DOI:
10.1039/D2FO02207A
(Paper)
Food Funct., 2023,
14, 1062-1071
Improvements in gait and balance in patients with multiple sclerosis after treatment with coconut oil and epigallocatechin gallate. A pilot study
Received
28th July 2022
, Accepted 10th December 2022
First published on 3rd January 2023
Abstract
Multiple sclerosis (MS) is a neurodegenerative disease that progressively decreases the muscular and functional capacity. Thus, there is an alteration in the ability to walk that affects balance, speed and resistance. Since MS pathology involves neuroinflammation, cellular oxidation and mitochondrial alterations, the objective of the study was to assess the impact of a nutritional intervention with coconut oil and epigallocatechin gallate (EGCG) on gait and balance. In order to do this, 51 patients with MS were enrolled and randomly distributed into an intervention group and a control group, which received either a daily dose of 800 mg of EGCG and 60 ml of coconut oil, or a placebo, all during a period of 4 months and which followed a Mediterranean isocaloric diet. Initial and final assessments consisted of the evaluation of quantitative balance (Berg scale), perceived balance (ABC scale), gait speed (10MWT) and resistance (2MWT). Besides, muscle strength was measured using a dynamometer and levels of β-hydroxybutyrate (BHB) were measured in serum samples. In the intervention group, there was a significant improvement in the gait speed, quantitative balance and muscle strength of the right quadriceps; an improvement in gait resistance was observed in both groups. There were also significant and positive correlations between balance and gait scales. In conclusion, the administration of EGCG and coconut oil seems to improve gait speed and balance in MS patients, although the latter was not perceived by them. Furthermore, these variables appear to be related and contribute to functionality.
Introduction
Multiple sclerosis (MS) is a neurodegenerative disease that causes progressive muscle atrophy linked to mitochondrial alterations.1 There are different types of the disease. However, the majority of patients present relapsing-remitting multiple sclerosis (RRMS), which can evolve into secondary progressive multiple sclerosis (SPMS).2–4 Anatomically, the disease shows damage to the myelin sheath that covers neurons, which will have a drastic effect on axonal activity.4 This neuronal damage in the cortical area, mainly in the gray matter, is related to the functional disability characteristic of the disease.5,6 At the same time, the loss of muscle mass and strength mainly in the lower extremities,7 which has been associated with a lack of functionality,8 leads to physical inactivity9 and, as a consequence, alterations in balance and gait10 already present in patients newly diagnosed with the disease.11 Particularly noteworthy is the deterioration in gait, for both resistance and speed.12
Pathogenically, one of the mechanisms that explains neuronal alterations is the inflammatory process mediated by alterations in the immune system that ends up altering mitochondrial activity with decreased ATP production and increased oxidative stress.13 Based on this mechanism, nutritional interventions supplemented with antioxidants could be a therapeutic alternative to drugs, which can improve the energy balance of neurons by reversing mitochondrial activity, so that axonal damage that is reversible can be repaired and neuroprotection generated.14
On this note, the ketone bodies obtained through hepatic β-oxidation are effective in restoring the correct mitochondrial activity and energy production problems, which may be due to the fact that they restore the activity of complex I of the electron transport chain, as seen in in vitro studies with cell cultures15 and in animal models.16 In this sense, primary motor neurons increase sirtuin expression (SIRT) after treatment with medium-chain triglycerides (MCTs), which are the most important source of ketone bodies. These metabolites regulate mitochondrial activity and cell survival precisely through sirtuin-mediated responses,17 which would explain the improvements observed in MS after following ketogenic diets.18 Among ketone bodies, β-hydroxybutyrate (BHB) stands out for its neuroprotective efficacy observed after stroke and neurodegenerative diseases,19 as well as its anti-inflammatory effects.20 Regarding the nutrients that contain the most MCTs, it should be noted that coconut oil is almost 90% saturated fat, highlighting those constituted by medium chain fatty acids (MCFAs) such as lauric, caprylic and caproic acids.21
The neuroprotective activity of MCTs could be achieved and improved if they were administered together with high levels of antioxidants. Epigallocatechin gallate (EGCG), the main component of green tea Camellia sinensis (Theaceae),22 shows a large number of biological effects, such as its anti-inflammatory and antioxidant activity,23,24 linked to the repressed proliferation of autoreactive T cells, reduced production of pro-inflammatory cytokines, decreased Th1 and Th17 cells, and increased regulatory T-cell populations.25 In relation to MS, the fact that EGCG accumulates within the mitochondria decreasing the apoptosis of neurons subjected to further oxidative stress26 could explain the improvement of the disease by limiting brain inflammation and reducing neuronal damage.27 In fact, mice of the experimental autoimmune encephalomyelitis (EAE) animal model show clear mitochondrial dysfunction, which leads to axonal injury that may be reversible.28 Finally, it should be noted that both ketone bodies and EGCG have shown clinical improvements related to muscle activity, with ketone bodies showing an anticatabolic effect in the skeletal muscle,29 specifically seen in multifactorial skeletal muscle atrophy and inflammation-induced catabolism,30 and with EGCG showing an anti-fatigue effect in the face of intense physical activity,31 which when administered specifically to patients with MS for 12 weeks improves muscular metabolism.32
Despite these findings, there are some studies that show possible adverse effects or lack of efficacy of coconut oil and EGCG respectively. Specifically, the consumption of oil has been linked to possible cardiocirculatory alterations that could cause problems for human health.33,34 On the other hand, no clinical improvements or absence of new brain lesions were observed in MS after the administration of high doses of EGCG.35
Therefore, the purpose of the present study was to determine the impact of coconut oil, as a source of ketone bodies, and EGCG on gait and balance, and the relationship between them in patients with MS.
Methods
A prospective, mixed and experimental pilot study with MS patients was conducted. The clinical trial ID for this study was NCT03740295.
Sample
The main MS associations in Spain were asked to inform their members about the nature of the research. The following selection criteria were applied to the 67 people interested in participating in the study: patients over 18 years of age diagnosed with MS at least 6 months before the study and treated with glatiramer acetate and interferon beta (common treatment for the disease). The exclusion criteria included pregnant or breastfeeding women, patients with tracheotomy, stoma or short bowel syndrome, patients with dementia or evidence of alcohol or drug abuse, patients with myocardial infarction, heart failure, cardiac dysrhythmia, angina or other heart conditions, patients with kidney problems with creatinine levels two times higher than normal, patients with elevated liver markers three times higher than normal or with chronic liver disease, patients with hyperthyroidism, acromegaly, or polycystic ovary syndrome or MS patients included in other studies with experimental drugs or treatment. A CONSORT diagram is attached that describes the flow of participants from joining the study to statistical data analysis (Fig. 1).
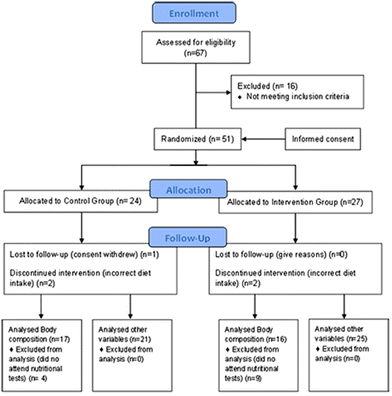 |
| Fig. 1 Consort flow diagram for enrollment of MS patients. | |
Procedure
After the final sample was selected, patients were provided with instructions indicating not to change the established diet as well as to take the capsules every day at the same time over the 4-month duration of the intervention. Weekly telephone calls were made to all patients to verify if they were complying with the treatment, or experiencing any medical issue. A final sample of 51 MS patients was randomly divided into the intervention group and the control group.
Intervention
Randomization of patients to either group was performed without stratification by drawing consecutively numbered sealed and opaque envelopes in a randomized order by a computer. The intervention group received an isocaloric diet (adapted to each patient's characteristics and divided into 5 meals a day: breakfast, mid-morning snack, lunch, afternoon snack and dinner) enriched with 60 ml of extra virgin coconut oil divided into 2 equal intakes (30 ml for breakfast and 30 ml for lunch), and supplemented with 800 mg of EGCG administered in two capsules of 400 mg to be taken twice a day (one capsule in the morning and another in the afternoon). The control group followed the same isocaloric diet, with the exception of coconut oil that was replaced with other lipids, such as olive oil, sunflower oil, fish and nuts. This ensured that a state of ketosis did not occur in the control group, as the percentages and the amounts of macronutrients were not characteristic of a ketogenic diet.36 Placebo (capsules containing microcrystalline cellulose, matching in size and color) was also administered to the control group with the same instructions as the intervention group.
The basal diet followed by both groups included the following percentage distribution of the 3 main macronutrients with respect to the total caloric value: 20% proteins, 40% carbohydrates and 40% Mediterranean lipids. This was a Mediterranean diet, characterized by its anti-inflammatory effect based on a low intake of saturated fat from butter, full fat milk and red meat, a high intake of monounsaturated fat found in olive oil, an adequate intake of omega-6 versus omega-3 polyunsaturated fatty acids, from fish, shellfish and nuts, low protein content obtained from red meat, high quantities of fruit, vegetables, virgin olive oil, herbs and spices, and high quantities of fiber found in products of plant origin, such as vegetables, fruit, whole grains, pulses and nuts. To avoid errors in the interpretation of the results, care was taken to avoid foods rich in polyphenols, especially tea, coffee and wine, when designing the basal diet for the placebo group.
Measurements
The following measurements were taken before and after the 4-month intervention, under the same conditions.
Functional ability was measured with the expanded disability status scale (EDSS).
The scale is an ordinal scale based on a neurological examination of the eight functional systems (pyramidal, cerebellar, brainstem, mental, sensory, visual, bowel and bladder), alongside assessing gait, which, as a result, provides a disability index between 0 and 10, 0 being understood as having normal health and 10 death by MS.37
Activity-specific balance confidence scale (ABC).
Patients completed the ABC scale, which quantifies how confident a person feels about not losing their balance while performing 16 activities of daily living.38 Scores range from 0 (lack of confidence) to 10 (complete confidence).39
Berg balance scale.
This scale was used to measure static and dynamic balance. The scale consists of 14 items, scored from 0 to 4, which are added to make a total score between 0 and 56. The higher the score, the better the balance.40
Ten-meter walk test (10MWT).
This test was used to measure walking ability in terms of speed. No assistance was provided for the entire 10 meters and the time the patients walked through the central 6 m section was recorded, except for the 2 m acceleration and 2 m deceleration distances. Measurements were performed 3 times, calculating a mean value ± standard deviation from these 3 measurements.41,42
Two-minute walk test (2MWT).
This test is used to determine gait resistance,43 being the most suitable alternative for MS patients since the six-minute walk test (6MWT), widely used to assess functional exercise resistance, does not seem to be applicable to all patients with this pathology.44 It was carried out in a closed, wide, long and flat 30 m corridor. Before the test, the participants were instructed to rest in a chair near the starting line for at least 10 min. The patients were then asked to walk back and forth, as far as possible without running, for a period of 2 min, and the distance was recorded.45
Maximal isometric strength.
It was assessed using the portable dynamometer NedDFM/IBV (Institute of Biomechanics of Valencia, Valencia, Spain) for lower limb muscles: quadriceps and anterior tibialis as described elsewhere.46,47
β-Hydroxybutyrate (BHB).
A blood sample was taken at 9 a.m. on an empty stomach, and the serum was separated from the plasma after centrifuging the samples. Concentrations of BHB were measured with commercial kits (Randox Laboratories, Crumlin, UK) in an automatic clinical biochemical analyzer (Olympus A 400).
Ethical concerns
This study was carried out in accordance with the Declaration of Helsinki,48 with prior approval of the protocol by the Human Research Committee of the University of Valencia of the Experimental Research Ethics Committee (procedure number H1512345043343). In addition, patients involved in the study signed a consent form after being informed about the procedures and nature of the study.
Statistical analysis
The SPSS v.23 (IBM Corporation, Armonk, NY, USA) tool was used for statistical analysis. Normality was assessed by the Shapiro–Wilk test. Inter-group differences for demographic and clinical parameters were assessed either with Student's t-test for independent data with normal distribution, or with the Mann–Whitney U test for non-normal distribution data. Pre- and post-treatment differences were assessed with paired sample t-test for data with normal distribution, or the Wilcoxon signed-rank test for non-normal distribution data. A chi-square test was used to analyse categorical data.
Spearman's correlation coefficient (rs) was used to look for correlations between functional and gait parameters in the overall group of EM patients, using only pre-intervention data. Coefficient values from 0 to 0.39 were considered low; from 0.40 to 0.69, moderate; and from 0.7 and onwards were considered high associations. A significance level of p < 0.05 was used for all tests. Data are presented as mean ± standard deviation, as well as median and interquartile range, or the number of patients and the percentage representing this number for each group (control and intervention groups).
Results
After the selection criteria were applied, the population sample of 51 patients with MS was divided into two groups: the intervention group and the control group. The sociodemographic and clinical characteristics of both groups are shown in Table 1, where no differences between the groups were observed regarding the type of MS, gender, age and time since diagnosis. In addition, there were no differences in the basal measurements of BHB serum levels, functional capacity (EDSS), perceived balance (ABC scale), quantitative balance (Berg scale), gait speed (10MWT), gait resistance (2MWT) and muscle strength of the anterior tibialis and quadriceps muscles.
Table 1 Demographic and clinical information about the study group before treatment
Sample composition |
CG |
IG |
p
|
Baseline parameters |
CG |
IG |
P
|
Count (%) |
Count (%) |
M (SD); Md [IQR] |
M (SD); Md [IQR] |
MS: multiple sclerosis; M: mean; SD: standard deviation; Md: median; IQR: interquartile range; c: significance from chi-square test; t: significance form Student's t test; u: significance from Mann Whitney U test; p: p-value. BHB: β-hydroxybutyrate; EDSS: expanded disability status scale. ABC: activities-specific balance confidence scale; Berg: Berg balance scale; 10MWT: 10-meter walk test; 2MWT: two-minute walk test; CD-R: strength in right quadriceps; CD-I: strength in left quadriceps; TA-R: strength in right tibialis; TA-L: strength in left tibialis determined by isokinetic dynamometer test; m = meter; s = seconds; N = Newton. |
RR MS |
17 (70.8) |
20 (74.1) |
0.796c |
BHB (mmol L−1) |
0.05 (0.02); 0.04 [0.02] |
0.06 (0.04); 0.05 [0.05] |
0.351u |
SP MS |
7 (29.2) |
7 (25.9) |
ABC scale |
58 (32); 63 [53] |
73 (24); 81 [34] |
0.170u |
Men |
10 (41.7) |
5 (18.5) |
0.070c |
Berg scale |
43 (16); 50 [19] |
49 (9.7); 54 [9] |
0.141u |
Women |
14 (58.3) |
22 (81.5) |
10MWT (m s−1) |
1.7 (0.74); 1.6 [1] |
1.6 (0.58); 1.5 [1] |
0.664t |
|
M (SD); Md [IQR] |
M (SD); Md [IQR] |
p
|
2MWT (m) |
107 (40); 95 [60] |
113 (38); 120 [50] |
0.353u |
Age (years) |
50 (12); 50.5 [15] |
45 (11); 43 [18] |
0.119t |
CD-R (N) |
8.7 (2.3); 8.3 [4] |
8 (3.9); 8.7 [5] |
0.670t |
Diagnosis (years) |
14 (8); 14.5 [14] |
12 (9.7); 8 [11] |
0.156u |
CD-L (N) |
7.9 (3.5); 7.8 [5] |
8.4 (3.2); 8.2 [4] |
0.784t |
EDSS |
3.7 (2.1); 3.8 [15] |
3.5 (2.1); 3 [4] |
0.586u |
TA-R (N) |
6 (2.9); 6.7 [5] |
6.8 (3.5); 6.5 [5] |
0.769u |
Fat mass (%) |
18.9 (5); 18.2 [7] |
19.5 (3.8); 19.4 [5] |
0.445t |
TA-L (N) |
5 (3.5); 6.2 [6] |
6.4 (2.8); 6.2 [4] |
0.719t |
Muscle mass (%) |
38.4 (4); 38.8 [7] |
39.4 (2.9); 39.7 [3] |
0.584t |
|
|
|
|
Impact on balance and walking ability
Regarding the changes produced after 4 months of intervention, as previously published, in the intervention group there was a significant increase in BHB in blood, as well as a significant improvement in functional capacity determined by the EDSS scale.49 In addition, it can be observed that the quantitative balance (Fig. 2B) improved significantly only in the intervention group, going from an initial score of 49 ± 9.6 to 52 ± 6.9 after treatment (z26 = −3.379; p < 0.001), while the control group went from an initial score of 43 ± 16 to a final score of 43 ± 17 (z23 = −0.866; p = 0.387). On the other hand, Fig. 2A shows that the perception of balance (ABC scale) did not show significant changes in any of the groups after the 4 months that the study lasted.
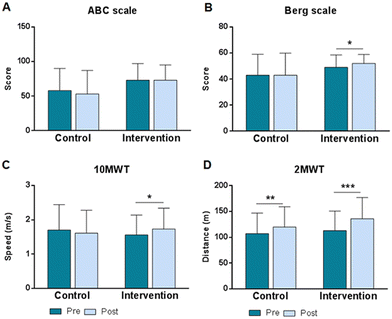 |
| Fig. 2 Pre- and post-intervention balance and gait measurements in control and intervention groups. A. Berg scale. B. ABC scale. C. Gait speed (10MWT). D. Gait resistance (2MWT). *p < 0.05; **p < 0.01; ***p < 0.001. | |
Regarding gait speed (Fig. 2C), significant differences were found only in the intervention group, where an increase from 1.56 ± 0.58 m s−1 to 1.73 ± 0.61 m s−1 was observed (t24 = −2.851, p = 0.009). While in the control group the mean speed decreased non-significantly from 1.70 ± 0.74 to 1.61 ± 0.67 m s−1 (t15 = 1.484, p = 0.1583). In terms of gait resistance (Fig. 2D), determined by the 2MWT test, a significant improvement was observed in both groups. In the intervention group, the change represented an increase of 23 meters, going from 113 ± 38 m to 136 ± 41 m (t25 = −6.173; p < 0.001), while in the control group, the increase was lower, going from 107 ± 40 to 120 ± 39 m (t16 = −3.296; p = 0.005).
Besides, a comparison of the strength of the muscles showed a significant increase in the strength of the right anterior tibialis in both groups, and higher strength in the right quadriceps only in the intervention group (Table 2).
Table 2 Effect of intervention in muscle strength in control and intervention groups
|
Control group |
Intervention group |
Pre |
Post |
Pre |
Post |
M ± SD |
M ± SD |
M ± SD |
M ± SD |
M = mean; SD = standard deviation; CD = quadriceps muscle; AT = anterior tibialis muscle; R = right; L = left; t = t test for parametric data; w = test of Wilcoxon for no parametric data. * p < 0.05. |
CD-R |
8.7 ± 2.3 |
9 ± 3.5 |
8 ± 3.9 |
10 ± 4.8t* |
CD-L |
7.9 ± 3.5 |
7.7 ± 4.5 |
8.4 ± 3.2 |
9.6 ± 4.2 |
AT-R |
6 ± 2.9 |
7.9 ± 4.3w* |
6.8 ± 3.5 |
8.3 ± 4.8t* |
AT-R |
5 ± 3.5 |
6.5 ± 4.9 |
6.4 ± 2.8 |
7 ± 4.2 |
Relationship between walking ability and balance
The correlation analysis using initial measurements found a significant positive association of rs = 0.743 (p < 0.001) between quantitative (Berg scale) and perceived (ABC scale) balance. In addition, both balance scales had significant positive associations with gait parameters as shown in Fig. 3. Thus, the better the balance shown by the patients, the less time it took to walk the 10 m distance (Fig. 3A, rs = 0.780, p < 0.001). Similarly, the patients with the best balance walked more meters during the 2 minutes (Fig. 3B; rs = 0.721, p < 0.001). Perceived balance (ABC scale) showed correlations in the same sense (Fig. 3C and D), with a greater speed and gait resistance for the patients with the best perception of balance (rs = 0.725, p < 0.001, and rs = 0.653, p < 0.00, respectively).
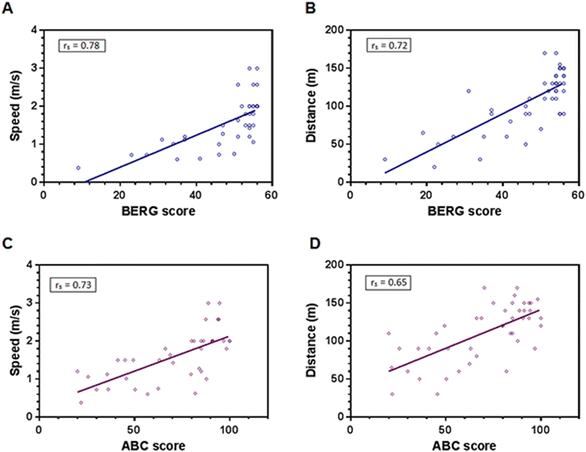 |
| Fig. 3 Associations between gait speed (10MWT) and gait resistance (2MWT) with: A and B. Quantitative balance (Berg scale) and C and D. Perceived balance (ABC scale). Overall group, pre-intervention data. Significant Spearman correlation coefficients (rs) are shown within rectangles. | |
Discussion
The nutritional intervention in the present pilot study achieved a significant improvement in both balance and gait in MS patients. This could be related to the muscular improvements, which have been evidenced both with the increase in ketone bodies in the blood29,30 and EGCG administration.31,32 On the other hand, ketogenic diets have neuroprotective effects, already seen in various neurological disorders like epilepsy,50 Parkinson's disease,51 juvenile traumatic brain injury,52 and MS.18 Likewise, polyphenol EGCG has great neuroprotective properties,53,54 which shows improvements in diseases such as Alzheimer's disease55 or Parkinson's disease,56 also showing to be effective in MS, specifically in the EAE animal model.27,28
Neuroprotective role of EGCG and coconut oil
The neuroprotective activity of ketone bodies and EGCG would justify the improvements seen in our study, especially considering the neuronal damage linked to alterations in balance and ability to walk10 as part or consequence of the functional disability5,6 and muscle wastage in the disease.57
As we already evidenced for this same sample of MS patients that the intervention group showed a significant increase in BHB levels, muscle mass and improved functional capacity (EDSS) after 4 months of intervention,49 in this new analysis we focus on gait and balance as important factors for the improvement in EDSS.
Regarding balance, prior to the intervention, a correlation is observed between the perceived and quantitative balance determined by the ABC and Berg scales, respectively (Fig. 3). However, after treatment, there are significant improvements in the quantitative balance in the intervention group, although it is interesting to note that these same patients did not show a change in the perception of this improvement, which coincides with what was observed by Jonsdottir J., et al. in 2018 after applying an intensive multimodal training.58
On the other hand, the balance and gait of all the patients in the study correlate significantly before the intervention, so that those with better quantitative balance (dynamic and static, determined by the Berg scale) and perceived balance (ABC scale) are also the ones that show the highest gait speed and resistance (Fig. 3). However, after treatment, both groups improved in gait endurance while only the intervention group improved in speed (Fig. 2). In this sense, it is interesting to note that in the literature, an association has been observed between frontal lobe activity and alterations in speed and balance for elder people.59 Besides, faster gait speed has been linked to better cognitive performance in the frontal lobe related questioners.60,61 It is noteworthy that the frontal lobe is especially damaged in MS patients62 and that is why we believe that our treatment had a bigger impact in this area, compared with the basal ganglia or extrapyramidal systems, which are more related to fatigue and extrapyramidal symptoms in the disease.63,64 Thus, the scheme in Fig. 4, shows our hypothesis that EGCG and ketone bodies from coconut oil had a neuroprotective effect mainly targeted to the frontal lobe cortex, which in turn improved gait and balance, both of them contributing to the increase in functionality experienced by the intervention group of the present study.
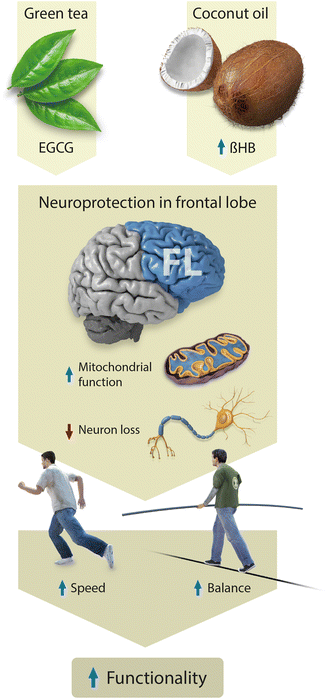 |
| Fig. 4 Neuroprotective action of EGCG and coconut oil in functionality through specific activity in the frontal lobe. | |
Muscular impact of the intervention
Furthermore, apart from neurologically induced changes in the musculoskeletal system, peripheral symptoms may be a consequence of the chronically reduced physical activity that characterizes this pathology.65 Changes in the fiber type have recently been found in the muscles of MS patients, which resemble those with a history of reduced activity: the fibers atrophied and the percentage of type I fibers, related to endurance in gait, decreased from 76 to 65%, while especially in the quadriceps there is a change towards a higher percentage of type II fibers, related to speed.66 This could help explain that a treatment like ours, linked to an improvement in muscle metabolism,67 especially improves speed precisely as a consequence of a greater presence of type II fibers, which also seems to be confirmed by an increase in muscle strength only in the right quadriceps of the intervention group. Based on this idea of the peripheral impact, we believe that the reason both groups improved gait resistance is not so much due to the intervention, but rather due to the change in diet of all the study participants, who began to follow an isocaloric Mediterranean diet (whose main characteristics have been described in the Methods section), linked to improvements in gait resistance, and not to other variables such as speed.68
In this sense, the nutritional habits that these patients followed before the intervention are also worth noting, as described by our team.69 In general, they followed unbalanced diets (which promoted the increase in fat mass), characterized by high caloric intake related to excess protein and fat, and carbohydrates intake below the recommended amount. According to the literature, the impact of high consumption of fats, especially those of animal origin, seems especially important in the prevalence and progression of the disease.70 And precisely with the Mediterranean diet followed by all the patients, this aspect was especially improved, since it was designed with a low intake of saturated fat, a high intake of monounsaturated fat and an adequate intake of omega-6 versus omega-3 polyunsaturated fatty acids. These characteristics promote an anti-inflammatory effect that has been associated with muscular improvements71 and specially with functional improvements in patients with MS.72 The main functional benefits of the Mediterranean diet seem to be based especially on the improvement of walking resistance, since it was a common factor of improvement in all the patients in the study (not only in those in the intervention group). This fact could be reinforced by the results we have obtained in the tibialis muscle, characterized by being mainly made up of type I fibers, and which significantly improved on the right in both groups. However, and despite that the patients who only followed the Mediterranean diet did not show improvement in speed, it has recently been highlighted that the Mediterranean diet, in older people with type 2 diabetes mellitus, is also linked to improvements in walking speed,73 as well as in balance and walking resistance.74 These results, on the one hand, reinforce the relationship between the walking capacity (speed and resistance) and balance, also observed in the patients with MS in this study. But on the other hand, they seem to indicate that the improvement in balance and walking speed could be especially due to the nutrients rich in polyphenols and characteristic of the Mediterranean diet (essentially wine, and coffee commonly consumed in European Mediterranean countries), which were excluded in the Mediterranean diet designed for the placebo group (so as not to interfere with the conclusions of the study). This fact seems to indicate that the characteristics of a classic Mediterranean diet can be a good complement to coconut oil and polyphenols, such as EGCG, to improve walking resistance and speed as well as balance. In short, the results obtained seem to indicate that our treatment improves the interaction that occurs between various systems such as locomotion, balance and the central nervous system (CNS) as a coordinating part, particularly of the executive functions of the frontal cortex in mobility,75 which would be confirmed by the significant correlations observed prior to treatment between that balance and gait variables (Fig. 3).
Regarding the limitations of the study, we need to mention the size of the population sample as it is a pilot study. In addition, it would be necessary to complete the results with muscle power data, as well as imaging tests that would help to understand the improvements that we believe could occur especially in certain regions of the cortex. Along these lines, on one hand, blood markers that inform about changes in metabolism and muscle damage should be considered and, on the other hand, we should consider the possible application of tests such as the quantitative electroencephalogram (QEEG) or brain mapping, which makes it possible to evaluate brain function while being able to monitor changes.
Conclusions
In conclusion, the administration of EGCG and coconut oil seems to improve gait speed and balance, although patients do not perceive this improvement, while the healthy Mediterranean diet followed by all the patients in the study could explain the improvement in gait resistance. In addition, patients with better balance, both perceived and quantitative, had greater gait speed and resistance.
Author contributions
Conceptualization, J. E. d. l. R. O. and M. C.-B.; methodology, B. P.; software, J. A.-J. and N. d. B. T.; formal analysis, C. V.-C.; investigation, J. A.-J., N. d. B. and C. V.-C.; resources, B. P.; data curation, J. E. d. l. R. O. and M. C.-B.; writing – original draft preparation, J. E. d. l. R. O.; writing – review and editing, J. M. L. R.; visualization, J. M. L. R.; supervision, M. C.-B.; project administration, J. E. d. l. R. O.; funding acquisition, J. E. d. l. R. O. All authors have read and agreed to the published version of the manuscript.
Institutional review board statement
The study was conducted according to the guidelines of the Declaration of Helsinki, and approved by the Institutional Review Board (or Ethics Committee) of Human Research Committee of the University of Valencia of the Experimental Research Ethics Committee (protocol code H1512345043343; 14 December, 2017).
Informed consent statement
Informed consent was obtained from all subjects involved in the study.
Conflicts of interest
There are no conflicts to declare.
Acknowledgements
The present research was funded by the Catholic University Foundation “San Vicente Mártir”, for the research project “The Impact of Triglycerides on Multiple Sclerosis” (promotion code 2018-203-001).
References
- M. A. Harp, K. K. McCully, M. Moldavskiy and D. Backus, Skeletal muscle mitochondrial capacity in people with multiple sclerosis, Mult. Scler. J. – Exp. Transl. Clin., 2016, 2 Search PubMed , 2055217316678020.
- B. Ferguson, M. K. Matyszak, M. M. Esiri and V. H. Perry, Axonal damage in acute multiple sclerosis lesions, Brain, 1997, 120, 393–399 CrossRef PubMed.
- B. D. Trapp, J. Peterson, R. M. Ransohoff, R. Rudick, S. Mörk and L. Bö, Axonal Transection in the Lesions of Multiple Sclerosis, N. Engl. J. Med., 1998, 338, 278–285 CrossRef PubMed.
- A. Bitsch, S. Bunkowski, T. Kuhlmann and W. Brück, Acute axonal injury in multiple sclerosis: Correlation with demyelination and inflammation, Brain, 2000, 123, 1174–1183 CrossRef PubMed.
- J. Lansley, D. Mataix-Cols, M. Grau, J. Radua and J. Sastre-Garriga, Localized grey matter atrophy in multiple sclerosis: A meta-analysis of voxel-based morphometry studies and associations with functional disability, Neurosci. Biobehav. Rev., 2013, 37, 819–830 CrossRef PubMed.
- A. Mike, B. I. Glanz, P. Hildenbrand, D. Meier, K. Bolden, M. Liguori, E. Dell'Oglio, B. C. Healy, R. Bakshi and C. R. G. Guttmann, Identification and Clinical Impact of Multiple mSclerosis Cortical Lesions as Assessed by Routine 3T MR Imaging, Am. J. Neuroradiol., 2011, 32, 515–521 CrossRef PubMed.
- P. Thoumie, Relation between walking speed and muscle strength is affected by somatosensory loss in multiple sclerosis, J. Neurol., Neurosurg. Psychiatry, 2002, 73, 313–315 CrossRef CAS PubMed.
- L. O. Pfeifer, C. E. Botton, F. Diefenthaeler, D. Umpierre and R. S. Pinto, Effects of a power training program in the functional capacity, on body balance and lower limb muscle strength of elderly with type 2 diabetes mellitus, J. Sports Med. Phys. Fitness, 2021, 1529–1537, DOI:10.23736/S0022-4707.21.11880-8.
- K. S. Dorans, J. Massa, T. Chitnis, A. Ascherio and K. L. Munger, Physical activity and the incidence of multiple sclerosis, Neurology, 2016, 87, 1770–1776 CrossRef.
- P. Ilett, N. Lythgo, C. Martin and K. Brock, Balance and Gait in People with Multiple Sclerosis: A Comparison with Healthy Controls and the Immediate Change after an Intervention based on the Bobath Concept: Balance and Gait in People with Multiple Sclerosis, Physiother. Res. Int., 2016, 21, 91–101 CrossRef CAS PubMed.
- C. L. Martin, B. A. Phillips, T. J. Kilpatrick, H. Butzkueven, N. Tubridy, E. McDonald and M. P. Galea, Gait and balance impairment in early multiple sclerosis in the absence of clinical disability, Mult. Scler. J., 2006, 12, 620–628 CrossRef CAS PubMed.
- L. Comber, R. Galvin and S. Coote, Gait deficits in people with multiple sclerosis: A systematic review and meta-analysis, Gait Posture, 2017, 51, 25–35 CrossRef.
- I. P. de Barcelos, R. M. Troxell and J. S. Graves, Mitochondrial Dysfunction and Multiple Sclerosis, Biology, 2019, 8, 37 CrossRef CAS.
- K. Herges, J. M. Millward, N. Hentschel, C. Infante-Duarte, O. Aktas and F. Zipp, Neuroprotective Effect of Combination Therapy of Glatiramer Acetate and Epigallocatechin-3-Gallate in Neuroinflammation, PLoS One, 2011, 6, e25456 CrossRef CAS PubMed.
- K. Tieu, C. Perier, C. Caspersen, P. Teismann, D.-C. Wu, S.-D. Yan, A. Naini, M. Vila, V. Jackson-Lewis, R. Ramasamy and S. Przedborski, D-β-Hydroxybutyrate rescues mitochondrial respiration and mitigates features of Parkinson disease, J. Clin. Invest., 2003, 112, 892–901 CrossRef CAS.
- Z. Zhao, D. J. Lange, A. Voustianiouk, D. MacGrogan, L. Ho, J. Suh, N. Humala, M. Thiyagarajan, J. Wang and G. M. Pasinetti, A ketogenic diet as a potential novel therapeutic intervention in amyotrophic lateral sclerosis, BMC Neurosci., 2006, 7, 29 CrossRef PubMed.
- S. Körner, S. Böselt, N. Thau, K. J. Rath, R. Dengler and S. Petri, Differential Sirtuin Expression Patterns in Amyotrophic Lateral Sclerosis (ALS) Postmortem Tissue: Neuroprotective or Neurotoxic Properties of Sirtuins in ALS?, Neurodegener. Dis., 2013, 11, 141–152 CrossRef.
- L. S. Bahr, M. Bock, D. Liebscher, J. Bellmann-Strobl, L. Franz, A. Prüβ, D. Schumann, S. K. Piper, C. S. Kessler, N. Steckhan, A. Michalsen, F. Paul and A. Mähler, Ketogenic diet and fasting diet as Nutritional Approaches in Multiple Sclerosis (NAMS): protocol of a randomized controlled study, Trials, 2020, 21, 3 CrossRef PubMed.
- M. Rahman, S. Muhammad, M. A. Khan, H. Chen, D. A. Ridder, H. Müller-Fielitz, B. Pokorná, T. Vollbrandt, I. Stölting, R. Nadrowitz, J. G. Okun, S. Offermanns and M. Schwaninger, The β-hydroxybutyrate receptor HCA2 activates a neuroprotective subset of macrophages, Nat. Commun., 2014, 5, 3944 CrossRef.
- E. C. Graff, H. Fang, D. Wanders and R. L. Judd, Anti-inflammatory effects of the hydroxycarboxylic acid receptor 2, Metabolism, 2016, 65, 102–113 CrossRef.
- J. Bezard, M. Bugaut and G. Clement, Triglyceride composition of coconut oil, J. Am. Oil Chem. Soc., 1971, 48, 134–139 CrossRef.
- R. Tsao, Chemistry and Biochemistry of Dietary Polyphenols, Nutrients, 2010, 2, 1231–1246 CrossRef PubMed.
- S. Riegsecker, D. Wiczynski, M. J. Kaplan and S. Ahmed, Potential benefits of green tea polyphenol EGCG in the prevention and treatment of vascular inflammation in rheumatoid arthritis, Life Sci., 2013, 93, 307–312 CrossRef PubMed.
- J. Steinmann, J. Buer, T. Pietschmann and E. Steinmann, Anti-infective properties of epigallocatechin-3-gallate (EGCG), a component of green tea: Anti-infective effects of EGCG, Br. J. Pharmacol., 2013, 168, 1059–1073 CrossRef PubMed.
- D. Wu, J. Wang, M. Pae and S. N. Meydani, Green tea EGCG, T cells, and T cell-mediated autoimmune diseases, Mol. Aspects Med., 2012, 33, 107–118 CrossRef PubMed.
- E. K. Schroeder, N. A. Kelsey, J. Doyle, E. Breed, R. J. Bouchard, F. A. Loucks, R. A. Harbison and D. A. Linseman, Green Tea Epigallocatechin 3-Gallate Accumulates in Mitochondria and Displays a Selective Antiapoptotic Effect Against Inducers of Mitochondrial Oxidative Stress in Neurons, Antioxid. Redox Signaling, 2009, 11, 469–480 CrossRef.
- O. Aktas, T. Prozorovski, A. Smorodchenko, N. E. Savaskan, R. Lauster, P.-M. Kloetzel, C. Infante-Duarte, S. Brocke and F. Zipp, Green Tea Epigallocatechin-3-Gallate Mediates T Cellular N-κB Inhibition and Exerts Neuroprotection in Autoimmune Encephalomyelitis, J. Immunol., 2004, 173, 5794–5800 CrossRef.
- I. Nikić, D. Merkler, C. Sorbara, M. Brinkoetter, M. Kreutzfeldt, F. M. Bareyre, W. Brück, D. Bishop, T. Misgeld and M. Kerschensteiner, A reversible form of axon damage in experimental autoimmune encephalomyelitis and multiple sclerosis, Nat. Med., 2011, 17, 495–499 CrossRef PubMed.
- A. P. Koutnik, D. P. D'Agostino and B. Egan, Anticatabolic Effects of Ketone Bodies in Skeletal Muscle., Trends Endocrinol. Metab., 2019, 30, 227–229 CrossRef.
- A. P. Koutnik, A. M. Poff, N. P. Ward, J. M. DeBlasi, M. A. Soliven, M. A. Romero, P. A. Roberson, C. D. Fox, M. D. Roberts and D. P. D'Agostino, Ketone Bodies Attenuate Wasting in Models of Atrophy, J. Cachexia Sarcopenia Muscle, 2020, 11, 973–996 CrossRef PubMed.
- Y.-S. Teng and D. Wu, Anti-Fatigue Effect of Green Tea Polyphenols (-)-Epigallocatechin-3-Gallate (EGCG), Pharmacogn. Mag., 2017, 13, 326–331 CrossRef.
- A. Mähler, J. Steiniger, M. Bock, L. Klug, N. Parreidt, M. Lorenz, B. F. Zimmermann, A. Krannich, F. Paul and M. Boschmann, Metabolic response to epigallocatechin-3-gallate in relapsing-remitting multiple sclerosis: a randomized clinical trial, Am. J. Clin. Nutr., 2015, 101, 487–495 CrossRef PubMed.
- L. Eyres, M. F. Eyres, A. Chisholm and R. C. Brown, Coconut oil consumption and cardiovascular risk factors in humans, Nutr. Rev., 2016, 74, 267–280 CrossRef PubMed.
- F. M. Sacks, Coconut Oil and Heart Health, Circulation, 2020, 141, 815–817 CrossRef PubMed.
- J. Bellmann-Strobl, F. Paul, J. Wuerfel, J. Dörr, C. Infante-Duarte, E. Heidrich, B. Körtgen, A. Brandt, C. Pfüller, H. Radbruch, R. Rust, V. Siffrin, O. Aktas, C. Heesen, J. Faiss, F. Hoffmann, M. Lorenz, B. Zimmermann, S. Groppa, K.-D. Wernecke and F. Zipp, Epigallocatechin Gallate in Relapsing-Remitting Multiple Sclerosis, Neurol. Neuroimmunol. Neuroinflammation, 2021, 8(3), e981 CrossRef PubMed.
- A. A. Gibson, R. V. Seimon, C. M. Y. Lee, J. Ayre, J. Franklin, T. P. Markovic, I. D. Caterson and A. Sainsbury, Do ketogenic diets really suppress appetite? A systematic review and meta-analysis: Do ketogenic diets really suppress appetite?, Obes. Rev., 2015, 16, 64–76 CrossRef.
- J. F. Kurtzke, Rating neurologic impairment in multiple sclerosis: An expanded disability status scale (EDSS), Neurology, 1983, 33, 1444 CrossRef.
- L. E. Powell and A. M. Myers, The Activities-specific Balance Confidence (ABC) Scale, J. Gerontol., Ser. A, 1995, 50, M28–M34 CrossRef PubMed.
- A. M. Myers, P. C. Fletcher, A. H. Myers and W. Sherk, Discriminative and Evaluative Properties of the Activities-specific Balance Confidence (ABC) Scale, J. Gerontol., Ser. A, 1998, 53, M287–M294 CrossRef PubMed.
- S. Downs, The Berg Balance Scale, J. Physiother., 2015, 61, 46 CrossRef PubMed.
- P. Scalzo, A. Kümmer, T. L. Bretas, F. Cardoso and A. L. Teixeira, Serum levels of brain-derived neurotrophic factor correlate with motor impairment in Parkinson's disease, J. Neurol., 2010, 257, 540–545 CrossRef.
- U. Dalgas, K. Severinsen and K. Overgaard, Relations Between 6 Minute Walking Distance and 10 Meter Walking Speed in Patients With Multiple Sclerosis and Stroke, Arch. Phys. Med. Rehabil., 2012, 93, 1167–1172 CrossRef.
- J. Zhang, X. Chen, S. Huang, Y. Wang, W. Lin, R. Zhou and H. Zou, Two-minute walk test: Reference equations for healthy adults in China, PLoS One, 2018, 13, e0201988 CrossRef.
- D. Langeskov-Christensen, P. Feys, I. Baert, M. Riemenschneider, E. Stenager and U. Dalgas, Performed and perceived walking ability in relation to the Expanded Disability Status Scale in persons with multiple sclerosis, J. Neurol. Sci., 2017, 382, 131–136 CrossRef.
- M. Izquierdo Redín and T. Smith, Comparison of the 2-, 6-, and 12-minute walk tests in patients with stroke, J. Rehabil. Res. Dev., 2004, 41, 103 CrossRef.
-
M. Izquiero, Biomecánica y bases neuromusculares de la actividad física y el deporte, Editorial Panamericana, 2008, 1 Search PubMed.
- L. E. Brown and J. P. Weir, ASEP Procedures recommendation I: Accurate assess-ment of muscular strength and power, J. Exercise Physiol. Online, 2001, 4, 1–21 Search PubMed.
- World Medical Asociation, World Medical Association Declaration of Helsinki: Ethical Principles for Medical Research Involving Human Subjects, J. Am. Med. Assoc., 2013, 310, 2191 CrossRef.
- M. Benlloch, M. Cuerda-Ballester, E. Drehmer, J. L. Platero, S. Carrera-Juliá, M. M. López-Rodríguez, J. J. Ceron, A. Tvarijonaviciute, M. Á. Navarro, M. L. Moreno and J. E. de la Rubia Ortí, Possible Reduction of Cardiac Risk after Supplementation with Epigallocatechin Gallate and Increase of Ketone Bodies in the Blood in Patients with Multiple Sclerosis. A Pilot Study, Nutrients, 2020, 12, 3792 CrossRef PubMed.
- C. E. Stafstrom and J. M. Rho, The Ketogenic Diet as a Treatment Paradigm for Diverse Neurological Disorders, Front. Pharmacol., 2012, 3, 59, DOI:10.3389/fphar.2012.00059.
- B. Cheng, X. Yang, L. An, B. Gao, X. Liu and S. Liu, Ketogenic diet protects dopaminergic neurons against 6-OHDA neurotoxicity via up-regulating glutathione in a rat model of Parkinson's disease, Brain Res., 2009, 1286, 25–31 CrossRef PubMed.
- Y. Deng-Bryant, M. L. Prins, D. A. Hovda and N. G. Harris, Ketogenic Diet Prevents Alterations in Brain Metabolism in Young but not Adult Rats after Traumatic Brain Injury, J. Neurotrauma, 2011, 28, 1813–1825 CrossRef PubMed.
- J. Cheng-Chung Wei, H. Hsiu-Chen, W.-J. Chen, C.-N. Huang, C.-H. Peng and C.-L. Lin, Epigallocatechin gallate attenuates amyloid β-induced inflammation and neurotoxicity in EOC 13.31 microglia, Eur. J. Pharmacol., 2016, 770, 16–24 CrossRef PubMed.
- N. A. Singh, A. K. A. Mandal and Z. A. Khan, Potential neuroprotective properties of epigallocatechin-3-gallate (EGCG), Nutr. J., 2015, 15, 60 CrossRef PubMed.
- M. Cascella, S. Bimonte, M. R. Muzio, V. Schiavone and A. Cuomo, The efficacy of Epigallocatechin-3-gallate (green tea) in the treatment of Alzheimer's disease: an overview of pre-clinical studies and translational perspectives in clinical practice, Infect. Agents Cancer, 2017, 12, 36 CrossRef.
- Q. Xu, M. Langley, A. G. Kanthasamy and M. B. Reddy, Epigallocatechin Gallate Has a Neurorescue Effect in a Mouse Model of Parkinson Disease, J. Nutr., 2017, 147, 1926–1931 CrossRef PubMed.
- D. J. P. Garner and J. J. Widrick, Cross-bridge mechanisms of muscle weakness in multiple sclerosis, Muscle Nerve, 2003, 27, 456–464 CrossRef PubMed.
- J. Jonsdottir, E. Gervasoni, T. Bowman, R. Bertoni, E. Tavazzi, M. Rovaris and D. Cattaneo, Intensive Multimodal Training to Improve Gait Resistance, Mobility, Balance and Cognitive Function in Persons With Multiple Sclerosis: A Pilot Randomized Controlled Trial, Front. Neurol., 2018, 9, 800 CrossRef PubMed.
- A. Demain, G. W. M. Westby, S. Fernandez-Vidal, C. Karachi, F. Bonneville, M. C. Do, C. Delmaire, D. Dormont, E. Bardinet, Y. Agid, N. Chastan and M.-L. Welter, High-level gait and balance disorders in the elderly: a midbrain disease?, J. Neurol., 2014, 261, 196–206 CrossRef.
- B. R. Ferguson and W.-J. Gao, Thalamic Control of Cognition and Social Behavior Via Regulation of Gamma Aminobutyric Acidergic Signaling and Excitation/Inhibition Balance in the Medial Prefrontal Cortex, Biol. Psychiatry, 2018, 83, 657–669 CrossRef PubMed.
- M. Seo, C. W. Won, S. Kim, J. H. Yoo, Y. H. Kim and B. S. Kim, The Association of Gait Speed and Frontal Lobe among Various Cognitive Domains: The Korean Frailty and Aging Cohort Study (KFACS), J. Nutr., Health Aging, 2020, 24, 91–97 CrossRef PubMed.
- W. W. Beatty, Memory and “frontal lobe” dysfunction in multiple sclerosis, J. Neurol. Sci., 1993, 115, S38–S41 CrossRef PubMed.
- C. Finke, J. Schlichting, S. Papazoglou, M. Scheel, A. Freing, C. Soemmer, L. Pech, A. Pajkert, C. Pfüller, J. Wuerfel, C. Ploner, F. Paul and A. Brandt, Altered basal ganglia functional connectivity in multiple sclerosis patients with fatigue, Mult. Scler. J., 2015, 21, 925–934 CrossRef PubMed.
- T. Drori, G. Givaty, J. Chapman, M. Lidar, P. Langevitz, Y. Shoenfeld and O. S. Cohen, Extrapyramidal signs in neurosarcoidosis versus multiple sclerosis: Is TNF alpha the link?, Immunobiology, 2018, 223, 259–263 CrossRef PubMed.
- A. V. Ng and J. A. Kent-Braun, Quantitation of lower physical activity in persons with multiple sclerosis, Med. Sci. Sports Exercise, 1997, 29, 517–523 CrossRef CAS PubMed.
- R. L. Lieber, J. O. Fridén, A. R. Hargens and E. R. Feringa, Long-term effects of spinal cord transection on fast and slow rat skeletal muscle, Exp. Neurol., 1986, 91, 435–448 CrossRef.
- A. Fukazawa, A. Koike, T. Karasawa, M. Tsutsui, S. Kondo and S. Terada, Effects of a Ketogenic Diet Containing Medium-Chain Triglycerides and Endurance Training on Metabolic Enzyme Adaptations in Rat Skeletal Muscle, Nutrients, 2020, 12, 1269 CrossRef PubMed.
- P. Galan-Lopez, A. J. Sánchez-Oliver, F. Ries and J. A. González-Jurado, Mediterranean Diet, Physical Fitness and Body Composition in Sevillian Adolescents: A Healthy Lifestyle, Nutrients, 2019, 11, 2009 CrossRef PubMed.
- E. Drehmer, J. L. Platero, S. Carrera-Juliá, M. L. Moreno, A. Tvarijonaviciute, M. Á. Navarro, M. M. López-Rodríguez and J. E. de la Rubia Ortí, The Relation between Eating Habits and Abdominal Fat, Anthropometry, PON1 and IL-6 Levels in Patients with Multiple Sclerosis, Nutrients, 2020, 12(3) DOI:10.3390/nu12030744.
- S. R. Jahromi, M. Toghae, M. J. R. Jahromi and M. Aloosh, Dietary pattern and risk of multiple sclerosis., Iran. J. Neurol., 2012, 11, 47–53 Search PubMed.
- M. M. Cervo, D. Scott, M. Seibel, R. Cumming, V. Naganathan, F. Blyth, D. Le Couteur, D. Handelsman, R. Ribeiro, L. Waite and V. Hirani, Adherence to Mediterranean Diet and Its Associations With Circulating Cytokines, Musculoskeletal Health and Incident Falls in Community-Dwelling Older Men, Curr. Dev. Nutr., 2021, 5, 6 CrossRef.
- I. Katz Sand, S. Levy, K. Fitzgerald, T. Sorets and J. F. Sumowski, Mediterranean diet is linked to less objective disability in multiple sclerosis, Mult. Sclerosis, 2022 DOI:10.1177/13524585221127414.
- R. McClure and A. Villani, Greater adherence to a Mediterranean Diet is associated with better gait speed in older adults with type 2 diabetes mellitus., Clin. Nutr. ESPEN, 2019, 32, 33–39 CrossRef PubMed.
- S. Tepper, A. A. Sivashensky, D. R. Shahar, D. Geva and T. Cukierman-Yaffe, The Association between Mediterranean Diet and the Risk of Falls and Physical Function Indices in Older Type 2 Diabetic People Varies by Age, Nutrients, 2018, 10(6) DOI:10.3390/nu10060767.
- A. H. Snijders, B. P. van de Warrenburg, N. Giladi and B. R. Bloem, Neurological gait disorders in elderly people: clinical approach and classification, Lancet Neurol., 2007, 6, 63–74 CrossRef PubMed.
|
This journal is © The Royal Society of Chemistry 2023 |