DOI:
10.1039/D2FO02688K
(Review Article)
Food Funct., 2023,
14, 56-73
Targeting AMPK signaling by polyphenols: a novel strategy for tackling aging
Received
10th September 2022
, Accepted 8th December 2022
First published on 10th December 2022
Abstract
Aging is an inevitable biological process and is accompanied by a gradual decline of physiological functions, such as the incidence of age-related diseases. Aging becomes a major burden and challenge for society to prevent or delay the occurrence and development of these age-related diseases. AMPK is a key regulator of intracellular energy and participates in the adaptation of calorie restriction. It is also an important mediator of nutritionally sensitive pathways that regulate the biological effects of nutrient active ingredients. AMPK can limit proliferation and activate autophagy. Recent studies have shown that nutritional intervention can delay aging and lessen age-related diseases in many animal and even human models. Polyphenols function as a natural antidote and are important anti-inflammatory and antioxidant agents in human diets. Polyphenols can prevent age-related diseases because they regulate complex networks of cellular processes such as oxidative damage, inflammation, cellular aging, and autophagy, and have also attracted wide attention as a potential beneficial substance for longevity. In this review, we systemically summarized the progress of targeting AMPK signaling by dietary polyphenols in aging prevention. Polyphenols can reduce oxidative stress and inflammatory response, and maintain the steady state of energy. Polyphenols can also modulate sirtuins/NAD+, nutrient-sensing, proteostasis, mitochondrial function, autophagy and senescence via targeting AMPK signaling. Therefore, targeting the AMPK signaling pathway by dietary polyphenols may be a novel anti-aging strategy.
1 Introduction
Aging is a process of progressive and cumulative changes, accompanied by a gradual decline in functional integrity and homeostasis, with serious negative impacts on healthy human life. By 2050, the global population over 65 years of age is expected to reach 1.6 billion, and aging will become a major socio-economic problem in all countries.1 At the molecular and cellular levels, aging may involve many events, including impaired nutrition sensing, genome instability, telomere attrition, loss of protein homeostasis (proteostasis), epigenetic changes, mitochondrial dysfunction, cellular aging, stem cell exhaustion and communication of cell-to-cell changes (e.g., inflammation).2 Therefore, it is important to understand the influencing factors and mechanisms behind aging and to investigate effective preventive and therapeutic interventions.
5′-Adenosine monophosphate activated protein kinase (AMPK) is a key nutrient sensor for maintaining energy balance and cell survival.3,4 It is closely related to the prolongation of human lifespan, which has attracted researchers’ attention.5,6 AMPK is not only involved in fat production and decomposition, fatty acid oxidation, and triglyceride and cholesterol synthesis, but it also regulates glucose metabolism, protein synthesis, inflammation and the steady state of energy after phosphorylation.7–10 Aging progress is also closely related to the metabolic rate and is accompanied by a progressive down-regulation of AMPK activity; thus younger animals tend to have higher AMPK activity than older animals. Wan et al.11 found that a decrease in AMPK activity in the skeletal muscle of aged rats correlated with metabolic syndrome, suggesting that a decrease in AMPK activity may be associated with many aging-related diseases such as cardiovascular disorders and metabolic syndrome. Therefore, the AMPK signaling pathway is a key mediating nutrient-sensitive pathway and plays an important role in delaying aging.
Recent studies have shown that polyphenols are among the most important anti-inflammatory and antioxidant agents in the human diet and exert beneficial effects on healthy longevity and aging by counteracting the signaling pathways that contribute to the aging cascade.12,13 Interestingly, the AMPK signaling pathway is an important mediator in regulating the biological effects of polyphenols,14–16 which suggests that dietary polyphenols targeting the AMPK signaling pathway may be a new and promising aging strategy for delaying aging or prolonging healthy life in humans. Therefore, it is necessary to evaluate the effectiveness of dietary polyphenols for anti-aging interventions, identify relevant biomarkers, and explore aging biomarker datasets using dynamic artificial intelligence (AI) and wearable device-based strategies to provide a clearer evaluation for clinical trials.
This review examined relevant research findings over the past few decades. We emphasized the ability of dietary polyphenols in delaying the ageing process and promoting health and longevity. We discussed their potential to provide signaling network targets for improving aging-related dietary interventions, revealing the important role of dietary polyphenols in aging research by modulating the AMPK signaling pathway and its intracellular mechanisms.
2 Aging and anti-aging therapy
Aging is a natural decay process in which the functions of tissues and organs are gradually lost over time, including inflammation, macromolecular damage, protein breakdown, epigenetic changes, and the accumulation of senescent cells.17–19 The excessive secretions of pro-inflammatory cytokines, chemokines, and matrix metalloproteinases (MMPs) during aging are collectively referred to as age-related symptoms.20 Aging has usually been considered as a random and inevitable death process and is also the main variable risk factor for most chronic diseases such as Alzheimer's disease and Parkinson's disease, cancer, cardiovascular disease and type 2 diabetes.21,22 Therefore, the research and development of pharmacological and nutritional treatment solutions for delaying or preventing aging and age-related diseases has usually been at the forefront of the field of aging.
Nine characteristics of aging were identified, including genome instability, telomere loss, epigenetic changes, protein deposition networks, impaired nutrition sensing, mitochondrial dysfunction, cellular senescence, stem cell failure, and changes in intercellular communication.23 To address the effects of aging on the body, interventions targeting aging mechanisms such as nutritional sensory impairment, mitochondrial dysfunction, and cellular senescence can provide potential strategies for developing anti-aging therapeutic regimens.24–27 Despite years of intensive research, the exact mechanisms that control the aging process remain unknown. There are significant differences in the internal tissue level of aging individuals and between aging individuals, and there are also significant differences between normal biological aging and pathological biological aging. Many interventions can prolong a patient's life, including drug treatments, calorie restriction (CR), and genetic engineering. However, aging and eventual death are inevitable. Despite this, research into age-related diseases aimed at slowing, halting and even reversing the aging process has not stopped, and extending the healthy lifespan of humans remains the focus of anti-aging research.28,29 Researchers have been trying to find out which molecules and mechanisms are involved in the ageing process so that more corresponding drugs and sensible dietary supplements can be produced. Senescence of senescent cells or telomere attrition is an important cause of senescence, and therefore, anti-aging strategies by scavenging senescent cells and reducing the attrition of telomeres are also potential pharmacological strategies.30,31 Current research suggests that aging is primarily associated with nutrient sensing disorders, mitochondrial dysfunction, and cellular senescence,23 but there are many questions about how to reverse or delay aging, which needs to be further explored.
In recent years, the focus of anti-aging research has been expanded from endocrine signaling pathways to the effects on regulatory gene networks. Dietary polyphenols have been recommended for healthy life management. However, this requires more convincing evidence and objective results. In this paper, we review the possible nutritional and pharmacological interventions of dietary polyphenols through the AMPK signaling pathway aimed at discovering approaches to mitigate and/or delay the aging process and prolong health and longevity.
3 AMPK and aging
3.1 Structure of AMPK
AMPK is a serine or threonine protein kinase comprising a catalytic α subunit (two subtypes: α1 and α2), a regulatory β subunit (two subtypes: β1 and β2), and a regulatory γ subunit base (three subtypes: γ1, γ2 and γ3).32,33 Depending on the type of AMPK subunit, 12 heterotrimers can be formed. The catalytic function of AMPK is attributed to subunit α, while the regulatory function is completed by subunits γ and β. There are two conserved regions in the central region and the carbon-terminal region of the β subunit and the γ subunit is related to AMPK activation. AMPK belongs to the family of energy-sensing enzymes, and it senses the energy state of cells through allosteric activated phosphorylation and automatic activation.8 Therefore, molecular-level drugs that activate and regulate AMPK are potential anti-aging candidate targets.
3.2 Regulation and activation of AMPK
AMPK is a highly conserved cell energy sensor that is activated by adjusting the ratio of adenosine and adenosine triphosphate and adenosine. The activation of AMPK reduces the biosynthesis of macromolecules and increases catabolic processes, thereby coordinating energy requirements, cellular processes and energy availability.35,36 The AMPK signaling pathway plays an important role in the energy homeostasis of the entire body, as it is regulated by adipokines or cytokines released by adipocytes and is sensitive to signals from ghrelin hormones such as leptin and adiponectin.37 Some upstream kinases, including Ca2+ or calmodulin-dependent protein kinase β (CaMK β), serine/threonine kinase 11 (STK11), and transforming growth factor-β activated kinase 1 (TAK1), activate AMPK via phosphorylating the α subunit of AMPK.34 AMPK activation can maintain protein stability through phosphorylation of eIF2α and inhibition of mTOR, both of which lead to decreased protein synthesis and activation of autophagy. The phosphorylated AMPK can also be inactivated by protein phosphatases such as protein phosphatase 2A (PP2A), protein phosphatase 2Ca (PP2Ca) and protein phosphatase, Mg2+/Mn2+-dependent 1E (PPM1E).
Exercise and CR, as metabolic stressors, decrease the ratio of ATP in the cell, leading to AMPK activation. Once activated, APMK triggers ATP synthesis reactions, including glycolysis and fatty acid oxidation. Oxidative stress induced by hydrogen peroxide activates AMPK by increasing the phosphorylation of Thr172. In the inactive form, α-subunits interact with their own self-inhibition sites. This kinase can be directly activated by other kinases at the phosphorylation site of Thr172, such as liver kinase B1 (LKB1), Ca2+/CaMK β and TAK1.38 AMPK exhibits significant activity only when it is phosphorylated at Thr172. Interestingly, AMPK activation is also the best intracellular trigger for mTOR inhibition. Activated AMPK phosphorylates tuberous sclerosis complex-2 (TSC2) and inhibits human cAMP-response element binding protein (CREB) regulated transcription coactivator 1 (TORC1) activity, resulting in a decrease in S6 kinase (S6K) activity.39 Therefore, the crosstalk between AMPK and mTOR is the fundamental process of regulating energy sensing. In summary, the overall effect of AMPK on energy metabolism pathways is to open the catabolic pathways that produce ATP and close the anabolic pathways that consume ATP. These anabolic pathways play a key role in the synthesis of cholesterol, fatty acids, proteins and glycogen (Fig. 1).
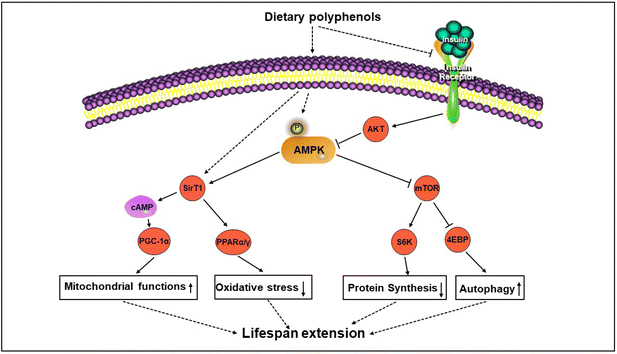 |
| Fig. 1 Antiaging mechanisms of dietary polyphenols are involved in AMPK signaling. AMPK, adenosine 5′-monophosphate-activated protein kinase; Akt: protein kinase B; PGC-1α: peroxisome proliferator-activated receptor gamma coactivator 1α; mTOR, mammalian target of rapamycin; PPARα/γ: peroxisome proliferator activated receptorα/γ; SIRT1: sirtuin 1; S6K: ribosomal S6 kinase; 4EBP: 4E-binding protein. | |
3.3 Role of the AMPK signaling pathway in aging
AMPK is a major metabolism-sensitive protein that acts on molecules through upstream and downstream regulatory actions. In mammals, the responsiveness of AMPK activation decreases with age. When the ratio of adenosine plus adenosine diphosphate to adenosine triphosphate is elevated, AMPK is activated to regulate long-term CR. Interestingly, current evidence suggests that AMPK activators reduce the risk of cardiovascular disease, certain common cancers and neurodegenerative diseases, and also improve metabolic syndrome, and may help prevent and control autoimmunity.40 The activation of AMPK can directly or indirectly regulate the functions of many transcription factors. The AMPK signaling pathway can stimulate sirtuin 1 (SIRT1), FoxO/DAF-16, and the nuclear factor (erythroid-derived 2)-like 2 (NFE2L2)/nuclear factor erythroid 2-related factor 2 (NRF2) pathway, enhancing anti-stress ability and prolonging lifespan. The downstream targets of AMPK (controlling energy metabolism, cell growth and autophagy) are actively sought to regulate its longevity effects.41 For example, in worms, the AMP-activated protein kinase (AAK)-2/AMPK signal prolongs lifespan by inhibiting the CRTC–CREB pathway.42 AMPK is the “node” where multiple metabolic pathways intersect. At the cellular level, AMPK activation maintains protein stability by phosphorylating eIF2α and UNC-51 to inhibit mTOR and activating UNC-51-like kinase 1 (ULK1) to activate autophagy.43 In addition, AMPK activation inhibits inflammation by inhibiting the nuclear factor kappa-B (NF-κB) signaling system.44 AMPK also blocks the activation of NF-κB signaling by inhibiting oxidative stress and endoplasmic reticulum stress.45 The AMPK signaling pathway can also inhibit the signal transducer and activator of transcription 3 (STAT3) indirectly.46 In addition, AMPK phosphorylated factors, including acetyl coenzyme A carboxylase (ACCS), sterol regulatory element binding protein 1 (SREBP1) and CREB co-activator TORC2 (CRTC2) are involved in energy metabolism.47,48 Therefore, the activation of AMPK is considered as a therapeutic target for anti-aging research, and it is one of the most promising methods in current successful anti-aging strategies.
In short, most of the physiological processes of the body are controlled by the AMPK signaling pathway to some extent. Therefore, active research on the role of AMPK in the non-cell-autonomous regulation of aging will be a very promising field soon. Despite the complexity, sensitivity and specificity of AMPK signaling, maintaining relative systems to control and protect AMPK throughout the life cycle may be one of the most important steps to prevent and treat aging.
4 Dietary polyphenols and aging
Polyphenols are the most common biologically active substances and plant-specific secondary metabolites, which are widely present in plant seeds, stems and other plant parts.49 They are divided into flavonoids and non-flavonoids, and are composed of glycosides and glycoconjugates. Polyphenols are structurally divided into hydroxybenzoic acid, hydroxycinnamic acid, flavonoids, astragalus and lignins. Among them, flavonoids are the most important type of polyphenol, which are divided into flavonols, flavonoids, isoflavones, flavonoids, anthocyanins and flavanols. Polyphenols have many important biological functions, such as anti-oxidation, scavenging free radicals, anti-cancer, anti-inflammatory, anti-allergic, anti-thrombosis and anti-microbial uses (Table 1).50
Table 1 Classes, chemical structures and the main sources of plant polyphenols
Classes |
Polyphenols |
Chemical structures |
Polyphenol source |
Phenolic acids (hydroxybenzoic acids) |
p-Hydroxybenzoic acids, gallic acid, vanillic acid, syringic acid, ellagic acid |
|
Berries, pineapples, pomegranate, walnuts, pecans, bananas, lemons and wines |
Phenolic acids (hydroxycinnamic acids) |
p-Hydroxycinnamic acids, p-coumaric acid, caffeic acid, ferulic acid, rosmarinic acid, sinapic acid, chlorogenic acid |
|
Coffee, kiwifruit, herbs, aubergine and cherry |
Flavonoids (flavones) |
Apigenin, luteolin, tangeritin, chrysin, 6-hydroxyflavone |
|
Grapefruit, parsley, pistachios, olive oil, onion, orange, tea and wheat |
Flavonoids (flavonols) |
Quercetin, myricetin, rhamnetin, isorhamnetin, kaempferol |
|
Onions, broccoli, apples, apricots, berries, nuts, seeds, tea, wine and cocoa |
Flavonoids (isoflavones) |
Soy glucosides, daidzin, genistein, soy flavonoids |
|
Soybeans, peanuts and lentils |
Flavonoids (flavanols) |
Condensed tannins, procyanidins, catechins, epicatechin, epigallocatechin gallate |
|
Red wine, cocoa, tea, nuts, apples and tropical fruit |
Stilbenes |
Resveratrol, pterostilbene |
|
Red wine, grapes, berries and peanuts |
Although aging is an inevitable process, biomedical research has revealed the key molecular pathways and key proteins of aging. They can regulate the development of therapeutic interventions at the molecular level to prevent or delay the occurrence of many age-related diseases. Surprisingly, natural polyphenols have become an effective tool for enhancing the identification of key factors/related to aging. So far, polyphenols targeting nutrition-sensing pathways may be the most promising candidate substances for anti-aging treatments. The researchers also found that polyphenols and a polyphenol-rich diet improved memory and cognitive function, suggesting that polyphenols may also be effective at preventing neurodegeneration diseases such as brain aging and dementia. Moreover, aging is associated with a gradual decline in physical and cognitive functions.51 In recent years, research on effective, non-toxic, and anti-aging natural polyphenols has been continuously promoted. Recent in vitro studies have shown that polyphenols can protect different cells from stress-induced senescence (SISP), delay cell replication and senescence, rejuvenate senescent cells, and have anti-aging effects on senescent cells.52 A diet rich in polyphenols can regulate many physiological processes involving cell functions and regulate the physiological integrity of gradual loss and damage to tissue and organ functions due to aging. As a powerful antioxidant, dietary plant polyphenols can block and eliminate the anti-inflammatory phenotype produced by the redox-regulated signaling pathway.
Polyphenols can also be used as epigenetic regulators of biological activity or high-efficiency autophagy inducers in vitro and in vivo to prevent aging and age-related diseases. Because of polyphenols’ inherent biocompatibility and safety, they have certain advantages compared to autophagy chemical inducers. For example, the anti-inflammatory properties of polyphenols can help resist aging and susceptibility to age-related diseases by reducing inflammation, destruction of the intestinal barrier, and neuroinflammation.53 Meanwhile, polyphenols can modulate epigenetic signals that lead to cancer progression or other potentially reversible age-related epigenetic modifications.54 Through integrating epigenetic information and “omics” data sets from the genome, transcriptome, proteome, and metabolome, combined with machine learning algorithms, researchers will identify and predict whether individuals’ potential targeted biomarkers can be derived from diet benefitting from polyphenols, aiming to solve the treatment of aging and age-related diseases.
Clinical trials have confirmed that polyphenols can be considered as a potential treatment tool for healthy aging, either as part of the diet or as a separate supplement. Although oral bioavailability is low and the existing data are not comprehensive, most polyphenols have significant biological effects.55 Natural polyphenols or their molecular scaffolds provide a theoretical basis for anti-aging and many related diseases as nutritional drugs.
Taken together, appropriate intake of clinical doses of plant polyphenols can effectively prevent inflammation and aging and extend healthy lifespan in humans by exerting antioxidant effects, which opens up new drug pathways and potential interventions. The fountain of youth may be, in humans’ body, protected by natural polyphenols. Among the many biological functions of polyphenols, indirect evidence seems to show their potential role in regulating cell senescence, senescence and longevity.
5 Targeting AMPK by polyphenols in aging
Aging is a complex biological process characterized by decreased functions of tissues, cells and organs and increased risk of death. To reduce the burden of disease and extend healthy lifespan, we should delay aging and improve healthy lifespan. Due to polyphenols’ great potential, they play a key role in regulating aging changes. Understanding the signal pathways between polyphenols and aging will provide deeper insight into the development of new methods that affect lifespan and aging, and may ultimately provide solutions for aging and age-related diseases. Polyphenolic compounds found in natural sources can be used to activate AMPK.56 Further research may discover the potential use of polyphenols as drug interventions for specific age-related disorders.57 Polyphenols are a superfamily of natural phytochemicals with highly diverse structures and abundant sources that can provide promising substances for major medical challenges in increasing health longevity or delaying aging. Diet plays an important role in mammalian health and in the prevention of cancer and chronic diseases. Asia's sirtuin-activating foods (sirtfoods) and polyphenols in the Mediterranean diet activating similar molecular targets, such as SIRT1, may be a promising dietary strategy for the prevention of aging, thereby ensuring healthy and regular aging.58 Polyphenols such as resveratrol, epigallocatechin gallate (EGCG) and curcumin are considered to have benefits for cardiovascular health and a protective effect in limiting cell aging. Several combinations of polyphenolic components may work synergically to counteract the burden of oxidative stress, influence signaling pathways to reduce the risks associated with cardiovascular disease and promote healthy aging.59
5.1 Polyphenols modulate homeostasis by targeting AMPK signaling
5.1.1 Reduced oxidative stress.
The most popular theory to explain the changes that occur during aging and lead to the gradual loss of cellular function is the accumulation of oxidative damage. The large-scale production of reactive oxygen species has a great impact on longevity. According to a theory of aging, oxidative stress and excessive production of active substances (free radicals, reactive oxygen species, reactive nitrogen, active electrophiles), especially at the mitochondrial level, can handle cellular senescence and age-related diseases. Damage caused by free radicals is a major cause of aging, which can be prevented or delayed by antioxidants, thus slowing down the aging process and improving health. According to the oxidative stress theory of aging, enhanced antioxidant defense plays an “anti-aging” role, leading to changes in longevity, age-related pathology and physiological functions.60 Excessive production of reactive oxygen species and insufficient storage of cellular antioxidants impair cell repair and metabolic homeostasis, which are mechanical switches for various senescence related pathways.61 Free radicals and oxidative stress have been recognized as important factors in the aging and many age-related degenerative diseases. Phytochemicals in fruits, vegetables, grains, and other foods can reduce the risk of oxidative stress-caused diseases. Therefore, one way to reduce the risk of aging and chronic diseases is to avoid the formation of free radicals and reduce oxidative stress by strengthening antioxidant defenses.62,63 Vitamin E could be used to accurately monitor individual functional antioxidant levels, which may be a promising key solution to fight against aging and age-related diseases.64 Oxidative stress is the main mechanism that limits lifespan, especially in healthy aging.
Normal cellular metabolism can also lead to the production of damaging free radicals such as ROS. If the expressions of antioxidant enzymes such as catalase, superoxide dismutase and glutathione peroxidase are reduced, it can promote the accumulation of reactive oxygen species, leading to DNA, lipid and protein damage. The accumulation of damaged molecules promotes the downregulation of mitochondrial function, which further exacerbates oxidative stress-induced damage, thereby promoting aging and increasing the incidence of age-related diseases.
Healthy dietary habits, including antioxidant intake, such as polyphenols, can positively influence the telomere length.65 Dietary plant polyphenols act as powerful antioxidants that block/eliminate anti-inflammation through modulating redox signaling pathways. For example, resveratrol can mediate antioxidant effects through AMPK and nicotinamide adenine dinucleotide (NAD+)-dependent deacetylase SIRT1, inhibiting the expression of senescence-associated secretory phenotype (SASP) factors secreted by senescent cells, thus contributing to an anti-aging effect.66 Moreover, SIRT1 can inhibit acetyl-CoA carboxylase and fatty acid synthase and prevent fatty acid mobilization and lipid accumulation in white adipocytes.67 Polyphenols are powerful antioxidants that trap and neutralize free radicals as direct antioxidants, preventing ROS-induced DNA, protein and lipid damage and blocking lipid peroxidation. They can also regulate many cellular signaling pathways that contribute to cell protection.
Resveratrol has high antioxidant activity and is also a high activator of SIRT1. Resveratrol can deacetylate several pro-inflammatory transcription factors, such as NF-κB and FoxO, and stimulate the SIRT1–AMPK–PGC-1α axis (PGC-1α, peroxisome proliferator-activated receptor γ-coactivator-1α) to suppress inflammation and autoimmune responses. Polyphenols can inhibit inflammation through activating SIRT1, suggesting that they may play active roles in preventing brain aging and improving cognition.68 Quercetin can resist oxidative stress through two such pathways, including the induction of Nrf2-ARE (ARE, an antioxidant response element) and antioxidant/anti-inflammatory enzyme paraoxonase-2 (Pon2). In addition, quercetin has been shown to activate silencing regulatory protein (SIRT1) and induce autophagy, which may provide its neuroprotective effect.69 At present, the clinical applications of polyphenols or polyphenols as auxiliary compounds to improve the efficacy of anti-aging treatments have feasibility, and need to be focused on.
5.1.2 Inflammatory response.
Chronic, low-grade inflammation is a hallmark of aging and involves several tissues (e.g. adipose tissue, muscle), organs (e.g. brain, liver), systems (e.g. immune system), and ecosystems (e.g. gut microbiota). As people grow older, most people will have a chronic low-grade proinflammatory state, their cells will appear SASP, and the number of senescent cells increases with the increase of age, and this is an important risk factor for various diseases, physical and cognitive impairment, frailty and death.70 The changes of immune functions and inflammatory pathways are the side factors of the aging process and the important conduction factors of tissue degeneration.71 Biological aging is characterized by chronic low levels of inflammation. This chronic phenomenon is known as “inflammatory aging” and is an important risk factor for morbidity and mortality in the elderly. The most common inflammatory aging theories include redox stress, mitochondrial dysfunction, glycosylation, immune system dysfunction, hormonal changes, epigenetic modifications, and telomere dysfunction.72
The accumulated data strongly suggest that due to age-related redox imbalance, chronic upregulation of pro-inflammatory mediators (e.g. tumor necrosis factor-α (TNF-α), interleukin-1β (IL-1β), IL-6, cyclooxygenase-2 (COX-2), and inducible nitric oxide synthase (iNOS)) during aging activates many pro-inflammatory pathways such as the NF-κB pathway due to age-related redox imbalance.73
The interaction between microbiome products, inflammatory mediators, and the immune system suggests that the regulation of the gut microbiome may contribute to the acceleration of physiological and non-pathological aging processes.74 Gut microbiomes are responsible for inducing the increase of proinflammatory markers to induce cellular inflammatory responses. Controlling or reducing inflammation represents a strategy for extending a healthy lifespan to avoid age-related diseases. AMPK inhibits the endoplasmic reticulum and oxidative stress, which are involved in metabolic disorders and the aging process. However, many of the proteins targeted by AMPK are so-called longevity factors, such as SIRT1, p53, and FoxOS, which not only increase stress resistance and prolong life in many organisms but also inhibit inflammatory responses. The activation of AMPK decreased during metabolic stress and with age, which may increase metabolic disease and accelerate the aging process.75
Inflammation can be considered as one of the main targets for developing anti-aging strategies. The primary source of inflammation is endogenous, altered, or misplaced molecules, resulting from cellular debris (damaged cells, dead cells, organelles) recognized by the innate immune system. Senescent cells (SCs) increase with age, and their secretory phenotype associated with aging promotes a chronic, inflammatory systemic state. Notably, since SCs spread inflammation at the systemic level through pro-oxidative and pro-inflammatory signals, foods rich in polyphenols have antioxidant and anti-inflammatory effects and may be used as “anti-aging foods” in nutritional supplements. The methods described above are the reasonable methods of ageing.76 However, among the recent approaches aimed at preventing inflammation and promoting healthy aging and longevity, dietary interventions with dietary polyphenols to eliminate senescent cells (aging therapy) and inflammatory body inactivation seem to be more effective.
Pathways and molecular mechanisms for improving longevity are controlled by behavioral, pharmacological and dietary factors, most of which remain unknown. Naturally occurring compounds, such as polyphenols, are potential anti-aging agents because of their ability to modulate several evolutionarily conserved markers of aging, including oxidative damage, inflammation, cellular senescence, and autophagy. Clinical trials and epidemiological studies have shown the importance of dietary polyphenols in reducing inflammation and oxidative stress, which may have positive effects in preventing, delaying, and improving many age-related chronic diseases. Due to their inherent natural biocompatibility and safety, polyphenols have advantages over autophagy chemical inducers.77 In recent years, due to their ability to regulate oxidative damage, inflammation, autophagy and intestinal microbiota, the potential anti-aging effects of polyphenols have attracted increasing attention from the scientific community. The effects of lemon polyphenol (LPP) on aging, such as age-related score, motor activity, cognitive function, and intestinal microbiome, were examined using senescence accelerated prone mice 1 (SAMP1) and senescence resistance mice 1 (SAMR1). LPP intake extended lifespan by approximately 3 weeks and reduced the increase in age-related scores (e.g. periocular lesions) and motor atrophy. Besides, the lifetime intake of LPP may have an anti-aging effect on phenotypes and the intestinal environment.78
There is growing evidence that plant polyphenols play a beneficial role in a hormone-like manner by activating adaptive stress response pathways and make the function of arousal fully applicable to the field of nutrition (Table 2).
Table 2 Anti-aging effects of dietary polyphenol compounds through modulation of AMPK activation and their molecular mechanisms
Compounds |
Experimental mode |
Anti-aging effects |
Molecular mechanism |
Ref. |
Plant polyphenols |
Mosquitoes |
Exacerbate AMPK-mediated autophagy, reduce the proliferation of mosquito midgut microbiota, and extend vector lifespan |
↑: AMPK, midgut autophagy |
13
|
↓: bacterial proliferation, TG |
Carnosol and curcumin |
Primary human dendritic cells |
Activate the cellular energy sensor AMPK, thereby inhibiting mTOR signalling in LPS-stimulated primary human dendritic cells. |
↑: AMPK, HO-1 |
14
|
↓: mTOR, ETC, ATP, ROS |
Berry and fruit polyphenols |
Apolipoprotein E deficient mice |
Reduce the size of atherosclerotic lesions, improve the lipid profile and reduce oxidative stress parameters |
↑: AMPK, eNOS, NO, SIRT1 |
58
|
↓: cellular lipid, oxidized LDL, SOD1, SOD2, GSR, Tnxrd1, PON1 |
Luteolin |
MCAO rat model |
Protects brain cells by affecting AMPK, mTOR and SIRT3 pathways |
↑: AMPK, SIRT3, p-mTOR, oxygen free radicals, SOD |
111
|
↓: p-AMPK, GFAP and Iba-1 positive glial cells |
Apple polyphenol extract |
HepG2 cells |
Alleviates palmitic and oleic acid-induced intracellular lipid accumulation while increasing autophagy, restoring lysosomal acidification, inhibiting lipid synthesis and slightly promoting fatty acid oxidation |
↑: AMPK, SIRT1, LKB1/AMPK pathway, TG, autophagy, ATG7 |
120
|
↓: mTOR |
|
HepG2 cells |
Alleviates lipid accumulation in free-fatty-acid-exposed HepG2 cells via activating autophagy mediated by SIRT1/AMPK signaling |
↑: SIRT1, LKB1/AMPK pathway, TG |
121
|
↓: mTOR |
Resveratrol |
Adult-inducible SIRT1 knockout mice; C2C12 myoblasts |
Increases phosphorylation of AMPK, induces mitochondrial biogenesis, increases ATP and NAD+ levels, and shifts toward more oxidative muscle fibers |
↑: AMPK, NAD(+) levels, PGC-1α, mitochondrial membrane potential, NRF-1, NRF-2, NDUFS8, SDHb, Uqcrc1, COX5b, ATP5a1 |
65
|
↓: LKB1 acetylation |
Senescent cells |
Modulates the expression of pro- and anti-apoptotic factors, neutralizes free radical species, affects mitochondrial functions, chelates redox-active transition metal ions, and prevents protein aggregation |
↑: AMPK, acetyl coenzyme A, LC3II/I ratio, NAD+ levels in skeletal |
76
|
↓: NF-κB, miRNA155, p62 |
Yeast and mice |
Potential induction of AMPK-mediated beneficial effects on longevity and aging |
↑: AMPK, sirtuins, SIRT1 |
88
|
↓: COX, p53 |
Caenorhabditis elegans, Drosophila melanogaster, and mice |
Increases lifespan through the regulation of oxidative stress, energy metabolism, nutrient sensing and epigenetics, primarily through the activation of sirtuin 1 |
↑: AMPK, SIRT1, LKB1, cellular NAD+ levels, cAMP, Ca2+ levels |
89
|
↓: ATP, mTOR, NF-κB, PDE |
Human aortic endothelial cells |
Significantly reduces the palmitic acid -induced endothelial ROS levels in human aortic endothelial cells and induces endothelial cell autophagy |
↑: AMPK |
122
|
↓: ROS, endothelial cell autophagy, mTOR |
EGCG, anthocyanin, resveratrol, phlopertin, spermidine, butyrate, and β-hydroxybutyrate are presented in senior-induced 3T3-L1 preadipocytes and modulate the pro-inflammatory SASP through the effect of NRF2 on SIRT3. Thus, targeted SIRT3-activating compounds such as EGCG may delay cell senescence and senescence-induced inflammatory processes.79 Polyphenols target nuclear factor-kappa B, the main driver of SASP, suggesting that they may play a role as anti-aging drugs. Resveratrol (RSV) reduces the levels of increased SASP, senescence related genetic markers (P53, P16, and P21), intracellular ROS levels, and the gene expression of enzymes that protect cells from oxidative damage (heme oxygenase 1 (HMOX1) and superoxide dismutase, extracellular (SOD3)).80 Understanding the molecular mechanisms of these protective effects may lead to better therapeutic strategies.
5.1.3 The steady state energy.
The main purpose of nutrition is to guide the right dietary principles to prevent and, when needed, treat nutritional deficiencies. However, when nutrients are insufficient or excessive, the body faces the problem of controlling the absorption and storage of nutrients. AMP activated protein kinase (AMPK) acts as an integrator and mediator of several pathways and processes linking energetics and longevity. When the energy supply is insufficient, the organism's responsibility is to delay aging and increase resistance to various age-related pathologies. Therefore, activating AMPK may regulate multiple longevity pathways to promote healthy aging, but revealing its full potential may require selective targeting of substrates related to longevity assurance.16 AMPK is a cellular energy sensor that monitors the ratio of adenosine-to-adenosine triphosphate. A decrease in ATP concentration results in increased adenosine levels and AMPK activation. Activated AMPK increases cellular ATP production and reduces ATP consumption by closing energy-consuming processes, such as mTOR dependent protein translation. After activation, AMPK opens the catabolic pathway for ATP production and close ATP consumption processes, including cell growth, biosynthesis and proliferation. As a result, the process described above promotes glycolysis and fatty acid oxidation for a short period of time, increases mitochondrial content and uses mitochondrial substrates as energy for a long period of time. Plant-derived polyphenols, such as carnosol and curcumin, induce and regulate human primary human dendritic cell (DC) metabolism and immune function through AMPK-dependent heme oxygenase. In addition, pharmacological inhibition of AMPK was found to reverse the observed reduction in DC maturation caused by carnosol and curcumin.15
Effective control of energy metabolic homeostasis, enhanced resilience to stress, and qualified cellular housekeeping are indicators of improved health and longevity. AMPK signals are involved in the regulation of all these characteristics through an integrated signal network. With age, the responsiveness of AMPK signals decreases significantly.5
Serine/threonine kinase AMPK is a key coordinator and controller of many molecular programs, including those that regulate cell metabolism (anabolism or catabolism), autophagy and protein stagnation, cell proliferation, and redox conditions.33 Conversely, AMPK activity is controlled by a variety of factors. As a regulator of cellular energy homeostasis, it is triggered by energy stress, which is guided by reduced ATP and increased AMP levels. AMPK is also activated by phosphorylation of two regulatory subunits by several kinases, including the energy-stress-sensitive LkB, protein kinase A (PKA), the glycogen synthase kinase 3β (CaMM-GSK 3β) axis, and other factors related to lipid metabolism and hypoxia, including PPARα.81
AMPK activation also improves glucose metabolism by promoting glucose transporter 4 transport to the cell membrane and lipid metabolism via peroxisome proliferator-activated receptor α (PPARα). Eventually, AMPK activation causes the inhibition of mTORC.82
As an energy sensor, AMPK is activated by low energy levels (e.g., a low ratio of ATP to adenosine diphosphate (ADP)). Activation of AMPK plays a critical role in inhibiting glucose production, increasing fat burning through β-oxidation, promoting mitochondrial biogenesis, and enhancing mitochondrial function.83
AMPK is an energy sensor that is activated when the levels of ATP in cells are too low. The ultimate role of autophagosomes is to fuse with lysosomes, where acidic proteases digest their contents and sustain cell growth by synthesizing new anabolite substrates (carbohydrates, amino acids, nucleic acids, and lipids).84
Typical adenine nucleotide-dependent and other atypical activation mechanisms activate AMPK when the cellular energy state is impaired, and once activated, AMPK restores energy homeostasis by promoting catabolic pathways, leading to ATP production and inhibiting the anabolic pathways that consume ATP.9 AMP activated protein kinase (AMPK) is a cellular energy sensor and signal converter regulated by a variety of metabolic stresses.85 AMP activated protein kinase (AMPK) is a heterotrimer αβγ complex that acts as a central regulator of energy homeostasis. Energy stress is manifested by a decrease in the ratio of ATP to AMP over ADP, which activates the kinase activity of AMPK and enables it to upregulate the catabolic pathways. ATP is produced in this process, reducing the energy-consuming catabolic pathways and cellular programs. AMPK senses the cellular energy state by strongly binding three adenine nucleotides AMP, ADP, and ATP to three sites in its γ subunit, each of which regulates AMPK activity in the kinase domain of its α subunit.86 The AMPK pathway is one of the most important pathways completely involved in cellular homeostasis and energy states. There is interesting evidence for the ability of H2O2 to modulate interactions with energy pathways through AMPK independent of the ratio of adenosine to triphosphate.
AMPK activator activated adenylate-activated protein kinase (AMPK) is an adaptive response to energy deficiency that regulates metabolism throughout the body. It senses the energy state of the cell and is activated when the ratio of adenosine to triphosphate increases. AMPK is the central regulator of energy homeostasis, coordinating metabolic pathways to balance nutrient supply and energy demand. Because of the metabolically beneficial physiological roles of AMPK activation, AMPK is considered an important therapeutic target for the control of human diseases, including metabolic syndrome and cancer. Therefore, the activator of AMPK may have potential as a new therapy for these diseases.87
Eukaryotic adenylate activated protein kinase (EAMPK) plays a major role in regulating cell energy balance. AMPK responds to changes in intracellular adenine nucleotide levels and is activated by an increase in the ratio of adenosine to ADP relative to ATP. AMPK activation increases the rate of catabolic pathways and decreases the rate of anabolic pathways. In addition to maintain intracellular energy balance, AMPK also regulates energy metabolism throughout the body. Currently, AMPK signaling activators are promising drug candidates for cancer treatment and extension of healthy life and longevity.88
AMPK appears to be another potential substrate for RSV activation. AMPK is a key enzyme that regulates cellular energy and controls energy homeostasis. RSV may induce AMPK-mediated beneficial effects on longevity and aging.89
The main challenge in studying ageing is to dissect the inter-relationships between candidate markers and their relative impact on ageing, thereby promoting future interventions and improving human health and longevity.23
5.2 Polyphenols modulate nutrient-sensing by targeting AMPK signaling
Mammalian AMPK is activated by a decline in the cellular energy state, represented by an increase in the ratio of AMP to ATP and ADP to ATP. Aging causes a variety of changes in biological processes, including energy metabolism and nutrient perception, which reduce cell proliferation and cause cell senescence. Among polyphenol phytochemicals, RSV is believed to reduce the negative effects of the aging process through its various biological activities.90 The longevity-promoting effects of future anti-aging drugs may be attributed to many interacting signaling pathways. Identifying these pathways and developing related anti-aging interventions appears to be a challenging task. Nutrients have a broad influence on cell physiology and homeostasis through signaling pathways that regulate the downstream response cascades of catabolism and anabolic homeostasis. Therefore, the nutrient-sensing pathway may be the most important in aging. In anti-aging strategies to slow the aging process and delay the onset of age-related diseases, the focus is on the nutrient sensing pathway (NSP) as a therapeutic target, although the molecular mechanisms that influence these processes may be influenced by different tissues.26,91 In addition, microRNAs have become important modulators of cell function and can be modified by diet. Some microRNAs target genes that encode proteins and enzymes belonging to nutrient sensing pathways, which may play a key role in regulating aging.92 Interventions that positively or negatively modulate nutrient sensing signaling pathways have been available clinically, highlighting the need for a full understanding of the role of nutrient signaling in aging and age-related diseases. Therefore, polyphenols may promote healthy aging through nutrient micromanagement and nutrient perception.
So far, the main anti-aging intervention has been intake nutrition. In particular, there has been a concerted focus on nutritional interventions to delay aging, particularly CR and intermittent fasting. The mechanism of the effect of CR on aging has been extensively studied. The focus of the research is on nutrient sensing pathways (mTOR, sirtuins, AMPK, insulin-like growth factor gene 1 (IGF 1)/insulin) that respond to changes in circulating nutrients (amino acids, glucose) or intracellular metabolites (NAD+, AMP) that reflect the energy supply of the cell.93 Studies should focus on intermittent fasting or certain nutrient-limited diets, and active ingredients that target these anti-aging dietary effects. By eliminating senescent cells or preventing aging such as telomere shortening has become a potential anti-aging strategy.
Dietary restriction (DR), usually an arbitrary reduction of 20%–40% or “normal” nutritional energy intake, has been reported to extend the lifespan of a variety of organisms, including yeast, nematodes, spiders, fruit flies, mice, rats, and rhesus monkeys.94 CR improves health and survival in rhesus monkeys.95
Clinical and animal studies clearly show that regulating the diet and meal frequency, as well as the application of fasting patterns, such as intermittent, regular or long-term fasting, extends life and healthy longevity and enhances intrinsic defenses against oxidative and metabolic stress. It also improves cognitive performance and reduces the cardiovascular risk of obesity and obese people.96
The most persuasive and widely studied intervention is CR. CR, reducing calorie intake without causing malnutrition, is one of the best ways to extend the lifespan of model organisms and resist age-related diseases. To date, the most promising candidate compounds for anti-aging therapies and the prevention/treatment of age-related diseases are also targeting the following pathways discussed below. Polyphenols affect specific cellular signaling pathways regulated by CR (such as the AMPK signaling pathway) and are implicated in the anti-aging process in humans. CR, often considered a quantitative and the most widely studied intervention, has been shown to delay aging and increase healthy lifespan and longevity in many species.97 CR is an established model for studying the biological mechanism of nutrition and aging. Although it is difficult to implement in the body, CR is the most promising anti-aging intervention. Healthy aging and human longevity are complex phenotypes influenced by environmental factors, such as physical exercise, diet, health habits and psychosocial status, and genetic factors. Diet and CR play a crucial role in healthy aging. In recent years, the control of molecular mechanisms associated with age-related progression through external intervention of polyphenols (an epigenetic regulation of diet) has received great attention. Natural products regulate cell longevity through post-translational modifications of histones and can also induce the upregulation of autophagy, reducing acetyl coenzyme A (AccoA) levels. In addition, the effects of CR on cancer-related chronic inflammation are of important significance for aging.98
The “Mediterranean and Asian” diet combining sirtfoods may be a promising dietary strategy to prevent chronic diseases, ensuring health and healthy aging.99 To date, CR appears to be the most effective intervention strategy for improving mitochondrial renewal in aging organisms. Polyphenols commonly found in fruits and vegetables have emerged as well-tolerated simulators of Cr targeting mitochondrial renewal.100 RSV activates sirtuins from Caenorhabditis elegans (C. elegans) and Drosophila melanogaster and extends the lifespan of these animals without reducing fecundity.101
In summary, the increase in life expectancy increases the incidence of age-related diseases beyond social and economic sustainability. Therefore, there is an urgent need for interventions that can reverse or at least prevent pathogenic age-related deterioration. Permanently or regularly reducing calorie intake without malnutrition (CR and fasting) is the only strategy to reliably extend a healthy lifespan in mammals (including non-human primates). Dietary polyphenols as calorie restriction simulators (CRMs) will become an important part of the pharmacological arsenal against aging.
DR refers to a broader dietary intervention aimed at reducing the consumption and feeding patterns of a particular number of nutrients, while CR refers to a 20–40% reduction in food consumption compared to normal intake.102 Reduced calorie intake causes significant metabolic changes, such as stimulation and fat mobilization, ketogenesis, and gluconeogenesis.
Both insulin and organismal metabolism pathways are nutrient and energy-dependent signaling pathways, and they are closely associated with AMPK. AMPK is a major nutrient sensor that acts as a downstream element in life-prolonging interventions, such as dietary restriction, to control the physiological processes of cells and aging. The most powerful and widely studied intervention is CR, and protein restriction and restriction of various amino acids (methionine, tryptophan) have also been shown to delay aging. However, the above methods’ major effect of aging is whether secondary to calorie intake, protein intake or specific amino acids is still a debate. Nutritional geometry provides a new perspective on the relationship between nutrition and aging by focusing on calories, macronutrients and their interactions throughout the dietary domain and considering compensatory feeding in a haphazard feeding experiment.103 Nutritional geometry is a state-space modeling approach that explores how animals respond to and balance changes in nutrient availability. Such studies in insects and mice have shown that a low-protein, high-carbohydrate diet is associated with the longest lifespan in randomly fed animals.
In addition, DR is one of the main experimental paradigms for studying the mechanism of longevity and aging. The emergence of the Nutritional Geometry Framework (GFN) has refocused interest from calories to dietary macronutrients.104
GFN experiments show that longevity and fertility are separated in the trophic space, causing a functional trade-off between the two traits.105 Nutritional geometry provides a new perspective on the relationship between nutrition and aging.
5.3 Polyphenols modulate proteostasis by targeting AMPK signaling
The protein homeostasis (or protein homeostasis) network is a key homeostasis system that regulates protein biosynthesis, folding, assembly, transportation, aggregation/depolymerization, and degradation to maintain proteome integrity. However, the ability of the protein-deposition network gradually decreases with aging, leading to many age-related diseases. PGC-1α has been widely described as a major regulator of mitochondrial biogenesis. AMPK and SIRT1 have been described to directly influence the activity of PGC-1α through phosphorylation and deacetylation, respectively. Metabolic sensors such as AMPK and SIRT1 are gatekeepers of the activity of the mitochondrial master regulator PGC-1α. It is an important link in the metabolic homeostasis regulatory network.106 Therefore, understanding their mechanisms of action can guide public to identify and improve prevention and treatment strategies for aging.
Interference with protein deposition, particularly the ability to remove misfolded proteins or protein aggregates, can extend the healthy lifespan of invertebrates and mammals.107 AMPK triggers the expression of antioxidant enzymes, specifically HO-1, through NRF2, and improves the control of protein stasis through the direct activation of FoxO3 and ULK1 and the indirect activation of transcription factor EB (TFEB).
The AMPK signaling pathway is closely associated with protein homeostasis (increased protein stability and removal of damaged proteins through proteasomal degradation or autophagy), mitochondrial biogenesis (control of mitochondrial redox homeostasis), and prevention of cell senescence.33 It is known that both AMPK expression and its activation decrease with age, causing toxic effects on certain exogenous compounds and increased susceptibility to age-related diseases. Therefore, the aging process and/or chronic diseases can be slowed down by modulating this signaling pathway.
5.4 Polyphenols modulate mitochondrial by targeting AMPK signaling
Impaired mitochondrial function has been linked to a variety of age-related diseases. Dysfunctional or impaired mitochondria are a major source of excess ROS production, which can increase genomic instability and trigger inflammation. They are important factors in cellular senescence, organ senescence, and age-related diseases. Therefore, they play a key role in the control of energy homeostasis at the cellular and systemic levels by regulating mitochondrial biogenesis and insulin sensitivity, and in autophagy and stress resistance processes. The decrease in AMPK activity associated with aging may be an important contributor to the decrease in mitochondrial function and dysregulation of intracellular lipid metabolism associated with aging.108 Notably, activation of PGC-1α (peroxisome proliferator-activated receptor γ-coactivator 1α), a major regulator of mitochondrial biogenesis, induces mitochondrial biogenesis and regulates energy metabolism. Interestingly, moderate inhibition or impairment of mitochondrial function extended the lifespan of a variety of model organisms, including worms, fruit flies, and mice. In general, restoration of mitochondrial homeostasis is considered as an important therapeutic strategy for promoting longevity and preventing age-related diseases.
Mitochondria can remain healthy by activating processes involved in the life-regulation pathway, including mitosis, mitochondrial division, and fusion. Reduced mitochondrial function is closely related to aging. Mitochondrial DNA damage (mtDNA) continues to be exposed to reactive oxygen species. Reduced mitochondrial function leads to decreased ATP synthesis through mitochondrial oxidative phosphorylation (OxPHOS), causing ROS production mainly in respiratory complexes I (NADH dehydrogenase) and III (ubiquitin-cytochrome C reductase).
AMPK regulates the expression of mitochondrial genes through SIRT1 and increases intracellular NAD+ levels, thereby modulating the PGC-1A coactivator activity of several stress-related pathways (such as FoxO3 and NF-κB) and PPAR. Therefore, AMPK may represent as a metabolic target that ameliorates mitochondrial dysfunction with age.
The protective effect of RSV on mitochondrial function has been well showed, and it is mainly mediated by the activation of SIRT1 and the subsequent deacetylation (activation) of PGC-1α, causing the improvement of mitochondrial biogenesis and function and the increase of antioxidant enzyme expression. RSV has been shown to extend the lifespan of mice on a high-calorie diet, which is associated with improved insulin sensitivity and an increased expression of AMPK and PGC-1α. In older subjects, resveratrol has also been shown to improve vascular function and mitochondrial quality.109
5.5 Polyphenols modulate sirtuins/NAD+ by targeting AMPK signaling
Sirtuins, direct homologues of yeast Sir2, are protein deacetylase that use NAD+ as a coenzyme to remove multiple protein acyl groups. In all the sirtuins, SIRT1 is one of the most widely studied in aging research.
AMPK is a major regulator of metabolic activity. Its activation enhances sirtuin activity.110 AMPK controls the expression of genes involved in the energy metabolism of skeletal muscle in mice by interacting with another metabolic sensor (NAD+ dependent type III deacetylase SIRT1). AMPK enhances SIRT1 activity by increasing cellular NAD+ levels, enabling active deacetylation and regulation of downstream SIRT1 targets. These include peroxisome proliferator-activated receptor-γ coactivator 1alpha and FoxO1 and FoxO3a transcription factors. AMPK-induced SIRT1-mediated deacetylation of these targets facilitates many of the converging biological effects of AMPK and SIRT1 on energy metabolism.111
Luteolin is a flavonoid with a variety of biological properties, including antioxidant activity, inhibition of apoptosis and regulation of autophagy. Luteolin enhances the scavenging of oxygen free radicals and the activity of SOD in mitochondria. In addition, it can enhance antioxidant capacity by reversing mitochondrial swelling and mitochondrial transmembrane potential. Luteolin can also increase the targeted expression of SIRT3 in mitochondria, decrease the phosphorylation of AMPK, and increase the level of phosphor-mTOR (p-mTOR).112 As a coenzyme, NAD+ not only plays an important role as an electron carrier in redox reactions, but also is necessary for enzymes such as sirtuins and poly(ADP-ribose) polymerases (PARPs). Therefore, changes in NAD+ levels have a wide range of effects on cell physiology.
Supplementation of NAD+ precursors promotes longevity and targeting NAD+ or SIRT1 may be a promising anti-aging intervention strategy. Dietary polyphenols act as CR mimetics associated with SIRT1 by modulating the ratio of NAD to NADH and the changes in mitochondrial respiratory activity. They may be involved in structural elements that regulate nicotinamide adenine dinucleotide phosphate (NADPH) oxidase activity and expression, and thus the most effective interventions for healthy aging include the AMPK signaling pathway.
5.6 Polyphenols modulate senescence by targeting AMPK signaling
Cellular senescence is a permanent state of cell cycle arrest induced by cellular stress, accompanied by the SASP. It has recently become a basic mechanism of aging. SASP-centric approaches are emerging as an alternative approach for targeting age-related diseases.113,114
However, DNA damage and oxidative stress that occur during aging can cause the accumulation of senescent cells, which have harmful effects on multiple organs and systemic functions through SASP. The aging process deteriorates body functions at multiple levels, causing a gradual decline in the ability to resist stress, injury and disease.115
By enforcing growth arrest, senescence limits the replication of old or damaged cells. In addition to exiting the cell cycle, senescent cells undergo many other phenotypic changes, such as metabolic reprogramming, chromatin rearrangement, or autophagy regulation.116 Strategies that reduce or eliminate senescent cells or their products may help slow aging. Senescent cells have been proposed as targets for interventions to delay senescence and its related diseases or to improve the treatment of diseases. Therapeutic interventions with senescent cells may help restore health and cure diseases that share basic processes, rather than curing each disease in a separate and symptomatic manner. Aging is considered a dynamic process, which means the properties of senescent cells constantly evolve and depend on the cell type. The most common characteristics of senescent cells are enlarged and flattened morphology, less regular shape, larger nuclei, single and larger nucleoli, more vacuoles in the cytoplasm, accumulation of lipofuscin in their lysosomes, increased ROS, and increased lysosomal activity. One of the key drivers of aging is cellular senescence, which is a state of irreversible growth arrest induced by many pro-tumor stresses. Senescent cells accumulate at the late stage of life and at the site of age-related lesions, causing the onset and progression of disease through complex cellular and acellular autonomic effects. Cellular senescence is a state of cell cycle stagnation, accompanied by the inhibition of apoptosis and the secretion of a variety of bioactive factors (such as SASP), which plays an important physiological role in multiple organs and systemic functions. A key feature of cellular senescence is stagnation of the cell cycle, activated by age-related stress, such as telomere shortening and oxidative stress.
Excessive accumulation of senescent cells plays an important role in the development of age-related diseases and other age-related morphological and physiological changes. Therefore, the goal of many researchers is to find a way to eliminate senescent cells and improve the health of older people.
Senescent cells are resistant to apoptosis and exhibit SASP, which includes secretion of various pro-inflammatory cytokines, chemokines, proteases, and growth factors. Senescent cells are more abundant in tissues that suffer from a variety of age-related diseases. Therefore, the use of dietary polyphenols to selectively target these senescent cells could be a promising strategy for anti-aging treatment or improving healthy longevity.
Natural polyphenolic products such as astragalus, flavonoids, and chalconone were shown to be effective at ameliorating a variety of age-related phenotypes, including oxidative stress, inflammation, impaired protein homeostasis, and cell senescence in vitro and in vivo.117
Resveratrol attenuates the typical aging changes of cell morphology, senescence-related β-galactosidase activity and cell proliferation. The mechanisms involved in this anti-aging effect seem to be independent of the regulation of senescence related genes and proteins but depend on the improvement of protein homeostasis in cells.118
Of the 10 flavonoids tested, fisetin was the most effective anti-aging agent. Acute or intermittent treatment with fisetin in presenile and elderly mice reduced the markers of aging in multiple tissues. Fisetin administration at a late stage of life in wild-type mice restored tissue homeostasis, reduced age-related pathology, and extended mean and maximum lifespan.119
The senescent cells exhibit metabolic imbalance, which is associated with reduced glycolysis and significantly reduced levels of ribonucleotide triphosphate (including ATP). The depletion of adenosine triphosphate causes an increase in cellular adenosine, which activates AMPK and contributes to the establishment of aging.
In a word, getting proper nutrition by harnessing the hormonal effects of dietary polyphenols is an exciting finding and there are still many unexplored areas in the prevention of ageing.
5.7 Polyphenols modulate autophagy by targeting AMPK signaling
Autophagy is a process in which defective or damaged cell components are transferred to lysosomes for degradation and recycling. Autophagy is a lysosomal degradation process that plays a central role in cell quality control by removing waste or excess proteins and organelles. Impairment of autophagy and an age-related decline in this pathway contribute to the pathogenesis of many diseases. Recent studies have suggested that the benefits of consuming polyphenols may also be related to autophagy induction.99 Mammalian experiments have shown that AMPK controls autophagy through mTOR and ULK1 signals, thus improving the quality of cell internal processing. EGCG plays a new role in promoting cell survival by altering the balance of the mTOR–AMPK pathway during ER stress. EGCG can also delay the apoptotic cell death by up-regulating autophagy-dependent survival.121 Apple polyphenol extract (APE) upregulated the expression of SIRT1, activated the LKB1/AMPK pathway and inhibited mTOR signaling. Overexpression or knockdown of SIRT1 positively modulates the expression of AMPK and anti-thymocyte globulin 7 (ATG7). APE treatment eased palmitic and oleic acid-induced intracellular lipid accumulation, restored lysosomal acidification with increased autophagy, inhibited lipid synthesis and slightly promoted fatty acid oxidation.122 The addition of polyphenols to the mosquito diet promoted the activation of AMPK. AMPK positively regulates midgut autophagy, enabling reduced bacterial proliferation and prolonged carrier life. Inhibition of AMPK activity caused a 6-fold increase in the midgut microbiota.14 RSV significantly reduced the level of PA-induced endothelial ROS in human aortic endothelial cells. It also induces endothelial autophagy, which mediates RSV's effect on ROS reduction. Resveratrol stimulates autophagy through the AMPK–mTOR pathway.123 Today, the health benefits of dietary polyphenols are becoming more apparent with the prevalence of overweight and obesity and the dramatic increase in the number of people suffering from chronic diseases worldwide. With the identification of new dietary polyphenols and the improvement of polyphenols’ separation technology, it will help to prolong people's life and bring great benefits to human health.120
6 Conclusions and perspectives
Aging is a growing health problem driven by different molecular pathways and events including environmental and genetic factors. It affects multiple systems and organs of the body and requires management strategies focusing on healthy aging and disease-free phenotypes to reduce additional healthcare burdens. Therefore, the study of the specific molecular mechanisms of individual aging highlights the importance of key signaling regulators and intercellular signaling pathways. The AMPK pathway has attracted extensive attention in delaying aging and promoting healthy longevity, and it is an important target and the most promising way to develop anti-aging drugs. The AMPK signaling pathway interacts with a variety of other signaling pathways to integrate multiple upstream and downstream pathways. Lifespan processes are affected by controlling cell proliferation, nutrient and energy flow, cell survival and degeneration. Dietary polyphenols have shown great potential in the prevention, improvement, and intervention of aging and age-related diseases, and they play an active role in aging by regulating the expression and function of the AMPK signaling pathway involved in aging. Polyphenols such as resveratrol, quercetin, and curcumin have shown the potential to modulate AMPK through different mechanisms.
Although many natural polyphenols are being investigated as possible modulators of the AMPK pathway, it remains unclear whether extensive long-term clinical trials are required to determine the long-term benefits of natural polyphenols in delaying aging and age-related diseases. Future clinical studies evaluating the relationship between polyphenols, aging, and AMPK signaling could enrich the knowledge of aging research strategies through epidemiological studies and clinical trials, as well as promising epigenetic and interaction tools, and thus, reveal the true significance of dietary polyphenols as anti-aging compounds in humans.
A better perspective is provided by understanding the contribution of signaling to the health benefits of polyphenols in delaying aging and promoting human health and longevity. Furthermore, with the aim of revealing the specific roles of natural polyphenols in aging and AMPK signaling, we understood the key pathways of aging in greater detail than ever before, with more targets and a richer source of natural dietary polyphenols to better develop the next generation of anti-aging treatments.
In conclusion, dietary polyphenols play an important role in human daily life. Dietary patterns and specific nutritional supplements can play an important role in promoting human health and longevity, paving the way for longer lifespans and slower onset of age-related diseases.
Abbreviations
AMPK | 5′ adenosine monophosphate activated protein kinase |
AI | Artificial intelligence |
CaMK β | Calmodulin-dependent protein kinase β |
STK11 | Serine/threonine kinase 11 |
TAK1 | Transforming growth factor-β activated kinase 1 |
mTOR | The mammalian target of rapamycin |
eIF2α | Eukaryotic translation initiation factor 2α |
PP2Ca | Protein phosphatase 2Ca |
PPM1E | Protein phosphatase, Mg2+/Mn2+ dependent 1E |
ATP | Adenosine triphosphate |
AMP | Adenosine monophosphate |
ADP | Adenosine diphosphate |
Thr172 | Phospho-AMPKα |
LKB1 | Liver kinase B1 |
TSC2 | Tuberous sclerosis complex-2 |
TORC1 | CREB regulated transcription coactivator 1 |
S6K | S6 kinase |
FoxO1/O3 | Forkhead box O1/O3 |
SIRT | Sirtuin |
NFE2L2 | Nuclear factor (erythroid-derived 2)-like 2 |
NRF2 | Nuclear factor erythroid 2-related factor 2 |
AAK | AMP-activated protein kinase |
CREB | cAMP-response element binding protein |
ULK1 | UNC-51-like kinase 1 |
NF-κB | Nuclear factor kappa-B |
STAT | Signal transducer and activator of transcription |
ACCS | Acetyl coenzyme A carboxylase |
SREBP1 | Sterol regulatory element binding protein 1 |
CRTC2 | CREB co-activator TORC2 |
SISP | Stress-induced senescence |
EGCG | Epigallocatechin gallate |
ROS | Reactive oxygen species |
NAD+ | Nicotinamide adenine dinucleotide |
SASP | Senescence associated secretory phenotype |
PGC-1α | Peroxisome proliferator-activated receptor γ-coactivator-1α |
ARE | Antioxidant responsive element |
PON2 | Paraoxonase-2 |
TNF-α | Tumor necrosis factor-α |
IL-1 | Interleukin-1 |
COX-2 | Cyclooxygenase-2 |
iNOS | Inducible nitric oxide synthase |
SCs | Senescent cells |
LPP | Lemon polyphenol |
RSV | Resveratrol |
HMOX1 | Heme oxygenase 1 |
SOD3 | Superoxide dismutase, extracellular |
DC | Dendritic cell |
PKA | Protein kinase A |
GSK3β | Glycogen synthase kinase |
PPAR | Peroxisome proliferator-activated receptor |
NSP | Nutrient sensing pathway |
IGF1 | Insulin-like growth factor gene 1 |
DR | Dietary restriction |
CR | Calorie restriction |
AccoA | Acetyl coenzyme A |
GFN | Nutritional Geometry Framework |
TFEB | Transcription factor EB |
OxPHOS | Oxidative phosphorylation |
PARPs | Poly(ADP-ribose) polymerases |
NADPH | Nicotinamide adenine dinucleotide phosphate |
APE | Apple polyphenol extract. |
Author contributions
Wei Xu: conception and writing; Yi Luo, Jiaxin Yin and Mengzhen Huang: review and editing; and Feijun Luo: funding acquisition and supervision.
Conflicts of interest
No potential conflict of interest was reported by the authors.
Acknowledgements
This work was financially supported by the Key Project of State Key R & D Program, China (No.2022YFF1100200), and it was also supported by the Program for Science & Technology Innovation Platform of Hunan Province (2019TP1029), the Graduate Innovative Research Project of Hunan Province and Central South University of Forestry and Technology [Grant Numbers CX20200699 and CX20201018].
References
- P. Brivio, M. S. Paladini, G. Racagni, M. A. Riva, F. Calabrese and R. Molteni, From healthy aging to frailty: In search of the underlying mechanisms, Curr. Med. Chem., 2019, 26, 3685–3701 CrossRef CAS PubMed.
- P. P. Singh, B. A. Demmitt, R. D. Nath and A. Brunet, The genetics of aging: A vertebrate perspective, Cell, 2019, 177, 200–220 CrossRef CAS PubMed.
- D. Kazyken, B. Magnuson, C. Bodur, H. A. Acosta-Jaquez, D. Zhang, X. Tong, T. M. Barnes, G. K. Steinl, N. E. Patterson, C. H. Altheim, N. Sharma, K. Inoki, G. D. Cartee, D. Bridges, L. Yin, S. M. Riddle and D. C. Fingar, AMPK directly activates mTORC2 to promote cell survival during acute energetic stress, Sci. Signaling, 2019, 12(585), eaav3249 CrossRef CAS PubMed.
- F. M. Russell and D. G. Hardie, AMP-activated protein kinase: Do we need activators or inhibitors to treat or prevent cancer?, Int. J. Mol. Sci., 2020, 22, 186 CrossRef PubMed.
- A. Salminen and K. Kaarniranta, AMP-activated protein kinase (AMPK) controls the aging process via an integrated signaling network, Ageing Res. Rev., 2012, 11, 230–241 CrossRef CAS.
- H. J. Weir, P. Yao, F. K. Huynh, C. C. Escoubas, R. L. Goncalves, K. Burkewitz, R. Laboy, M. D. Hirschey and W. B. Mair, Dietary restriction and AMPK increase lifespan via mitochondrial network and peroxisome remodeling, Cell Metab., 2017, 26, 884–896 CrossRef CAS PubMed.
- Q. Wang, S. Liu, A. Zhai, B. Zhang and G. Tian, AMPK-mediated regulation of lipid metabolism by phosphorylation, Biol. Pharm. Bull., 2018, 41, 985–993 CrossRef CAS.
- S. C. Lin and D. G. Hardie, AMPK: Sensing glucose as well as cellular energy status, Cell Metab., 2018, 27, 299–313 CrossRef CAS.
- D. G. Hardie, B. E. Schaffer and A. Brunet, AMPK: An energy-sensing pathway with multiple inputs and outputs, Trends Cell Biol., 2016, 26, 190–201 CrossRef CAS.
- C. L. Lyons and H. M. Roche, Nutritional modulation of AMPK-impact upon metabolic-inflammation, Int. J. Mol. Sci., 2018, 19(10), 3092 CrossRef PubMed.
- Q. Wan, W. Kang, Q. Zhu, P. Zhang and Y. Liu, Aging impairs insulin-stimulated glucose uptake in rat skeletal muscle via suppressing AMPKα, Exp. Mol. Med., 2007, 39, 535–543 CrossRef PubMed.
- H. Pazoki-Toroudi, H. Amani, M. Ajami, S. F. Nabavi, N. Braidy, P. D. Kasi and S. M. Nabavi, Targeting mTOR signaling by polyphenols: A new therapeutic target for ageing, Ageing Res. Rev., 2016, 31, 55–66 CrossRef CAS.
- A. Zia, T. Farkhondeh, A. M. Pourbagher-Shahri and S. Samarghandian, The role of curcumin in aging and senescence: Molecular mechanisms, Biomed. Pharmacother., 2021, 134, 111119 CrossRef CAS PubMed.
- R. D. Nunes, G. Ventura-Martins, D. M. Moretti, P. Medeiros-Castro, C. Rocha-Santos, C. R. Daumas-Filho, P. R. Bittencourt-Cunha, K. Martins-Cardoso, C. O. Cudischevitch, R. F. Menna-Barreto, J. H. Oliveira, D. S. Gusmao, F. J. Alves Lemos, D. S. Alviano, P. L. Oliveira, C. Lowenberger, D. Majerowicz, R. M. Oliveira, R. D. Mesquita, G. C. Atella and M. A. Silva-Neto, Polyphenol-rich diets exacerbate AMPK-mediated autophagy, decreasing proliferation of mosquito midgut microbiota, and extending vector lifespan, PLoS Neglected Trop. Dis., 2016, 10, e0005034 CrossRef.
- N. K. Campbell, H. K. Fitzgerald, J. M. Fletcher and A. Dunne, plant-derived polyphenols modulate human dendritic cell metabolism and immune function via AMPK-dependent induction of heme oxygenase-1, Front. Immunol., 2019, 10, 345 CrossRef CAS.
- K. Burkewitz, Y. Zhang and W. B. Mair, AMPK at the nexus of energetics and aging, Cell Metab., 2014, 20, 10–25 CrossRef CAS.
- K. H. Wagner, D. Cameron-Smith, B. Wessner and B. Franzke, Biomarkers of aging: From function to molecular biology, Nutrients, 2016, 8(6), 338 CrossRef PubMed.
- L. Partridge, J. Deelen and P. E. Slagboom, Facing up to the global challenges of ageing, Nature, 2018, 561, 45–56 CrossRef CAS PubMed.
- J. Campisi, P. Kapahi, G. J. Lithgow, S. Melov, J. C. Newman and E. Verdin, From discoveries in ageing research to therapeutics for healthy ageing, Nature, 2019, 571, 183–192 CrossRef CAS PubMed.
- S. Freitas-Rodriguez, A. R. Folgueras and C. Lopez-Otin, The role of matrix metalloproteinases in aging: Tissue remodeling and beyond, Biochim. Biophys. Acta, Mol. Cell Res., 2017, 1864, 2015–2025 CrossRef CAS PubMed.
- B. K. Kennedy, S. L. Berger, A. Brunet, J. Campisi, A. M. Cuervo, E. S. Epel, C. Franceschi, G. J. Lithgow, R. I. Morimoto, J. E. Pessin, T. A. Rando, A. Richardson, E. E. Schadt, T. Wyss-Coray and F. Sierra, Geroscience: Linking aging to chronic disease, Cell, 2014, 159, 709–713 CrossRef CAS.
- P. Song, J. An and M. H. Zou, Immune Clearance of senescent cells to combat ageing and chronic diseases, Cell, 2020, 9(3), 671 CrossRef CAS PubMed.
- C. Lopez-Otin, M. A. Blasco, L. Partridge, M. Serrano and G. Kroemer, The hallmarks of aging, Cell, 2013, 153, 1194–1217 CrossRef CAS PubMed.
- A. Bratic and N. G. Larsson, The role of mitochondria in aging, J. Clin. Invest., 2013, 123, 951–957 CrossRef CAS PubMed.
- S. B. Kritchevsky, Nutrition and healthy aging, J. Gerontol., Ser. A, 2016, 71, 1303–1305 CrossRef PubMed.
- A. Aiello, G. Accardi, G. Candore, C. M. Gambino, M. Mirisola, G. Taormina, C. Virruso and C. Caruso, Nutrient sensing pathways as therapeutic targets for healthy ageing, Expert Opin. Ther. Targets, 2017, 21, 371–380 CrossRef CAS PubMed.
- S. M. Robinson, Improving nutrition to support healthy ageing: What are the opportunities for intervention?, Proc. Nutr. Soc., 2018, 77, 257–264 CrossRef.
- D. G. L. Couteur and N. Barzilai, New horizons in life extension, healthspan extension and exceptional longevity, Age Ageing, 2022, 51(8), afac156 CrossRef PubMed.
- M. E. Ramaker, D. L. Corcoran, A. T. Apsley, M. S. Kobor, V. B. Kraus, W. E. Kraus, D. T. S. Lin, M. C. Orenduff, C. F. Pieper, R. Waziry, K. M. Huffman and D. W. Belsky, Epigenome-wide association study analysis of calorie restriction in humans, CALERIE TM Trial analysis, J. Gerontol., Ser. A, 2022 DOI:10.1093/gerona/glac168.
- D. Rysanek, P. Vasicova, J. N. Kolla, D. Sedlak, L. Andera, J. Bartek and Z. Hodny, Synergism of BCL-2 family inhibitors facilitates selective elimination of senescent cells, Aging, 2022, 14(16), 6381–6414 CrossRef.
- A. da Silva, B. K. S. Silveira, H. H. M. Hermsdorff, W. da Silva and J. Bressan, Effect of omega-3 fatty acid supplementation on telomere length and telomerase activity: A systematic review of clinical trials, Prostaglandins, Leukotrienes Essent. Fatty Acids, 2022, 181, 102451 CrossRef CAS PubMed.
- D. Carling, AMPK signalling in health and disease, Curr. Opin. Cell Biol., 2017, 45, 31–37 CrossRef CAS PubMed.
- S. Herzig and R. J. Shaw, AMPK: Guardian of metabolism and mitochondrial homeostasis, Nat. Rev. Mol. Cell
Biol., 2018, 19, 121–135 CrossRef CAS PubMed.
- A. Salminen, K. Kaarniranta and A. Kauppinen, Age-related changes in AMPK activation: Role for AMPK phosphatases and inhibitory phosphorylation by upstream signaling pathways, Ageing Res. Rev., 2016, 28, 15–26 CrossRef CAS PubMed.
- C. G. Langendorf and B. E. Kemp, Choreography of AMPK activation, Cell Res., 2015, 25, 5–6 CrossRef CAS PubMed.
- F. Francini, G. R. Schinella and J. L. Rios, Activation of AMPK by medicinal plants and natural products: Its role in type 2 diabetes mellitus, Mini-Rev. Med. Chem., 2019, 19, 880–901 CrossRef CAS PubMed.
- Y. Dagon, E. Hur, B. Zheng, K. Wellenstein, L. C. Cantley and B. B. Kahn, p70S6 kinase phosphorylates AMPK on serine 491 to mediate leptin's effect on food intake, Cell Metab., 2012, 16, 104–112 CrossRef CAS PubMed.
- M. Chen, N. Huang, J. Liu, J. Huang, J. Shi and F. Jin, AMPK: A bridge between diabetes mellitus and Alzheimer's disease, Behav. Brain Res., 2021, 400, 113043 CrossRef CAS.
- Y. Zhang, A. Lanjuin, S. R. Chowdhury, M. Mistry, C. G. Silva-Garcia, H. J. Weir, C. L. Lee, C. C. Escoubas, E. Tabakovic and W. B. Mair, Neuronal TORC1 modulates longevity via AMPK and cell nonautonomous regulation of mitochondrial dynamics in C. elegans, eLife, 2019, 8, e49158 CrossRef CAS PubMed.
- M. F. McCarty, AMPK activation–protean potential for boosting healthspan, Age, 2014, 36, 641–663 CrossRef CAS.
- R. Curtis, G. O'Connor and P. S. DiStefano, Aging networks in Caenorhabditis elegans: AMP-activated protein kinase (aak-2) links multiple aging and metabolism pathways, Aging Cell, 2006, 5, 119–126 CrossRef CAS.
- W. Mair, I. Morantte, A. P. Rodrigues, G. Manning, M. Montminy, R. J. Shaw and A. Dillin, Lifespan extension induced by AMPK and calcineurin is mediated by CRTC-1 and CREB, Nature, 2011, 470, 404–408 CrossRef CAS PubMed.
- J. Kim, M. Kundu, B. Viollet and K. L. Guan, AMPK and mTOR regulate autophagy through direct phosphorylation of Ulk1, Nat. Cell Biol., 2011, 13, 132–141 CrossRef CAS.
- A. Kauppinen, T. Suuronen, J. Ojala, K. Kaarniranta and A. Salminen, Antagonistic crosstalk between NF-kappaB and SIRT1 in the regulation of inflammation and metabolic disorders, Cell. Signalling, 2013, 25, 1939–1948 CrossRef CAS PubMed.
- V. O. Kaminskyy and B. Zhivotovsky, Free radicals in cross talk between autophagy and apoptosis, Antioxid. Redox Signal., 2014, 21, 86–102 CrossRef CAS PubMed.
- H. Gong, H. Tai, N. Huang, P. Xiao, C. Mo, X. Wang, X. Han, J. Zhou, H. Chen, X. Tang, T. Zhao, W. Xu, C. Gong, G. Zhang, Y. Yang, S. Wang and H. Xiao, Nrf2-SHP Cascade-Mediated STAT3 Inactivation Contributes to AMPK-Driven Protection Against Endotoxic Inflammation, Front. Immunol., 2020, 11, 414 CrossRef CAS PubMed.
- Y. S. Cho, J. I. Lee, D. Shin, H. T. Kim, H. Y. Jung, T. G. Lee, L. W. Kang, Y. J. Ahn, H. S. Cho and Y. S. Heo, Molecular mechanism for the regulation of human ACC2 through phosphorylation by AMPK, Biochem. Biophys. Res. Commun., 2010, 391, 187–192 CrossRef CAS PubMed.
- G. Zhou, R. Myers, Y. Li, Y. Chen, X. Shen, J. Fenyk-Melody, M. Wu, J. Ventre, T. Doebber, N. Fujii, N. Musi, M. F. Hirshman, L. J. Goodyear and D. E. Moller, Role of AMP-activated protein kinase in mechanism of metformin action, J. Clin. Invest., 2001, 108, 1167–1174 CrossRef CAS PubMed.
- V. Cheynier, F. A. Tomas-Barberan and K. Yoshida, Polyphenols: From plants to a variety of food and nonfood uses, J. Agric. Food Chem., 2015, 63, 7589–7594 CrossRef CAS PubMed.
- R. Tsao, Chemistry and biochemistry of dietary polyphenols, Nutrients, 2010, 2, 1231–1246 CrossRef CAS.
- S. Schaffer, H. Asseburg, S. Kuntz, W. E. Muller and G. P. Eckert, Effects of polyphenols on brain ageing and Alzheimer's disease: focus on mitochondria, Mol. Neurobiol., 2012, 46, 161–178 CrossRef CAS PubMed.
- J. Maria and Z. Ingrid, Effects of bioactive compounds on senescence and components of senescence associated secretory phenotypes in vitro, Food Funct., 2017, 8, 2394–2418 RSC.
- G. C. Roman, R. E. Jackson, R. Gadhia, A. N. Roman and J. Reis, Mediterranean diet: The role of long-chain omega-3 fatty acids in fish; polyphenols in fruits, vegetables, cereals, coffee, tea, cacao and wine; probiotics and vitamins in prevention of stroke, age-related cognitive decline, and Alzheimer disease, Rev. Neurol., 2019, 175, 724–741 CrossRef CAS PubMed.
- P. Selvakumar, A. Badgeley, P. Murphy, H. Anwar, U. Sharma, K. Lawrence and A. Lakshmikuttyamma, Flavonoids and other polyphenols act as epigenetic modifiers in breast cancer, Nutrients, 2020, 12(3), 761 CrossRef CAS PubMed.
- J. Bensalem, S. Dudonne, N. Etchamendy, H. Pellay, C. Amadieu, D. Gaudout, S. Dubreuil, M. E. Paradis, S. Pomerleau, L. Capuron, C. Hudon, S. Laye, Y. Desjardins and V. Pallet, Polyphenols from grape and blueberry improve episodic memory in healthy elderly with lower level of memory performance: A bicentric double-blind, randomized, placebo-controlled clinical study, J. Gerontol., Ser. A, 2019, 74, 996–1007 CrossRef CAS PubMed.
- J. T. Hwang, D. Y. Kwon and S. H. Yoon, AMP-activated protein kinase: A potential target for the diseases prevention by natural occurring polyphenols, New Biotechnol., 2009, 26, 17–22 CrossRef CAS.
- B. L. Queen and T. O. Tollefsbol, Polyphenols and aging, Curr. Aging Sci., 2010, 3, 34–42 CrossRef CAS PubMed.
- K. Pallauf and G. Rimbach, Autophagy, polyphenols and healthy ageing, Ageing Res. Rev., 2013, 12, 237–252 CrossRef CAS PubMed.
- S. Khurana, K. Venkataraman, A. Hollingsworth, M. Piche and T. C. Tai, Polyphenols: Benefits to the cardiovascular system in health and in aging, Nutrients, 2013, 5, 3779–3827 CrossRef CAS PubMed.
- A. B. Salmon, A. Richardson and V. I. Perez, Update on the oxidative stress theory of aging: Does oxidative stress play a role in aging or healthy aging?, Free Radicals Biol. Med., 2010, 48, 642–655 CrossRef CAS PubMed.
- F. Chen, Y. Liu, N. K. Wong, J. Xiao and K. F. So, Oxidative stress in stem cell aging, Cell Transplant., 2017, 26, 1483–1495 Search PubMed.
- M. Garrido, M. P. Terron and A. B. Rodriguez, Chrononutrition against oxidative stress in aging, Oxid. Med. Cell. Longevity, 2013, 2013, 729804 CAS.
- S. F. Vatner, J. Zhang, M. Oydanich, T. Berkman, R. Naftalovich and D. E. Vatner, Healthful aging mediated by inhibition of oxidative stress, Ageing Res. Rev., 2020, 64, 101194 CrossRef CAS PubMed.
- J. Luo, K. Mills, S. le Cessie, R. Noordam and D. van Heemst, Ageing, age-related diseases and oxidative stress: What to do next?, Ageing Res. Rev., 2020, 57, 100982 CrossRef CAS PubMed.
- M. Maleki, N. Khelghati, F. Alemi, M. Bazdar, Z. Asemi, M. Majidinia, A. Sadeghpoor, A. Mahmoodpoor, F. Jadidi-Niaragh, N. Targhazeh and B. Yousefi, Stabilization of telomere by the antioxidant property of polyphenols: Anti-aging potential, Life Sci., 2020, 259, 118341 CrossRef CAS PubMed.
- N. L. Price, A. P. Gomes, A. J. Ling, F. V. Duarte, A. Martin-Montalvo, B. J. North, B. Agarwal, L. Ye, G. Ramadori, J. S. Teodoro, B. P. Hubbard, A. T. Varela, J. G. Davis, B. Varamini, A. Hafner, R. Moaddel, A. P. Rolo, R. Coppari, C. M. Palmeira, R. de Cabo, J. A. Baur and D. A. Sinclair, SIRT1 is required for AMPK activation and the beneficial effects of resveratrol on mitochondrial function, Cell Metab., 2012, 15, 675–690 CrossRef CAS PubMed.
- Y. Zang, L. Fan, J. Chen, R. Huang and H. Qin, Improvement of lipid and glucose metabolism by capsiate in palmitic acid-treated HepG2 cells via activation of the AMPK/SIRT1 signaling pathway, J. Agric. Food Chem., 2018, 66, 6772–6781 CrossRef CAS PubMed.
- F. Sarubbo, S. Esteban, A. Miralles and D. Moranta, Effects of resveratrol and other polyphenols on sirt1: Relevance to brain function during aging, Curr. Neuropharmacol., 2018, 16, 126–136 CAS.
- L. G. Costa, J. M. Garrick, P. J. Roque and C. Pellacani, Mechanisms of neuroprotection by quercetin: Counteracting oxidative stress and more, Oxid. Med. Cell. Longevity, 2016, 2016, 2986796 Search PubMed.
- A. Bektas, S. H. Schurman, R. Sen and L. Ferrucci, Aging, inflammation and the environment, Exp. Gerontol., 2018, 105, 10–18 CrossRef CAS PubMed.
- J. Neves and P. Sousa-Victor, Regulation of inflammation as an anti-aging intervention, FEBS J., 2020, 287, 43–52 CrossRef CAS PubMed.
- B. Fougere, E. Boulanger, F. Nourhashemi, S. Guyonnet and M. Cesari, chronic inflammation: Accelerator of biological aging, J. Gerontol., Ser. A, 2017, 72, 1218–1225 CrossRef CAS PubMed.
- H. Y. Chung, M. Cesari, S. Anton, E. Marzetti, S. Giovannini, A. Y. Seo, C. Carter, B. P. Yu and C. Leeuwenburgh, Molecular inflammation: underpinnings of aging and age-related diseases, Ageing Res. Rev., 2009, 8, 18–30 CrossRef CAS PubMed.
- F. Mangiola, A. Nicoletti, A. Gasbarrini and F. R. Ponziani, Gut microbiota and aging, Eur. Rev. Med. Pharmacol. Sci., 2018, 22, 7404–7413 CAS.
- A. Salminen, J. M. Hyttinen and K. Kaarniranta, AMP-activated protein kinase inhibits NF-kappaB signaling and inflammation: impact on healthspan and lifespan, J. Mol. Med., 2011, 89, 667–676 CrossRef CAS PubMed.
- A. Serino and G. Salazar, Protective role of polyphenols against vascular inflammation, aging and cardiovascular disease, Nutrients, 2018, 11(1), 53 CrossRef PubMed.
- A. Yessenkyzy, T. Saliev, M. Zhanaliyeva, A. R. Masoud, B. Umbayev, S. Sergazy, E. Krivykh, A. Gulyayev and T. Nurgozhin, Polyphenols as caloric-restriction mimetics and autophagy inducers in aging research, Nutrients, 2020, 12(5), 1344 CrossRef CAS PubMed.
- C. Shimizu, Y. Wakita, T. Inoue, M. Hiramitsu, M. Okada, Y. Mitani, S. Segawa, Y. Tsuchiya and T. Nabeshima, Effects of lifelong intake of lemon polyphenols on aging and intestinal microbiome in the senescence-accelerated mouse prone 1 (SAMP1), Sci. Rep., 2019, 9, 3671 CrossRef PubMed.
- S. Lilja, J. Oldenburg, A. Pointner, L. Dewald, M. Lerch, B. Hippe, O. Switzeny and A. Haslberger, Epigallocatechin gallate effectively affects senescence and Anti-SASP via SIRT3 in 3T3-L1 preadipocytes in comparison with other bioactive substances, Oxid. Med. Cell. Longevity, 2020, 2020, 4793125 Search PubMed.
- D. Ali, L. Chen, J. M. Kowal, M. Okla, M. Manikandan, M. AlShehri, Y. AlMana, R. AlObaidan, N. AlOtaibi, R. Hamam, N. M. Alajez, A. Aldahmash, M. Kassem and M. Alfayez, Resveratrol inhibits adipocyte differentiation and cellular senescence of human bone marrow stromal stem cells, Bone, 2020, 133, 115252 CrossRef CAS PubMed.
- G. K. Cork, J. Thompson and C. Slawson, Real talk: The inter-play between the mTOR, AMPK, and hexosamine biosynthetic pathways in cell signaling, Front. Endocrinol., 2018, 9, 522 CrossRef PubMed.
- M. Grabacka, M. Pierzchalska, M. Dean and K. Reiss, Regulation of ketone body metabolism and the role of PPARalpha, Int. J. Mol. Sci., 2016, 17(12), 2093 CrossRef PubMed.
- F. R. Jornayvaz and G. I. Shulman, regulation of mitochondrial biogenesis, Essays Biochem., 2010, 47, 69–84 CrossRef CAS PubMed.
- R. C. Laker, J. C. Drake, R. J. Wilson, V. A. Lira, B. M. Lewellen, K. A. Ryall, C. C. Fisher, M. Zhang, J. J. Saucerman, L. J. Goodyear, M. Kundu and Z. Yan, Ampk phosphorylation of Ulk1 is required for targeting of mitochondria to lysosomes in exercise-induced mitophagy, Nat. Commun., 2017, 8, 548 CrossRef PubMed.
- K. Inoki, J. Kim and K. L. Guan, AMPK and mTOR in cellular energy homeostasis and drug targets, Annu. Rev. Pharmacol. Toxicol., 2012, 52, 381–400 CrossRef CAS PubMed.
- Y. Yan, X. E. Zhou, H. E. Xu and K. Melcher, Structure and physiological regulation of AMPK, Int. J. Mol. Sci., 2018, 19(11), 3534 CrossRef PubMed.
- J. Kim, G. Yang, Y. Kim, J. Kim and J. Ha, AMPK activators: Mechanisms of action and physiological activities, Exp. Mol. Med., 2016, 48, e224 CrossRef CAS PubMed.
- A. Salminen, A. Kauppinen and K. Kaarniranta, AMPK activation inhibits the functions of myeloid-derived suppressor cells (MDSC): impact on cancer and aging, J. Mol. Med., 2019, 97, 1049–1064 CrossRef CAS PubMed.
- A. F. Fernandez and M. F. Fraga, The effects of the dietary polyphenol resveratrol on human healthy aging and lifespan, Epigenetics, 2011, 6, 870–874 CrossRef CAS PubMed.
- I. S. Pyo, S. Yun, Y. E. Yoon, J. W. Choi and S. J. Lee, Mechanisms of aging and the preventive effects of resveratrol on age-related diseases, Molecules, 2020, 25(20), 4649 CrossRef CAS PubMed.
- N. M. Templeman and C. T. Murphy, Regulation of reproduction and longevity by nutrient-sensing pathways, J. Cell Biol., 2018, 217, 93–106 CrossRef CAS PubMed.
- V. Mico, L. Berninches, J. Tapia and L. Daimiel, NutrimiRAging: Micromanaging nutrient sensing pathways through nutrition to promote healthy aging, Int. J. Mol. Sci., 2017, 18(5), 915 CrossRef PubMed.
- M. Ros and J. M. Carrascosa, Current nutritional and pharmacological anti-aging interventions, Biochim. Biophys. Acta, Mol. Basis Dis., 2020, 1866, 165612 CrossRef CAS PubMed.
- A. R. Mendelsohn and J. W. Larrick, Dietary restriction: critical co-factors to separate health span from life span benefits, Rejuvenation Res., 2012, 15, 523–529 CrossRef PubMed.
- J. A. Mattison, R. J. Colman, T. M. Beasley, D. B. Allison, J. W. Kemnitz, G. S. Roth, D. K. Ingram, R. Weindruch, R. de Cabo and R. M. Anderson, Caloric restriction improves health and survival of rhesus monkeys, Nat. Commun., 2017, 8, 14063 CrossRef CAS PubMed.
- F. Wilhelmi de Toledo, F. Grundler, C. R. Sirtori and M. Ruscica, Unravelling the health effects of fasting: a long road from obesity treatment to healthy life span increase and improved cognition, Ann. Med., 2020, 52, 147–161 CrossRef CAS PubMed.
- E. W. Flanagan, J. Most, J. T. Mey and L. M. Redman, calorie restriction and aging in humans, Annu. Rev. Nutr., 2020, 40, 105–133 CrossRef CAS PubMed.
- C. S. Catana, A. G. Atanasov and I. Berindan-Neagoe, Natural products with anti-aging potential: Affected targets and molecular mechanisms, Biotechnol. Adv., 2018, 36, 1649–1656 CrossRef CAS PubMed.
- K. Pallauf, K. Giller, P. Huebbe and G. Rimbach, Nutrition and healthy ageing: Calorie restriction or polyphenol-rich “MediterrAsian” diet?, Oxid. Med. Cell. Longevity, 2013, 2013, 707421 Search PubMed.
- S. Davinelli, D. De Stefani, I. De Vivo and G. Scapagnini, Polyphenols as caloric restriction mimetics regulating mitochondrial biogenesis and mitophagy, Trends Endocrinol. Metab., 2020, 31, 536–550 CrossRef CAS PubMed.
- J. G. Wood, B. Rogina, S. Lavu, K. Howitz, S. L. Helfand, M. Tatar and D. Sinclair, Sirtuin activators mimic caloric restriction and delay ageing in metazoans, Nature, 2004, 430, 686–689 CrossRef CAS PubMed.
- C. Lee and V. Longo, Dietary restriction with and without caloric restriction for healthy aging, F1000Research, 2016, 5, Rev-117 Search PubMed.
- S. J. Simpson, D. G. Le Couteur, D. Raubenheimer, S. M. Solon-Biet, G. J. Cooney, V. C. Cogger and L. Fontana, Dietary protein, aging and nutritional geometry, Ageing Res. Rev., 2017, 39, 78–86 CrossRef CAS PubMed.
- J. P. Moatt, M. A. Fyfe, E. Heap, L. J. M. Mitchell, F. Moon and C. A. Walling, Reconciling nutritional geometry with classical dietary restriction: Effects of nutrient intake, not calories, on survival and reproduction, Aging Cell, 2019, 18, e12868 CrossRef CAS PubMed.
- C. J. Hosking, D. Raubenheimer, M. A. Charleston, S. J. Simpson and A. M. Senior, Macronutrient intakes and the lifespan-fecundity trade-off: A geometric framework agent-based model, J. R. Soc., Interface, 2019, 16, 20180733 CrossRef CAS PubMed.
- C. Canto and J. Auwerx, PGC-1alpha, SIRT1 and AMPK, an energy sensing network that controls energy expenditure, Curr. Opin. Lipidol., 2009, 20, 98–105 CrossRef CAS PubMed.
- R. Andersson, A. M. Eisele-Burger, S. Hanzen, K. Vielfort, D. Oling, F. Eisele, G. Johansson, T. Gustafsson, K. Kvint and T. Nystrom, Differential role of cytosolic Hsp70s in longevity assurance and protein quality control, PLoS Genet., 2021, 17, e1008951 CrossRef CAS PubMed.
- R. M. Reznick, H. Zong, J. Li, K. Morino, I. K. Moore, H. J. Yu, Z. X. Liu, J. Dong, K. J. Mustard, S. A. Hawley, D. Befroy, M. Pypaert, D. G. Hardie, L. H. Young and G. I. Shulman, Aging-associated reductions in AMP-activated protein kinase activity and mitochondrial biogenesis, Cell Metab., 2007, 5, 151–156 CrossRef CAS PubMed.
- M. Lagouge, C. Argmann, Z. Gerhart-Hines, H. Meziane, C. Lerin, F. Daussin, N. Messadeq, J. Milne, P. Lambert, P. Elliott, B. Geny, M. Laakso, P. Puigserver and J. Auwerx, Resveratrol improves mitochondrial function and protects against metabolic disease by activating SIRT1 and PGC-1alpha, Cell, 2006, 127, 1109–1122 CrossRef CAS PubMed.
- M. Sarikhani, J. C. Garbern, S. Ma, R. Sereda, J. Conde, G. Krahenbuhl, G. O. Escalante, A. Ahmed, J. D. Buenrostro and R. T. Lee, Sustained activation of AMPK enhances differentiation of human iPSC-derived cardiomyocytes via sirtuin activation, Stem Cell Rep., 2020, 15, 498–514 CrossRef CAS PubMed.
- C. Canto, Z. Gerhart-Hines, J. N. Feige, M. Lagouge, L. Noriega, J. C. Milne, P. J. Elliott, P. Puigserver and J. Auwerx, AMPK regulates energy expenditure by modulating NAD+ metabolism
and SIRT1 activity, Nature, 2009, 458, 1056–1060 CrossRef CAS PubMed.
- S. Liu, Y. Su, B. Sun, R. Hao, S. Pan, X. Gao, X. Dong, A. M. Ismail and B. Han, Luteolin protects against CIRI, potentially via regulation of the SIRT3/AMPK/mTOR signaling pathway, Neurochem. Res., 2020, 45, 2499–2515 CrossRef CAS PubMed.
- J. Birch and J. Gil, Senescence and the SASP: Many therapeutic avenues, Genes Dev., 2020, 34, 1565–1576 CrossRef CAS PubMed.
- M. Amaya-Montoya, A. Perez-Londono, V. Guatibonza-Garcia, A. Vargas-Villanueva and C. O. Mendivil, Cellular senescence as a therapeutic target for age-related diseases: A review, Adv. Ther., 2020, 37, 1407–1424 CrossRef PubMed.
- P. Davalli, T. Mitic, A. Caporali, A. Lauriola and D. D'Arca, ROS, Cell senescence, and novel molecular mechanisms in aging and age-related diseases, Oxid. Med. Cell. Longevity, 2016, 2016, 3565127 Search PubMed.
- N. Herranz and J. Gil, Mechanisms and functions of cellular senescence, J. Clin. Invest., 2018, 128, 1238–1246 CrossRef PubMed.
- A. Rolt and L. S. Cox, Structural basis of the anti-ageing effects of polyphenolics: Mitigation of oxidative stress, BMC Chem., 2020, 14, 50 CrossRef CAS PubMed.
- L. Matos, A. M. Gouveia and H. Almeida, Resveratrol attenuates copper-induced senescence by improving cellular proteostasis, Oxid. Med. Cell. Longevity, 2017, 2017, 3793817 Search PubMed.
- M. J. Yousefzadeh, Y. Zhu, S. J. McGowan, L. Angelini, H. Fuhrmann-Stroissnigg, M. Xu, Y. Y. Ling, K. I. Melos, T. Pirtskhalava, C. L. Inman, C. McGuckian, E. A. Wade, J. I. Kato, D. Grassi, M. Wentworth, C. E. Burd, E. A. Arriaga, W. L. Ladiges, T. Tchkonia, J. L. Kirkland, P. D. Robbins and L. J. Niedernhofer, Fisetin is a senotherapeutic that extends health and lifespan, EBioMedicine, 2018, 36, 18–28 CrossRef PubMed.
- A. Argyropoulou, N. Aligiannis, I. P. Trougakos and A. L. Skaltsounis, Natural compounds with anti-ageing activity, Nat. Prod. Rep., 2013, 30, 1412–1437 RSC.
- M. Holczer, B. Besze, V. Zambo, M. Csala, G. Banhegyi and O. Kapuy, Epigallocatechin-3-Gallate (EGCG) promotes autophagy-dependent survival via influencing the balance of mTOR-AMPK pathways upon endoplasmic reticulum stress, Oxid. Med. Cell. Longevity, 2018, 2018, 6721530 Search PubMed.
- D. Li, Y. Cui, X. Wang, F. Liu and X. Li, Apple polyphenol extract alleviates lipid accumulation in free-fatty-acid-exposed HepG2 cells via activating autophagy mediated by SIRT1/AMPK signaling, Phytother. Res., 2021, 35, 1416–1431 CrossRef CAS PubMed.
- J. Song, Y. Huang, W. Zheng, J. Yan, M. Cheng, R. Zhao, L. Chen, C. Hu and W. Jia, Resveratrol reduces intracellular reactive oxygen species levels by inducing autophagy through the AMPK-mTOR pathway, Front. Med., 2018, 12, 697–706 CrossRef PubMed.
|
This journal is © The Royal Society of Chemistry 2023 |
Click here to see how this site uses Cookies. View our privacy policy here.