the pores as a result of the pressure gradient generated, accelerating the entry of the solution into the product.8
Even though the soaking is shortened, it takes up a significant amount of time that can be used to incorporate water-soluble compounds and fortify legumes.9 In this sense, iron is an interesting compound as iron deficiency is one of the main causes of micronutrient malnutrition worldwide.10,11 Nevertheless, adding iron to legumes may not have the expected effect because they contain iron-absorption inhibitors such as phytic acid and tannins that could chelate it and form insoluble complexes. In these cases, it is advisable to add already chelated iron, such as NaFeEDTA, which has been shown to have two to three times greater absorption level than ferrous sulphate.11,12
Iron fortification of staple food such as cereals, legumes, flours, and bread made from these flours would be an effective way to treat or prevent iron deficiency.10 Furthermore, foods of high nutritional value, such as legumes and their flours, could help to prevent protein-energy malnutrition and to ensure an optimal uptake of essential micronutrients.13,14
Thus, the main purpose of this study was to obtain iron-fortified broad bean flours using vacuum impregnation during soaking. The specific objectives were (a) to evaluate the effect of vacuum impregnation and iron fortification on the hydration kinetics of broad beans, and (b) to study the impact of processing on the iron-absorption inhibitors, iron content, iron bioaccessibility, and the physicochemical and techno-functional properties of the flours.
2. Materials and methods
2.1 Samples and reagents
Broad beans (Vicia faba), purchased from a supermarket (Madrid, Spain), were manually cleaned to remove damaged seeds and foreign materials, and stored in plastic bags until use.
Enzymes α-amylase (A3171) and pancreatin (P7545) from porcine pancreas and pepsin from porcine gastric mucosa (P7012), bovine bile extract (B3883), ethylenediaminetetraacetic acid iron(III) sodium salt hydrate (NaFeEDTA), hydroxylamine monohydrochloride, 1,10-phenanthroline, 5-sulfosalicylic acid dihydrate, tannic acid, and phytic acid sodium salt hydrate were purchased from Sigma-Aldrich, Co. (St Louis, MO, USA). Trichloroacetic acid (TCA) and sodium acetate trihydrate were purchased from Labbox Labware S.L. (Premià de Dalt, Barcelona, Spain). Folin–Ciocalteu's reagent was purchased from Panreac Química S.L.U. (Castellar del Vallès, Barcelona, Spain) and ferric chloride was purchased from MP Biomedicals (Illkirch, France). All other reagents and chemicals were of analytical grade.
2.2 Broad bean hydration kinetics during soaking
The determination of the hydration rate of the broad beans during soaking was carried out at atmospheric pressure (atmospheric soaking, AS) and using vacuum impregnation during the first 5 min of soaking and re-establishing the atmospheric pressure after that time (vacuum soaking, VS). In both cases, broad beans were soaked in a ratio of 1
:
3 (w/v) with deionised water at room temperature (22 ± 2 °C) and were periodically removed, superficially dried with paper and weighed. For AS, the beans were immersed in deionised water for 16 h and the water gain was measured for the initial 6 h (at 1 h interval) and up to 10 h (at 2 h interval). Likewise, for VS, the beans were soaked for 3 h and the water gain was measured for the first hour (at 10 min interval), for the second hour (at 30 min interval), and finally at 3 h. The absorbed water was calculated using eqn (1). | 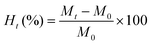 | (1) |
where Ht is the water absorbed at time t, Mt is the weight of the sample at time t, and M0 is the initial weight of the sample.
To study if the iron solution affected the hydration kinetics, the VS was repeated but replacing the soaking water with an iron solution, which contained 0.5 mg NaFeEDTA per mL. The selected concentration was 0.5 mg NaFeEDTA per mL since the EFSA recommends not exceeding the amount of 1.9 mg EDTA per kg b.w. per day, which is established as safe, and the maximum amount of NaFeEDTA allowed in foodstuff is not legislated in the European Union.15 All soaking tests were duplicated, and independent samples (50 g of dry broad beans) were prepared each time.
The Peleg's equation was used to model the hydration kinetics of broad beans because it is one of the most common equations used to model the hydration or rehydration of different food products.6,16 However, the water gained by the beans instead of the moisture content was modelled as shown in the eqn (2):8
| Ht = H0 + t/(k1 + k2t) | (2) |
where
Ht is the water absorbed at time
t (g H
2O per g sample);
H0 is the water absorbed at
t = 0 min (considered 0 g H
2O per g sample);
t is the soaking time;
k1 is the Peleg constant 1 (min g sample per g H
2O), which is inversely related to the initial water absorption rate;
k2 is the Peleg constant 2 (g sample per g H
2O), which is inversely associated with the maximum water absorption capacity.
2.3 Broad bean flours processing
Fig. 1 shows the processing of the broad bean flours. Broad beans were vacuum soaked (1
:
3, w/v) with water or with the iron solution (0.5 mg NaFeEDTA per mL) until reached 100% hydration. After draining the soaking medium, half of the water-soaked beans and half of the iron-soaked beans were cooked by steaming using an autoclave for 5 min at 121 °C. To obtain the flours, the soaked and soaked–autoclaved broad beans were dried in a convection dryer at 50 °C until constant weight, and then milled using a ball mill (Mixer Mill MM400, Retsch GmbH, Haan, Germany). Flours were processed with and without hull. A total of 8 types of flour, 4 non-fortified flours (soaked broad bean flour, BS; soaked broad bean flour with hull, BS-H; soaked–autoclaved broad bean flour, BSA; soaked–autoclaved broad bean flour with hull, BSA-H) and 4 fortified flours (iron-soaked broad bean flour, BSFe; iron-soaked broad bean flour with hull, BSFe-H; iron-soaked–autoclaved broad bean flour, BSFeA; iron-soaked–autoclaved broad beans flour with hull, BSFeA-H) were obtained. Two batches per flour were processed.
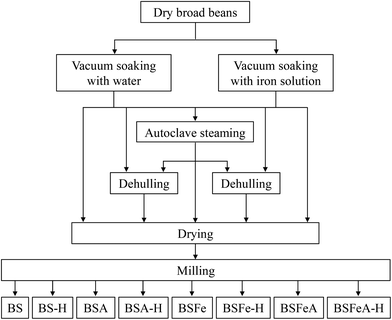 |
| Fig. 1 Broad bean flours processing. BS, soaked broad bean flour; BS-H, soaked broad bean flour with hull; BSA, soaked–autoclaved broad bean flour; BSA-H, soaked–autoclaved broad bean flour with hull; BSFe, iron-soaked broad bean flour; BSFe-H, iron-soaked broad bean flour with hull; BSFeA, iron-soaked–autoclaved broad bean flour; BSFeA-H, iron-soaked–autoclaved broad bean flour with hull. | |
2.4 Phytic acid and tannin determination of broad bean flours
The phytic acid content was determined following the method described by Embaby17 with some modifications. For that, 5 mL of HCl (2.4%) were added to 0.1 g of broad bean flours in tubes of 15 mL, and incubated with agitation for 1 h at room T for phytic acid extraction. Then, the samples were centrifugated at 6000g, 4 °C for 10 min and 0.75 mL of the supernatants were mixed with 0.25 mL of wade reagent (0.03% solution of FeCl3·6H2O containing 0.3% sulfosalicylic acid in water). The mixture was centrifuged at 7000g for 10 min and the absorbance of the collected supernatant was measured at 500 nm using a UV-Visible spectrophotometer (Helios Zeta, Thermo Scientific, UK). Results were expressed as mg phytic acid per g flour.
The determination of tannins was performed according to the method standardised by the AOAC.18 So, 0.2 g of flour were mixed with 70 mL of acetone 70% and incubated with agitation for 24 h at room T for tannins extraction. Samples were then centrifuged at 8000g for 10 min, and 0.1 mL of these supernatants were mixed with 0.2 mL of Na2CO3 20%, 1.6 mL of distilled water and 0.1 mL of Folin–Ciocalteu reagent. After 30 min of incubation, the absorbance was measured at 760 nm. Results were expressed as mg tannic acid per g flour.
For the extractions of both compounds, an Intelli-Mixer™ RM-2 (ELMI Ltd, Riga, Latvia) at 30 rpm was used to agitate the samples. The extractions were performed in duplicate and the determinations were done in triplicate.
2.5 Iron determination of broad bean flours
The iron content was determined spectrophotometrically according to the method of Kosse et al.19 slightly modified by Zunin et al.20 Kosse et al.19 proved that the total Fe2+ determination provides an accurate measure of iron content in most fortified and unfortified foods.
The iron-extracting solution was prepared by dissolving 10 g of hydroxylamine monohydrochloride in 40 mL of water and adding 20 mL of concentrated HCl and 20 g of TCA to the solution, which was finally brought to 200 mL with water. Note that Fe3+ is reduced to Fe2+ with an excess of hydroxylamine monohydrochloride. To prepare the chromogen solution, 36 mg of 1,10-phenanthroline were added to 10 mL of water and heated to dissolve it. After cooling, the solution was brought to 100 mL with 3 M sodium acetate and cold stored in the dark until use. To obtain the standard curve, iron standard solutions of 0, 5, 10, 20, 25 and 50 μg Fe per mL were prepared from an iron stock solution of 0.5 mg Fe per mL using NaFeEDTA.
In order to extract iron from samples, 0.5 g of flour and 5 mL of iron-extracting solution were added to glass tubes and mixed. The tubes were put in a boiling water bath for 15 min. After incubation, the tubes were cooled and centrifuged at 8228g, 8 °C for 10 min, and the supernatant was filtered.
Finally, for iron determination, 0.5 mL of filtrated sample or standard solution was mixed with 1.5 mL of chromogen solution and incubated at room temperature in the dark for 15 min. The absorbance was measured at 510 nm against a blank containing 0.5 mL iron-extracting solution and 1.5 mL 3 M sodium acetate. A sample blank was also prepared for each sample by adding 0.5 mL filtrated sample to 1.5 mL 3 M sodium acetate. The absorbance obtained was subtracted from the absorbance obtained for the corresponding sample. Iron extraction and determination was done in duplicate, and the results were expressed as mg Fe per 100 g flour.
2.6 Iron bioaccessibility of broad bean flours
In order to determine the bioaccessible iron content of broad bean flours, a simulation of gastrointestinal digestion (GID) was performed following the INFOGEST protocol,21,22 and the soluble iron content in the supernatant digest was determined by inductively coupled plasma mass spectrometry (ICP-MS) using an Agilent 7900 ICP-MS (Agilent Technologies, Inc. Headquarters, Santa Clara, California, US).
The GID was performed in duplicate at 37 °C under constant gentle mixing using an Intelli-Mixer™ RM-2 and an incubator chamber Selecta (JP Selecta, S.A., Barcelona, Spain). Simulated salivary fluid (SSF), simulated gastric fluid (SGF), and simulated intestinal fluid (SIF) were prepared.21 For the oral phase, 0.5 g of flour was reconstituted with 0.5 g of water, and the paste was diluted 1
:
1 (w/v) with SSF containing α-amylase enzyme (75 U mL−1). After 2 min, the phase was stopped by decreasing the pH to 3 with 2 M HCl, and the gastric phase was started by diluting the oral bolus 1
:
1 (v/v) with SGF containing pepsin (2000 U mL−1). After 120 min, the gastric phase was stopped by increasing the pH to 7 with 1 M NaOH. Lastly, for the intestinal phase, the gastric chyme was diluted 1
:
1 (v/v) with SIF containing pancreatin (based on trypsin activity to achieve 100 U mL−1) and bovine bile extract (10 mM). The digestion was stopped after 120 min by heating (100 °C, 5 min), and the samples were cooled in an ice bath and centrifugated at 8228g, 4 °C for 10 min. The supernatants were lyophilised, and the total iron was determined by ICP-MS after digestion in a microwave oven because the spectrophotometric method presented interferences probably resulting from the compounds added during GID. To obtain blank samples of the digestion, 0.5 g of flour was replaced by 0.5 g of water.
2.7 Physicochemical properties of broad bean flours
2.7.1 Colour.
A Minolta CM-700d spectrophotometer (Konica Minolta Sensing, Inc., Osaka, Japan) was used to measure, in triplicate, the colour of the different broad bean flours. CIELAB colour coordinates L*, a*, and b* were obtained using the standard light source D65 and standard observer 10°. The whiteness index (WI) was calculated using eqn (3).23 |  | (3) |
2.7.2 Particle size distribution.
The particle size distribution of broad bean flours was measured using a Mastersizer 2000 laser scattering (Malvern Instruments, Malvern, UK) equipped with a dry powder unit Scirocco.24 The measurement conditions were an absorption index of 0.1 and a refractive index of 1.52. Six replicates of each sample were analysed. Results were expressed as the maximum particle diameter below 50% of sample is contained (d(0.5)) and equivalent spherical diameter (D[4,3]).
2.7.3 Bulk density.
The bulk density (BD) of broad bean flours was determined according to Du et al.25 The flour sample was transferred into a 10 mL graduated cylinder that was previously tared. The cylinder was gently tapped on a flat surface several times until the sample level was not diminished after filling to the 10 mL mark. BD was measured in triplicate and calculated as the weight of the sample (g) per volume (mL).
2.7.4 Water absorption index, water solubility index, and swelling power.
The water absorption index (WAI), water solubility index (WSI), and swelling power (SP) were determined following the methods of Du et al.25 and Cornejo and Rosell26 with some modifications. Briefly, 0.83 g of bean flour was mixed with 10 mL of deionised water and cooked in a water bath at 60 °C for 15 min. The cooked flour was cooled at room temperature and centrifuged at 3000g, 4 °C for 10 min. The supernatant was transferred into a pre-weighed recipient, lyophilised, and weighed to determine the solid content, and the weight of the sediment was taken. WAI, WSI, and SP were measured in triplicate and calculated using eqn (4)–(6). | 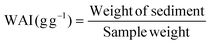 | (4) |
|  | (5) |
|  | (6) |
2.8 Techno-functional properties of broad bean flours
2.8.1 Water-holding capacity and oil-binding capacity.
Water-holding capacity (WHC) and oil-binding capacity (OBC) were determined according to Setia et al.27 in triplicate. To measure the WHC, 1 g of flour was suspended in 5 mL of deionised water in a centrifuge tube and kept vertically for 20 min, with agitation for 10 s at time intervals of 5 min. After the 20 min, the suspension was centrifuged at 1000g, 22 °C for 15 min and the supernatant was carefully decanted. The weight of the sediment was recorded, and WHC was expressed as the weight of retained water over the dry weight of flour (g water per g sample).
The procedure to determine OBC was very similar to that of WHC, but 1 g of flour was suspended in 10 mL of sunflower oil and kept for 30 min. After agitation and centrifugation, the sediment was weighted and OBC was expressed as the weight of bound oil over the dry weight of flour (g oil per g sample).
2.8.2 Emulsifying activity and emulsion stability.
The emulsifying activity (EA) and emulsion stability (ES) of broad bean flours were determined in duplicated using the method described by Stone et al.28 with slight modifications. Briefly, 1.75 g of flour was dispersed in 25 mL of deionised water. The pH was then adjusted to 7 using 0.1 M HCl/NaOH and left overnight stirring. The following day, the pH was readjusted to 7, and 25 mL of sunflower oil was added to the flour suspension. The mixture was homogenised for 1 min at level 4 speed using an Ultra-Turrax, and then divided into four centrifuge tubes. Two tubes were centrifugated at 1300g for 5 min to measure the EA, and another two tubes were heated to 80 °C in a water bath for 30 min, cooled to room temperature, and centrifuged at 1300g for 5 min to determine the ES. EA and ES were expressed in percentages and calculated as shown in eqn (7) and (8). |  | (7) |
|  | (8) |
2.8.3 Gelation capacity.
The gelation capacity of flours was determined in duplicate according to Sosa et al.29 Suspensions between 2–20% (w/v) were prepared, and 5 mL of each suspension was put in a test tube and heated for 1 h in a boiling water bath. After the incubation, the tubes were tempered with running water and cooled at 4 °C for 2 h. The least gelation concentration (LGC) was taken when the sample did not fall or slip when the tube was inverted.
2.9 Statistical analysis
One-way analysis of variance (ANOVA) with Tukey's honest significance tests was carry out to evaluate the differences in means of phytic acid, tannins, and iron content, and the physicochemical and techno-functional properties studied, while Pearson product moment correlations were carried out to measure the strength and direction of the linear relationship between the physicochemical and techno-functional properties of the broad bean flours. Both statistical analyses were performed using Statgraphics Centurion XVII software (Statgraphics Technologies, Inc., The Plains, VA, USA). Results were expressed as the mean of replicates ± standard deviations, and differences were considered significant at p < 0.05.
3. Results and discussion
3.1 Effect of vacuum impregnation on broad bean hydration kinetics
Water absorption curves of dry broad beans during AS and VS are shown in Fig. 2A. Water absorption increased with soaking time until reaching the equilibrium moisture, but the behaviour of the hydration kinetics was different depending on the type of soaking (AS or VS). The hydration kinetics of atmospheric-soaked broad beans had a sigmoidal shape probably because the hull is impermeable to water and water would only enter through the hilum. Nevertheless, once the hull is hydrated from the inside, it becomes permeable to water and the moisture content steadily increases until the equilibrium moisture is reached.6 Conversely, vacuum-soaked broad beans had a downward concave shape and a faster water imbibition in the initial phase than atmospheric-soaked broad beans. So, during the first hour of soaking, vacuum-soaked broad beans absorbed 85.7% water, while atmospheric-soaked beans reached only 15.5%. The rapid initial water absorption of vacuum-soaked broad beans may be due to the internal structure of the seeds and the hydrodynamic mechanism generated by the vacuum impregnation. At the initial stages of the hydration, the water absorption is mainly through the hilum to fill the spaces between the hull and the cotyledon, and later the gap between the two cotyledons.6,30 Broad beans are one of the legumes with higher porosity, approximately 10.9 ± 2.5%,31 probably because it is one of the largest legumes and the spaces between the hull and the cotyledon and the two cotyledons are bigger. The air occluded in these spaces and pores is pressurised with the advance of the wetting front, which could exert resistance to hydration. Therefore, when vacuum impregnation is applied during soaking, the air occluded is expelled, and when the pressure is restored, the water flows inwards accelerating the legume hydration7 and the permeation of the hull. Therefore, as shown in Fig. 2A, broad beans with AS required 840 min to reach the equilibrium moisture whereas broad beans with VS required only 90 min, significantly shortening the soaking time. In this regard, few studies have researched the effect of vacuum impregnation or vacuum pressure soaking on the legumes’ hydration kinetics. Xiao et al.32 studied the impact of vacuum soaking in soybeans after 8 and 16 h of soaking, obtaining a significant increase in the water absorption of vacuum-soaked soybeans with regard to atmospheric pressure-soaked soybeans. Similarly, Zanella-Díaz et al.8 studied the shortening of the soaking time of tepary and pinto beans by applying pressure gradients at room temperature, obtaining the best results at high-pressure gradients. Li et al.33 and Tian et al.34 used vacuum soaking to hydrate rice, and both studies observed an increase in the water absorption rate of vacuum-soaked rice during the first hour of soaking compared to rice soaked at atmospheric pressure.
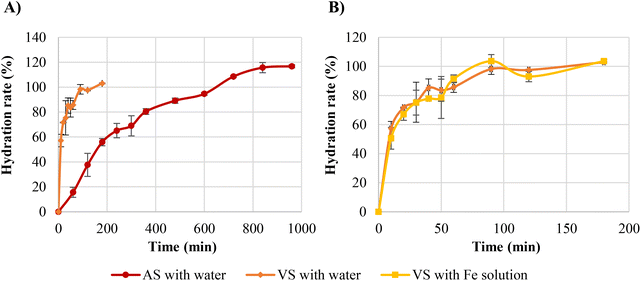 |
| Fig. 2 Hydration curves of dry broad beans during soaking. (A) Water absorption of broad beans during atmospheric pressure soaking (AS) and vacuum pressure soaking (VS). (B) Water or Fe solution absorption of broad beans during VS. | |
The experimental hydration curves were modelled with Peleg's equation for predicting the water absorption rate at any soaking time. Eqn (9) and (10) were obtained, which describe, respectively, the hydration level (Ht) of broad beans with AS and broad beans with VS as a function of the soaking time.
| 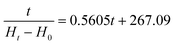 | (9) |
| 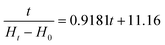 | (10) |
As seen in the equations, both constants were affected by the vacuum impregnation, but k1 to a greater extent. This constant is inversely related to the initial water absorption rate, which fits with the experimental results obtained. The broad beans soaked at atmospheric pressure, with a k1 value of 267.09, had a low initial water absorption rate. In contrast, the broad beans soaked at vacuum pressure, with a k1 value of 11.16, had a high initial water absorption rate (Fig. 2A). The k2 values obtained also agree with the experimental data. Broad beans soaked at atmospheric pressure showed a k2 value slightly lower than broad beans soaked at vacuum pressure. This means that atmospheric-soaked broad beans had a higher maximum water absorption capacity than those vacuum-soaked broad beans, as shown in Fig. 2A. In other food matrices, the maximum water absorption capacity did not change or increase after vacuum impregnation.33,35
Using the equations obtained after modelling (eqn (9) and (10)), atmospheric-soaked broad beans required 608 min to reach 100% water hydration rate, whereas vacuum-soaked broad beans required only 136 min.
3.2 Effect of iron fortification on broad bean hydration kinetics
To obtain iron-fortified broad beans, the water used during soaking was replaced by an iron solution, and it was tested whether the change of the soaking medium affected the hydration kinetics. The iron salt used to prepare the iron solution was NaFeEDTA because it is water-soluble and has two to three times better absorption than ferrous sulphate in food such as legumes.12 Furthermore, it is stable during processing and storage and causes few organoleptic problems.36
Fig. 2B compares the hydration curves of broad beans using water and Fe solution during vacuum soaking. According to the results, the iron solution did not alter the hydration kinetics since there were no significant differences between the % absorption of water and iron solution at any soaking time. Similar results were obtained by Miano and Augusto9 when comparing the hydration kinetics of carioca kidney beans soaked with water and soaked with ferrous sulphate solution. Ferrous sulphate solution did not alter the hydration kinetic at neither of the two concentrations tested (0.271% and 0.542%, w/v), probably due to these concentrations did not modify variables that might affect the hydration kinetics, such as the pH and ionic strength of the medium.9
3.3 Effect of processing on the phytic acid and tannin content of broad bean flours
Phytic acid and tannins were determined due to they are able to chelate and form insoluble complexes with iron, affecting its bioaccessibility. The results obtained are shown in Fig. 3. The phytic acid content was around 18.2 and 20.2 mg of phytic acid per g of flour, so it was not greatly affected by processing conditions neither with hull or without hull flours (indicated by capital letters in Fig. 3A). Nevertheless, statistically significant differences were observed between flours with hull and without hull within the same treated flour (indicated by lowercase letters in Fig. 3A). Dehulling significantly increased the phytic acid content which could be due to this compound is mainly located in the cotyledon.37,38
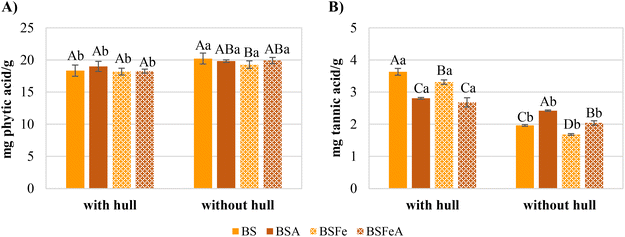 |
| Fig. 3 (A) Phytic acid content and (B) tannin content of broad bean flours. BS, soaked broad bean flour; BSA, soaked–autoclaved broad bean flour; BSFe, iron-soaked broad bean flour; BSFeA, iron-soaked–autoclaved broad bean flour. Capital letters indicate significant differences between treated flours within flours with hull or without hull, whereas lowercase letters indicate significant differences between flours with hull or without hull within the same treated flour. | |
Regarding the tannin content, the results are presented in the Fig. 3B. Dehulling also had the greatest impact on the tannin content. The flours with hull presented between 2.68 and 3.63 mg of tannic acid per g of flour, whereas the flours without hull showed between 1.68 and 2.42 mg g−1. The decrease observed with dehulling is due to tannins are mainly located in the hull.37,38 Moreover, it was observed that after autoclaving, the tannin content of flours with hull decreased whereas the content of flours without hull increased. These results could be due to hull's tannins, during autoclaving, diffused into the condensed steam around the beans and to the cotyledon causing an increase in tannin content when the hull was removed.
3.4 Effect of processing on the iron content and iron bioaccessibility of broad bean flours
The iron content of the different types of broad bean flours (BS, BSA, BSFe, BSFeA with hull and without hull) was determined to study how the production process of the flour affected the iron content, and results are shown in Fig. 4A. In all cases except in the iron-soaked–autoclaved flours, the iron content of the flours decreased when the hull was removed, which would mean that a significant part of the iron, both naturally present and added, was found in the hull. Similar results were obtained by Luo et al.,39 which showed that the total iron content of hulls (5.26 mg per 100 g d.m.) was significantly higher than that of dehulled broad beans (2.38 mg per 100 g d.m.). In the case of iron-soaked–autoclaved flours, it was observed that the iron content of BSFeA flour was significantly higher than that of BSFeA-H but lower than the content of BSFe-H. This increase after dehulling could be because, as the autoclaving was carried out on broad beans with hull, part of the added iron in the hull diffuses towards the cotyledon.
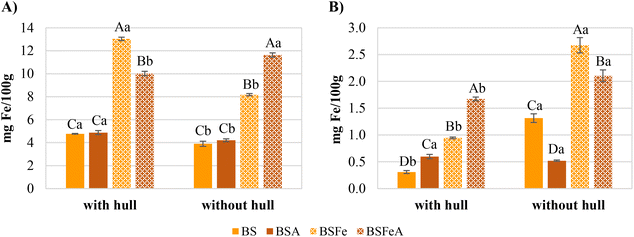 |
| Fig. 4 Iron content of broad bean flours (A) before gastrointestinal digestion and (B) after gastrointestinal digestion (bioaccessible iron). BS, soaked broad bean flour; BSA, soaked–autoclaved broad bean flour; BSFe, iron-soaked broad bean flour; BSFeA, iron-soaked–autoclaved broad bean flour. Capital letters indicate significant differences between treated flours within flours with hull or without hull, whereas lowercase letters indicate significant differences between flours with hull or without hull within the same treated flour. | |
Regarding how the soaking and cooking could affect the iron content of broad bean flours (Fig. 4A), the content of iron increased approximately twice in samples without hull (BSFe) and almost three times in those with hull (BSFe-H) after soaking with iron solution. Nevertheless, the results after soaking and autoclaving were different in presence and absence of hull. The iron content of BSFeA-H flour decreased after autoclaving, probably because an amount of the iron contained in the hull was lost through the steam condensed on the beans. However, the iron content of BSFeA flour increased because, as mentioned above, some of the iron contained in the hull could diffuse into the cotyledon. Even so, both fortified flours after autoclaving (BSFeA-H and BSFeA) had higher iron content than their controls (BSA-H and BSA), in particular, BSFeA-H twice as much as BSA-H and BSFeA almost three times more than BSA. As regards non-fortified flours, the cooking by steam-autoclaving did not affect the iron content of broad bean flours neither with the hull (BS-H, BSA-H) nor without the hull (BS, BSA).
Nevertheless, not all the iron in broad bean flours is usually available to be absorbed because it could form insoluble complexes with tannins and phytates.10,40 Therefore, the soluble iron after GID was determined as it is a good indicator of bioaccessibility, which is defined as the fraction of a compound that is released from its food matrix in the gastrointestinal tract and thus becomes available for intestinal absorption.12,41 The results of bioaccessible iron in broad bean flours are shown in Fig. 4B. As expected, the iron concentration in the different flours after GID was much lower than that before GID (Fig. 4). However, fortified flours maintained higher iron content than non-fortified flours, specifically, BSFe-H presented three times more bioaccessible iron than BS-H, BSFeA-H almost three times more than BSA-H, BSFe two times more than BS, and BSFeA four times more than BSA. Moreover, dehulling significantly impacted on the bioaccessible iron content. Each flour without hull had a higher bioaccessible iron content than the flour with hull, except for soaked–autoclaved broad bean flours (BSA, BSA-H) in which no significant differences were observed. The reduction of bioaccessible iron in the flours with hull could be due to the large proportion of tannins present in the hull of broad beans (Fig. 3B), which could act as chelating agents of iron and form stable complexes reducing iron bioaccessibility.42,43 Krishnan et al.44 studied the influence of dehulling on the iron bioaccessibility of finger millet, obtaining that the bioaccessible iron increased in samples without hull. However, Gwala et al.45 observed that dehulling did not reduce or improve the iron bioaccessibility of Bambara groundnuts. In addition, the effect of autoclaving depended on the presence of the hull. The soaked–autoclaved flours with hull had higher bioaccessible iron content than their corresponding soaked flours. Nevertheless, the soaked–autoclaved flours without hull presented lower bioaccessible iron content than the soaked flours. These results were in concordance with those of tannin content (Fig. 3B) because flours with higher tannin content had lower bioaccessible iron content. Hemalatha et al.46 obtained the same results when they studied the iron bioaccessibility of raw and pressure-cooked chickpeas with and without hulls. Nevertheless, they showed an increased iron bioaccessibility after pressure cooking the green gram in both with and without hull.
3.5 Effect of processing on the physicochemical and techno-functional properties of broad bean flours
The physicochemical properties of the different treated broad bean flours, including colour through the whiteness index (WI), particle size distribution expressed as d(0.5) and D[4,3], bulk density (BD), water absorption index (WAI), water solubility index (WSI) and swelling power (SP), were determined and are presented in Table 1. Generally, all physicochemical properties have been affected by the thermal treatment but not by iron fortification or dehulling.
Table 1 Physico-chemical properties of broad bean flours
Flour |
WI |
d(0.5) (μm) |
D[4,3] (μm) |
BD (g mL−1) |
WAI (g g−1) |
WSI (g per 100 g) |
SP (g g−1) |
Physicochemical properties are: WI, whiteness index; d(0.5), maximum particle diameter below 50% of sample is contained; D[4,3], equivalent spherical diameter; BD, bulk density; WAI, water absorption index; WSI, water solubility index; SP, swelling power. Flours are: BS, soaked broad bean flour; BS-H, soaked broad bean flour with hull; BSA, soaked–autoclaved broad bean flour; BSA-H, soaked–autoclaved broad bean flour with hull; BSFe, iron-soaked broad bean flour; BSFe-H, iron-soaked broad bean flour with hull; BSFeA, iron-soaked–autoclaved broad bean flour; BSFeA-H, iron-soaked–autoclaved broad bean flour with hull. Different letters in each column indicate significant differences between the types of flour. |
BS |
86.0 ± 0.1b |
54.9 ± 1.7e |
106.1 ± 1.8d |
0.763 ± 0.007b |
2.21 ± 0.04d |
27.4 ± 0.3a |
3.05 ± 0.05c |
BSFe |
85.9 ± 0.2b |
64.0 ± 2.3d |
129.9 ± 2.9c |
0.791 ± 0.024b |
2.20 ± 0.02d |
27.7 ± 0.1a |
3.05 ± 0.03c |
BSA |
73.5 ± 0.2f |
164.9 ± 8.1a |
211.9 ± 6.0a |
0.955 ± 0.008a |
3.85 ± 0.03a |
13.7 ± 0.1e |
4.46 ± 0.03a |
BSFeA |
75.1 ± 0.3d |
134.3 ± 2.3c |
178.8 ± 2.7b |
0.944 ± 0.003a |
3.74 ± 0.01b |
14.1 ± 0.2de |
4.36 ± 0.01a |
BS-H |
84.6 ± 0.2c |
44.7 ± 3.3f |
132.1 ± 6.4c |
0.786 ± 0.005b |
2.59 ± 0.01c |
25.0 ± 0.3b |
3.45 ± 0.01b |
BSFe-H |
86.9 ± 0.2a |
45.4 ± 5.2f |
171.5 ± 24.9b |
0.776 ± 0.014b |
2.64 ± 0.03c |
24.0 ± 0.5c |
3.48 ± 0.02b |
BSA-H |
70.2 ± 0.3g |
155.7 ± 6.9b |
212.7 ± 4.0a |
0.930 ± 0.003a |
3.80 ± 0.04ab |
14.1 ± 0.2de |
4.42 ± 0.04a |
BSFeA-H |
74.4 ± 0.3e |
127.2 ± 4.8c |
180.3 ± 4.4b |
0.952 ± 0.012a |
3.77 ± 0.04b |
14.7 ± 0.2d |
4.42 ± 0.06a |
The WI of broad bean flours varied between 70.2 and 86.9. Although statistically significant differences were observed between practically all samples, two groups of samples could be differentiated. So, the WI of soaked–autoclaved flours were lower than those of soaked flours whether or not flours had added iron and/or hull. The darkening of soaked–autoclaved flours could be due to the compounds derived from Maillard reactions generated during the thermal treatment.23
The particle size distributions of broad bean flours are represented in Fig. 5 and the results obtained of d(0.5) and D[4,3] are shown in Table 1. Soaked–autoclaved flours had a bimodal distribution with a small first peak in the region of 91 to 120 μm and a defined second peak in the region of 316 to 363 μm. Soaked flours presented a trimodal distribution with a defined first peak around 3–4 μm, a defined second peak around 23–26 μm, and a broader and less defined third peak in the region of 240 to 417 μm. Taking into account the values of d(0.5) and D[4,3], the flours with larger particle sizes were BSA and BSA-H, followed by BSFeA and BSFeA-H, BSFe and BSFe-H, and BS and BS-H, the latter being the smallest in particle size. Jiang et al.24 reported the particle size distributions of conventional thermal treated and microwave treated broad bean flours, showing a bimodal distribution and d(0.5) values in the range of 430–530 μm. These differences between results would be due to the particle size distribution of flours depends not only on the type of legume and the treatment carried out, but also on the type of milling and the conditions used.47
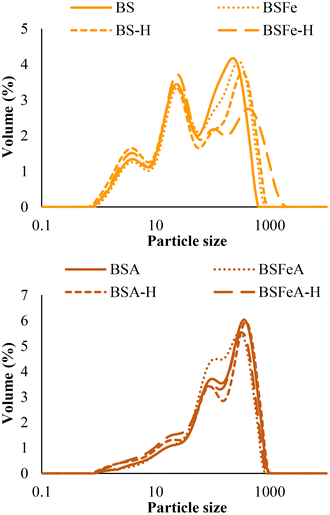 |
| Fig. 5 Particle size distribution of broad bean flours. BS, soaked broad bean flour; BS-H, soaked broad bean flour with hull; BSA, soaked–autoclaved broad bean flour; BSA-H, soaked–autoclaved broad bean flour with hull; BSFe, iron-soaked broad bean flour; BSFe-H, iron-soaked broad bean flour with hull; BSFeA, iron-soaked–autoclaved broad bean flour; BSFeA-H, iron-soaked–autoclaved broad bean flour with hull. | |
The BD of flours were in the range of 0.763–0.955 g mL−1, as shown in Table 1. Thermal treatment affected this property since the values of soaked–autoclaved flours were higher than those of the soaked flours. In fact, the first ones were in the range of 0.930 to 0.955 g mL−1, whereas the second ones were between 0.763 and 0.791 g mL−1. These results suggest that soaked–autoclaved flours have a denser structure than soaked flours.25,48 Kumar et al.48 who measured the BD of soaked broad bean flour and pressure-cooked broad bean flour, obtained results with the same tendency but with lower values (0.58 and 0.71 g mL−1, respectively). Naiker et al.49 also reported the same tendency but with hyacinth bean flour.
As regards the results of WAI, WSI and SP, it can be seen in Table 1 that WAI and SP were higher in soaked–autoclaved flours than in soaked flours. The values of WAI and SP were, respectively, in the range of 2.2–2.64 g g−1 and 3.05–3.48 g g−1 in soaked flours and in the range of 3.74–3.85 g g−1 and 4.36–4.46 g g−1 in soaked–autoclaved flours. The WAI is related to the capacity of flour to be associated with water molecules, which in turn is related to the hydrophilicity and gelation capacity of biomolecules.25,50 The increase in WAI and SP values after the soaking–autoclaving treatment could be due to starch gelatinisation during the heat treatment.51,52 Gelatinised starch loses the crystalline structure and increases the number of amorphous structures, leading to an increase of sites and groups taking part in water binding.53 The WSI presented an opposite tendency to WAI and SP. Soaked flours had a WSI of 24–27.7 g per 100 g, whereas soaked–autoclaved flours presented a WSI of 13.7–14.7 g per 100 g. The WSI is an indicator of the degree of leaching of soluble compounds from flour.51 The lower WSI of soaked–autoclaved flours may be attributed to the denaturation of proteins and the fact that before obtaining the flour, during the cooking of seeds, the soluble compounds of the broad beans could be leached out in the steam that condenses around them.52,54 Results with the same tendency were reported by Naiker et al.49 and Simons and Hall.52 Naiker et al.49 determined the WSI and SP of soaked and soaked–autoclaved hyacinth bean flours at different temperatures, and Simons and Hall52 determined the WAI and WSI of raw and cooked pinto bean flours.
Table 2 presents the determined techno-functional properties (water-holding capacity (WHC), oil-binding capacity (OBC), emulsifying activity (EA), emulsion stability (ES) and gelation capacity expressed as least gelation concentration (LGC)), and Table 3 the Pearson's correlation coefficients of physicochemical and techno-functional properties of the broad bean flours. As with the physicochemical properties, the techno-functional properties are mainly affected by the heat treatment.
Table 2 Techno-functional properties of broad bean flours
Flour |
WHC (g g−1) |
OBC (g g−1) |
EA (%) |
ES (%) |
LGC (%) |
Techno-functional properties are: WHC, water-holding capacity; OAC, oil-binding capacity; EA, emulsifying activity; ES, emulsion stability; LGC, least gelation concentration. Flours are: BS, soaked broad bean flour; BS-H, soaked broad bean flour with hull; BSA, soaked–autoclaved broad bean flour; BSA-H, soaked–autoclaved broad bean flour with hull; BSFe, iron-soaked broad bean flour; BSFe-H, iron-soaked broad bean flour with hull; BSFeA, iron-soaked–autoclaved broad bean flour; BSFeA-H, iron-soaked–autoclaved broad bean flour with hull. Different letters in each column indicate significant differences between the types of flour. |
BS |
1.07 ± 0.05d |
0.80 ± 0.04ab |
46.5 ± 0.8a |
68.3 ± 0.7a |
8b |
BSFe |
1.28 ± 0.09c |
0.84 ± 0.05a |
45.5 ± 0.4a |
65.4 ± 0.1a |
8b |
BSA |
1.79 ± 0.10ab |
0.86 ± 0.14a |
8.6 ± 0.3bc |
5.5 ± 0.4c |
20a |
BSFeA |
1.65 ± 0.04b |
0.78 ± 0.05ab |
4.5 ± 0.4c |
3.5 ± 0.0c |
20a |
BS-H |
1.34 ± 0.09c |
0.81 ± 0.04ab |
43.9 ± 3.4a |
55.7 ± 1.3b |
8b |
BSFe-H |
1.44 ± 0.02c |
0.83 ± 0.03a |
46.5 ± 0.8a |
53.9 ± 2.1b |
8b |
BSA-H |
1.84 ± 0.05a |
0.66 ± 0.02b |
10.3 ± 0.9b |
6.0 ± 0.1c |
20a |
BSFeA-H |
1.80 ± 0.02ab |
0.69 ± 0.04ab |
10.1 ± 0.8b |
6.0 ± 0.1c |
20a |
Table 3 Pearson's correlation coefficients of physicochemical and techno-functional properties of broad bean flours
|
WAI |
WSI |
SP |
BD |
WI |
d(0.5) |
D[4,3] |
WHC |
OBC |
EA |
ES |
LGC |
WAI: water absorption index; WSI: water solubility index; SP: swelling power; BD: bulk density; WI: whiteness index; d(0.5), maximum particle diameter below 50% of sample is contained; D[4,3], equivalent spherical diameter; WHC, water-holding capacity; OBC, oil-binding capacity; EA, emulsifying activity; ES, emulsion stability; LGC, least gelation concentration. Values in bold type correspond to p < 0.05. |
WAI |
1 |
|
|
|
|
|
|
|
|
|
|
|
WSI |
−0.9987
|
1 |
|
|
|
|
|
|
|
|
|
|
SP |
0.9989
|
−0.9956
|
1 |
|
|
|
|
|
|
|
|
|
BD |
0.9739
|
−0.9761
|
0.9655
|
1 |
|
|
|
|
|
|
|
|
WI |
−0.9555
|
0.9570
|
−0.9470
|
−0.9574
|
1 |
|
|
|
|
|
|
|
d(0.5) |
0.9318
|
−0.9399
|
0.9164
|
0.9599
|
−0.9689
|
1 |
|
|
|
|
|
|
D[4,3] |
0.8966
|
−0.8944
|
0.8994
|
0.8304
|
−0.8431
|
0.8483
|
1 |
|
|
|
|
|
WHC |
0.9563
|
−0.9477
|
0.9611
|
0.9217
|
−0.9134
|
0.8799
|
0.9493
|
1 |
|
|
|
|
OBC |
−0.5406 |
0.5330 |
−0.5430 |
−0.5104 |
0.6438 |
−0.4655 |
−0.3959 |
−0.5545 |
1 |
|
|
|
EA |
−0.9731
|
0.9794
|
−0.9630
|
−0.9911
|
0.9576
|
−0.9547
|
−0.8100
|
−0.8924
|
0.5221 |
1 |
|
|
ES |
−0.9965
|
0.9978
|
−0.9927
|
−0.9847
|
0.9587
|
−0.9391
|
−0.8767
|
−0.9463
|
0.5473 |
0.9867
|
1 |
|
LGC |
0.9777
|
−0.9826
|
0.9678
|
0.9926
|
−0.9740
|
0.9675
|
0.8333
|
0.9127
|
−0.5655 |
−0.9944
|
−0.9876
|
1 |
The WHC is the water that 1 g of flour imbibes and retains under low-speed centrifugation. Results obtained (Table 2) showed that the thermal treatment increased the WHC. In fortified flours, the WHC increased around 1.26 times whereas in non-fortified flours increased 1.67 times in samples without hull and 1.37 times in those with hull. These results are in agreement with several previous studies where flours from pigeon pea, dolichos bean, jack bean, cannellini bean, common bean, and hyacinth bean were analysed.49,55–57 WHC was positively correlated with WAI (r 0.9563, p < 0.001) and SP (r 0.9611, p < 0.001), and negatively correlated with WSI (r −0.9477, p < 0.001) (Table 3). Furthermore, samples with hulls presented slightly higher values than samples without hulls. BSA-H had the highest WHC with 1.84 g g−1, and BS had the lowest WHC with 1.07 g g−1. Similar results were obtained by Kumar et al.48 The increase of WHC after autoclaving could be related to protein denaturation and starch gelatinisation.48 During denaturation, proteins are unfolded and some polar side chains and hidden peptide bonds are exposed, which could increase the ability to hold water. Moreover, as mentioned above, gelatinised starch loses its crystalline structure, favouring water-holding.55
The high values of WHC, WAI, and SP of the soaked–autoclaved flours would make them suitable for doughs and meat products because it probably imbibes water without proteins dissolution, thereby attaining a thickening and viscous body.56 Moreover, the low WSI would make them suitable for pasta processing as they will have less soluble solids losses in the cooking water.50
The OBC is related to mouthfeel and flavour retention.55 The OBC of broad bean flours was around 0.8 g g−1 (Table 2), which was lower than the values reported in other legume flours.49,55–57 This would make them suitable for preparing fried products as they would not cause a greasy mouthfeel.56 No significant differences were observed between the samples except for the BSA-H, which had lower OBC, 0.66 g g−1. The obtained results are close to those of Kumar et al.,48 who measured the OBC of soaked and thermal treated broad bean flours and obtained results around 0.6 g g−1. The OBC has been attributed to the physical entrapment of oil within the protein through non-covalent bonds such as hydrophobic, electrostatic, and hydrogen bonds.57 The differences between samples could be related to the protein conformation change due to flour processing.
The EA and ES results of broad bean flours are also presented in Table 2. The EA is a techno-functional property related to the ability of proteins and other surface-active molecules to adsorb the oil–water interface, contributing to the formation of emulsions, whereas ES is a property related to the stability of this adsorbed layer over a period of time.55 Both properties were strongly affected by the autoclaving process carried out during the processing of flours. The soaked flours had an EA of approximately 45%, with and without hull and iron, which is similar to that obtained by Setia et al.27 (47.2%). After autoclaving, the EA was reduced by 78 to 89%, depending on the type of flour, which has been reported in previous studies.50,56,58 As regards ES, soaked flours without hull presented values of 65–68%, and soaked flours with hull of 53–55%, which is similar to the result (54.2%) of Setia et al.27 Soaked–autoclaved flours had a much lower ES, around 5%. Other studies with colourful beans (Phaseolus vulgaris L.) and hyacinth beans have also shown a decrease in ES after thermal treatment, but not as abruptly.49,50 The high EA and ES of soaked flours could be attributed to starch and fibre, which increase the viscosity of the continuous phase, as well as to the strength of the protein adsorbed in the interface.55 Likewise, the low EA and ES of soaked–autoclaved flours may be due to the protein denaturation resulting from the autoclaving process.50 These results agree with the correlations obtained as EA and ES were negatively correlated with WAI, SP, and WHC but positively correlated with WSI (Table 3).
Finally, the gelation capacity of broad bean flours was measured through the LGC. The lower the value of the LGC of the flour, the better its gelation capacity. The LGC of soaked and soaked–autoclaved flours were 8% and 20%, respectively (Table 2). This property was positively correlated with WAI, SP, and WHC, while it was negatively correlated with WSI, EA, and ES (Table 3). However, it means the opposite because the higher the LGC, the lower the CG. Like other techno-functional properties, gelation capacity was affected by the thermal treatment of the flours, but fortification and dehulling did not affect it. Kumar et al.48 and Aguilera et al.54 observed the same effect after thermal treatment. However, Kumar et al.,48 who studied the LGC of soaked and pressure-cooked broad bean flour, obtained lower LGC, with values of 6 and 10%, respectively. The decrease in the gelation capacity after autoclaving might be due to the denaturation and aggregation of proteins.54
4. Conclusions
Results of hydration kinetics showed that the use of vacuum impregnation during soaking reduced by 77% the time to reach 100% hydration of the broad beans in comparison to soaking without vacuum impregnation. Furthermore, using iron solution as a soaking medium did not affect the hydration kinetics of the broad beans. Iron content and its bioaccessibility are influenced by flour processing. Soaking with iron increased iron content and its bioaccessibility, whereas autoclaving process affects tannin, iron content, and its bioaccessible fraction. Finally, dehulling showed a decrease in iron content, although increased iron bioaccessibility was observed, occurred mainly due to the reduction in tannin concentrations. Regarding the physicochemical and techno-functional properties of flours, autoclaving was responsible of significant changes whereas iron fortification and dehulling did not affect practically any of them. The thermal process increased the water holding capacity and absorption rate, swelling capacity, bulk density, and particle size, while decreased the solubility index, whiteness index, emulsifying activity, emulsion stability, and gelling capacity of the flours. This study shows that vacuum impregnation can be used as a suitable technology to obtain iron-fortified broad bean flours with a higher amount of bioaccessible iron. Physicochemical and technological properties of the flours will depend on the applied processing conditions, which could be selected depending on the desired characteristics when the flours are added to food products.
Author contributions
Milagros Arnal: conceptualization, formal analysis, investigation, writing – original draft, writing – review & editing, visualization. Marta Gallego: conceptualization, supervision, writing – review & editing. Leticia Mora: conceptualization, supervision, writing – review & editing, project administration. Pau Talens: conceptualization, formal analysis, supervision, writing – review & editing, project administration, funding acquisition.
Conflicts of interest
There are no conflicts of interest to declare.
Acknowledgements
M. A. gratefully acknowledges the predoctoral contract (FPU19/02401) from the Spanish Ministry of Science, Innovation and Universities. Funding for open access charge: Universitat Politècnica de València.
References
- S. B. Dhull, M. K. Kidwai, R. Noor, P. Chawla and P. K. Rose, A review of nutritional profile and processing of faba bean (Vicia faba L.), Legume Sci., 2021, 4(3), e129 Search PubMed.
- K. A. Rahate, M. Madhumita and P. K. Prabhakar, Nutritional composition, anti-nutritional factors, pretreatments-cum-processing impact and food formulation potential of faba bean (Vicia faba L.): a comprehensive review, LWT – Food Sci. Technol., 2021, 138, 1–15 CrossRef.
-
L. L'Hocine, D. Martineau-Côté, A. Achouri, J. P. D. Wanasundara and G. W. Loku Hetti Arachchige, Broad bean (faba bean), in Pulses, ed. A. Manickavasagan and P. Thirunathan, Springer Nature Switzerland AG, Switzerland, 2020, vol. 3, pp. 27–54 Search PubMed.
- S. Sharan, G. Zanghelini, J. Zotzel, D. Bonerz, J. Aschoff and A. Saint-Eve,
et al., Fava bean (Vicia faba L.) for food applications: from seed to ingredient processing and its effect on functional properties, antinutritional factors, flavor, and color, Compr. Rev. Food Sci. Food Saf., 2021, 20(1), 401–428 CrossRef CAS PubMed.
- K. A. Millar, E. Gallagher, R. Burke, S. McCarthy and C. Barry-Ryan, Proximate composition and anti-nutritional factors of fava-bean (Vicia faba), green-pea and yellow-pea (Pisum sativum) flour, J. Food Compos. Anal., 2019, 82, 1–8 CrossRef.
- A. C. Miano and P. E. D. Augusto, The hydration of grains: a critical review from description of phenomena to process improvements, Compr. Rev. Food Sci. Food Saf., 2018, 17(2), 352–370 CrossRef PubMed.
- C. M. Chigwedere, D. M. Njoroge, A. M. Van Loey and M. E. Hendrickx, Understanding the relations among the storage, soaking, and cooking behavior of pulses: a scientific basis for innovations in sustainable foods for the future, Compr. Rev. Food Sci. Food Saf., 2019, 18, 1135–1165 CrossRef PubMed.
- E. Zanella-Díaz, H. Mújica-Paz, M. C. Soto-Caballero, J. Welti-Chanes and A. Valdez-Fragoso, Quick hydration of tepary (Phaseolus acutifolius A. Gray) and pinto beans (Phaseolus vulgaris L.) driven by pressure gradients, LWT – Food Sci. Technol., 2014, 59(2P1), 800–805 CrossRef.
- A. C. Miano and P. E. D. Augusto, The ultrasound assisted hydration as an opportunity to incorporate nutrients into grains, Food Res. Int., 2018, 106, 928–935 CrossRef CAS PubMed.
- Y. Man, T. Xu, B. Adhikari, C. Zhou, Y. Wang and B. Wang, Iron supplementation and iron-fortified foods: a review, Crit. Rev. Food Sci. Nutr., 2021, 1–22 CAS.
-
O. Dary and R. Hurrell, Guidelines on food fortification with micronutrients, World Health Organization, Food and Agricultural Organization of the United Nations, Geneva, Switzerland, 2006, pp. 1–376 Search PubMed.
- G. Mattar, A. Haddarah, J. Haddad, M. Pujola and F. Sepulcre, New approaches, bioavailability and the use of chelates as a promising method for food fortification, Food Chem., 2022, 373, 1–11 CrossRef PubMed.
- S. M. F. Bessada, J. C. M. Barreira and M. B. P. P. Oliveira, Pulses and food security: dietary protein, digestibility, bioactive and functional properties, Trends Food Sci. Technol., 2019, 93(228), 53–68 CrossRef CAS.
- M. C. Temba, P. B. Njobeh, O. A. Adebo, A. O. Olugbile and E. Kayitesi, The role of compositing cereals with legumes to alleviate protein energy malnutrition in Africa, Int. J. Food Sci. Technol., 2016, 51(3), 543–554 CrossRef CAS.
- EFSA, Scientific Opinion on the use of ferric sodium EDTA as a source of iron added for nutritional purposes to foods for the general population (including food supplements) and to foods for particular nutritional uses, EFSA J., 2010, 8(1), 1–32 Search PubMed.
- M. Peleg, An empirical model for the description of moisture sorption curves, J. Food Sci., 1988, 53(4), 1216–1217 CrossRef.
- H. E. S. Embaby, Effect of soaking, dehulling, and cooking methods on certain antinutrients and in vitro protein digestibility of bitter and sweet lupin seeds, Food Sci. Biotechnol., 2010, 19(4), 1055–1062 CrossRef CAS.
- AOAC, Official Methods of Analysis Method, 1995, 952.03.
- J. S. Kosse, A. C. Yeung, A. I. Gil and D. D. Miller, A rapid method for iron determination in fortified foods, Food Chem., 2001, 75(3), 371–376 CrossRef CAS.
- P. Zunin, F. Turrini, R. Leardi and R. Boggia, Olive fruits and vacuum impregnation, an interesting combination for dietetic iron enrichment, J. Food Sci. Technol., 2017, 54(2), 481–487 CrossRef CAS PubMed.
- A. Brodkorb, L. Egger, M. Alminger, P. Alvito, R. Assunção and S. Ballance,
et al., INFOGEST static in vitro simulation of gastrointestinal food digestion, Nat. Protoc., 2019, 14(4), 991–1014 CrossRef CAS PubMed.
- M. Minekus, M. Alminger, P. Alvito, S. Ballance, T. Bohn and C. Bourlieu,
et al., A standardised static in vitro digestion method suitable for food-an international consensus, Food Funct., 2014, 5(6), 1113–1124 RSC.
- H. Marta, Y. Cahyana, S. Bintang, G. P. Soeherman and M. Djali, Physicochemical and pasting properties of corn starch as affected by hydrothermal modification by various methods, Int. J. Food Prop., 2022, 25(1), 792–812 CrossRef CAS.
- Z. Q. Jiang, M. Pulkkinen, Y. J. Wang, A. M. Lampi, F. L. Stoddard and H. Salovaara,
et al., Faba bean flavour and technological property improvement by thermal pre-treatments, LWT – Food Sci. Technol., 2016, 68, 295–305 CrossRef CAS.
- S. K. Du, H. Jiang, X. Yu and J. L. Jane, Physicochemical and functional properties of whole legume flour, LWT – Food Sci. Technol., 2014, 55(1), 308–313 CrossRef CAS.
- F. Cornejo and C. M. Rosell, Influence of germination time of brown rice in relation to flour and gluten free bread quality, J. Food Sci. Technol., 2015, 52(10), 6591–6598 CrossRef CAS PubMed.
- R. Setia, Z. Dai, M. T. Nickerson, E. Sopiwnyk, L. Malcolmson and Y. Ai, Impacts of short-term germination on the chemical compositions, technological characteristics and nutritional quality of yellow pea and faba bean flours, Food Res. Int., 2019, 122, 263–272 CrossRef CAS PubMed.
- A. K. Stone, S. Parolia, J. D. House, N. Wang and M. T. Nickerson, Effect of roasting pulse seeds at different tempering moisture on the flour functional properties and nutritional quality, Food Res. Int., 2021, 147, 1–10 CrossRef PubMed.
- E. F. Sosa, C. Thompson, M. G. Chaves, B. A. Acevedo and M. V. Avanza, Legume seeds treated by high hydrostatic pressure: effect on functional properties of flours, Food Bioprocess Technol., 2020, 13(2), 323–340 CrossRef CAS.
- V. B. Sasikala, R. Ravi and H. V. Narasimha, Textural changes of green gram (Phaseolus aureus) and horse gram (Dolichos biflorus) as affected by soaking and cooking, J. Texture Stud., 2011, 42(1), 10–19 CrossRef.
-
M. Arnal, M. Gallego, L. Mora and P. Talens, Use of vacuum impregnation as a technology to reduce soaking time and to increase iron content in pulses, presented in poster session presentation at 14th International FRUTIC Symposium, Valencia, Spain, June–July, 2022.
- G. Xiao, J. Gong, Q. Ge and Y. You, Effect of vacuum soaking on the properties of soybean (Glycine max L. Merr.), Int. J. Food Eng., 2015, 11(1), 151–155 CAS.
- Q. Li, S. Li, X. Guan, K. Huang and F. Zhu, Effects of vacuum soaking on the hydration, steaming, and physiochemical properties of japonica rice, Biosci. Biotechnol. Biochem., 2021, 85(3), 634–642 CrossRef PubMed.
- Y. Tian, J. Zhao, Z. Xie, J. Wang, X. Xu and Z. Jin, Effect of different pressure-soaking treatments on color, texture, morphology and retrogradation properties of cooked rice, LWT – Food Sci. Technol., 2014, 55(1), 368–373 CrossRef CAS.
- P. García-Segovia, A. Andrés-Bello and J. Martínez-Monzó, Rehydration of air-dried Shiitake mushroom (Lentinus edodes) caps:
comparison of conventional and vacuum water immersion processes, LWT – Food Sci. Technol., 2011, 44(2), 480–488 CrossRef.
- N. Martínez-Navarrete, M. M. Camacho, J. Martínez-Lahuerta, J. Martínez-Monzó and P. Fito, Iron deficiency and iron fortified foods – a review, Food Res. Int., 2002, 35(2–3), 225–231 CrossRef.
- R. Alonso, A. Aguirre and F. Marzo, Effects of extrusion and traditional processing methods on antinutrients and in vitro digestibility of protein and starch in faba and kidney beans, Food Chem., 2000, 68(2), 159–165 CrossRef CAS.
- Y.-W. Luo and W.-H. Xie, Effect of different processing methods on certain antinutritional factors and protein digestibility in green and white faba bean (Vicia faba L.), CyTA – J. Food, 2013, 11(1), 43–49 CrossRef CAS.
- Y. Luo, W. Xie and Q. Cui, Effects of phytases and dehulling treatments on in vitro iron and zinc bioavailability in faba bean (Vicia faba L.) flour and legume fractions, J. Food Sci., 2010, 75(2), 191–198 CrossRef PubMed.
- K. Shubham, T. Anukiruthika, S. Dutta, A. V. Kashyap, J. A. Moses and C. Anandharamakrishnan, Iron deficiency anemia: a comprehensive review on iron absorption, bioavailability and emerging food fortification approaches, Trends Food Sci. Technol., 2020, 99, 58–75 CrossRef CAS.
- A. Cilla, G. López-García and R. Barberá,
In vitro bioavailability of iron and calcium in cereals and derivatives: a review, Food Rev. Int., 2018, 34(1), 1–33 CrossRef CAS.
- S. Multari, D. Stewart and W. R. Russell, Potential of fava bean as future protein supply to partially replace meat intake in the human diet, Compr. Rev. Food Sci. Food Saf., 2015, 14(5), 511–522 CrossRef.
- Y. W. Luo, W. H. Xie and Q. X. Cui, Effects of phytase, cellulase, and dehulling treatments on iron and zinc in vitro solubility in faba bean (Vicia faba L.) flour and legume fractions, J. Agric. Food Chem., 2010, 58(4), 2483–2490 CrossRef CAS PubMed.
- R. Krishnan, U. Dharmaraj and N. G. Malleshi, Influence of decortication, popping and malting on bioaccessibility of calcium, iron and zinc in finger millet, LWT – Food Sci. Technol., 2012, 48(2), 169–174 CrossRef CAS.
- S. Gwala, C. Kyomugasho, I. Wainaina, S. Rousseau, M. Hendrickx and T. Grauwet, Ageing, dehulling and cooking of Bambara groundnuts: consequences for mineral retention and in vitro bioaccessibility, Food Funct., 2020, 11(3), 2509–2521 RSC.
- S. Hemalatha, K. Platel and K. Srinivasan, Influence of heat processing on the bioaccessibility of zinc and iron from cereals and pulses consumed in India, J. Trace Elem. Med. Biol., 2007, 21(1), 1–7 CrossRef CAS PubMed.
- S. Thakur, M. G. Scanlon, R. T. Tyler, A. Milani and J. Paliwal, Pulse flour characteristics from a wheat flour miller's perspective: a comprehensive review, Compr. Rev. Food Sci. Food Saf., 2019, 18(3), 775–797 CrossRef CAS PubMed.
- S. R. Kumar, M. B. Sadiq and A. K. Anal, Comparative study of physicochemical and functional properties of soaked, germinated and pressure cooked faba bean, J. Food Sci. Technol., 2022, 59(1), 257–267 CrossRef CAS PubMed.
- T. S. Naiker, H. Baijnath, E. O. Amonsou and J. J. Mellem, The effect of soaking, steaming, and dehydration on the microstructure, physicochemical properties and in vitro starch digestibility of flour produced from Lablab purpureus (L.) Sweet (hyacinth bean), J. Food Process. Preserv., 2020, 44(5), 1–11 Search PubMed.
- J. A. C. Bento, P. Z. Bassinello, R. N. Carvalho, M. A. de Souza Neto, M. Caliari and M. S. Soares Júnior, Functional and pasting properties of colorful bean (Phaseolus vulgaris L.) flours: influence of the cooking method, J. Food Process. Preserv., 2021, 45(11), 1–14 Search PubMed.
- J. Ahmed, A. Taher, M. Z. Mulla, A. Al-Hazza and G. Luciano, Effect of sieve particle size on functional, thermal, rheological and pasting properties of Indian and Turkish lentil flour, J. Food Eng., 2016, 186, 34–41 CrossRef.
- C. W. Simons and C. Hall, Consumer acceptability of gluten-free cookies containing raw cooked and germinated pinto bean flours, Food Sci. Nutr., 2018, 6(1), 77–84 CrossRef CAS PubMed.
- X. Lv, L. Wu, J. Wang, J. Li and Y. Qin, Characterization of water binding and dehydration in gelatinized starch, J. Agric. Food Chem., 2011, 59(1), 256–262 CrossRef CAS PubMed.
- Y. Aguilera, R. M. Esteban, V. Benítez, E. Mollá and M. A. Martín-Cabrejas, Starch, functional properties, and microstructural characteristics in chickpea and lentil as affected by thermal processing, J. Agric. Food Chem., 2009, 57(22), 10682–10688 CrossRef CAS PubMed.
- T. Lin and C. Fernández-Fraguas, Effect of thermal and high-pressure processing on the thermo-rheological and functional properties of common bean (Phaseolus vulgaris L.) flours, LWT – Food Sci. Technol., 2020, 127, 1–12 CrossRef.
- Y. Aguilera, I. Estrella, V. Benitez, R. M. Esteban and M. A. Martín-Cabrejas, Bioactive phenolic compounds and functional properties of dehydrated bean flours, Food Res. Int., 2011, 44(3), 774–780 CrossRef CAS.
- B. A. Acevedo, C. M. B. Thompson, N. S. González Foutel, M. G. Chaves and M. V. Avanza, Effect of different treatments on the microstructure and functional and pasting properties of pigeon pea (Cajanus cajan L.), dolichos bean (Dolichos lablab L.) and jack bean (Canavalia ensiformis) flours from the north-east Argentina, Int. J. Food Sci. Technol., 2017, 52(1), 222–230 CrossRef CAS.
- S. Medhe, S. Jain and A. K. Anal, Effects of sprouting and cooking processes on physicochemical and functional properties of moth bean (Vigna aconitifolia) seed and flour, J. Food Sci. Technol., 2019, 56(4), 2115–2125 CrossRef CAS PubMed.
|
This journal is © The Royal Society of Chemistry 2023 |
Click here to see how this site uses Cookies. View our privacy policy here.