DOI:
10.1039/D2FO01835G
(Paper)
Food Funct., 2023,
14, 1520-1529
Cinnamon (Cinnamomum cassia) water extract improves diarrhea symptoms by changing the gut environment: a randomized controlled trial†
Received
29th June 2022
, Accepted 11th January 2023
First published on 19th January 2023
Abstract
Cinnamon is a spice obtained from the bark of Cinnamomum and contains anti-inflammatory ingredients such as coumarin, cinnamaldehyde, and cinnamic acid. This study evaluated the effect of cinnamon water extract (CWE) on the symptoms of subjects with diarrhea in an 8-week randomized controlled trial. Seventy subjects with diarrhea symptoms were randomized and received three capsules of 400 mg CWE or placebo twice daily for 8 weeks. CWE intake significantly increased colonic transit time (p = 0.019) and fecal isobutyric acid (p = 0.008) and spermidine (p = 0.009) contents compared to placebo intake. In contrast, CWE decreased fecal indole (p = 0.032) and agmatine (p = 0.018) contents. Gut microbiota analysis showed increased alpha diversity and significant changes in strains such as Bifidobacterium longum ATCC 55813 (LDA = 1.38) in the CWE group compared with the placebo group. Bifidobacterium longum ATCC 55813 showed a positive correlation with colon transit time and stool phenol and spermidine contents. CWE improved diarrhea symptoms and changed the composition of stools and the gut microbiota. These results indicate that cinnamon intake relieves diarrhea symptoms through metabolic changes due to changes in intestinal microbial groups.
Introduction
Diarrhea is a common condition that occurs through disruptions in gut immune function and homeostasis. Chronic diarrhea is a frequent symptom for both general physicians and gastroenterologists. The differential diagnosis is extensive and can be complex in diagnostic evaluation.1,2 Chronic diarrhea, especially functional diarrhea, is a significant clinical problem. Despite a large number of causes of chronic diarrhea and extensive investigations, no cause of symptoms was found in a large number of patients with functional diarrhea.3 According to the Rome III criteria for diagnosing functional gastrointestinal disorders, functional diarrhea is defined as having loose or watery feces in at least 75% of the feces in the absence of pain.4 There is growing evidence that an imbalance in the intestinal microbiota increases susceptibility to various pathogens and causes many diseases, such as diarrhea, irritable bowel syndrome (IBS), allergies, cardiovascular diseases, and obesity.5
Food digested in our bodies goes to the gut and is metabolized by the gut microbiota.6 The gut microbiota preferentially ferments carbohydrates from nonprotein energy sources, and it can decrease indole production by tryptophan metabolism.7 Microbial fermentation can contribute to the formation of toxic materials, such as p-cresol, which can cause cytotoxicity and reduce barrier protection.8 Dietary fibers and carbohydrates enter the intestinal system and are fermented by microorganisms to form short-chain fatty acids (SCFAs).9 SCFAs maintain intestinal mucosa homeostasis and contribute to mucus production capacity, immune function, and intestinal integrity.10 In addition, biogenic amines produced by the microbiota act as important signaling factors between hosts and symbiotic microorganisms.11
Cinnamon is a spice obtained from the bark of Cinnamomum and has been widely used for medicinal purposes. Cinnamomum cassia bark has treatment effects on inflammation.12 In addition, bioactive materials in C. cassia, such as coumarin, cinnamaldehyde, and cinnamic acid, can reduce the inflammatory response by inhibiting nuclear factor-κB-associated pathways.13,14 Cinnamon is also known to have an inhibitory effect on the growth of certain microbes in vitro.15 However, the effect of C. cassia water extract on intestinal health has not been elucidated through clinical trials.
In this study, it was hypothesized that cinnamon water extract (CWE) prepared through the hot water extraction method would improve diarrhea symptoms in a clinical model by altering the intestinal environment and microbial fermentation products. The relationship between the analyzed biomarkers and gut microbiota was determined through correlation analysis.
Materials and methods
Study materials
CWE was manufactured by Dainnatural (Cheonan, Korea). The C. cassia branch was extracted with hot water as a solvent. Briefly, 100 kg of C. cassia branch were mixed with 10 times distilled water (w/v), and extraction was performed at 121 °C for 8 h. The specimens were filtered and evaporated. After evaporation under reduced pressure at a low temperature, the concentrated extract was converted into dried particles by spray drying.
The experimental capsule was formulated as 96.85% cinnamon extract, 0.97% magnesium stearate, 1.94% silicon dioxide, and 0.24% flavoring. The placebo capsule was formulated as 92.58% corn starch, 0.97% magnesium stearate, 6.22% pigments, and 0.23% flavoring. The extract was formulated to contain 400 mg CWE per capsule. Samples were stored below refrigeration temperature, avoiding sunlight. Subjects received three capsules of CWE or placebo twice daily for 8 weeks. Consumption of 2400 mg of CWE was determined from our previous study16 and calculated as follows. As a result of our previous study, the effect of 500 mg kg−1 cinnamon water extract on gut health improvement was confirmed using a mouse colitis model induced by 5% dextran sodium sulfate.
Quantification of bioactive compounds by ultrahigh-performance liquid chromatography (UHPLC)
For the detection of the compounds in CWE, quantitative analysis was performed on a Vanquish UHPLC coupled with a TSQ Altis (Thermo Fisher, Waltham, MA, USA). Analytes were separated on a Waters Cortects C18 (1.6 μm, 150 mm × 2.1 mm) column by gradient elution using mobile phase A (0.1% formic acid in D. W) and mobile phase B (0.1% formic acid in acetonitrile) at 0.25 mL min−1. Heated electrospray ionization (H-ESI) parameters were as follows: positive ion spray voltage: 3500 V, negative ion spray voltage: 2500 V; sheath gas: 50 (arbitrary units); auxiliary gas: 10 (arbitrary units); sweep gas: 1 (arbitrary units); ion transfer tube temperature 325 °C; vaporizer temperature 350 °C. The triple quadrupole was operated in the selected reaction monitoring (SRM) mode. The samples were determined in triplicate.
Subjects and study design
The study protocol was approved by the Institutional Review Board and Ethics Committees of the Jecheon Oriental Medicine Hospital affiliated with Semyung University (Jecheon, Korea) (approval number 2018-02-02), where the research subjects were recruited, and the Clinical Research Information Service (registration number KCT0003399). Biomarker analysis was performed at Seoul National University of Science and Technology. The study was designed as a randomized, double-blind, parallel, and placebo-controlled study. Randomization was accomplished with a computer-generated random list. Block randomization designs were used to balance the sample size across groups. In this study, sample size determination was performed as follows, based on the comparison means between the two groups. If the observations came from a normal distribution, then with the anticipated standardized effect size between them, based on the results of Shin et al.'s study,17 specified as a result of assuming that the mean ± SD for the change in the number of bowel movements is −0.50 ± 0.64 times, the sample size in each group for a two-sided test α = 0.05 and power 1 − β = 0.8 was 52. Therefore, the number of target subjects per group was 26 each, and a total of 70 subjects were registered (35 per group), considering the 25% dropout rate.
The inclusion criteria were as follows: adults over 20 years of age with diarrhea according to the Rome III criteria (functional diarrhea). The exclusion criteria were as follows: consuming products that affect intestinal function for more than a week within 4 weeks of the first visit; having irritable bowel syndrome, alcoholism, hypertension, cardiovascular disease, immune disease, mental disease, liver function disorder, or renal dysfunction; having high dietary fiber intake; having hypersensitivity to the ingredients within the test food; participating in another clinical study within 4 weeks of the first visit; being pregnant or lactating; and being judged by the researcher as ineligible to participate in this study. Written informed consent was obtained from all subjects prior to study initiation.
After a run-in period of 1 week, subjects were randomly assigned to the placebo group or the test group according to the order registered at visit 2 (week 0). Food containing cinnamon, probiotics, and prebiotics, as well as products in the form of pills and extracts and herbal medicines and drugs, were prohibited until the end of the study. Fruit intake was limited to 2 servings per day, and vegetable intake was limited to 6 servings per day. To compare the differences in nutrient intake among subjects, the dietary intake contents for 3 days (2 weekdays, 1 weekend), representative of the usual diet, were submitted with a smartphone application or diary prior to the study visit. Except for the intake standards, subjects were instructed to maintain a usual diet and lifestyle. To ensure safety, vital sign examinations and clinical pathology and urine tests were performed, and adverse reactions were recorded. Compliance was checked by counting the remaining sample capsules. At 0 and 8 weeks from the start of the test, 10 mL of venous blood, 15 mL of urine, and stool samples were collected.
Assessment of bowel habits
To investigate the effects of CWE on bowel habits, the CTT after sample intake was measured using radio-opaque Kolomark markers (MI Tech, Pyeongtaek, Korea). After 72 hours and 144 hours, abdominal images were taken in the supine position, including the diaphragm and pubis. The CTT value was defined as multiplied by 1.2 by adding all the remaining markers as a result of the imaging. The percentage of bowel movements with diarrhea, satisfaction with defecation, and Bristol stool scale score were recorded as weekly averages. Abdominal pain, urgency, and distension were surveyed at each visit using a visual analog scale (VAS).
Assessment of fecal contents
Phenolic compounds and SCFAs were analyzed with a GC Agilent 6890 N system (Agilent Technologies, Santa Clara, CA, USA) equipped with a flame ionization detector. The compounds were analyzed by the method described in Flickinger et al.18 and Park et al.19 with a DB-17 column (0.25 μm, 30 m × 0.25 mm; Agilent Technologies). 4-Isopropylphenol (Sigma Aldrich Corporation. Ltd, St Louis, MO, USA) was used as an internal standard. The fecal samples were dissolved in 1.0 mL of acetonitrile, vortexed, and sonicated. The emulsion was centrifuged at 3000 rpm for 5 min at 4 °C, and the supernatants were used for analysis.
To analyze SCFAs in feces, the method of Costabile et al. was used.20 Branched SCFAs were used as external standards (Sigma Aldrich Corporation). 2-Ethyl butyric acid was used as an internal standard. Analysis was performed using a DB-FATWAX UI column (0.25 μm, 30 m × 0.25 mm; Agilent Technologies).
Biogenic amines in feces were analyzed with a Shiseido SI-2 HPLC system with a fluorescence detector (Shiseido, Kyoto, Japan) using Saarinen's method.21 The separation of amine components was performed with a Cadenza 5CD-C18 (5 μm, 250 mm × 3 mm) column equipped with a Cadenza 5CD-C18 Guard Cartridge (5 μm, 5 mm × 2 mm; Imtakt USA, Portland, OR, USA). Using heptylamine as an internal standard, the total amounts of each component were summed to obtain the total amine concentration.
Microbiota analysis
The analysis was performed on stool samples obtained before and after CWE ingestion from 16 subjects randomly selected from the CWE group. Stool samples were diluted 1
:
10 in 10 mL of sterilized PBS for 24 hours and filtered with a cell strainer (SPL Life Sciences Co., Ltd, Pocheon, Republic of Korea). Bacteria in stools were isolated by centrifugation at 10
000g for 10 min at 4 °C. The pellet was boiled for 40 min at 100 °C, and a DNeasy PowerSoil Kit (QIAGEN, Hilden, Germany) was used to extract bacterial DNA. Bacterial DNA was quantified by using a QIAxpert system (QIAGEN), and the V3–V4 regions of 16S rDNA were amplified with a MiSeq system (Illumina, San Diego, USA). Taxonomy assignment was carried out by using UCLUST and QIIME against the Greengenes database.
Alpha diversity was assessed using the ACE, Chao1, Observed, Shannon, Simpson, and Fisher indices to compare gut microbiota diversity. Linear discriminant analysis of effect size (LEfSe) analysis was performed to confirm alterations in microbes due to CWE treatment.22 A logarithmic linear discriminant analysis (LDA) score of more than one point was considered to represent a significant difference between two different groups. Correlations with functional markers present in feces were analyzed using Spearman's method for species showing differences before and after CWE ingestion.
Statistical analysis
The analysis was performed using the intention-to-treat method. For comparisons of subject characteristics between groups at baseline, Student's t test was performed for continuous variables. The chi-square test or Fisher's exact test was performed for categorical variables. Intergroup comparisons of compliance were analyzed by Student's t test. To analyze the differences between groups according to the intake period and for group comparisons before and after the intake period, evaluation variables were calculated from a linear mixed-effect model in consideration of group, time (week), and the group × time interaction (group × week). Correlations between biomarkers were analyzed using Spearman's method. Statistical analysis was performed using Statistical Analysis System (Version 9.4; SAS Institute, Cary, NC, USA). Statistical significance was set at p < 0.05.
Results
Baseline characteristics of subjects
The Consolidated Standards of Reporting Trials (CONSORT) flow diagram of this study is shown in Fig. 1. A total of 76 subjects were screened, and 70 subjects who satisfied the inclusion and exclusion criteria were enrolled in the study. The subjects were randomly assigned to either the placebo group or the CWE group, with 35 subjects in each group. Sixty-seven subjects completed the experiment (placebo group, 33 subjects; CWE group, 34 subjects). The analysis was performed with the intention-to-treat method. There were no significant differences in the baseline characteristics (Table S1†) or compliance at the endpoint between groups (Table S2†). The baseline clinical features of the subjects were within the normal range (Tables S3–8†). There were no reports of adverse reactions related to the test materials, and there were no significant adverse events related to the test materials.
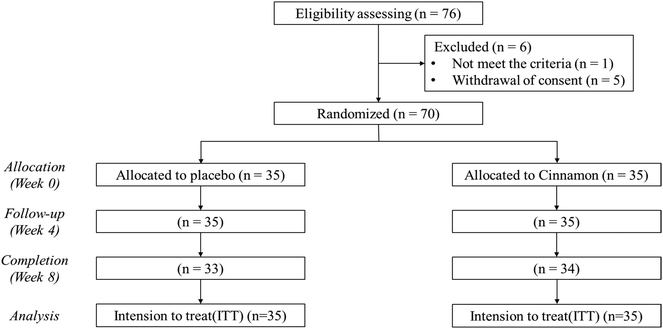 |
| Fig. 1 CONSORT flow diagram of the study. Two people from the placebo group and one person from the CWE group dropped out. A total of 67 people completed the experiment. | |
Bioactive compounds in CWE
Several bioactive components of the CWE are shown in Table 1. Chlorogenic acid, cinnamaldehyde, cinnamic acid, coumarin, isovanillin, p-coumaric acid, protocatechuic acid, quercitrin, salicylic acid, and sinapyl aldehyde were identified and quantified. The highest values were observed in coumarin (9805.88 ± 95.57 mg kg−1), followed by cinnamic acid (2920.83 ± 18.96 mg kg−1).
Table 1 The concentration of bioactive compounds of CWEa
Compounds |
Concentration (mg kg−1) |
Data are described as the mean ± standard deviation.
|
Chlorogenic acid |
100.65 ± 1.35 |
Cinnamaldehyde |
15.33 ± 0.23 |
Cinnamic acid |
2920.83 ± 18.96 |
Coumarin |
9805.88 ± 95.57 |
Isovanillin |
87.53 ± 0.64 |
p-Coumaric acid |
168.22 ± 1.98 |
Protocatechuic acid |
1138.44 ± 42.63 |
Quercitrin |
29.17 ± 0.54 |
Salicylic acid |
119.66 ± 0.83 |
Sinapyl aldehyde |
131.95 ± 0.62 |
CWE improved CTT
Bowel habits were investigated to determine whether diarrhea symptoms were improved. CWE intake prevented a decrease in CTT, while CTT decreased from 25.6 ± 3.4 hours to 15.6 ± 3.4 hours in the placebo group (p < 0.05) and showed a significant difference in the group × week interaction (p = 0.019) (Table 2). Subjects with a percentage of bowel movements with diarrhea of 75% or higher who consumed CWE showed improvements in VAS scores, the percentage of bowel movements with diarrhea, satisfaction with defecation, and Bristol stool scale scores, but these improvements were not statistically significant compared to the placebo group.
Table 2 Effect of cinnamon extract on the bowel habits of subjects (n = 70)a
Variables |
Percentage of bowel movements with diarrhea |
Placebo group (n = 35) |
CWE group (n = 35) |
p valueb |
Week 0 |
Week 8 |
Week 0 |
Week 8 |
Data were described as the LS mean ± standard error.
p values were calculated from a linear mixed-effect model to analyze the effect of the group × week interaction. *p < 0.05 indicates a statistically significant. |
Bowel habits |
Colonic transit time (hours) |
— |
25.6 ± 3.4 |
15.6 ± 3.4 |
19.2 ± 3.4 |
21.7 ± 3.4 |
0.019* |
Percentage of bowel movements with diarrhea |
75–100% |
84.4 ± 11.5 |
51.8 ± 11.5 |
87.8 ± 18.8 |
22.9 ± 18.8 |
0.628 |
50–74% |
62.4 ± 12.0 |
28.4 ± 12.0 |
52.0 ± 12.0 |
36.0 ± 12.0 |
0.622 |
25–49% |
30.6 ± 7.6 |
0.8 ± 8.0 |
35.8 ± 9.3 |
28.9 ± 9.3 |
0.675 |
Satisfaction with defecation (%) |
75–100% |
25.8 ± 13.6 |
48.4 ± 14.8 |
20.0 ± 22.2 |
58.1 ± 22.2 |
0.840 |
50–74% |
37.1 ± 13.3 |
75.4 ± 13.3 |
50.5 ± 13.3 |
66.0 ± 13.3 |
0.603 |
25–49% |
56.4 ± 11.5 |
58.2 ± 12.0 |
56.7 ± 14.0 |
48.9 ± 14.0 |
0.629 |
Bristol stool scale score |
75–100% |
6.0 ± 0.2 |
5.5 ± 0.2 |
6.2 ± 0.3 |
5.9 ± 0.4 |
0.869 |
50–74% |
5.7 ± 0.3 |
5.4 ± 0.3 |
5.8 ± 0.3 |
5.8 ± 0.3 |
0.645 |
25–49% |
5.8 ± 0.3 |
5.7 ± 0.5 |
6.0 ± 0.3 |
6.0 ± 0.3 |
0.885 |
Abdominal pain |
75–100% |
44.2 ± 5.9 |
33.1 ± 6.1 |
36.5 ± 5.9 |
31.9 ± 5.9 |
0.730 |
50–74% |
48.3 ± 7.0 |
32.3 ± 7.4 |
41.3 ± 6.1 |
33.2 ± 6.3 |
0.615 |
25–49% |
35.9 ± 5.8 |
25.0 ± 5.8 |
23.5 ± 6.1 |
10.6 ± 6.1 |
0.886 |
Bowel urgency |
75–100% |
42.3 ± 6.6 |
26.5 ± 6.8 |
45.4 ± 6.6 |
25.4 ± 6.6 |
0.368 |
50–74% |
61.7 ± 8.1 |
49.7 ± 8.4 |
44.2 ± 7.0 |
27.6 ± 7.2 |
0.422 |
25–49% |
36.5 ± 6.9 |
28.6 ± 6.9 |
21.5 ± 7.2 |
19.1 ± 7.2 |
0.868 |
Abdominal distension |
75–100% |
43.1 ± 6.8 |
28.7 ± 7.0 |
49.2 ± 6.8 |
30.8 ± 6.8 |
0.907 |
50–74% |
43.3 ± 7.4 |
23.7 ± 7.8 |
45.4 ± 6.4 |
32.3 ± 6.6 |
0.819 |
25–49% |
37.0 ± 7.0 |
27.7 ± 7.0 |
34.5 ± 7.3 |
18.5 ± 7.3 |
0.768 |
Fecal condition |
pH |
— |
6.4 ± 0.1 |
6.6 ± 0.1 |
6.7 ± 0.1 |
6.8 ± 0.1 |
0.380 |
Water content (%) |
— |
79.6 ± 1.0 |
77.9 ± 1.0 |
79.2 ± 1.0 |
80.9 ± 1.1 |
0.107 |
Fecal indole and phenols (mg g−1) |
Indole |
— |
20.3 ± 1.9 |
21.9 ± 1.9 |
21.6 ± 2.0 |
16.9 ± 2.0 |
0.032* |
Skatole |
— |
2.3 ± 0.8 |
3.8 ± 0.8 |
1.6 ± 0.8 |
2.4 ± 0.8 |
0.598 |
Phenol |
— |
0.2 ± 0.7 |
0.7 ± 0.7 |
3.7 ± 0.7 |
3.1 ± 0.7 |
0.277 |
p-Cresol |
— |
28.9 ± 4.4 |
31.4 ±.4.6 |
24.3 ± 4.7 |
22.9 ± 4.7 |
0.639 |
Fecal short-chain fatty acids (mg g−1) |
Acetic acid |
— |
0.532 ± 0.009 |
0.526 ± 0.009 |
0.517 ± 0.009 |
0.524 ± 0.009 |
0.098 |
Propionic acid |
— |
0.445 ± 0.006 |
0.442 ± 0.006 |
0.450 ± 0.006 |
0.446 ± 0.006 |
0.909 |
Butyric acid |
— |
0.287 ± 0.001 |
0.286 ± 0.001 |
0.287 ± 0.001 |
0.286 ± 0.001 |
0.614 |
Isobutyric acid |
— |
0.579 ± 0.011 |
0.569 ± 0.011 |
0.561 ± 0.011 |
0.573 ± 0.011 |
0.008* |
Valeric acid |
— |
0.389 ± 0.002 |
0.387 ± 0.002 |
0.389 ± 0.003 |
0.388 ± 0.003 |
0.394 |
Isovaleric acid |
— |
0.493 ± 0.002 |
0.492 ± 0.002 |
0.499 ± 0.002 |
0.497 ± 0.002 |
0.434 |
Fecal biogenic amines (μg g−1) |
Agmatine |
— |
217.6 ± 101.6 |
282.0 ± 98.1 |
429.4 ± 98.2 |
27.2 ± 105.6 |
0.018* |
Methylamine |
— |
1762.1 ± 251.2 |
1091.1 ± 261.6 |
1658.1 ± 258.9 |
910.5 ± 270.4 |
0.857 |
Ethylamine |
— |
2966.1 ± 362.2 |
2910.3 ± 357.5 |
3895.3 ± 358.5 |
3363.3 ± 363.2 |
0.392 |
Pyrrolidine |
— |
560.6 ± 140.5 |
661.8 ± 142.8 |
210.9 ± 153.3 |
452.3 ± 153.3 |
0.608 |
Dimethylamine |
— |
127.2 ± 80.7 |
306.9 ± 77.5 |
300.0 ± 79.0 |
327.4 ± 80.0 |
0.217 |
Propylamine |
— |
513.1 ± 62.4 |
460.4 ± 64.1 |
404.1 ± 63.0 |
390.7 ± 61.4 |
0.677 |
Tryptamine |
— |
28.3 ± 5.9 |
12.6 ± 6.0 |
19.3 ± 5.8 |
2.7 ± 6.2 |
0.924 |
Butylamine |
— |
2817.0 ± 460.4 |
2200.8 ± 472.1 |
3331.9 ± 455.0 |
1915.8 ± 492.2 |
0.255 |
Phenylethylamine |
— |
129.9 ± 24.1 |
115.1 ± 25.6 |
95.6 ± 26.1 |
86.1 ± 26.1 |
0.909 |
Putrescine |
— |
3591.2 ± 595.0 |
3225.8 ± 599.3 |
3919.3 ± 599.8 |
4447.6 ± 604.1 |
0.158 |
Cadaverine |
— |
2557.2 ± 292.4 |
2096.6 ± 296.1 |
3345.1 ± 296.1 |
2787.8 ± 300.1 |
0.829 |
Histamine |
— |
436.6 ± 71.6 |
324.7 ± 73.7 |
447.5 ± 70.5 |
370.4 ± 69.9 |
0.684 |
Tyramine |
— |
3598.8 ± 754.2 |
3538.1 ± 764.1 |
3730.3 ± 754.2 |
3956.2 ± 756.4 |
0.568 |
Spermidine |
— |
471.7 ± 51.3 |
329.5 ± 53.6 |
404.8 ± 52.1 |
501.4 ± 53.6 |
0.009* |
Spermine |
— |
1069.7 ± 116.6 |
1120.5 ± 120.6 |
911.3 ± 120.6 |
885.1 ± 116.6 |
0.727 |
Total amine |
— |
27183.8 ± 2319.0 |
23136.3 ± 2338.3 |
27374.5 ± 2354.5 |
26 106.1 ± 2374.7 |
0.306 |
CWE changed fecal contents
The contents of indole, phenol, SCFAs, and biogenic amines in the feces changed with the consumption of CWE. No significant change was found in the indole content of the placebo group; however, the indole content decreased significantly, by 21.8%, from 21.6 ± 2.0 to 16.9 ± 2.0 mg g−1 in the CWE group (p < 0.05), demonstrating a significant group × week interaction (p = 0.032). Except for skatole, other phenolic compounds decreased in the placebo group and increased in the CWE group, but there was no significant difference. CWE did not change the stool skatole content, p-cresol content, pH, or water content (Table 2).
As a result of analyzing the content of SCFAs in stool samples, no significant change was found in the fecal isobutyric acid content with placebo ingestion; conversely, CWE ingestion significantly increased the isobutyric acid content, from 0.561 ± 0.011 to 0.573 ± 0.011 mg g−1 (p < 0.05), and it showed a significant difference in the group × week interaction (p = 0.008). CWE did not change the content of other SCFAs (Table 2).
The fecal biogenic amine contents of the subjects were confirmed. Agmatine in the placebo group showed no significant change, but CWE intake led to a 93.7% decrease in agmatine, from 429.4 ± 98.2 to 27.2 ± 105.6 μg g−1 (p < 0.05), and it demonstrated a significant difference in the group × week interaction (p = 0.018). Spermidine significantly decreased by 30.2%, from 471.7 ± 51.3 to 329.5 ± 53.6 μg g−1 (p < 0.05), in the placebo group, while the CWE group showed no significant difference. As a result, a significant difference in the group × week interaction was found for spermidine content (p = 0.009) (Table 2).
CWE altered the gut microbiota
The microbiota composition in stool samples was analyzed by 16S rRNA sequencing using feces obtained from the CWE group (n = 16). After CWE intake, Verrucomicrobia, Cyanobacteria, Saccharibacteria, and Actinobacteria were increased approximately 2.5-fold, 1.8-fold, 1.4-fold, and 1.2-fold, respectively, and at the phylum level, Tenericutes was reduced by one-quarter. Additionally, the Firmicutes/Bacteroidetes ratio decreased by 16%, confirming that Bacteroidetes increased relative to the phylum Firmicutes (Fig. 2A). Alpha-diversity analysis was performed to confirm changes in the richness and evenness of the intestinal microbiota (Fig. 2B). Significant differences were found in the observed, Chao1, ACE, Simpson, and Fisher indices but not in the Shannon index (p < 0.05).
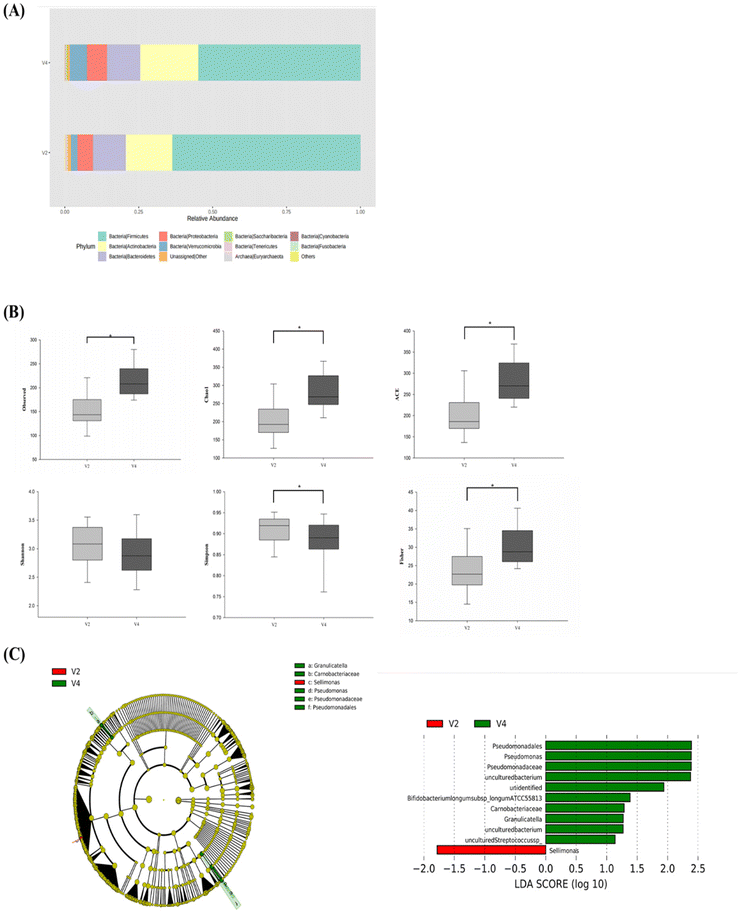 |
| Fig. 2 (A) Altered microbiota composition after the ingestion of CWE, analyzed at the phylum level. (B) Alpha-diversity analysis was performed at the species level based on paired-sample t tests and the Wilcoxon rank sum test. *p < 0.05. (C) LEfSe cladogram shows differences in the compositions of the microbiota from the phylum to the genus level before and after CWE ingestion. The Y axis represents the taxa with significant differences, while the X axis visually depicts the LDA score of the corresponding taxa by a bar chart (LDA score ≥1). | |
LEfSe analysis was performed to confirm the change in c from the phylum to species level between the groups. In Fig. 2C, Sellimonas was significantly abundant before consuming CWE (LDA = −1.78). After CWE ingestion, uncultured Pseudomonas, unidentified Bifidobacterium, Bifidobacterium longum ATCC 55813, uncultured Granulicatella, and uncultured Streptococcus were significantly increased (LDA = 2.38, 1.94, 1.38, 1.27, 1.14).
The correlation between biomarkers and changes in the gut microbiota was analyzed by Spearman's method. Fig. 3 shows the relationship between the two variables. Blue indicates a negative correlation, and red indicates a positive correlation. The uncultured species of Bifidobacterium, which increased after the ingestion of CWE, showed a negative correlation with an improvement in the number of bowel movements with diarrhea and the Bristol stool scale score (r = −0.359, −0.359; p < 0.05). Bifidobacterium longum ATCC 55813 showed a positive correlation with the amounts of phenol and spermidine and CTT (r = 0.350, 0.494, 0.376; p < 0.05). Conversely, Bifidobacterium longum ATCC 55813 showed a negative correlation between the number of bowel movements with diarrhea and the Bristol stool scale score (r = −0.528, −0.466; p < 0.05). The uncultured species of Streptococcus showed a correlation with an improvement in the Bristol stool scale score (r = −0.373; p < 0.05).
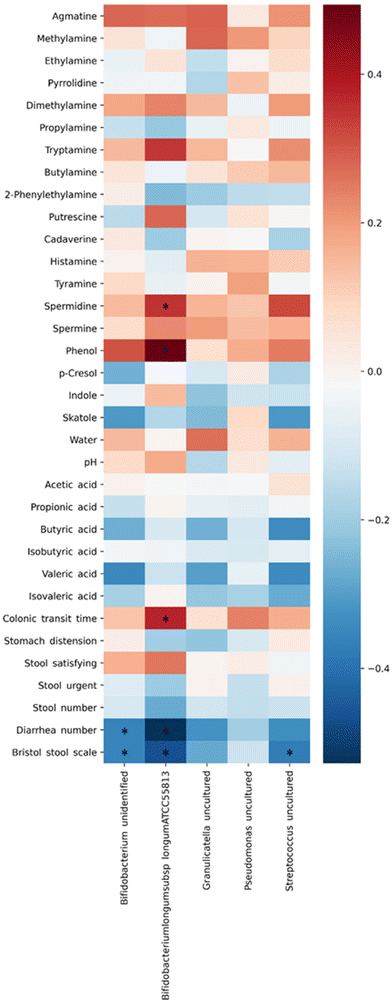 |
| Fig. 3 Correlation analysis between the gut microbiota and biomarkers in feces based on Spearman's method. Blue indicates a negative correlation, and red shows a positive correlation. *p < 0.05. | |
Discussion
Cinnamon is a plant that has been used for a long time due to its unique fragrance. In a previous study, C. cassia was shown to alleviate inflammation and inhibit specific microbes.15,23C. cassia is spicy but bitter in taste and high in coumarin.24 Moreover, coumarin and cinnamic acid, a component of cinnamon, can reduce the inflammatory response by inflammatory cytokines.13,14 Hot water extraction is an efficient way to extract coumarin and cinnamic acid from C. cassia.25 Hot water extraction also has the advantage of being less toxic than ethanol extraction.26 Therefore, in this study, the effects of CWE prepared through the hot water extraction method on relieving symptoms of diarrhea were investigated through a clinical trial.
CTT is a representative indicator of diarrhea and plays an important role in the formation of metabolites and microbiota alterations.27 Improved CTT in the transverse colon increases SCFAs.28 SCFAs strengthen junctional complexes and upregulate tight junction assembly by engaging the 5′ adenosine monophosphate-activated protein kinase pathway.29 CTT and isobutyric acid were significantly increased in the CWE group. Isobutyric acid is a branched-chain fatty acid produced in the process of fermenting branched-chain amino acids, such as valine, leucine, and isoleucine, by the intestinal flora.30 This finding suggests the possibility that the increased isobutyric acid resulting from CWE ingestion might improve gut health and alleviate inflammation. CWE also decreased fecal indole concentrations. Indole is also known as an interspecies signaling molecule, and the composition of the gut microbiota can control the indole concentration.31 The amount of agmatine formed by the intestinal microflora is an important physiological source of agmatine in human organisms. Various types of intestinal bacteria differ greatly in their ability to form agmatine. Therefore, the amount of absorbable agmatine should vary considerably depending on the composition of the bacterial flora.32
The microbial phylum ratio is associated with gut health indicators such as alpha diversity. CWE decreased the Firmicutes/Bacteroidetes ratio, which can be seen as a marker of improved alpha diversity in subjects.33 Increased diversity of the gut microbiota leads to resistance to perturbations, such as inflammatory responses, antibiotics, and external stimuli.34 LEfSe analysis showed that CWE ingestion significantly increased Bifidobacterium, Granulicatella, Streptococcus, and Pseudomonas and decreased Sellimonas (LDA score ≥ 1.0). Streptococcus and Pseudomonas can biosynthesize spermidine by catabolizing agmatine during arginine and proline metabolism.35,36 Increased spermidine production by the microbiota improves permeability and suppresses the inflammatory response.37 Some Pseudomonas species, such as P. aeruginosa, have the ability to degrade indoles and enhance their biofilms in indole-rich environments.38,39 This fact suggests that the decreased fecal indole concentration through the ingestion of cinnamon is due to an increase in the genus Pseudomonas. However, there was no significant difference observed in the correlation analysis, so verification through future studies will be needed.
Correlation analyses between specific microbes and stool contents and functional biomarkers, such as an increase in CTT and a decrease in the number of bowel movements with diarrhea and the Bristol stool scale score, showed a positive correlation with uncultured species of Bifidobacterium and Bifidobacterium longum ATCC 55813. Additionally, Bifidobacterium longum ATCC 55813 showed a positive correlation with spermidine and phenol in feces. Bifidobacterium longum has the ability to produce spermidine via an unknown pathway.40 Studies have reported that Bifidobacterium longum can significantly reduce diarrhea in children.41 Uncultured species of Streptococcus showed a negative correlation with the Bristol stool scale score, and no significant correlation was found in the uncultured species of Pseudomonas and Granulicatella. These findings demonstrate that the intestinal microbiota, especially Bifidobacterium, was altered through the ingestion of CWE, which can improve gut health and alleviate diarrhea symptoms.
In conclusion, the ingestion of CWE improved CTT shortened by diarrhea. Moreover, CWE altered the biogenic amines, SCFAs, and indole in the stools of subjects with diarrhea by altering microorganism composition and metabolism in the human body. Coumarin and cinnamic acid were likely partially responsible for the effects of CWE, suggesting additive effects with other active compounds of CWE. In a previous study, polyphenol-rich cinnamon bark extract possessed antimicrobial activity against the tested Gram-positive bacteria, producing an increase in cinnamic acid in vitro digestion. In addition, cinnamon bark extract was found to exhibit antitumor effects on colon cell lines implemented in the presence of probiotic fermentation.42 In this study, CWE, characterized by the presence of coumarin and cinnamic acid, showed the possibility of relieving diarrhea symptoms by reorganizing the intestinal environment. However, this study has certain limitations. The present study could not confirm the complex effects that may arise from the interaction of the intestinal environment and changes in the body state according to the metabolism of each microorganism. Further studies should investigate the potential effects of metabolic changes in each microbiota on colonic disease and evaluate the efficacy of CWE for diarrhea improvement with other drugs or alternatives, such as oligosaccharides.
Author contributions
Soo-yeon Park analyzed and interpreted the results and edited the draft. Yong Dae Kim performed the formal analysis and wrote the first draft of the manuscript. Min Seo Kim conceptualized the experiments and methodology. Ki-Tae Kim provided resources and performed investigations. Ji Yeon Kim reviewed and edited the draft, supervised the study, and provided project administration. All authors meet the CRediT criteria for authorship and have approved the final version of the manuscript for publication.
Conflicts of interest
There are no conflicts of interest to declare.
Acknowledgements
This work was supported by the Korea Institute of Planning and Evaluation for Technology in Food, Agriculture, Forestry and Fisheries (IPET) through the High Value-added Food Technology Development Program, funded by the Ministry of Agriculture, Food and Rural Affairs (MAFRA) (Project No. 116012-3), and National Research Foundation of Korea (NRF) grant funded by the Ministry of Science and ICT (No. 2021R1A2C2009309).
References
- K. D. Fine and L. R. Schiller, AGA technical review on the evaluation and management of chronic diarrhea, Gastroenterology, 1999, 116, 1464–1486 CrossRef CAS PubMed.
- L. R. Schiller, Chronic diarrhea, Gastroenterology, 2004, 127, 287–293 CrossRef PubMed.
- G. F. Longstreth, W. G. Thompson, W. D. Chey, L. A. Houghton, F. Mearin and R. C. Spiller, Functional bowel disorders, Gastroenterology, 2006, 130, 1480–1491 CrossRef PubMed.
- J. Tack, Functional diarrhea, Gastroenterol. Clin., 2012, 41, 629–637 CrossRef PubMed.
- Y. Li, S. Xia, X. Jiang, C. Feng, S. Gong, J. Ma, Z. Fang, J. Yin and Y. Yin, Gut microbiota and diarrhea: an updated review, Front. Cell. Infect. Microbiol., 2021, 11, 625210 CrossRef PubMed.
- W. J. Dahl, D. R. Mendoza and J. M. Lambert, Diet, nutrients and the microbiome, Prog. Mol. Biol. Transl. Sci., 2020, 171, 237–263 CAS.
- J. Gao, K. Xu, H. Liu, G. Liu, M. Bai, C. Peng, T. Li and Y. Yin, Impact of the Gut Microbiota on Intestinal Immunity Mediated by Tryptophan Metabolism, Front. Cell. Infect. Microbiol., 2018, 8, 13–13 CrossRef PubMed.
- Y. Saito, T. Sato, K. Nomoto and H. Tsuji, Identification of phenol- and p-cresol-producing intestinal bacteria by using media supplemented with tyrosine and its metabolites, FEMS Microbiol. Ecol., 2018, 94, 1–13 CrossRef PubMed.
- C. Martin-Gallausiaux, L. Marinelli, H. M. Blottière, P. Larraufie and N. Lapaque, SCFA: mechanisms and functional importance in the gut, Proc. Nutr. Soc., 2021, 80, 37–49 CrossRef CAS PubMed.
- E. E. Blaak, E. E. Canfora, S. Theis, G. Frost, A. K. Groen, G. Mithieux, A. Nauta, K. Scott, B. Stahl, J. van Harsselaar, R. van Tol, E. E. Vaughan and K. Verbeke, Short chain fatty acids in human gut and metabolic health, Benefic. Microbes, 2020, 11, 411–455 CrossRef CAS PubMed.
- N. Sudo, Biogenic Amines: Signals Between Commensal Microbiota and Gut Physiology, Front. Endocrinol., 2019, 10, 504–504 CrossRef PubMed.
- N. Mahmood, R. Nazir, M. Khan, A. Khaliq, M. Adnan, M. Ullah and H. Yang, Antibacterial Activities, Phytochemical Screening and Metal Analysis of Medicinal Plants: Traditional Recipes Used against Diarrhea, Antibiotics, 2019, 8, 194 CrossRef CAS PubMed.
- E. A. Ibrahim, F. S. M. Moawed and E. M. Moustafa, Suppression of inflammatory cascades via novel cinnamic acid nanoparticles in acute hepatitis rat model, Arch. Biochem. Biophys., 2020, 696, 108658 CrossRef CAS PubMed.
- J.-C. Liao, J.-S. Deng, C.-S. Chiu, W.-C. Hou, S.-S. Huang, P.-H. Shie and G.-J. Huang, Anti-inflammatory activities of Cinnamomum cassia constituents in vitro and in vivo, Evidence-Based Complementary Altern. Med., 2012, 2012, 429320 Search PubMed.
- H.-S. Lee and Y.-J. Ahn, Growth-Inhibiting Effects of Cinnamomum cassia Bark-Derived Materials on Human Intestinal Bacteria, J. Agric. Food Chem., 1998, 46, 8–12 CrossRef CAS PubMed.
- M. S. Kim, Y. D. Kim, S. Kang, O. Kwon, J.-H. Shin and J. Y. Kim, Cinnamon (Cinnamomum japonicum) subcritical water extract suppresses gut damage induced by dextran sodium sulfate in mouse colitis model, J. Funct. Foods, 2021, 87, 104775 CrossRef CAS.
- S. P. Shin, Y. M. Choi, W. H. Kim, S. P. Hong, J.-M. Park, J. Kim, O. Kwon, E. H. Lee and K. B. Hahm, A double blind, placebo-controlled, randomized clinical trial that breast milk derived-Lactobacillus gasseri BNR17 mitigated diarrhea-dominant irritable bowel syndrome, J. Clin. Biochem. Nutr., 2018, 62, 179–186 CrossRef CAS PubMed.
- E. A. Flickinger, E. M. Schreijen, A. R. Patil, H. S. Hussein, C. M. Grieshop, N. R. Merchen and G. C. Fahey Jr., Nutrient digestibilities, microbial populations, and protein catabolites as affected by fructan supplementation of dog diets, J. Anim. Sci., 2003, 81, 2008–2018 CrossRef CAS PubMed.
- K. Park, M. Kim, K. Woo, N. Lee and H. Paik, Rapid Analysis of Major Putrefactive Metabolites by GC and GC/MSD, Korean J. Microbiol. Biotechnol., 2003, 18, 74–77 Search PubMed.
- A. Costabile, A. Klinder, F. Fava, A. Napolitano, V. Fogliano, C. Leonard, G. R. Gibson and K. M. Tuohy, Whole-grain wheat breakfast cereal has a prebiotic effect on the human gut microbiota: a double-blind, placebo-controlled, crossover study, Br. J. Nutr., 2008, 99, 110–120 CrossRef CAS PubMed.
- M. T. Saarinen, Determination of biogenic Amines as dansyl derivatives in intestinal digesta and Feces by reversed phase HPLC, Chromatographia, 2002, 55, 297–300 CrossRef CAS.
- N. Segata, J. Izard, L. Waldron, D. Gevers, L. Miropolsky, W. S. Garrett and C. Huttenhower, Metagenomic biomarker discovery and explanation, Genome Biol., 2011, 12, R60 CrossRef PubMed.
- H. K. Kwon, J. S. Hwang, J. S. So, C. G. Lee, A. Sahoo, J. H. Ryu, W. K. Jeon, B. S. Ko, S. H. Lee, Z. Y. Park and S. H. Im, Cinnamon extract induces tumor cell death through inhibition of NFkappaB and AP1, BMC Cancer, 2010, 10, 392 CrossRef PubMed.
-
P. Mishra, S. Devi, M. Sahu, E. Gupta and H. Prakash, Miracle & Functional Spice: Cinnamon, Advances in Essential Oils and Natural Products, AkiNik Publications, 2020, pp. 37–43 Search PubMed.
- M. Akkawi, S. Abu-Lafi, H. A. Attieh, Q. Abu-Remeleh, S. Makhamra and M. Qutob, Preparative HPLC fractionation of Cinnamomum cassia Water Extract and their in vitro Antimalarial Activities, J. Appl. Pharm. Sci., 2017, 7, 129–134 CrossRef CAS.
- Y. Cheng, F. Xue, S. Yu, S. Du and Y. Yang, Subcritical Water Extraction of Natural Products, Molecules, 2021, 26, 4004 CrossRef CAS PubMed.
- H. M. Roager, L. B. Hansen, M. I. Bahl, H. L. Frandsen, V. Carvalho, R. J. Gøbel, M. D. Dalgaard, D. R. Plichta, M. H. Sparholt, H. Vestergaard, T. Hansen, T. Sicheritz-Pontén, H. B. Nielsen, O. Pedersen, L. Lauritzen, M. Kristensen, R. Gupta and T. R. Licht, Colonic transit time is related to bacterial metabolism and mucosal turnover in the gut, Nat. Microbiol., 2016, 1, 16093 CrossRef CAS PubMed.
- W. Tottey, D. Feria-Gervasio, N. Gaci, B. Laillet, E. Pujos, J. F. Martin, J. L. Sebedio, B. Sion, J. F. Jarrige, M. Alric and J. F. Brugère, Colonic Transit Time Is a Driven Force of the Gut Microbiota Composition and Metabolism: In Vitro Evidence, J. Neurogastroenterol. Motil., 2017, 23, 124–134 CrossRef PubMed.
- J. P. B. Silva, K. C. Navegantes-Lima, A. L. B. Oliveira, D. V. S. Rodrigues, S. L. F. Gaspar, V. V. S. Monteiro, D. P. Moura and M. C. Monteiro, Protective Mechanisms of Butyrate on Inflammatory Bowel Disease, Curr. Pharm. Des., 2018, 24, 4154–4166 CrossRef CAS PubMed.
- Y. Gao, H. Chen, J. Li, S. Ren, Z. Yang, Y. Zhou and R. Xuan, Alterations of gut microbiota–derived metabolites in gestational diabetes mellitus and clinical significance, J. Clin. Lab. Anal., 2022, 36, e24333 CAS.
- J.-H. Lee, T. K. Wood and J. Lee, Roles of Indole as an Interspecies and Interkingdom Signaling Molecule, Trends Microbiol., 2015, 23, 707–718 CrossRef CAS PubMed.
- B. Haenisch, I. von Kügelgen, H. Bonisch, M. Göthert, T. Sauerbruch, M. Schepke, G. n. Marklein, K. Höfling, D. Schröder and G. J. Molderings, Regulatory mechanisms underlying agmatine homeostasis in humans, Am. J. Physiol.: Gastrointest. Liver Physiol., 2008, 295, G1104–G1110 CrossRef CAS PubMed.
- O. Manor, C. L. Dai, S. A. Kornilov, B. Smith, N. D. Price, J. C. Lovejoy, S. M. Gibbons and A. T. Magis, Health and disease markers correlate with gut microbiome composition across thousands of people, Nat. Commun., 2020, 11, 5206 CrossRef CAS PubMed.
- M. Fassarella, E. E. Blaak, J. Penders, A. Nauta, H. Smidt and E. G. Zoetendal, Gut microbiome stability and resilience: elucidating the response to perturbations in order to modulate gut health, Gut, 2021, 70, 595–605 CrossRef CAS PubMed.
- J. M. Luengo and E. R. Olivera, Catabolism of biogenic amines in Pseudomonas species, Environ. Microbiol., 2020, 22, 1174–1192 CrossRef CAS PubMed.
- A. J. Potter and J. C. Paton, Spermidine biosynthesis and transport modulate pneumococcal autolysis, J. Bacteriol., 2014, 196, 3556–3561 CrossRef PubMed.
- B. Morón, M. Spalinger, S. Kasper, K. Atrott, I. Frey-Wagner, M. Fried, D. F. McCole, G. Rogler and M. Scharl, Activation of protein tyrosine phosphatase non-receptor type 2 by spermidine exerts anti-inflammatory effects in human THP-1 monocytes and in a mouse model of acute colitis, PLoS One, 2013, 8, e73703 CrossRef PubMed.
- S. K. Kim, H. Y. Park and J. H. Lee, Anthranilate deteriorates the structure of Pseudomonas aeruginosa biofilms and antagonizes the biofilm-enhancing indole effect, Appl. Environ. Microbiol., 2015, 81, 2328–2338 CrossRef CAS PubMed.
- N. Doukyu and R. Aono, Biodegradation of indole at high concentration by persolvent fermentation with Pseudomonas sp. ST-200, Extremophiles, 1997, 1, 100–105 CrossRef CAS PubMed.
- Y. Sugiyama, M. Nara, M. Sakanaka, A. Kitakata, S. Okuda and S. Kurihara, Analysis of polyamine biosynthetic- and transport ability of human indigenous Bifidobacterium, Biosci., Biotechnol., Biochem., 2018, 82, 1606–1614 CrossRef CAS PubMed.
- D. K. Lee, J. E. Park, M. J. Kim, J. G. Seo, J. H. Lee and N. J. Ha, Probiotic bacteria, B. longum and L. acidophilus inhibit infection by rotavirus in vitro and decrease the duration of diarrhea in pediatric patients, Clin. Res. Hepatol. Gastroenterol., 2015, 39, 237–244 CrossRef PubMed.
- A. De Giani, S. Pagliari, J. Zampolli, M. Forcella, P. Fusi, I. Bruni, L. Campone and P. Di Gennaro, Characterization of the Biological Activities of a New Polyphenol-Rich Extract from Cinnamon Bark on a Probiotic Consortium and Its Action after Enzymatic and Microbial Fermentation on Colorectal Cell Lines, Foods, 2022, 11, 3202 CrossRef CAS.
Footnote |
† Electronic supplementary information (ESI) available: Table S1: Baseline demographic characteristics of study participants. Table S2: Compliance. Table S3: Dietary intake and physical activity. Table S4: Vital signs. Table S5: Hematological test results. Table S6: Blood chemistry test results. Table S7: Urine test results. Table S8: Normal ranges for hematological test, blood chemical test, and urine test results. Fig. S1: Altered microbiota composition after the ingestion of CWE, analyzed at the (A) family and (B) genus level. See DOI: https://doi.org/10.1039/d2fo01835g |
|
This journal is © The Royal Society of Chemistry 2023 |
Click here to see how this site uses Cookies. View our privacy policy here.