DOI:
10.1039/D2FO03151E
(Paper)
Food Funct., 2023,
14, 3600-3612
Ingestion of taxifolin-rich foods affects brain activity, mental fatigue, and the whole blood transcriptome in healthy young adults: a randomized, double-blind, placebo-controlled, crossover study†
Received
19th October 2022
, Accepted 27th February 2023
First published on 22nd March 2023
Abstract
The antioxidant properties of polyphenols, which are found in most plants, have been shown to be useful for maintaining health, including enhancing brain function and alleviating stress. We aimed to investigate the effect of a single intake of taxifolin-containing foods on cognitive task performance and whole blood gene expression in healthy young adults. This study was a randomized, placebo-controlled, double-blind, crossover trial in which healthy young adults were administered a single dose of either a placebo or food containing taxifolin. Cognitive tests (serial 3s, serial 7s, and rapid visual information processing) to examine brain activity and visual analog scale questionnaires to analyze mental fatigue were applied. The set of tests was repeated four times. The findings showed that taxifolin intake improved calculation abilities and reduced mental fatigue. An analysis of whole blood gene expression before and after the test revealed that the expression of foreign substance removal-related genes increased following the ingestion of taxifolin and that most differentially expressed genes were enriched in granulocytes. Taxifolin intake was shown to affect the brain activity of healthy young adults and demonstrated an antifatigue effect, thereby reducing subjective fatigue. A single intake of taxifolin may enhance the removal of foreign substances by strengthening the innate immune system and suppressing the occurrence of injury.
Introduction
Polyphenols are a component of pigments and bitterness in most plants, and their antioxidant activity is believed to be useful for maintaining health. Functional research into this is ongoing. To date, anti-obesity, cardiovascular protective, immunomodulatory, anti-inflammatory, antiviral, and other beneficial effects have been reported following polyphenol intake.1 In recent years, studies have investigated the effects of polyphenols as a countermeasure against the decline in brain function and stress associated with aging, which has become a social problem. For example, cocoa,2,3 grapes,4 Ginkgo biloba extract,5 and tea6 have been suggested to improve the performance of cognitive tasks, improve mood, and reduce vascular endothelial function deterioration associated with stress in healthy humans.
Taxifolin is a strong antioxidant and is believed to exhibit various functions such as microcirculatory improvement, liver protection, and anti-inflammatory effects.7–11 In addition, attention has been focused on the effects of taxifolin on brain function. This is because it has been shown to improve Alzheimer's disease and cerebral amyloid angiopathy in the elderly and to have a neuroprotective effect.12–14 However, there are few studies on the function of taxifolin in healthy young humans. Therefore, we investigated the function of taxifolin in healthy young individuals by performing brain activity tests, such as subtraction tasks. As a result, it was found that taxifolin intake has the possibility of increasing calculation abilities and the amount of change in fatigue level tends to be small. Therefore, taxifolin intake is expected to have a reducing effect on fatigue accumulation due to loads such as brain activity tests.15
Recently, the diagnosis of diseases by liquid biopsy, which analyzes blood and urine samples with a low degree of invasiveness, has gained interest. In liquid biopsy, markers such as DNA and RNA are analyzed and are being used as new biomarkers for cancer and depression. The advantages of liquid biopsy include the reduction of damage to the body and quick response, and it can be used to evaluate the functionality of stress and food. Gene expression in blood (blood cells) has been investigated for the detection of stress responses. Morita (2005) reported gene expression changes in blood cells under the stress of a presentation and question and answer session.16 It was reported that the Trier social stress test (consisting of speech and mental arithmetic) also affected blood cell gene expression, suggesting its potential use in stress research.17 Based on these findings, this study examined the effects of the load on the brain from the brain activity test on gene expression in blood.
In this study, we aimed to investigate the effects of taxifolin-containing foods on stress loading by brain activity testing, blood cortisol concentration measurements (a common biomarker of stress), and measuring the concentration of urinary 8-OHdG (a candidate for fatigue biomarkers), a marker of oxidative stress in vivo. In addition, we have introduced a blood transcriptome analysis, a new index for stress and fatigue, and studied detailed biological responses.
Materials and methods
Participants
This study was conducted in accordance with the tenets of World Medical Association's Declaration of Helsinki and the Ethical Guidelines for Medical and Health Research Involving Human Subjects in Japan. This research project was approved by the Tokai University School of Medicine (20R-063) and Kanagawa Institute of Industrial Science and Technology (S-2020-01) and was registered at the University Hospital Medical Information Network Clinical Trials Registry (UMIN-CTR, UMIN000041423).
Twenty-eight healthy students (13 males and 15 females), aged 20–29 years, studying in university were recruited from October 2020 to December 2020, and written informed consent was obtained from all participants.
The selection criteria for the study participants were (1) general good health, (2) aged 20–29 years, regardless of gender, and (3) ability to provide informed consent and to understand the test procedures. Exclusion criteria were as follows: (1) those who participated in the previous study (19R-082) and underwent cognitive measure tests (CMTs, e.g., serial 3s, serial 7s, and rapid visual information processing [RVIP]) for brain activity within 3 months before the start of this study; (2) those with diseases that could interfere with the study; (3) if inclusion might affect the evaluation of academic achievement (as adjudged by Professor Yasuhiro Nishizaki of Tokai University, the principal investigator of this study), and (4) involvement with the study as investigators or coordinators. The registered study participants completed health- and lifestyle-related questionnaires before the start of the study (Table S1†).
Trial design and procedure
The study was conducted as a randomized, placebo-controlled, double-blind crossover trial. A consortium diagram of the test is shown in Fig. 1. A series of intervention trials was conducted at the Tokai University. The participants were randomly divided into two groups: Sequence I and Sequence II. A 24 h daily questionnaire was administered the day before the rehearsal exercises as well as the Phase I and Phase II tests, and the meals of the previous day were recorded. In addition, participants were prohibited from taking chocolate, cocoa, coffee, black tea, green tea, oolong tea, and supplement foods (described as polyphenols and flavonoids) with a high content of polyphenols and flavonoids 24 h before the rehearsal exercise and Phase I and Phase II tests. In addition, from 12 h before the start of the test, participants were asked to refrain from alcohol intake and smoking. Furthermore, tests were not conducted during the menstruation period for female participants. In this study, blood and urine samples were collected in Phases I and II.
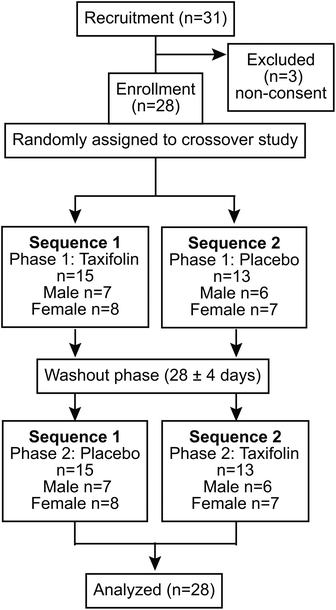 |
| Fig. 1 Consortium diagram for study participants. | |
The procedure was as follows: the participants came to the test site without having breakfast, blood and urine samples were collected, and then the participants ate breakfast at the test site. After breakfast, 1 unit of CMT was administrated as baseline for the day. After obtaining the baseline data, the participants ingested either a taxifolin-containing food or a placebo food. Four units of CMTs were administered 90 min after food intake. Subjective fatigue measurements were taken immediately before ingestion of the test and placebo foods, at the beginning of the test, and at the end of each test unit. Blood and urine samples were collected after the CMTs were conducted (Fig. 2).
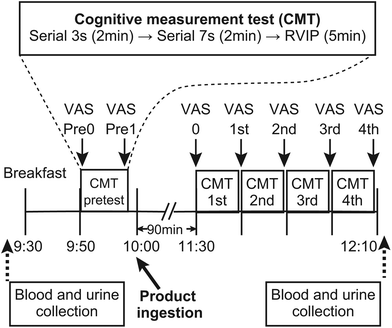 |
| Fig. 2 Time schedules of CMTs. | |
The Phase II test was performed at least 5 days after the Phase I test. In Phase II, different foods from those ingested in Phase I were ingested (those who ingested the taxifolin-containing food in Phase I ingested placebo food in Phase II), and the procedure was the same as in Phase I. A questionnaire regarding the study was conducted at the end of Phase II. The effect of taxifolin-containing or placebo food intake on brain activity was investigated.
Tested foods and dosage information
The compositions of the taxifolin-containing food and placebo food are listed in Table 1. The taxifolin-containing food was a tablet containing an extract from the xylem and cambium of the Dahurian larch tree, and six tablets (1500 mg) were ingested with water (250 mL). According to product specifications of the extract, the total amount of taxifolin ingested from the six tablets was 108 mg, which was set as the recommended daily intake of commercially available taxifolin-containing health foods. Placebo tablets were prepared by replacing the larch extract with starch. The taxifolin content of the taxifolin-containing foods used in the study was measured by HPLC and the actual value was 7.1 g per 100 g (107 mg per 1500 mg) and that of placebo foods was not detected.
Table 1 Test food compositions (6 tablets)
Raw materials |
Taxifolin food |
Placebo food |
≈90% of the Dahurian larch extract is composed of taxifolin.
The amount of taxifolin contained in 1500 mg of taxifolin food is about 108 mg.
|
Dahurian larch extracta (mg) |
120 |
0 |
Vitamin C (mg) |
300 |
300 |
Microcrystalline cellulose (mg) |
600 |
600 |
Starch (mg) |
420 |
540 |
Rapeseed hydrogenated oil (mg) |
60 |
60 |
Totalb (mg) |
1500 |
1500 |
CMTs
CMT, which combines serial 3s, serial 7s and RVIP, has been used as a cognitive task for acute response to foods such as cocoa, ginkgo etc.18 All tests were performed using a computer. CMTs were performed in the order of serial 3s (2 min), serial 7s (2 min), and RVIP (5 min), and this set was repeated four times.
Serial 3s testing involved randomly displaying a 3-digit number from which participants mentally subtracted three (−3) and inputted their answers. If the participant entered an incorrect answer but the calculation after that value was correct, it was counted as a correct answer. Serial 7s testing was performed in the same manner as serial 3s, but the subjects subtracted seven (−7) from the number displayed. For both Serial 3s and 7s, the measured items were the number of answers, correct answers, and incorrect answers, as well as the correct response rate, the number of re-entries (i.e., self-corrections), the time required to answer (correct and incorrect answers), and the time required to correct the answers (not including incorrect answers). Each question could be answered as many times as necessary before the Enter key was pressed to confirm the number, and the number of attempts was counted as the number of re-entered numbers. The reaction time was the time from one answer to the next (i.e., the time between pressing the Enter key, regardless of whether the response was correct or incorrect), and the time required to answer correctly was the time taken to input a correct answer to each question. During RVIP, the Enter key was pressed when three consecutive odd or even numbers were displayed among the numbers from 1 to 9 displayed one-by-one on the screen. The measurements taken following the RVIP test were the number of correct and incorrect answers, as well as the correct response rate, the number of missed answers (i.e., the number of odd or even numbers that increased three times in a row but were not recognized by the participant), and the reaction time.
Visual analog scale (VAS) for fatigue
Mental fatigue was measured using a VAS questionnaire in which the feeling of fatigue was described as a straight line with reference to the sensations shown on the left and right ends of the scale. The scale was set so that the best condition (0) on the left side indicated that no fatigue was felt and the worst condition (100) on the right side indicated that nothing could be done due to exhaustion according to the methods proposed by the Japanese Society of Fatigue Science (https://www.hirougakkai.com/).19
Measurement of serum cortisol and urinary 8-OhdG
Serum cortisol concentrations were measured at the SRL (Tokyo, Japan) using the electrochemiluminescence immunoassay. Urinary creatinine correction was performed to measure the urinary 8-OHdG concentration, which was measured at NIKKEN SEIL Co., Ltd (Shizuoka, Japan) using the enzyme-linked immunosorbent assay.
Collection of whole blood RNA
Blood for RNA extraction was collected using a PAXgene® RNA blood collection tube (Becton, Dickinson and Company, NJ, USA). After mixing, the mixture was allowed to stand at room temperature (20–21 °C) for 2 h before being frozen at −20 °C, and total RNA was extracted using the PAXgene® blood miRNA kit (PreAnalytiX GmbH, Hombrechtikon, Switzerland).
The concentration and quality of the extracted total RNA was measured using a bioanalyzer (2100 Bioanalyzer, Agilent Technologies, Inc., CA, USA) (RNA Integrity Number 7.7–9.6, average 8.6). RNA was stored at −80 °C until use.
Whole blood DNA microarray data collection and data analysis
Total RNA (50 ng) was fragmented and labeled with cDNA using the GeneChipWT PLUS reagent kit (Thermo Fisher Scientific, MA, USA) according to the manufacturer's protocol. The labeled fragment was hybridized to Clariom S, a human array (Thermo Fisher Scientific), for 16 h, and then washed and stained using the GeneChip ™ hybridization, wash, and stain kit (Thermo Fisher Scientific). The washed array was scanned using a GeneChip™ Scanner 3000 7G (Thermo Fisher Scientific) to obtain the CEL data.
CEL data were normalized by qFARMS for each food, and the two groups, before and after CMTs, were compared using Rankproduct 2.0. Genes with a false discovery rate (FDR) < 0.001 were identified as differentially expressed genes (DEGs) after the brain activity test. Next, the biological functions of the DEGs were investigated using the gene ontology (GO) term classification. Database for Annotation, Visualization and Integrated Discovery (DAVID) v6.8 was used for classification, and the GO term with an FDR correction with p-value <0.05 was selected.20
In addition, the Human Protein Atlas (https://www.proteinatlas.org) was used to classify the DEGs according to the types of blood cells expressed as enriched or enhanced.21,22 Subcategories, “cell type enriched”, “group enriched”, and “cell type enhanced”, were aggregated as being classified as highly specific. Furthermore, pathways involving DEGs were investigated by performing the ingenuity pathway analysis (IPA, Qiagen).
Statistical analysis
The results of CMTs and VAS questionnaires after ingestion of taxifolin-containing foods and placebo foods were statistically analyzed using a linear-mixed model using SPSS version 26 (IBM). Statistical significance was set at p < 0.05. Mann–Whitney's U-test was performed for the increased values obtained on the VAS questionnaire, serum cortisol concentrations, and urinary 8-OHdG values.
Results
Subject demographics
The mean age of the study participants was 21.4 ± 0.4 years for Sequence I (ntotal(I) = 15, nmale(I) = 7, nfemale(I) = 8) and 21.5 ± 0.6 for Sequence II (ntotal(II) = 13, nmale(II) = 6, nfemale(II) = 7).
Serial 3s
The number of responses and the number of correct answers in the female–male mixed analysis (total) were significantly higher following taxifolin intake than those after placebo intake. In addition, taxifolin intake led to a significantly shorter time required to answer and the time required to answer correctly in the female–male mixed analysis. Following taxifolin intake, female participants tended to reenter answers less frequently (p = 0.087) (Fig. 3 and Table S2†). However, the number of incorrect answers and the rate of correct answers were not affected by the foods ingested (Table S2†).
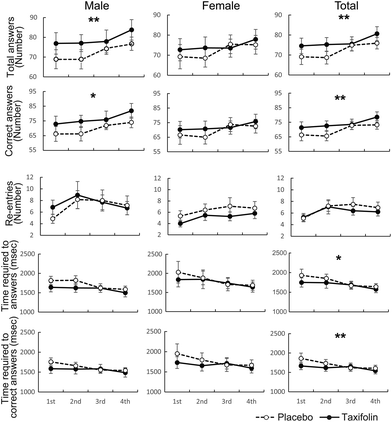 |
| Fig. 3 Change in serial 3s scores. Placebo treatment: white circles and broken lines. Taxifolin treatment: black circles and lines. Data are expressed as mean ± SE. nmale = 13; nfemale = 15; ntotal = 28. All p-value were calculated using linear-mixed models. * and ** indicate food effects at p < 0.05 and 0.01, respectively. | |
Serial 7s
Data were analyzed regardless of gender, and the number of responses and number of correct answers were significantly higher following taxifolin intake than those after placebo intake. Data obtained from men demonstrated that taxifolin intake led to an increase in responses (p = 0.058) and correct answers (p = 0.091). The data obtained from the female participants showed that following taxifolin intake, the participants re-entered their answers less frequently (p = 0.084) (Fig. 4 and Table S2†). However, the number of incorrect answers and the rate of correct answers were not affected by the foods ingested (Table S2†).
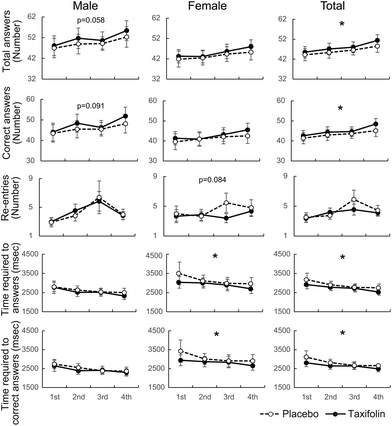 |
| Fig. 4 Change in serial 7s scores. Placebo treatment: white circles and broken lines. Taxifolin treatment: black circles and lines. Data are expressed as mean ± SE. nmale = 13; nfemale = 15; ntotal = 28. All p-value were calculated using linear-mixed models. * and ** indicate food effects at p < 0.05 and 0.01, respectively. | |
RVIP
There was no difference between taxifolin intake and placebo intake in any of the measured items (Table S2†).
VAS questionnaire
The analysis of all repetitions of the CMT unit showed that male participants tended to be less fatigued after taxifolin intake than after placebo intake (p = 0.061). There was no difference observed between taxifolin-containing and placebo foods in the female-only and female–male mixed analyses (Fig. 5A–C and Table S3†).
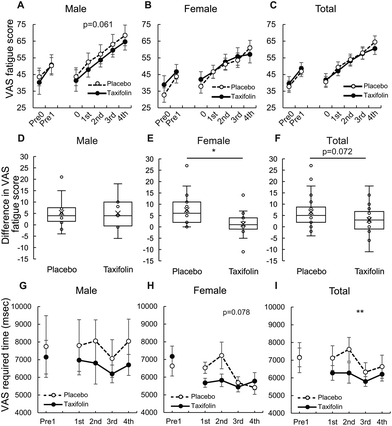 |
| Fig. 5 The change in VAS fatigue scores. The change in VAS fatigue scores through the CMTs (A: male, B: female, C: total). The difference of change in VAS fatigue scores between the 3rd and 4th CMT (D: male, E: female, F: total). VAS required time (msec) (G: male, H: female, I: total). Data are expressed in mean ± SE. nmale = 13, nfemale = 15, ntotal = 28. * and ** indicate food effects at p < 0.05 and 0.01, respectively. P-Values of A, B, C, G, H and I were calculated using linear-mixed model. P-Values of D, E and F were calculated using Mann-Whitney's U-test. | |
When comparing the differences in VAS scores between the 3rd and 4th CMT, no difference was observed between taxifolin-containing and placebo foods in the male-only analysis. However, the difference in VAS scores between the 3rd and 4th CMT of taxifolin food was significantly lower than that of placebo food in the female-only analysis. A similar tendency was observed in the female–male mixed analyses (p = 0.072) (Fig. 5D–F).
Data obtained from male participants showed that individual differences in the time required for VAS were large, but the average time required for VAS was shorter following taxifolin intake. The data from the female participants demonstrated that the time required for VAS tended to be shorter following taxifolin intake (p = 0.078). The average time required for VAS in both sexes was significantly shorter following taxifolin intake than following placebo intake (Fig. 5G–I).
Serum cortisol and urinal 8-OHdG
There was no difference in serum cortisol and urinary creatinine-corrected 8-OHdG levels before and after CMTs between taxifolin-containing foods and placebo food (Fig. S1†) in all three gender-based analyses (Fig. S1†).
Whole blood transcriptome
DEGs.
To compare gene expression before and after CMTs, Venn diagrams were created based on males or females and both sexes, and the GO term classification of specific DEGs was performed for each food. The results showed that there was no significant difference between males and females. Therefore, whole blood gene expression has been analyzed using data for both males and females. The number of DEGs was upregulated after CMTs compared with before CMTs was 167 after placebo and 316 after taxifolin. The number of DEGs was downregulated after CMTs compared with before CMTs was 238 after placebo and 356 after taxifolin.
We further investigated the genes that changed similarly following both placebo and taxifolin intake and genes that changed specific to each condition. GO term classification was performed for each gene, and the GO term with an FDR-corrected p-value <0.05 is shown in Fig. 6.
 |
| Fig. 6 Venn diagram and GO terms. GO terms listed for an FDR-corrected p-value <0.05. The same GO terms are colored by the same background. | |
Genes commonly expressed after CMTs, following ingestion of placebo or taxifolin-containing foods, were classified into GO:0006955 immune response and GO:0006954 inflammatory response, and there were many receptors related to the immune system and stimulus response. Conversely, the GO term gene with decreased expression was associated with protein biosynthesis and transport and was composed of eukaryotic initiation factors and ribosomal RNA. These are GO:0006413 translational initiation, GO:0006614 membrane-targeted signal recognition protein-dependent cotranslational protein targeting the membrane, GO:0019083 viral transcription, GO:0000184 nuclear-transcribed mRNA catabolic process nonsense-mediated decay, and GO:0006412 translation. Among the genes that were upregulated after CMTs, there was no highly enriched GO term with an FDR-corrected p-value <0.05, for each food-specific DEG that changed only following placebo or taxifolin intake.
Among the genes that were downregulated after the CMTs, the placebo-specific downregulated genes were related to GO:0002576 platelet degranulation, and their contents were mainly cytoskeletal proteins. The top six GO terms of the taxifolin-specific downregulated genes were the same GO terms as the common DEGs (GO:0000184, GO:0006413, GO:0006614, GO:0019083, GO:0006412, and GO:0006364). In addition, the genes classified into these GO terms were almost similar and contained a large amount of ribosomal RNA that did not change following ingestion of the placebo. For example, in GO:0006413 translation initiation, ten ribosomal RNA genes were commonly downregulated in both taxifolin and placebo conditions, while 34 ribosomal RNA genes were downregulated only following taxifolin intake. After taxifolin intake, the number of ribosomal RNA genes with reduced expression was approximately three times that of the placebo condition. GO:0002181 cytoplasmic translation and GO:0000027 ribosomal large subunit assembly were also composed of ribosomal RNA. Other GO terms were GO:0098609 cell–cell adhesion, GO:0006414 translational elongation, GO:0042102 positive regulation of T-cell proliferation, GO:0007166 cell surface receptor signaling pathway, GO:0050862 positive regulation of T-cell receptor signaling pathway, GO:0050690 regulation of defense response to virus by virus, GO:0045727 positive regulation of translation, and GO:0017148 negative regulation of translation. Most of the genes contained in these GO terms were CD antigens expressed on T cells and various regulators.
Reversal DEGs.
Table 2 summarizes the genes in which the direction of gene expression was reversed between placebo and taxifolin intake conditions. In addition, these genes were classified according to the blood cells that were enriched or enhanced. As a result, these genes that were upregulated after ingestion of taxifolin and downregulated after ingestion of placebo were classified as being enriched or enhanced in monocytes and granulocytes. Furthermore, the genes that were downregulated after ingestion of taxifolin and upregulated after ingestion of placebo were classified as being enriched or enhanced in T cells. In addition, when the functions of the DEGs that exhibited a reversed expression between the taxifolin-containing and placebo intake conditions were investigated, CD68, monocyte to macrophage differentiation-associated (MMD) protein, and G protein-coupled receptor 65 (GPR65) were involved in removal of foreign substances. Thus, a difference was observed in the foreign substance removal function between the placebo intake and taxifolin ingestion conditions.
Table 2 Blood cell type in which the expression of the DEGs was reversed between placebo and taxifolin conditions
Gene symbol |
Expression |
Blood cell type |
Placebo |
Taxifolin |
Up/down: gene expression increased/decreased after CMTs. All gene symbols and their full names are listed in Table S4.† |
CD68
|
Down |
Up |
Intermediate monocyte, nonclassical monocyte, and classical monocyte |
CHP1
|
Down |
Up |
Low cell type specificity |
F11R
|
Down |
Up |
Eosinophils and neutrophils |
G6PD
|
Down |
Up |
Eosinophil |
LYL1
|
Down |
Up |
Eosinophil |
MMD
|
Down |
Up |
Low cell type specificity |
MOB3A
|
Down |
Up |
Low cell type specificity |
PHC2
|
Down |
Up |
Neutrophils |
ANKRD36
|
Up |
Down |
Low cell type specificity |
ANKRD36B
|
Up |
Down |
Low cell type specificity |
APOBEC3C
|
Up |
Down |
Low cell type specificity |
ARPC2
|
Up |
Down |
Low cell type specificity |
DCAF16
|
Up |
Down |
Low cell type specificity |
DDX39B
|
Up |
Down |
Low cell type specificity |
DDX5
|
Up |
Down |
Low cell type specificity |
GPR65
|
Up |
Down |
Low cell type specificity |
GZMK
|
Up |
Down |
MAIT T-cell and memory CD8 T-cell |
HNRNPM
|
Up |
Down |
Low cell type specificity |
MATR3
|
Up |
Down |
Low cell type specificity |
NOTCH2NL
|
Up |
Down |
Low cell type specificity |
RSRP1
|
Up |
Down |
Low cell type specificity |
S1PR5
|
Up |
Down |
gdT-cell, MAIT T-cell, memory CD8 T-cell, and naive CD8 T-cell |
ZNF107
|
Up |
Down |
Low cell type specificity |
DEGs related to foreign substance removal.
DEGs involved in foreign substance removal are listed in Table 3. Lysosomes are organelles that degrade intracellular and extracellular components and contain various hydrolases. Taxifolin intake increased the expression of lysosomal enzyme genes, such as cathepsin D (CTSD), cathepsin S (CTSS), glucosidase beta acid (GBA), glucosamine (N-acetyl)-6-sulfatase (GNS), and iduronate 2-sulfatase (IDS). In the lysosomal membrane protein, the genes for CD68, lysosomal associated membrane protein 2 (LAMP2), lysosomal protein transmembrane 5 (LAPTM5), lipopolysaccharide-induced TNF factor (LITAF), mucolipin 1 (MCOLN1), and solute carrier family 11 (proton-coupled divalent metal ion transporter) member 1 (SLC11A1) were upregulated. In contrast, a few genes were downregulated. Phagosomes, such as macrophages, are organelles that degrade microorganisms, senescent cells, or apoptotic cells, and fuse with lysosomes to degrade substances. Phagosomes acquire specific degrading enzymes, depending on their maturity. Ingestion of taxifolin increased the expression of many genes, which are enzymes from early phagosomes to mature phagosomes (neutrophil cytosolic factor 2 [NCF2], member RAS oncogene family [RAB5C and RAB7A]), Fc receptors (Fc fragment of IgG receptor IIa [FCGR2A] and IIb[FCGR2B]), Toll-like receptor 4 (TLR4), and v-type ATPases (ATPase, H+ transporting, lysosomal V0 subunit a1 [ATP6V0A1], and ATPase, H+ transporting, lysosomal 9 kDa, and V0 subunit e1 [ATP6VE1]). However, a few genes showed reduced expression. Placebo intake had approximately the same number of upregulated and downregulated DEGs related to lysosomes and phagosomes, but their expression levels were less than those after taxifolin intake. Therefore, the results indicate that taxifolin intake had more genes that were upregulated and that foreign substance removal was more advanced than placebo intake.
Table 3 Genes related to foreign substances removal
Organelle |
Classification |
Placebo |
Taxifolin |
Up |
Down |
Up |
Down |
Up/down: genes upregulated/downregulated compared with before and after the CMTs. All gene symbols and their full names are listed in Table S4.† |
Lysosome |
Acidification |
|
|
ATP6V0A1, TCIRG1
|
|
Adaptor protein complex |
|
|
|
AP1S2
|
Lysosomal acid hydrases |
|
|
|
|
proteases |
CTSS, CTSZ
|
CTSA, CTSB, CTSW
|
CTSD, CTSS
|
CTSA
|
Glycosidases |
|
|
GBA
|
|
Sulfatases |
IDS
|
|
GNS, IDS
|
|
Lipases |
|
LIPA
|
|
LIPA
|
Ceramidase |
ASAH1
|
|
ASAH1
|
|
Other lysosomal enzymes and activators |
|
|
PSAP
|
|
Lysosomal membrane proteins |
LAMP2
|
CD68
|
CD68, LAMP2, LAPTM5, LITAF, MCOLN1, SLC11A1
|
SORT1
|
Others |
IGF2R
|
|
IGF2R
|
|
Phagosome |
Phagocytosis-promoting receptors |
|
|
|
|
Fc receptors |
FCGR2B
|
|
FCGR2A, FCGR2B
|
|
Toll-like receptors |
|
|
TLR4
|
|
NADPH oxidase complex |
|
|
NCF2
|
|
Early phagosome |
|
|
RAB5C
|
CALR, TFRC
|
Mature phagosome |
|
TUBA4A, TUBB4B
|
RAB7A, TUBA1A
|
|
Phagolysosome |
LAMP2, CTSS
|
|
LAMP2, CTSS
|
|
ATPase |
|
|
ATP6V0A1, ATP6V0E1, TCIRG1
|
|
Others |
|
|
|
CORO1A
|
Classification of DEGs by blood cell type.
The proportion of low specificity DEGs that had no bias for the cells in which they were expressed was approximately 46% for the DEGs that were common between the taxifolin and placebo conditions; approximately 70% for placebo–taxifolin reversal DEGs, approximately 60% for placebo-specific DEGs, and approximately 54% for taxifolin-specific DEGs. Approximately half of the DEGs were widely expressed regardless of cell type. Conversely, among the food intake-specific and reversal DEGs, those that were upregulated in any type of blood cell (for example, neutrophils or T cells) were classified by blood cell type (Table 4). The upregulated DEGs following taxifolin intake were mainly upregulated in granulocytes, especially neutrophils, monocytes, and DCs. Of the 220 genes that exhibited an increase in expression after taxifolin intake, 123 genes accounted for approximately 56% of the enriched or enhanced genes in neutrophils. Of the 234 downregulated DEGs, only five genes were enriched or enhanced in neutrophils. Among the genes that were upregulated following taxifolin intake, few genes were classified as enriched or enhanced in NK, B cells, and T cells, and more genes were downregulated. Many genes that are classified as being enriched or enhanced in T cells were downregulated. The cell types in which DEGs were classified as being enriched or enhanced following the placebo or taxifolin ingestion differed depending on the food type ingested. Placebo intake did not lead to the accumulation of genes that were enriched or enhanced in neutrophils, which contrasted with the findings of taxifolin intake. The genes that were similarly regulated following placebo and taxifolin intake showed the same tendency as taxifolin intake, and the upregulated genes were classified as being enriched or enhanced in granulocytes (mostly neutrophils), monocytes, and DCs. In addition, many downregulated genes were classified as being enriched or enhanced in T cells (Table 4). These results suggest that taxifolin intake and placebo intake affected blood cells in different ways.
Table 4 Classification of DEGs with enriched or enhanced expression by cell type
Food |
Group |
Gene expression |
Granulocytes |
Monocytes |
DC |
Lymphocytes |
Basophils |
Eosinophils |
Neutrophils |
NK cells |
B cells |
T cells |
Up/down: genes that were upregulated/downregulated compared with before and after the CMTs. |
Placebo |
Total |
Up |
22 |
21 |
87 |
45 |
19 |
2 |
11 |
6 |
Down |
19 |
19 |
26 |
19 |
13 |
8 |
4 |
77 |
Specific |
Up |
5 |
4 |
19 |
25 |
12 |
1 |
6 |
0 |
Down |
16 |
9 |
19 |
14 |
12 |
2 |
4 |
10 |
Reverse |
Up |
0 |
0 |
0 |
0 |
0 |
0 |
0 |
6 |
Down |
0 |
3 |
2 |
3 |
0 |
0 |
0 |
0 |
Taxifolin |
Total |
Up |
30 |
43 |
191 |
72 |
25 |
4 |
13 |
7 |
Down |
11 |
11 |
10 |
16 |
13 |
16 |
15 |
163 |
Specific |
Up |
13 |
23 |
121 |
49 |
18 |
3 |
8 |
7 |
Down |
8 |
4 |
5 |
14 |
12 |
10 |
15 |
90 |
Reverse |
Up |
0 |
3 |
2 |
3 |
0 |
0 |
0 |
0 |
Down |
0 |
0 |
0 |
0 |
0 |
0 |
0 |
6 |
Common |
Up |
17 |
17 |
68 |
20 |
7 |
1 |
5 |
0 |
|
Down |
3 |
7 |
5 |
2 |
1 |
6 |
0 |
67 |
|
IPA pathway and biofunctional analyses.
Using IPA, we analyzed the functions and pathways that were increased or decreased, considering the direction of gene expression changes. Pathway analysis revealed that eukaryotic translation initiation factor-2 signaling was the most affected by CMTs following both placebo and taxifolin-containing food intake, and the expression of many ribosomal RNAs was reduced. Biofunctions with |Z| scores >2 were extracted in the inflammatory response category (Table 5). The immune response of leukocytes was common following the intake of placebo and taxifolin-containing foods. It was speculated that placebo reduced the platelet count, and taxifolin reduced encephalitis. In addition, taxifolin was inferred to have a decreasing tendency for inflammation of the central nervous system at z = −1.99.
Table 5 Biofunctional annotation of the inflammatory response category
Food |
Diseases or functional annotation |
Predicted activation state |
Activation z-score |
Placebo |
Quantity of blood platelets |
Decreased |
−2.4 |
Dermatitis |
Increased |
2.79 |
Antibody response |
Increased |
2.4 |
Immune response of leukocytes |
Increased |
2.34 |
Taxifolin |
Encephalitis |
Decreased |
−2.13 |
Experimental autoimmune encephalomyelitis |
Decreased |
−2.09 |
Immune response of leukocytes |
Increased |
2.14 |
Discussion
Effect of taxifolin on brain activity
This study investigated the effects of taxifolin-containing foods on brain activity, stress, and blood gene expression. Serial 3s and serial 7s in the CMT used in this study reported different stress loads on the brain, with serial 7s reporting a higher load.23 In this study, as in previous reports, serial 7s had fewer answers than serial 3s and took longer to respond. In serial 7s, although the number of responses was small and the errors were large, a clear difference could not be obtained, but there was a tendency for taxifolin to have better results. In this study, a single intake of taxifolin-containing food increased the number of answers and the number of correct answers for the serial 3s and serial 7s tests, which are subtraction tasks in the brain activity test. As attention and calculation ability (mental arithmetic) are measured for each subtraction task, it is considered that the improvement of the performance in the serial 3s and serial 7s subtraction tasks is due to taxifolin intake improving working memory and attention. As the time required for answering and the time required to give correct answers were decreased, it was likely that the increase in the number of answers and the number of correct answers was the result of the decrease in the time required for providing an answer. This result is in agreement with the results of a previous study.15 Examples of an improved response rate following intake of a single food, like this test, were obtained with Ginkgo biloba extract and cocoa.2,5 A transient increase in cerebral blood flow has been suggested as the mechanism for decreasing the speed of response, and fMRI has confirmed an increase in blood flow following cocoa intake.24 Glucose and oxygen, which are energy sources for cranial nerve cells, are supplied to the necessary sites by cerebral blood flow. Cranial nerve cells increase local cerebral blood flow when nerve activity increases transiently and supplies glucose and oxygen where needed. Taxifolin has been reported to increase cerebral blood flow in an ischemic brain disease mouse model.12 In a report by Saito, et al. (2017), no effect of taxifolin administration on blood flow was observed in quiescent wild-type mice.12 However, as the brain activity test is a load that reduces blood flow,25 taxifolin intake may have increased neural activity by increasing blood flow in the brain activity test. Taxifolin and its metabolites were faintly detected in the brain after taxifolin administration in rats, suggesting that they are capable of slightly crossing the brain–blood barrier.26 These results suggested that a small amount of taxifolin and its metabolites that entered the brain may affect the cranial nerves. It has also been reported that some polyphenols that are difficult to absorb affect the central nervous system via the nerves of the digestive tract.27 Further study would clarify the mechanisms of this responses with taxifolin. It is presumed that the result was an increase in the number of answers and a decrease in the time required to provide answers. The time required to answer is the total time required to recognize numbers, calculate, and input calculation results, and food intake can affect any of these processes. As the extract containing taxifolin activates nerve cells, it may have affected the cranial nerves as well as peripheral nerve transmission, affecting muscle activity when inputting the answer number.28 Based on these results, taxifolin intake affected working memory but demonstrated no effect on RVIP, a vigilance task; therefore, taxifolin showed no significant effect on sustained attention.
Effect of taxifolin on mental fatigue
The degree of mental fatigue in this study was lower following taxifolin intake than placebo intake in males, and there was no difference between food intake throughout the CMTs in the female-only and female–male mixed analyses. However, the difference in VAS scores between the 3rd and 4th repeated CMTs was small in the female–male mixed and female-only analyses, and taxifolin intake reduced the accumulation of fatigue or acted to relieve fatigue. Various reports have been published regarding the reduction of mental fatigue caused by polyphenols. Cacao flavanols,2,29 matcha,6,30 and purple grape31 have been reported to reduce reaction time and mental fatigue in acute food responses in brain function tests. These responses may be a common effect of easily absorbable polyphenols. The absorption kinetics of the taxifolin-containing extract used in this experiment was unknown, but taxifolin was classified as easy-absorbing because the tmax was as fast as 0.5 h in rabbits and rats. It is believed that this has a similar effect.32,33
Effect of taxifolin on decision-making skills
With taxifolin intake, the time required for VAS was shorter in female-only and female–male mixed analyses, suggesting that taxifolin intake led to faster decision making and improved judgment. The ventral prefrontal cortex functions in decision making, and a difference exists in left–right functional differentiation between male and female individuals.34–36 However, in this study, although the error was large in the required time for VAS in males, the average score following taxifolin intake was lower than that of placebo, suggesting that both male and female participants have the same tendency in judgment.
Effect of taxifolin on serum cortisol and urinary 8-OHdG levels
As there was no difference in serum cortisol concentration (a general stress marker) and urinary 8-OHdG (an oxidative stress marker) after food intake under the loading conditions of CMTs used in this study, taxifolin did not affect the hypothalamic–pituitary–adrenal system or systemic oxidative stress in the stress responses.
This study was conducted from morning to noon, the time of day when the diurnal variation in cortisol is most pronounced, and as in the report, there was a marked decrease in cortisol at noon at the end of the study. However, it has not been verified whether CMT suppresses the decrease in cortisol associated with this diurnal variation (whether it remains high). Therefore, it is unknown (and cannot be discussed) whether the CMT run caused any changes in cortisol. On the other hand, some reports suggest that stresses that alter cortisol, such as performance tasks characterized by threats to social reputation and/or loss of control, cause significant increases in cortisol levels.37 Since the CMT task performance in this study was not to be compared or evaluated with others, and the element of threat to social evaluation was considered low, it is possible that it was not of a nature to cause changes in cortisol. If CMT task execution induces subjective fatigue but does not induce cortisol change, it is assumed that the effect of taxifolin on fatigue reduction during CMT task execution was also difficult to assess from the cortisol level.
Function of whole blood DEGs
This study detected blood genes whose expression varied before and after CMT. Because the CMT used in this study was different from the studies by Morita (2005) and Nater (2009) in terms of mental load, the DEGs were not exactly the same as those reported.16,17 However, it was found that the load on brain activity by CMT affected blood gene expression, and that the changes were partially ameliorated by taxifolin.
The number of DEGs before and after the CMTs was higher following taxifolin ingestion than those after placebo ingestion, demonstrating that taxifolin and the placebo exerted different responses to gene expression. Among DEGs that were common following placebo and taxifolin ingestion, those with an increased expression after the test were genes related to immune and inflammatory responses. As many of these were immune-related receptors, they were hypothesized to affect the intracellular transmission of stimuli and information. Many of the genes that were downregulated after the CMTs were related to protein synthesis. When cells are exposed to stress, such as infection, endoplasmic reticulum stress, UV light, and nutrient deficiency, they reduce the load on the cells by inhibiting the protein synthesis pathway.38,39 Although the CMTs in this experiment did not significantly change the blood cortisol level, a load might have reduced the protein synthesis of blood cells. Taxifolin-specific DEGs demonstrated high protein synthesis-related enrichment and contained a large amount of ribosomal RNA, similar to the common DEGs that decreased when placebo and taxifolin were ingested. Therefore, it was speculated that taxifolin intake reduced protein synthesis to a greater extent than the placebo, reducing the load on cells. The genes that were regulated in opposing directions between the placebo and the taxifolin-containing food conditions contained CD68, MMD, and GPR65, which are related to foreign substances removal; therefore, there is a great difference in the effects on foreign substances removal between foods. The foreign substances in this study are dead cell fragments or abnormal proteins that are generated by a brain activity test load. Lysosomes and phagosomes play a role in removing foreign substances, and we found that many of the genes contained in them were upregulated after the CMTs, following taxifolin ingestion. In addition, many of the DEGs following taxifolin ingestion were enriched or enhanced by monocytes, macrophages, and granulocytes. As these blood cells have an innate immune function and act to remove foreign substances by phagocytosis, the removal of foreign substances caused by loading is more advanced after ingestion of taxifolin than after placebo. Zhang, et al. (2019) reported that taxifolin promotes autophagy-induced foreign substance removal in cultured microglial cells (BV2 cells) injured by chlorpyrifos (a pesticide component).40 In this study, it is possible that unnecessary proteins generated in the cells are removed. Many lysosomal enzymes work during phagocytosis, resulting in the production of reactive oxygen species. Reactive oxygen species are useful for the elimination of pathogens,41 and H2O2 and NO promote inflammatory mediator production, neurotransmission, and vasodilation.42,43 Conversely, reactive oxygen species show cytotoxicity when overproduced. Reactive oxygen species remained low when taxifolin was added to human lung embryonic fibroblasts.44 Taxifolin suppressed the production of reactive oxygen species and NO in leukocytes and microglia in a rat model of cerebral infarction, by inducing oxidative stress and inflammation.45 Based on these findings, taxifolin intake in the current study may function to suppress cytotoxicity because of the overproduction of reactive oxygen species due to the removal of foreign substances after CMTs.
Relationship between taxifolin and the brain
The relationship between peripheral blood gene expression and brain function has been reported in many psychiatric disorders.46,47 Gene expression in peripheral blood is be useful for elucidating depression.48,49 Similarities in gene expression between peripheral blood and the brain have also been reported,50–52 highlighting the usefulness of blood gene expression. Analysis of whole blood gene expression in this study using IPA suggested that taxifolin suppresses encephalitis. Oxidative stress loading increased serum NO levels in mice, but it is suppressed to control levels following taxifolin administration.53 Physiological concentrations of NO increase cerebral blood flow. Based on these results, taxifolin intake in this study may have reduced the load on the brain by the CMTs and increased cerebral blood flow by maintaining NO at physiological concentrations, a factor that might have improved the CMT results.
Relationship between taxifolin and immunity
In this experiment, a single intake of taxifolin was shown to affect whole blood gene expression. The results of the gene expression analysis inferred that the immune response of leukocytes was activated. In addition to reports demonstrating that taxifolin is related to immunity, such as having anti-inflammatory effects,54,55 recent studies have shown taxifolin to reduce the symptoms of COVID-19.11,56 Although this study was conducted under the load of CMTs, the process of suppressing protein synthesis, which is essential for virus particle replication, was strongly suppressed by taxifolin, suggesting that it may contribute to the suppression of virus growth. In addition, the innate immune relationship might be strengthened because the expression of genes that were enriched in granulocytes was increased.
Limitations
This study has some limitations. For example, students attending the medical school of the university participated in this study. All the participants belonging to the nursing department were females, which could have introduced bias. In the Japanese education system, medical students are more trained in mathematics as compared to nursing students. As no blood count was performed in this study, the change in blood cell numbers and the proportion of cell types were unknown. Therefore, it is unclear whether taxifolin intake increases neutrophils or regulated neutrophil gene expression. In addition, because this study was conducted on healthy young adults, the results may differ in children, the middle-aged, and the elderly.
Conclusions
This study revealed that taxifolin intake decreased the time required to provide responses to CMTs and affected the brain activity of healthy young adults. The load of the brain activity test conducted in this study did not increase blood cortisol, but taxifolin intake showed an antifatigue effect that reduced subjective fatigue. The results of the gene expression analysis of blood cells suggested that ingestion of a single intake might enhance the removal of foreign substances by strengthening the innate immune system and suppressing the occurrence of injury. Therefore, taxifolin-containing foods are expected to be useful to remove foreign substances from the body.
Author contributions
Conceptualization, F. S., A. K. and K. A.; data curation, F. S., A. K. and K. S.; formal analysis, F. S. and T. S.; funding acquisition, F. S., A. K. and K. A.; investigation, F. S., A. K., H. M. and T. S.; methodology, F. S. and A. K.; project administration, Y. N. and K. A.; resources, H. M., T. S., M. I., K. Y., T. O., N. K., S. T., Y. N.; supervision, K. A.; validation, F. S. and A. K.; visualization, F. S.; writing – original draft preparation, F. S., A. K. and K. A.; writing – review and editing, all members.
Conflicts of interest
The authors have nothing to disclose.
Acknowledgements
We thank the Research Institute for Nutrition and Aging Co., Ltd, for supplying the test food and the participants of this clinical trial. This study was funded by Advanced Innovation Development Co., Ltd and Research Institute for Nutrition and Aging Co., Ltd. This study was also supported by a research grant from JSPS KAKENHI Grant Numbers 18K05514 (to A. K.), 20K02401 (to F. S.), and 20H01623 (to K. A.). The funders had no role in the study design, data collection and analysis, decision to publish, or preparation of the manuscript.
References
- H. Cory, S. Passarelli, J. Szeto, M. Tamez and J. Mattei, The role of polyphenols in human health and food systems: A mini-review, Front. Nutr., 2018, 5, 87 CrossRef PubMed.
- A. B. Scholey, S. J. French, P. J. Morris, D. O. Kennedy, A. L. Milne and C. F. Haskell, Consumption of cocoa flavanols results in acute improvements in mood and cognitive performance during sustained mental effort, J. Psychopharmacol., 2010, 24, 1505–1514 CrossRef CAS PubMed.
- R. Baynham, J. J. C. S. Veldhuijzen van Zanten, P. W. Johns, Q. S. Pham and C. Rendeiro, Cocoa flavanols improve vascular responses to acute mental stress in young healthy adults, Nutrients, 2021, 13, 1103 CrossRef CAS PubMed.
- P. Philip, P. Sagaspe, J. Taillard, C. Mandon, J. Constans and L. Pourtau,
et al., Acute intake of a grape and blueberry polyphenol-rich extract ameliorates cognitive performance in healthy young adults during a sustained cognitive effort, Antioxidants, 2019, 8, 650 CrossRef CAS PubMed.
- D. O. Kennedy, P. A. Jackson, C. F. Haskell and A. B. Scholey, Modulation of cognitive performance following single doses of 120 mg Ginkgo biloba extract administered to healthy young volunteers, Hum. Psychopharmacol., 2007, 22, 559–566 CrossRef PubMed.
- A. Schmidt, F. Hammann, B. Wölnerhanssen, A. C. Meyer-Gerspach, J. Drewe and C. Beglinger,
et al., Green tea extract enhances parieto-frontal connectivity during working memory processing, Psychopharmacology, 2014, 231, 3879–3888 CrossRef CAS PubMed.
- A. G. Schauss, S. S. Tselyico, V. A. Kuznetsova and I. Yegorova, Toxicological and genotoxicity assessment of a dihydroquercetin-rich Dahurian larch tree (Larix gmelinii Rupr) extract (Lavitol), Int. J. Toxicol., 2015, 34, 162–181 CrossRef CAS PubMed.
- F. Topal, M. Nar, H. Gocer, P. Kalin, U. M. Kocyigit and I. Gülçin,
et al., Antioxidant activity of taxifolin: an activity-structure relationship, J. Enzyme Inhib. Med. Chem., 2016, 31, 674–683 CrossRef CAS PubMed.
- M. B. Plotnikov, O. I. Aliev, A. V. Sidekhmenova, A. Y. Shamanaev, A. M. Anishchenko and T. I. Fomina,
et al., Dihydroquercetin improves microvascularization and microcirculation in the brain cortex of SHR rats during the development of arterial hypertension, Bull. Exp. Biol. Med., 2017, 163, 57–60 CrossRef CAS PubMed.
- C. L. Yang, Y. S. Lin, K. F. Liu, W. H. Peng and C. M. Hsu, Hepatoprotective mechanisms of taxifolin on carbon tetrachloride-induced acute liver injury in mice, Nutrients, 2019, 11, 2655 CrossRef CAS PubMed.
- M. Ricke-Hoch, E. Stelling, L. Lasswitz, A. P. Gunesch, M. Kasten and F. J. Zapatero-Belinchón,
et al., Impaired immune response mediated by prostaglandin E2 promotes severe COVID-19 disease, PLoS One, 2021, 16, e0255335 CrossRef CAS PubMed.
- S. Saito, Y. Yamamoto, T. Maki, Y. Hattori, H. Ito and K. Mizuno,
et al., Taxifolin inhibits amyloid-β oligomer formation and fully restores vascular integrity and memory in cerebral amyloid angiopathy, Acta Neuropathol. Commun., 2017, 5, 26 CrossRef PubMed.
- Y. Wang, Q. Wang, X. Bao, Y. Ding, J. Shentu and W. Cui,
et al., Taxifolin prevents β-amyloid-induced impairments of synaptic formation and deficits of memory via the inhibition of cytosolic phospholipase A2/prostaglandin E2 content, Metab. Brain Dis., 2018, 33, 1069–1079 CrossRef CAS PubMed.
- T. Inoue, S. Saito, M. Tanaka, H. Yamakage, T. Kusakabe and A. Shimatsu,
et al., Pleiotropic neuroprotective effects of taxifolin in cerebral amyloid angiopathy, Proc. Natl. Acad. Sci. U. S. A., 2019, 116, 10031–10038 CrossRef CAS PubMed.
- F. Shinozaki, A. Kamei, K. Shimada, H. Matsuura, T. Shibata and M. Ikeuchi,
et al., Single administration of taxifolin—rich extract to young adults improves their sequential single task performance—A randomized, placebo—controlled, double—blind, crossover trial, Jpn. Pharmacol. Ther., 2022, 50, 93–107 Search PubMed.
- K. Morita, T. Saito, M. Ohta, T. Ohmori, K. Kawai and S. Teshima-Kondo,
et al., Expression analysis of psychological stress-associated genes in peripheral blood leukocytes, Neurosci. Lett., 2005, 381, 57–62 CrossRef CAS PubMed.
- U. M. Nater, T. Whistler, W. Lonergan, T. Mletzko, S. D. Vernon and C. Heimb, Impact of acute psychosocial stress on peripheral blood gene expression pathways in healthy men, Biol. Psychol., 2009, 82, 125–132 CrossRef PubMed.
- L. Bell, D. J. Lamport, L. T. Butler and C. M. Williams, A review of the cognitive effects observed in humans following acute supplementation with flavonoids, and their associated mechanisms of action, Nutrients, 2015, 7, 10290–10306 CrossRef CAS PubMed.
-
Japanese Society of Fatigue Science, Fatigue VAS inspection method, 2011, available from: https://www.hirougakkai.com/ [in Japanese] Search PubMed.
- D. W. Huang, B. T. Sherman and R. A. Lempicki, Systematic and integrative analysis of large gene lists using DAVID bioinformatics resources, Nat. Protoc., 2009, 4, 44–57 CrossRef CAS PubMed.
- Human protein atlas. Available from: https://www.proteinatlas.org.
- P. J. Thul, L. Åkesson, M. Wiking, D. Mahdessian, A. Geladaki and H. Ait Blal,
et al., A subcellular map of the human proteome, Science, 2017, 356, eaal3321 CrossRef PubMed.
- T. Bristow, C.-S. Jih, A. Slabich and J. Gunn, Standardization and adult norms for the sequential subtracting tasks of serial 3's and 7′s, Appl. Neuropsychol.: Adult, 2016, 23, 372–378 Search PubMed.
- D. J. Lamport, D. Pal, C. Moutsiana, D. T. Field, C. M. Williams and J. P. Spencer,
et al., The effect of flavanol-rich cocoa on cerebral perfusion in healthy older adults during conscious resting state: A placebo controlled, crossover, acute trial, Psychopharmacology, 2015, 232, 3227–3234 CrossRef CAS PubMed.
- S. J. Catchlove, T. B. Parrish, Y. Chen, H. Macpherson, M. E. Hughes and A. Pipingas, Regional cerebrovascular reactivity and cognitive performance in healthy aging, J. Exp. Neurosci., 2018, 12, 1179069518785151 Search PubMed.
- Y. Li, H. Su, Z.-P. Yin, J.-E. Li, E. Yuan and Q.-F. Zhang, Metabolism, tissue distribution and excretion of taxifolin in rat, Biomed. Pharmacother., 2022, 150, 112959 CrossRef CAS PubMed.
- Y. Nakagawa, K. Ishimura, S. Oya, M. Kamino, Y. Fujii, F. Nanba and T. Toda,
et al., Comparison of the sympathetic stimulatory abilities of B-type procyanidins based on induction of uncoupling protein-1 in brown adipose tissue (BAT) and increased plasma catecholamine (CA) in mice, PLoS One, 2018, 13, e0201203 CrossRef PubMed.
- G. Loers, D. V. Yashunsky, N. E. Nifantiev and M. Schachner, Neural cell activation by phenolic compounds from the Siberian larch (Larix sibirica), J. Nat. Prod., 2014, 77, 1554–1561 CrossRef CAS PubMed.
- A. Karabay, J. D. Saija, D. T. Field and E. G. Akyürek, The acute effects of cocoa flavanols on temporal and spatial attention, Psychopharmacology, 2018, 235, 1497–1511 CrossRef CAS PubMed.
- C. Dietz and M. Dekker, Effect of green tea phytochemicals on mood and cognition, Curr. Pharm. Des., 2017, 23, 2876–2905 CrossRef CAS PubMed.
- C. F. Haskell-Ramsay, R. C. Stuart, E. J. Okello and A. W. Watson, Cognitive and mood improvements following acute supplementation with purple grape juice in healthy young adults, Eur. J. Nutr., 2017, 56, 2621–2631 CrossRef CAS PubMed.
- O. N. Pozharitskaya, M. V. Karlina, A. N. Shikov, V. M. Kosman, M. N. Makarova and V. G. Makarov, Determination and pharmacokinetic study of taxifolin in rabbit plasma by high-performance liquid chromatography, Phytomedicine, 2009, 16, 44–251 CrossRef PubMed.
-
S. B. Seredin, V. P. Zherdev, G. B. Kolyvanov, A. A. Litvin, A. K. Sariev and O. Y. Kravtsova, Preclinical investigation of pharmacokinetics of dihydroquercetin. Report, Stanford University National Insurance Institute of Israel, Moscow, 2007 Search PubMed.
- P. J. Eslinger and A. R. Damasio, Severe disturbance of higher cognition after bilateral frontal lobe ablation: patient EVR, Neurology, 1985, 35, 1731–1741 CrossRef CAS PubMed.
- A. Bechara, A. R. Damasio, H. Damasio and S. W. Anderson, Insensitivity to future consequences following damage to human prefrontal cortex, Cognition, 1994, 50, 7–15 CrossRef CAS PubMed.
- H. Damasio, T. Grabowski, R. Frank, A. M. Galaburda and A. R. Damasio, The return of Phineas Gage: clues about the brain from the skull of a famous patient, Science, 1994, 264, 1102–1105 CrossRef CAS PubMed.
- S. S. Dickerson and M. E. Kemeny, Acute stressors and cortisol responses: a Theoretical Integration and synthesis of laboratory research, Psychol. Bull., 2004, 130, 355–391 Search PubMed.
- T. E. Dever, Gene-specific regulation by general translation factors, Cell, 2002, 108, 545–556 CrossRef CAS PubMed.
-
D. Ron and H. P. Harding, EIF2α phosphorylation in cellular stress responses and disease, in Translational control in biology and medicine, ed. M. B. Mathews, N. Sonenberg and J. W. B. Hershey, Cold Spring Harbor, Cold Spring Harbor Laboratory Press, New York, 2007, pp. 345–368 Search PubMed.
- C. Zhang, J. Zhan, M. Zhao, H. Dai, Y. Deng and W. Zhou,
et al., Protective mechanism of taxifolin for chlorpyrifos neurotoxicity in BV2 cells, Neurotoxicology, 2019, 74, 74–80 CrossRef CAS PubMed.
- G. Weiss and U. E. Schaible, Macrophage defense mechanisms against intracellular bacteria, Immunol. Res., 2015, 264, 182–203 CAS.
- M. Ohashi, T. Hirano, K. Watanabe, K. Katsumi, N. Ohashi and H. Baba,
et al., Hydrogen peroxide modulates synaptic transmission in ventral horn neurons of the rat spinal cord, J. Physiol., 2016, 594, 115–134 CrossRef CAS PubMed.
- N. Di Marzo, E. Chisci and R. Giovannoni, The role of hydrogen peroxide in redox-dependent signaling: homeostatic and pathological responses in mammalian cells, Cells, 2018, 7, 156 CrossRef CAS PubMed.
- M. Matsuo, N. Sasaki, K. Saga and T. Kaneko, Cytotoxicity of flavonoids toward cultured normal human cells, Biol. Pharm. Bull., 2005, 28, 253–259 CrossRef CAS PubMed.
- Y. H. Wang, W. Y. Wang, C. C. Chang, K. T. Liou, Y. J. Sung and J. F. Liao,
et al., Taxifolin ameliorates cerebral ischemia-reperfusion injury in rats through its anti-oxidative effect and modulation of NF-kappa B activation, J. Biomed. Sci., 2006, 13, 127–141 CrossRef CAS PubMed.
- J. Iga, S. Ueno, K. Yamauchi, S. Numata, S. Kinouchi and S. Tayoshi-Shibuya,
et al., Altered HDAC5 and CREB mRNA expressions in the peripheral leukocytes of major depression, Prog. Neuropsychopharmacol. Biol. Psychiatry, 2007, 31, 628–632 CrossRef CAS PubMed.
- E. Lin and S. J. Tsai, Genome-wide microarray analysis of gene expression profiling in major depression and antidepressant therapy, Prog. Neuropsychopharmacol. Biol. Psychiatry, 2016, 64, 334–340 CrossRef CAS PubMed.
- D. Pan, Y. Xu, L. Zhang, Q. Su, M. Chen and B. Li,
et al., Gene expression profile in peripheral blood mononuclear cells of postpartum depression patients, Sci. Rep., 2018, 8, 10139 CrossRef PubMed.
- A. Anand, K. Nakamura, J. M. Spielberg, J. Cha, H. Karne and B. Hu, Integrative analysis of lithium treatment associated effects on brain structure and peripheral gene expression reveals novel molecular insights into mechanism of action, Transl. Psychiatry, 2020, 10, 103 CrossRef PubMed.
- P. F. Sullivan, C. Fan and C. M. Perou, Evaluating the comparability of gene expression in blood and brain, Am. J. Med. Genet., Part B, 2006, 141, 261–268 CrossRef PubMed.
- S. H. Witt, W. H. Sommer, A. C. Hansson, C. Sticht, M. Rietschel and C. C. Witt, Comparison of gene expression profiles in the blood, hippocampus and prefrontal cortex of rats, In Silico Pharmacol., 2013, 1, 15 CrossRef PubMed.
- Y. Iturria-Medina, A. F. Khan, Q. Adewale and A. H. Shirazi, Alzheimer's Disease Neuroimaging Initiative. Blood and brain gene expression trajectories mirror neuropathology and clinical deterioration in neurodegeneration, Brain, 2020, 143, 661–673 CrossRef PubMed.
- Y. Zeng, J. Song, M. Zhang, H. Wang, Y. Zhang and H. Suo, Comparison of in vitro and in vivo antioxidant activities of six flavonoids with similar structures, Antioxidants, 2020, 9, 732 CrossRef CAS PubMed.
- M. Zhao, J. Chen, P. Zhu, M. Fujino, T. Takahara and S. Toyama,
et al., Dihydroquercetin (DHQ) ameliorated concanavalin A-induced mouse experimental fulminant hepatitis and enhanced HO-1 expression through MAPK/Nrf2 antioxidant pathway in RAW cells, Int. Immunopharmacol., 2015, 28, 938–944 CrossRef CAS PubMed.
- Z. Y. Zhan, M. Wu, Y. Shang, M. Jiang, J. Liu and C. Y. Qiao,
et al., Taxifolin ameliorate high-fat-diet feeding plus acute ethanol binge-induced steatohepatitis through inhibiting inflammatory caspase-1-dependent pyroptosis, Food Funct., 2021, 12, 362–372 RSC.
- A. Fischer, M. Sellner, S. Neranjan, M. Smieško and M. A. Lill, Potential inhibitors for novel coronavirus protease identified by virtual screening of 606 million compounds, Int. J. Mol. Sci., 2020, 21, 3626 CrossRef CAS PubMed.
|
This journal is © The Royal Society of Chemistry 2023 |
Click here to see how this site uses Cookies. View our privacy policy here.