Cellulose-based natural nanofibers for fresh produce packaging: current status, sustainability and future outlook
Received
3rd May 2023
, Accepted 18th May 2023
First published on 16th June 2023
Abstract
In recent years, the packaging of fresh produce has attracted the attention of researchers. The development of eco-friendly and biodegradable packaging materials with exceptional mechanical and barrier properties has reached great heights in research and development. To minimize the use of synthetic materials, natural nanofibers serve as an alternative to synthetic food packaging materials. This article focuses on the derivation of natural nanofibers (NNFs) from various sources along with their different production routes in detail. An in-depth analysis was performed to obtain the desired properties of natural nanofiber-based food packaging materials and modifications using their active agents to extend the shelf life of fresh produce. The article also presents a comprehensive analysis of the life cycle assessment and sustainability of natural nanofibers along with an outlook on its future prospects.
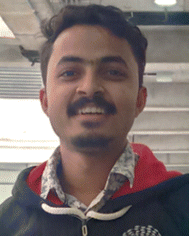 Partha Pratim Das | Partha Pratim Das is pursuing his Master of Technology (Materials Science and Metallurgical Engineering) in the Department of Materials Science and Metallurgical Engineering at Indian Institute of Technology Hyderabad (IITH), India. Currently, he is working in the field of active food packaging to extend the shelf-life of fresh produce with the Cellulose and Composites Research Group at IIT Hyderabad. He completed his B.Tech in Mechanical Engineering with first-class distinction in the year 2021 from Amity University Uttar Pradesh, Noida, India. He has presented several research papers at national and international conferences and published a good number of research papers in SCI journals and book chapters with reputed publishers including Springer, CRC Press (T&F), and Elsevier. His area of research includes natural fibre-based composites, processing and characterization of polymer matrix composites, and biodegradable food packaging materials. |
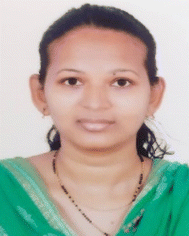 Peddapapannagari Kalyani | Peddapapannagari Kalyani is a research scholar in the Department of Materials Science and Metallurgical Engineering at Indian Institute of Technology Hyderabad. Her research work focuses on the tuning of nanocellulose based carriers for transdermal drug delivery system and active food packaging applications. She is also interested in research areas like novel drug delivery systems for chronic diseases (like diabetes, arthritis, pain management, wound healing and hormonal management, etc.) and sustainable food packaging systems for fresh fruits and vegetables. |
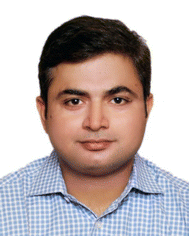 Rahul Kumar | Dr Rahul Kumar is currently working as an Assistant Professor at the Department of Plant Sciences, University of Hyderabad. His lab focuses on understanding the hormonal regulation of fruit ripening and root system architecture plasticity under low P-stress in plants using tomato and Arabidopsis as model systems. He is also interested in understanding the host genetic regulatory control mechanisms controlling mycorrhizal symbiosis. In a collaborative study, his lab has recently unraveled ERF.D7 mediated regulation of tomato fruit ripening. In another breakthrough collaborative study, his lab has reported the critical role of methylglyoxal in tomato fruit ripening. |
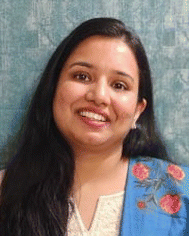 Mudrika Khandelwal | Dr Mudrika Khandelwal is an Associate Professor in the Department of Materials Science and Metallurgical Engineering at Indian Institute of Technology Hyderabad. Her group is focused on exploring nanofibrous materials for energy, health and the environment. In particular the group develops materials for food packaging, drug delivery, and energy storage and conversion. |
Sustainability spotlight
The commonly existing packaging is made of synthetic plastic for keeping food fresh and plastic dominates and is widely used in food packaging due to its low cost of production, mechanical resistance, ability to be heat sealed, and shape flexibility. But plastic packaging has the drawback of not degrading naturally but harming the ecosystem and the health of both terrestrial and marine species. In this regard, natural material-derived packaging materials have the potential to revolutionize the packaging industry, particularly in the area of sustainability and green food packaging. The use of cellulose-based natural nanofibers in the packaging of fresh produce can provide several benefits and gain importance globally. Our work emphasizes the importance of UN sustainability development goals (SDG 2023): zero hunger (SDG 2), industry, innovation and infrastructure (SDG 9), and responsible consumption and production (SGD 12).
|
1. Introduction
Fruits and vegetables are the basic commodities that belong to a category of plants that vary widely in terms of their nutrient content and everyday consumption. They are regarded as being healthy and contain vitamins, minerals, and other phytochemicals that can support the body's efficient operation and act as a defence against certain ailments. Active food packaging of fruits and vegetables (as harvested as well as fresh cut) has become important in recent years due to the need for convenience goods for customers and to maintain freshness for a longer duration.1,2 In general, water loss, microbial growth, discolouration due to oxidation, deterioration of texture and flavour, an increase in respiration rate, and the ripening process are responsible for the deterioration of fresh food quality and reduction in shelf life.3,4 To ensure that the global food supply remains secure, minimize wastage, reduce the economic burden on the public and improve ready availability throughout the year, the shelf-life extension of fresh produce becomes crucial. As fresh produce like fruits and vegetables may have a higher level of contamination after harvest, there is an increase in susceptibility to infection, which causes it to degrade quickly. Hence, maintaining the freshness of fruits and vegetables with sufficient packing became a top priority.5–7 Newer items are being introduced in the market in response to consumer demands motivated by nutritious content, trends, knowledge, tastes, exposure, and safety. Further, recently fresh-cut vegetables and fruits are also being made available commercially to support evolving lifestyles. Fresh produce requires food packaging to safeguard the products and distribute them to consumers in a secure manner as well as to extend the shelf life by using natural active agents. Commonly existing packaging is made of synthetic plastic for keeping food fresh. Plastic dominates and is widely used in food packaging due to its low cost of production, mechanical resistance, ability to be heat sealed, and shape flexibility. But plastic packaging does have the drawback of not degrading naturally, harming the ecosystem and the health of both terrestrial and marine species. Biodegradable polymers have the potential to revolutionize the packaging industry, particularly in the area of sustainability and green food packaging. Hence, to establish a passive or active packaging system for fresh fruits and vegetables, they are placed in packaging films and active agents are used inside the packaging respectively.8,9Fig. 1 shows a broad classification of biodegradable polymers used for food packaging.
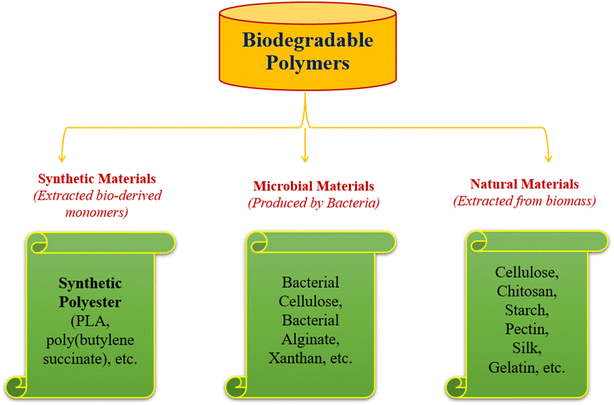 |
| Fig. 1 Broad classification of biodegradable polymers. | |
Plant cellulose can be processed into various forms including microfibers, microcrystalline cellulose nanofibers, nanowhiskers and nanocrystals, a property that makes them suitable for various applications. Each form offers specific advantages as raw materials. The use of nanofibers in the packaging of fruits and vegetables can provide several benefits. First, the small size of nanofibers means that they can be used to create extremely thin films or coatings that are transparent, lightweight, and flexible.10 The use of nanofibers in the packaging of fruits and vegetables can offer excellent barrier properties against water vapour, oxygen, and other gases due to their high surface area-to-volume ratio which can help to prevent spoilage and extend the shelf life of fresh produce. This is particularly important for fruits and vegetables, which are highly perishable and require careful handling and storage to maintain their quality. Cellulose has gained significant attention due to its abundance in nature, economical nature, non-toxic properties, etc. which make it a promising material for various applications.11 Nanofibers produced from plant-based cellulose through electrospinning, known as cellulose nanofibers (CNF), are a type of nanomaterial that can be produced in the range of 1–100 nm. CNF is used as a food packaging material due to its unique mechanical and barrier properties that can improve the shelf life and quality of fresh produce. It is produced from renewable and biodegradable materials, such as cellulose from plants, which can help to reduce the environmental impact of packaging waste. Overall, the use of cellulose nanofibers in packaging fruits and vegetables has the potential to improve the freshness, quality, and sustainability of fresh produce, which can benefit both consumers and the environment. However, further research and development are needed to optimize the properties and performance of cellulose-based nanofiber-based packaging materials for this application.12 Generally, CNF is used as a sustainable food packaging film as an alternative option to plastic packaging material. Later modifications are done by using active agents which are effectively embedded in the cellulose nanofiber packaging material for gas adsorption and antimicrobial activity, and optimize the barrier properties by slowing down the respiration rate, which helps to extend the shelf life. The active agents embedded in the CNF packaging material could trigger ethylene gas release, adsorb oxygen and carbon dioxide, and inhibit the microbial infection inside the packaging making the fruits and vegetables look fresh and nutrient. Researchers are moving towards finding new natural materials which are eco-friendly and sustainable but preferred, especially in the form of nanofibers as they are lightweight with small diameters, and have controllable pore structures and high surface-to-volume ratio. The high surface-to-volume ratio of cellulose nanofibrous materials is beneficial for the integration and enhancement of added active ingredients. Fresh produce has a longer shelf life when treated with active agents such as ethylene adsorbers, moisture absorbers or oxygen/carbon scavengers.13–16Fig. 2 illustrates the number of articles published in the field of natural nanofibers used for food packaging and their development, as well as the increase in the importance of packaging made based on natural nanofibers. A summary of the reported studies on natural nanofiber-based food packaging is presented in Table 1.
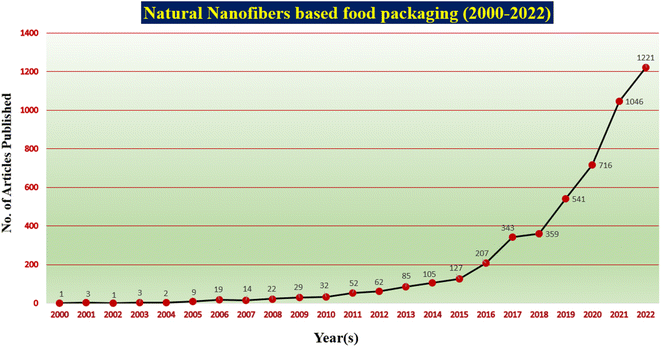 |
| Fig. 2 Number of articles published on natural nanofiber-based food packaging (source: https://www.sciencedirect.com/; keywords used: natural nanofiber, food packaging). | |
Table 1 Reported studies on nanofiber-based food packaging
Materials |
Packaging forms |
Applications |
Outcome |
References |
Cellulose/banana peel nanofibers |
Composite film |
Food packaging |
Good mechanical properties |
13
|
Cellulose/ginger nanofibers |
Composite film |
Food packaging |
Improved antimicrobial properties |
14
|
Cellulose/SB nanofibers |
Composite film |
Food packaging |
Improved barrier properties |
15
|
NC-nanofibers |
Composite film |
Fresh cut spinach |
Reduces the respiration of the leaves, which contributes to the extension of shelf life |
16
|
NC/PLA nanofibers |
Composite film |
Food packaging |
The films exhibit improved tensile properties, wettability and reduced respiration in the packaging |
17
|
Cellulose/phenolic acid nanofibers |
Active packaging film |
Food packaging |
Provides good antioxidant and antimicrobial properties |
18
|
Chitosan nanofibers |
Packaging film |
Food packaging |
Improved mechanical and barrier properties |
19
|
Cellulose/chitosan nanofibers |
Composite film |
Fruit and vegetable packaging |
Better resistance to water and moisture |
20
|
Cellulose nanofibers |
Packaging film |
Fruit and vegetable packaging |
The film shows improved rheological properties |
21
|
Cellulose/montmorillonite nanofibers |
Nanocomposite film |
Fruit packaging |
The films show good barrier properties |
22
|
Cellulose/silver nanoparticle based nanofibers |
Active packaging film |
Fresh cut melons |
Helps to get improved antimicrobial properties and able to extend the shelf life |
23
|
Cellulose/PLA nanofibers |
Composite film |
Mango packaging |
Helps to reduce the respiration rate, thereby enhancing the shelf life |
24
|
1.1 Natural nanofibers (NNF)
Nanofibers are materials which have diameter ranges in nanometers (nm). Natural nanofibers derived from natural resources are termed as natural nanofibers (NNF). They are natural, non-toxic and eco-friendly and find a wide spectrum of applications in every domain of engineering, healthcare, energy, etc.25 Nanofibers have gained significant attention in the field of food packaging due to their unique properties such as high surface area, porosity, and mechanical strength.12 These properties make them ideal candidates for developing food packaging components with improved functionality and performance. One potential application of nanofibers in food packaging is as a barrier material to prevent the permeation of gases, moisture, and aroma. By using nanofibers with controlled porosity, it is possible to design a packaging material that allows the desired gas and moisture transmission rates while maintaining the quality and freshness of the food product. By incorporating nanofibers into the packaging material, it is possible to increase its strength, stiffness, and toughness, making it more resistant to damage during transportation and storage. Furthermore, nanofibers can be used to develop intelligent packaging that can detect and monitor food spoilage by changing colour, releasing antimicrobial agents, or emitting gases. This can help reduce food waste and increase the shelf life of food products. The use of nanofibers in food packaging components has the potential to revolutionize the food packaging industry by providing improved functionality, performance, and sustainability.26,27 Natural materials are environmentally beneficial because they can be broken down by microbes by the end of their life cycle.
1.2 Sources of natural nanofibers and their production routes
Natural polymers such as cellulose, chitosan, gelatin, and silk fibroin can also be electrospun to produce nanofibers. They are renewable, biodegradable, and sustainable, making them attractive materials for various applications, including food packaging.28 Some of the sources to produce nanofibers are shown in Fig. 3 and discussed below.
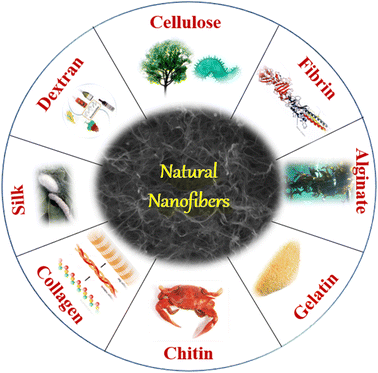 |
| Fig. 3 Sources of natural nanofibers. | |
(a) Cellulose: cellulose is the most abundant natural polymer and is found in plants, such as wood, cotton, and bamboo. It is used to produce paper, textiles, and food packaging. Cellulose can also be electrospun to produce nanofibers with high mechanical strength and biodegradability.29
(b) Chitosan: chitosan is derived from chitin, which is found in the exoskeletons of crustaceans such as crabs and shrimp. Chitosan is biodegradable, biocompatible, and antimicrobial, making it an attractive material for food packaging.30
(c) Gelatin: gelatin is derived from collagen, which is found in animal skin, bones, and connective tissue. It is used as a gelling agent in food products such as gummy candies and as a coating material in food packaging.31
(d) Silk fibroin: silk fibroin is derived from silkworms and is used to produce silk textiles and medical implants. Silk fibroin is biocompatible, biodegradable, and has excellent mechanical properties, making it an attractive material for food packaging.32
(e) Starch: starch is derived from corn, potatoes, and other plants and is used as a thickener and gelling agent in food products. Starch-based polymers can also be used to produce biodegradable food packaging.33
There are several production routes for nanofibers which are shown in Fig. 4. The routes are discussed below as follows.
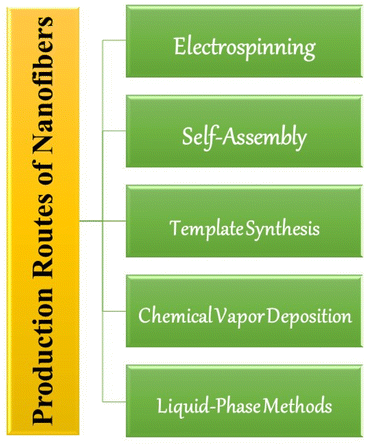 |
| Fig. 4 Production routes of nanofibers. | |
(a) Electrospinning: this is a widely used technique for producing polymer nanofibers. In this method, a high voltage is applied to a polymer solution or melt, which is then ejected from a needle tip. The electric field causes the polymer solution to form a thin jet, which is rapidly elongated and solidified as it travels towards a collector plate. This results in the formation of nanofibers with diameters ranging from a few nanometers to several micrometres. It is primarily used for the fabrication of nanofibrous structures, which have a high surface area-to-volume ratio and unique properties that make them suitable for various applications.34
(b) Self-assembly: self-assembly involves the spontaneous formation of nanofibers through non-covalent interactions, such as hydrogen bonding, electrostatic interactions, and van der Waals forces. This techniques are highly versatile and can be applied to various materials, including polymers, metals, semiconductors, and biological molecules. This method is commonly used to produce peptide and protein nanofibers, which can have applications in food packaging.35
(c) Template synthesis: this method involves the use of a template, such as a porous membrane or a colloidal crystal, to guide the formation of nanofibers. The template is coated with a precursor material, which is then solidified or chemically transformed to form the nanofibers. This method can produce nanofibers with a high degree of control over their diameter and morphology.36
(d) Chemical vapor deposition: chemical vapor deposition (CVD) involves the deposition of thin films of nanofibers through the controlled reaction of gaseous precursor molecules. The gaseous precursors are introduced into a reaction chamber, where they react and form solid nanofibers on a substrate. This method can be used to produce nanofibers with controlled composition, crystallinity, and orientation.37
(e) Liquid-phase methods: liquid-phase methods involve the dispersion of nanofibers in a liquid medium, such as water or a solvent. These methods include techniques such as sonication, microfluidization, and high-pressure homogenization. Liquid-phase methods can be used to produce nanofibers from a variety of materials, including cellulose, chitin, and graphene.38
Each of these production routes has its own advantages and limitations, and the choice of method depends on the specific application and material properties required. The feasibility of a particular production route depends on the desired characteristics of the nanofibers, intended applications, and economic considerations. Electrospinning is the most commonly used technique for producing cellulose nanofibers for fresh produce packaging. Electrospinning has proven to be a valuable method for synthesizing cellulose nanofibers, providing fresh food packaging with enhanced mechanical properties, barrier properties, and sustainability. Its commercial viability, coupled with its ability to incorporate functional additives, makes it an important technique for the development of advanced packaging solutions in the food industry. Researchers and industry professionals are actively working on optimizing existing methods and developing novel approaches to improve the feasibility and scalability of nanofiber production at an industrial scale.
1.3 Use of cellulose nanofibers in different forms
Cellulose nanofibers can be incorporated into various types of food packaging components, such as biofilm fillers, wrapping, or antimicrobial agent carriers, to improve their performance and functionality.39 Biofilm fillers are used to create a barrier layer in food packaging to minimize the transport of moisture, oxygen, and other gases. Nanofibers can be added to biofilm fillers to enhance their mechanical and barrier properties, improving the shelf life of food products. Nanofibers can also be used as wrapping material to create a contact barrier that prevents the growth of bacteria and mold. By incorporating antimicrobial agents into the nanofibers, it is possible to create a wrapping material that not only prevents the growth of microorganisms but also actively kills them, reducing the risk of foodborne illnesses. Furthermore, nanofibers can be used as carriers for antimicrobial agents. By loading antimicrobial agents onto the surface of the nanofibers, it is possible to create a packaging material that slowly releases the agents over time, prolonging the shelf life of food products.40 Overall, the use of nanofibers in food packaging components such as biofilm fillers, wrapping, or antimicrobial agent carriers has the potential to improve the safety and shelf life of food products while reducing the environmental impact of packaging waste.
Although a review of nanofibers used in food packaging was found, to the authors' best knowledge there is no direct review which focuses on a concise overview of the importance of natural nanofibers (NNF) especially cellulose based nanofibers as a sustainable packaging material and active packaging material for fresh produce. The present review article extensively discusses the wide range of natural nanofibers along with their sources, different forms in which nanofibers can be utilised and various modification processes that are carried out to extend the shelf life. The article also presents a comprehensive analysis of the life cycle assessment and sustainability of NNF along with an outlook on its future prospects.
2. Modification in cellulose natural nanofibers
Fresh produce packaging often incorporates cellulose natural materials in the form of nanofibers, but at times these materials may not offer sufficient mechanical strength, barrier properties, and other properties that are necessary for good food packaging performance. Hence, modification is needed to increase the performance of the packaging systems. This section has mostly concentrated on various modification approaches to uplift the mechanical as well as barrier properties, followed by modification utilising various active agents which includes O2 scavengers, CO2 emitters, ethylene scavengers, and essential oils (antioxidant and antimicrobial).
2.1 Mechanical and barrier properties
The desired mechanical performance becomes important in the design of packaging films, as they play an important role in maintaining the structural integrity of the packaging and preventing it from leaking during transport and storage. The technological and practical use of chitosan-based bioplastic faced significant challenges due to the high brittleness and poor mechanical efficiency of the nanofibers.41 To overcome this problem, nanofibers can be combined with other materials such as polymers, essential oils, nanoparticles and plant extracts to improve their mechanical and functional properties.
It is becoming more important to modify natural nanofibers during or after manufacture. The most straightforward procedure for membrane alteration, however, is pre-electrospinning or in situ modification. In this case, the spinning solution is immediately supplemented with additives.42 After the electrospinning procedure, nanofibers are frequently modified to encourage surface modifications. By altering or eliminating the surface functions already present, adding new chemical groups to nanofiber surfaces results in the creation of the required properties. By distributing the active ingredients into polymeric fibres, changes are made for various applications using a variety of materials and methods.43,44 It has been reported in the literature that a polylactic acid nanocomposite reinforced with modified cellulose nanofibers was prepared by modifying the surface of CNF by esterification to improve the dispersion of CNF and its interfacial adhesion with polylactic acid. This composite is more permeable to water vapor than without cellulose nanofiber modification.45 In another study, reinforced carboxylated cellulose nanofibers were developed for collagen membranes by intermolecular/intramolecular electrostatic interaction between cationic acid–swollen collagen fibers and anionic carboxylated cellulose nanofibers. The results showed that carboxylated cellulose nanofibers incorporated into collagen fiber membranes showed good mechanical and barrier properties at a collagen fiber concentration of ≤50 g kg−1.46 The study reports that polypropylene (PP)-based cellulose nanofibers (CNF) with nanoclay nanocomposites improve the mechanical and sealing properties and proposed use in the field of food packaging.47
Modification of cellulose nanofibrous films was carried out with fatty acids such as palmitic, lauric and stearic acids. The modifications were done in two ways: one is the bulk modification, where whole fibers were exposed to the reaction medium, and the other one is surface modification, where only CNF surfaces were exposed. The modified films showed that both modification methods increased the surface hydrophobicity, but the films formed by bulk modification of CNF significantly improved their water vapor barrier property (p < 0.05).48 Electrospun coatings made from PCL fibers containing carotene significantly improved the water vapor and oxygen barrier properties, while a chitosan–TiO2 nanocomposite membrane exhibited higher tensile strength as well as good barrier properties. The presence of palladium nanoparticles improves the protective capacity of electrospun films and their use as interlayer systems or coatings in active food packaging.49 For an efficient barrier, it must be ensured that food packaging materials adhere to the substrate of the packaging material giving a barrier against atmospheric conditions and permitting the flow of oxygen gas. A recent paper50 described how different nanofillers can improve the mechanical and barrier properties of chitosan films for food packaging. This study amply demonstrated that the composition of chitosan, the orientation of individual nanofillers and the control of their intermolecular interaction with the required matrix are three important factors in the preparation of nanostructured films with significant mechanical performance.51 The composition and usefulness of packaging materials have improved to previously unheard-of levels as a result of the reinforcement of diverse natural elements at various scales—from macro to nano—into matrix materials.
2.2 O2 scavengers, CO2 emitters, ethylene scavengers and antimicrobial properties using active agents
Many active packaging systems include additives with multiple active functions such as absorbent and scavenging properties (e.g. oxygen, carbon dioxide, ethylene, moisture, aromas, stains, and UV light); releasing and emitting properties (e.g. ethanol, carbon dioxide, antioxidants, preservatives, sulfur dioxide and aromas); elimination properties (to catalyse the removal of nutrients such as lactose and cholesterol); and temperature. The production of these active packaging systems may require immobilization, coating, integration or modification of the surfaces of the packaging materials. Fig. 5 shows a broad classification of active ingredients/agents used in food packaging.
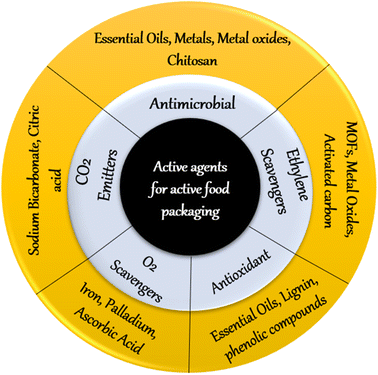 |
| Fig. 5 Broad classification of active agents used in active food packaging (MOF: metal–organic framework). | |
Fig. 6 shows fresh produce in regular packaging. In general, several parameters such as O2, CO2, ethylene gas, moisture and microbes affect shelf life by attacking fruits/vegetables and reducing shelf life as shown in Fig. 6 (path a). In connection with the development of technology and the needs of customers, it becomes important to improve the shelf life of fresh agricultural products with active ingredients. The active ingredients can be used either in the form of sachets or integrated directly into the packaging material. Based on the requirements, the different active agents are highlighted in Fig. 6 with colour codes and can be used according to our needs. Using active agents as shown in Fig. 6 (path b) helps to enhance the shelf life of fresh produce.
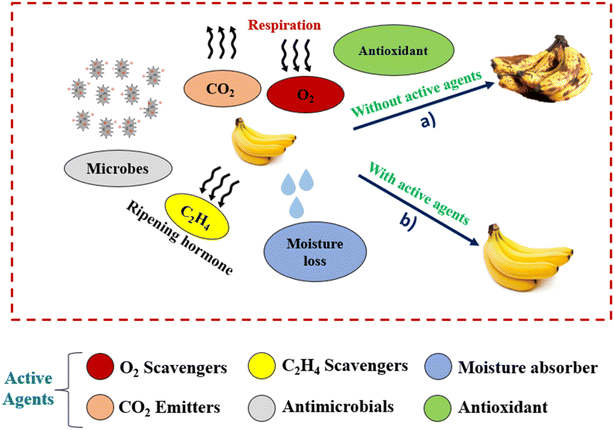 |
| Fig. 6 Fresh produce in a regular packaging. | |
Antimicrobial compounds are one of the most studied active ingredients/agents as the growth of harmful and/or spoilage bacteria is by far the most important cause of food spoilage. These microbes (yeasts) include but are not limited to, the following: Salmonella species, Staphylococcus aureus, Listeria monocytogenes, Bacillus cereus, Escherichia coli, Pseudomonas species, Klebsiella species, Lactobacillus species, Rhizopus species, Aspergillus species, and Torulopsis species.52
Therefore, one of the largest classes of active agents with commercially available products are antimicrobial agents, which are available as emission bags and absorbent pads.53 Metals in various forms have been used for countless years as antibacterial agents and are currently one of the most well-liked antimicrobial compounds. Among all metals with antimicrobial properties, silver and silver compounds such as metallic silver (Ag0), silver ions (the most common Ag+) and silver nanoparticles (Ag NP) have been found to have exceptional antimicrobial activity against a wide range of microorganisms at low concentrations and have very low systemic toxicity in humans.54 Other metals and metal-containing compounds such as copper, gold, zinc oxide and titanium dioxide have also been found to have positive antibacterial effects even in their nanoscale form. Recently, more information about metallic and inorganic nanoparticles and their antibacterial activity in food packaging applications has been widely studied.58 Li et al.55 studied ZnO nanoparticles incorporated in a poly(lactic acid) (PLA) matrix that produced films that significantly inhibited microbial growth. In reality, ZnO nanoparticles reduced the number of bacteria, yeasts and fungi in fresh apples.
Gaseous carbon dioxide (CO2) is soluble in food's aqueous and lipid phases, where it forms carbonic acid and simultaneously causes the food product to become acidic. The food sector makes considerable use of carbon dioxide's advantageous antibacterial qualities to preserve food quality and extend shelf life. Although some of the mechanisms by which CO2 exerts its effects are still not fully understood, it is generally accepted that these mechanisms involve a combination of cytoplasmic pH changes, inhibition of bacterial enzymes, and disruption of the bacterial cell membrane. The combined effect lengthens the lag phase and consequently inhibits the growth of many spoilage microorganisms.52
In the food industry, gas cleaning or modified atmosphere packaging technologies (MAP) or, more effectively, deoxygenation equipment (OS) are used to control residual oxygen. Ethylene is a small volatile molecule that acts as a phytohormone and accelerates the ripening and ageing of fruits and vegetables.56 Controlling the concentration of ethylene around fresh foods after harvest during transport, storage and handling is important to improve their quality and extend their shelf life. The most commonly used ethylene scavenger consists of potassium permanganate (KMnO4) supported on an inert matrix (such as silica gel or alumina) which oxidizes the ethylene, turning it from purple to brown. For example, researchers57 used this colour change to signal the removal of ethylene on a nanostructured substrate made of silica (SiO2) and alumina (Al2O3) nanoparticles impregnated with KMnO4. In reality, this inorganic substance has excellent applications as in the case of ethylene absorption bags when, for example, several varieties of tomatoes are stored in a refrigerator (similar to domestic consumers). However, KMnO4 cannot be used in direct contact with foodstuffs due to its high toxicity and low long-term effectiveness under humid conditions. A summary of studies on modification processes carried out to enhance the performance of nanofiber based food packaging is shown in Table 2.
Table 2 Summary of reported studies on modification processes carried out to enhance the properties of packaging materials
Packaging material |
Modification process |
Impact on properties |
References |
Cellulose nanofibers |
NaOH and NaHCO3 treatment |
Improved mechanical and water vapor permeability |
58
|
Cellulose nanofibers |
Maleic anhydride treatment |
Decrease in moisture content |
59
|
Cellulose nanofibers |
Ethanol and NaOH treatment |
Increased surface roughness, better thermal stability, and higher mechanical strength |
60
|
Self-fibrillating cellulose (SFC) nanofibers |
TEMPO-periodate oxidation treatment |
Better mechanical properties |
61
|
Cellulose nanofibers |
NaOH treatment |
High thermal stability, better mechanical properties |
62
|
Nanocellulose |
Polysaccharides coatings |
Improved water resistance and rheological properties |
63
|
Cellulose nanofibers |
Heat treatment, TEMPO-periodate oxidation treatment |
Improved barrier properties, enhanced mechanical properties |
64
|
Cellulose/PLA nanofibers |
Silane treatment, esterification |
Improved mechanical and barrier properties |
65
|
Cellulose nanofibers |
Alkali and oxalic acid treatment |
Enhanced barrier properties with significant improvement in mechanical strength |
66
|
Chitosan nanofibers |
Antimicrobial essential oil |
Increased shelf life, inhibition of the growth of fungal infection |
67
|
Cellulose nanofibers |
Chemical modification (esterification, alkali treatment, carbanilation) |
Improved mechanical and barrier properties |
68
|
Chitosan nanofibers |
Cinnamon essential oil |
Increased shelf life and reduced weight loss and bacterial growth |
69
|
Cellulose nanofibers |
Essential oil (Cinnamomum zelanicum) |
Barrier properties are increased and the shelf life is prolonged |
70
|
Cellulose nanofibers |
Savory essential oil |
Enhancement in water vapour permeability inhibits the growth of Gram-positive bacteria |
71
|
Chitosan nanofibers |
Alginate emulsion with olive oil |
Decreasing water loss, respiration rate, and firmness, when compared to uncoated ones, and prolonging the shelf life |
72
|
Cellulose nanofibers |
Edible coatings |
Enhanced shelf life |
21
|
Chitosan nanofibers |
Physical and chemical modifications |
Extended shelf life and storage characteristics |
73
|
Zein nanofibers |
Star anise essential oil and beta-cyclodextrin |
Better mechanical properties and antioxidant activity |
74
|
Cellulose nanofibers |
PVA/starch coated treatment |
Improved mechanical strength and imparts antimicrobial properties |
75
|
Cellulose nanofibers |
Ultrasound treatment |
Good effect against bacteria and oxidation |
48
|
Cellulose-acetate nanofibers |
Gelatin–eugenol |
Increased surface hydrophobicity and mechanical strength |
76
|
Cellulose nanofibers |
TEMPO oxidation treatment |
Improved vapour barrier properties and excellent mechanical strength |
77
|
Cellulose nanofibers |
Carboxymethyl cellulose (CMC) |
Improved mechanical properties, barrier properties and antimicrobial properties |
78
|
3. Life cycle assessment and sustainability of packaging materials
Throughout a product's life cycle, an LCA finds, quantifies, and evaluates sources of environmental impact. It looks at all the processes involved in creating, utilising, and discarding a product. LCA helps to assess the effects of various packaging options on the environment and decide which solution makes the most sense. Fig. 7 illustrates the steps of life cycle assessment. An LCA aids in prioritising how to enhance processes or goods.
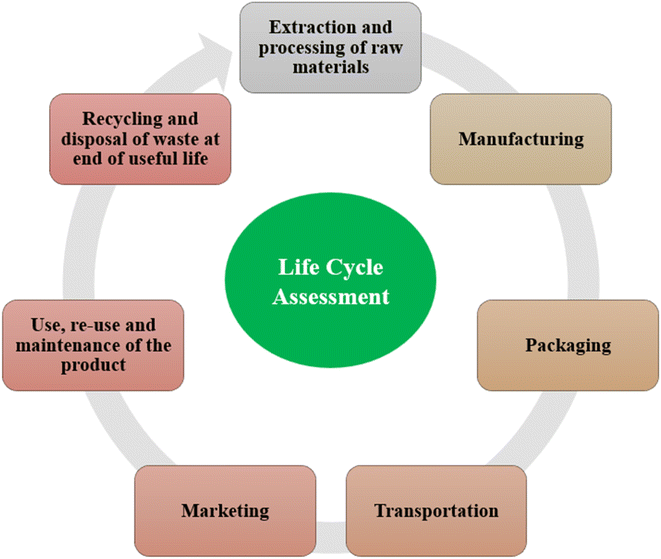 |
| Fig. 7 Life cycle assessment stages. | |
By quantifying the energy and materials utilised, LCA aids in assessing the environmental costs associated with a product. ISO 14040 and 14044 generally provide a general framework for carrying out a life cycle assessment (Fig. 8). The significance of using LCA in food packaging techniques has increased in recent times.79 Packaging-related food waste and losses encompass not only material wastage but also the loss of actual food. When food remains on discarded packaging, it reduces the packaging's recycling capacity and has a detrimental impact on the environment. A circular economy can help to prevent these problems. A circular economy is nothing more than a service flow that uses a cyclical material flow to go from linear nature to society to natural resources.80 The contribution a product makes to a circular economy is described below using the figurative term “circularity”. It makes references to cyclical material fluxes and renewable energy. It is entirely produced using renewable energy, and it is also distributed and recycled. Different meanings can be attributed to the word “recyclable”. The definition of “recyclable” in ISO 14021:2012 states that it refers to a product's ability to be removed from the waste stream and put back into circulation.
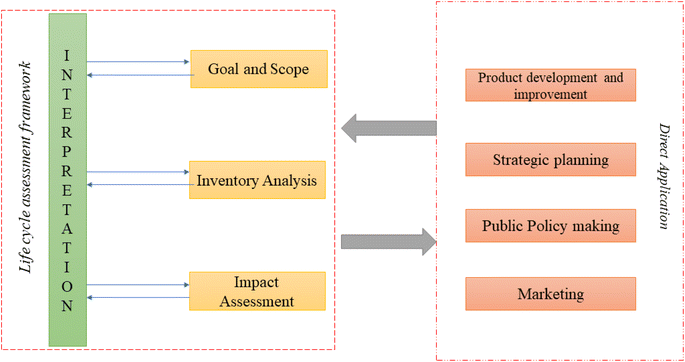 |
| Fig. 8 Life cycle assessment procedure according to ISO 14040.82 | |
Table 3 provides a detailed discussion of several life cycle analyses of natural materials. The product's contribution to the circular economy is described below using the figurative term “circulation”. It refers to cyclic material flows and renewable energy. The concept of biological and technological cycles is based on Korhonen et al.80 Since circular packaging is made from recycled or renewable materials, it should ideally be recycled or composted after use. It is produced entirely with renewable energy, as well as distributed and recycled. The word “recyclable” can be given different meanings. According to ISO 14021:2012, “recyclable” refers to the ability of the product to be removed from the waste stream and recycled. Note that “recyclable” in this definition does not mean that the recycled material is used for the same purpose. The cyclos-HTP Institute, on the other hand, defines recyclable packaging as that which can be recycled in a way that replaces primary material with recycled material.81 Several life cycle analyses of natural materials are detailed in Table 3.
Table 3 LCA of various natural materials used in food packaging
Natural materials |
Outcome |
References |
Cellulose (viscose, modal and tencel) |
Cotton and petrochemical synthetic fibres have a clear potential to have less of an adverse effect on the environment than modern man-made cellulose fibres |
83
|
Chitosan |
PP (polypropylene) exhibits a higher amount of carcinogens and fossil fuels than chitosan films |
84
|
Cellulose |
The water-based enzymatic process had environmental advantages over other nanocellulose extraction technologies |
85
|
Cotton |
The optimum solution is opted to reduce the environmental impact by shifting towards organic cultivation of cotton |
86
|
Cellulose nano-sponges |
Low level of photochemical traces, and less impact on the environment |
87
|
Cellulose |
Recycled sources are better approaches for providing sustainability |
88
|
Wood nanofibers |
Compression-moulded wood fibres and lignin-containing nanofibers should be examined for characteristics that measure environmental stress. Comparing high-lignin fibre materials to low-lignin fibre materials allows for improved moisture durability and perhaps service life |
89
|
Nanocellulose |
Consumes less energy and produces less carcinogens as compared to the plastic based packaging |
90
|
Cellulose |
Human societies have paid close attention to economic and climate change challenges, and it is now crucial to reduce energy-intensive processes and create low-carbon materials. In this regard, certain methods for achieving these objectives in various phases of the production of cellulose products have been proposed in the study's concluding part |
91
|
Cellulose nanocomposites |
In comparison to their petroleum-based equivalents, biobased/biodegradable polymers and recycled plastics are generally preferred since they support sustainability and a greener society |
92
|
Table 3 shows that the LCA of various natural materials is relatively under-investigated and often disregarded. However, investigating the LCA is crucial and is likely to emerge as a significant area of study in the near future. Sustainability is also an important aspect which needs to be considered during food packaging. A material is said to be sustainable if it meets the three pillars i.e., environmental, social and economic aspects. Sustainable food packaging offers the best product protection, has minimal environmental effects during both manufacturing and disposal and is as circular as feasible. In practice, there are frequently compromises made between these goals. Although using less packaging lessens the direct environmental effects caused by packaging, this can result in increased food waste.93,94 Despite being recycled more often than PET bottles, single-use glass bottles have a greater negative impact on the environment.95,96 Despite being lightweight, effective, and providing adequate product protection, multilayer plastic packaging is typically not recyclable.97Fig. 9 represents the three sustainability factors for food packaging.
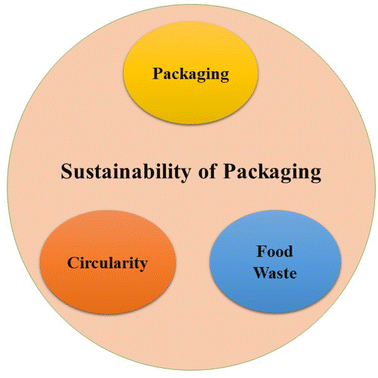 |
| Fig. 9 Sustainability of packaging. | |
“Sustainability” refers to the capacity of a system to endure and thrive over the long term while minimizing its negative impacts on the environment, society, and economy. Discussing sustainability in the context of raw materials and plastic production involves considering the availability of sources of raw materials and the applicable process technology. Plastic derived from petroleum, also known as petrochemical-based plastic, relies on fossil fuel resources such as crude oil and natural gas. These resources are finite and non-renewable, meaning they cannot be replenished within a human lifetime. As the demand for plastics continues to rise, concerns arise about the long-term availability of petroleum-based raw materials. In contrast, sustainable alternatives to petrochemical-based plastics aim to utilize renewable resources as raw materials. These may include plant-based sources like biomass, agricultural waste, or specific crops cultivated for plastic production, known as bio-based plastics. By utilizing renewable sources, the availability of raw materials can be sustained over time. To enhance sustainability, innovative process technologies can be employed. These technologies aim to reduce energy consumption, minimize greenhouse gas emissions, and decrease the overall environmental impact of plastic production. Examples include advanced recycling techniques, biodegradable plastics, and the use of renewable energy sources in the manufacturing process. In summary, sustainability in the context of raw materials and plastic production involves considering the availability of sources of raw materials and the use of applicable process technologies. By transitioning away from petroleum-based raw materials and adopting sustainable alternatives, such as bio-based plastics, and implementing environmentally friendly production methods, we can reduce reliance on finite resources, decrease environmental impacts, and work towards a more sustainable future.
Researchers are favouring the development of edible coatings, which are primarily found in the food category of fresh or preserved fruits and vegetables, to attain sustainability.98 These coatings reduce packaging and food losses, both of which have a negative influence on the environment. Fresh food loses a large portion of its quality during storage and transit. It frequently contains components that ought to be avoided or minimised, such as moisture loss, shrinkage, weight loss, metabolic and microbiological alterations, mechanical damage, and sensory changes. Adding edible packaging to fresh fruit or vegetables may affect the gas permeability, notably the oxygen diffusion, and so inhibit the ripening processes because these food classes continue to have respiratory activities long after harvest.99 Edible packaging can be made from a variety of materials, including proteins, carbohydrates, lipids, and their combinations. The chemical composition of edible coatings can vary depending on the specific material used, but here are some examples of common edible coating materials and their chemical components. The chemical composition of edible coatings can vary depending on the specific material used, but they typically consist of proteins, carbohydrates, lipids, or their combinations.100
4. Future and global market trends of fruit and vegetable packaging
The market for fruit and vegetable packaging is expected to reach US$ 545 billion by 2030, expanding at a projected CAGR of 5.98% from 2022 to 2030, from its estimated size of US$ 323.2 billion in 2021. The need for the field of fruit and vegetable processing is being driven by shifting consumer lifestyles and dual-income households. Fruit and vegetable processing improves these foods' quality and shelf life while also extending both. Processing fruits and vegetables is particularly beneficial in keeping food products' vitamins, nutrients, and flavour. The processed foods are kept in cans as pulped fruit bits or liquids. There are various processing techniques, such as freezing, drying, and creating jams, jellies, or juices. Humans consume a wide variety of plants. Even though vegetables are extremely perishable, processing techniques must be used to preserve them and extend their shelf life. The worldwide trade of vegetables, which is limited to processed forms, has benefited from the availability of processing techniques in many different countries. Processing doesn't affect the nutritional value of fruits and vegetables in any way. Most consumers hold the opinion that fresh vegetables are preferable to those that have been processed. Due to interference from numerous variables during storage and transportation, fresh vegetables may lose some of their vitamin content, and their quality may also be changed. The future market trend of fruits and vegetables is shown in Fig. 10. There are no preservatives in minimally processed fruits and vegetables. Various factors like growth factors of fruits and vegetables, their types, product insights, and geographic insight affect the overall market trend of fruits and vegetables. The future of new types of packaging materials for sustainability looks very promising. As the world becomes more aware of the impact of packaging on the environment, there is increasing demand for packaging that is more sustainable and eco-friendly. The use of natural fibers in food packaging looks promising. There is an increasing demand for sustainable and eco-friendly packaging materials, and natural fibers such as bamboo, hemp, jute, cotton, and kenaf are being considered as alternatives to traditional plastic-based packaging. These natural fibers have several advantages over plastic-based packaging as they are biodegradable, renewable, and compostable, which makes them more environmentally friendly. They also have good strength and durability, which makes them suitable for a wide range of food packaging applications. In addition, natural fibers have the potential to enhance food safety and quality.
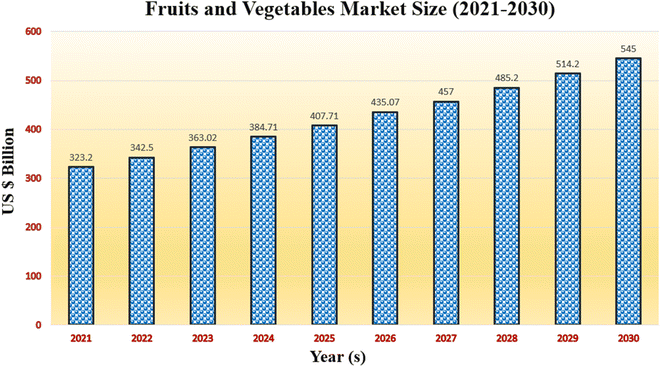 |
| Fig. 10 Market trend of fruits and vegetables (source: https://www.grandviewresearch.com/; keywords: food packaging, fruits and vegetables). | |
Cellulose-based nanofibers as a food packaging material has the potential to be a feasible and sustainable alternative to plastic based materials. Cellulose-based nanofibers are derived from renewable resources, such as plant fibers and microbial sources, which involves fermentation. All the processes involved in production of nanofibers are already scaled up industrially. In fact, one form of cellulose nanofiber (bacterial cellulose) is available as a food material having the name nata de coco. However, further research, development, and optimization are needed to overcome challenges and ensure its feasibility in terms of cost, performance, and waste management. End-of-life considerations, including composting or recycling, should be taken into account during the design and implementation of cellulose-based nanofiber packaging.
5. Conclusions
This article provides a detailed summary of research on natural nanofibers (NNF) in food packaging and their role in extending the shelf life of fresh produce. A wide variety of biodegradable polymers are used in the production of various food packaging materials. Although there is extensive literature available on natural nanofibers to extend the shelf life of fresh agricultural products, there are still some important aspects that are worth highlighting. Most of the previously reported studies emphasize the use of natural nanofibers in food packaging for shelf-life extension using different techniques. The economic aspects and scalability of these technologies must be thoroughly investigated. Although various natural nanofibers are available, various sources of fully biodegradable and readily available nanofibers remain to be explored. Various modifications are being made to alter the properties of natural nanofibers. However, further explanation of the underlying mechanism of how nanofibers are changed during any modification is required. Another important aspect is that although different active agents are used in food packaging systems, the effect of these active agents on nutritional quality is an area that requires a lot of attention. The use of natural nanofibers as active agents in food packaging is gaining more attention because it helps to create natural active ingredients. Most often, natural nanofibers are used in the form of films or coatings to extend the shelf life of fresh agricultural products, but one important aspect to investigate is the packaging of fresh-cut fruits and vegetables using nanofibers. Only a few studies have been reported on the life cycle assessment of different natural nanofibers. The life cycle assessment of natural nanofibers and their impact on the environment will be an important area of research in the near future.
Author contributions
Partha Pratim Das: writing – original manuscript, reviewing and editing; Peddapapannagari Kalyani: writing – original manuscript, reviewing and editing; Rahul Kumar: supervision; Mudrika Khandelwal: ideas, conceptualization, supervision.
Conflicts of interest
The authors declare that they have no known competing financial interests or personal relationships that could have appeared to influence the work reported in this paper.
Acknowledgements
The authors would like to acknowledge IIT Hyderabad for providing us with free access to necessary journals. The authors would also like to thank the University of Hyderabad, India for their support throughout the work and Waycool Private Limited for their financial support.
References
- M. C. Giannakourou and T. N. Tsironi, Application of processing and packaging hurdles for fresh-cut fruits and vegetables preservation, Foods, 2021, 10(4), 830 CrossRef CAS PubMed.
- J. Deng,
et al., Overview of renewable polysaccharide-based composites for biodegradable food packaging applications, Green Chem., 2022, 24(2), 480–492 RSC.
- T. Ghosh, G. B. Raj and K. K. Dash, A comprehensive review on nanotechnology based sensors for monitoring quality and shelf life of food products, Meas.: Food, 2022, 100049 Search PubMed.
- S. Shahid,
et al., Role of active food packaging developed from microencapsulated bioactive ingredients in quality and shelf life enhancement: A review, Am. J. Sci., 2021, 17(2), 12–28 Search PubMed.
- M. R. Yan, S. Hsieh and N. Ricacho, Innovative food packaging, food quality and safety, and consumer perspectives, Processes, 2022, 10(4), 747 CrossRef CAS.
-
P. Dubey, O. Yousuf, and A. Singh, Advancement in Packaging Technologies for Agri-Food Sector, in Agri-Food 4.0, Emerald Publishing Limited, 2022 Search PubMed.
- M. Zhang,
et al., Recent advances in polymers and polymer composites for food packaging, Mater. Today, 2022, 53, 134–161 CrossRef CAS.
- I. Ahmed,
et al., A comprehensive review on the application of active packaging technologies to muscle foods, Food Control, 2017, 82, 163–178 CrossRef CAS.
- O. J. Caleb, K. Ilte, A. Fröhling, M. Geyer and P. V. Mahajan, Integrated modified atmosphere and humidity package design for minimally processed Broccoli (Brassica oleracea L. var. italica), Postharvest Biol. Technol., 2016, 121, 87–100 CrossRef CAS.
- M. Aman Mohammadi,
et al., New perspectives on electrospun nanofiber applications in smart and active food packaging materials, Crit. Rev. Food Sci. Nutr., 2022, 1–17 CrossRef CAS PubMed.
- K. Badgar, N. Abdalla, H. El-Ramady and J. Prokisch, Sustainable applications of nanofibers in agriculture and water treatment: a review, Sustainability, 2022, 14(1), 464 CrossRef CAS.
-
A. Meftahi, M. E. Momeni Heravi, A. Barhoum, P. Samyn, H. Najarzadeh, and S. Alibakhshi, Cellulose Nanofibers: Synthesis, Unique Properties, and Emerging Applications, Handbook of Nanocelluloses: Classification, Properties, Fabrication, and Emerging Applications, 2022, pp. 233–262 Search PubMed.
- H. Tibolla, F. M. Pelissari, J. T. Martins, A. A. Vicente and F. C. Menegalli, Cellulose nanofibers produced from banana peel by chemical and mechanical treatments: characterization and cytotoxicity assessment, Food Hydrocolloids, 2018, 75, 192–201 CrossRef CAS.
- H. Abral,
et al., Transparent and antimicrobial cellulose film from ginger nanofiber, Food Hydrocolloids, 2020, 98, 105266 CrossRef CAS.
- R. K. Gond and M. K. Gupta, A novel approach for isolation of nanofibers from sugarcane bagasse and its characterization for packaging applications, Polym. Compos., 2020, 41(12), 5216–5226 CrossRef CAS.
- E. Souza, L. Gottschalk and O. Freitas-Silva, Overview of nanocellulose in food packaging, Recent Pat. Food, Nutr. Agric., 2020, 11(2), 154–167 CrossRef PubMed.
- K. J. Nagarajan,
et al., A comprehensive review on cellulose nanocrystals and cellulose nanofibers: pretreatment, preparation, and characterization, Polym. Compos., 2021, 42(4), 1588–1630 CrossRef CAS.
- S. LakshmiBalasubramaniam, A. S. Patel, B. Nayak, C. Howell and D. Skonberg, Antioxidant and antimicrobial modified cellulose nanofibers for food applications, Food Biosci., 2021, 44, 101421 CrossRef CAS.
- N. D. Tien, S. P. Lyngstadaas, J. F. Mano, J. J. Blaker and H. J. Haugen, Recent developments in chitosan-based micro/nanofibers for sustainable food packaging, smart textiles, cosmeceuticals, and biomedical applications, Molecules, 2021, 26(9), 2683 CrossRef CAS PubMed.
- S. M. Mohammadi Sadati, N. Shahgholian-Ghahfarrokhi, E. Shahrousvand, J. Mohammadi-Rovshandeh and M. Shahrousvand, Edible chitosan/cellulose nanofiber nanocomposite films for potential use as food packaging, Mater. Technol., 2022, 37(10), 1276–1288 CrossRef CAS.
- Z. Jiang and T. Ngai, Recent Advances in Chemically Modified Cellulose and Its Derivatives for Food Packaging Applications: A Review, Polymers, 2022, 14(8), 1533, DOI:10.3390/polym14081533.
- N. Gürler and G. Torğut, Physicomechanical, thermal and dielectric properties of eco-friendly starch-microcrystalline cellulose-clay nanocomposite films for food packaging and electrical applications, Packag. Technol. Sci., 2022, 35(6), 473–483 CrossRef.
- Ł. Łopusiewicz, S. Macieja, M. Śliwiński, A. Bartkowiak, S. Roy and P. Sobolewski, Alginate biofunctional films modified with melanin from watermelon seeds and zinc oxide/silver nanoparticles, Materials, 2022, 15(7), 2381 CrossRef PubMed.
- M. E. Lozano Fernandez and N. Miskolczi, Production of Cellulose Nano-Fibers and Its Application in Poly-Lactic-Acid: Property Improvement by New Types of Coupling Agents, Polymers, 2022, 14(9), 1887 CrossRef CAS PubMed.
- J. Li, A. He, C. C. Han, D. Fang, B. S. Hsiao and B. Chu, Electrospinning of hyaluronic acid (HA) and HA/gelatin blends, Macromol. Rapid Commun., 2006, 27(2), 114–120 CrossRef CAS.
- W.-H. Han,
et al., Recent Advances in the Food Application of Electrospun Nanofibers, J. Ind. Eng. Chem., 2022, 110, 15–26 CrossRef CAS.
-
A. B. Perumal, R. B. Nambiar, J. A. Moses, and C. Anandharamakrishnan, Nanocellulose: Recent Trends and Applications in the Food Industry, Food Hydrocoll., 2022, p. 107484 Search PubMed.
- A. C. Silva, A. J. Silvestre, C. Vilela and C. S. Freire, Natural polymers-based materials: a contribution to a greener future, Molecules, 2022, 27(1), 94 CrossRef CAS PubMed.
- K. Huang and Y. Wang, Recent applications of regenerated cellulose films and hydrogels in food packaging, Curr. Opin. Food Sci., 2022, 43, 7–17 CrossRef CAS.
- M. Flórez, E. Guerra-Rodríguez, P. Cazón and M. Vázquez, Chitosan for food packaging: recent advances in active and intelligent films, Food Hydrocolloids, 2022, 124, 107328 CrossRef.
- Q. Luo,
et al., Gelatin-based composite films and their application in food packaging: a review, J. Food Eng., 2022, 313, 110762 CrossRef CAS.
- J. T. Low, N. I. S. M. Yusoff, N. Othman, T.-W. Wong and M. U. Wahit, Silk fibroin-based films in food packaging applications: a review, Compr. Rev. Food Sci. Food Saf., 2022, 21(3), 2253–2273 CrossRef CAS PubMed.
- L. García-Guzmán, G. Cabrera-Barjas, C. G. Soria-Hernández, J. Castaño, A. Y. Guadarrama-Lezama and S. Rodríguez Llamazares, Progress in starch-based materials for food packaging applications, Polysaccharides, 2022, 3(1), 136–177 CrossRef.
- N. Angel, S. Li, F. Yan and L. Kong, Recent advances in electrospinning of nanofibers from bio-based carbohydrate polymers and their applications, Trends Food Sci. Technol., 2022, 120, 308–324 CrossRef CAS.
- C. Xie,
et al., Recent Advances in Self-Assembly and Application of Para-Aramids, Molecules, 2022, 27(14), 4413 CrossRef CAS PubMed.
- A. Ganesan and R. Jaiganesh, A review on fabrication methods of nanofibers and a special focus on application of cellulose nanofibers, Carbohydr. Polym. Technol. Appl., 2022, 100262 Search PubMed.
- R. Shoukat and M. I. Khan, Carbon nanotubes/nanofibers (CNTs/CNFs): a review on state of the art synthesis methods, Microsyst. Technol., 2022, 28(4), 885–901 CrossRef CAS.
- A. Nadaf, A. Gupta, N. Hasan, S. Ahmad, P. Kesharwani and F. J. Ahmad, Recent update on electrospinning and electrospun nanofibers: current trends and their applications, RSC Adv., 2022, 12(37), 23808–23828 RSC.
- F. Fitriani, M. R. Bilad, S. Aprilia and N. Arahman, Biodegradable Hybrid Polymer Film for Packaging: A Review, J. Nat. Fibers, 2023, 20(1), 2159606 CrossRef.
- R. Priyadarshi, S. Roy, T. Ghosh, D. Biswas and J.-W. Rhim, Antimicrobial nanofillers reinforced biopolymer
composite films for active food packaging applications-a review, Sustainable Mater.Technol., 2022, 32, e00353 CrossRef CAS.
- H. Wang, Y. Liao, A. Wu, B. Li, J. Qian and F. Ding, Effect of sodium trimetaphosphate on chitosan-methylcellulose composite films: physicochemical properties and food packaging application, Polymers, 2019, 11(2), 368 CrossRef CAS PubMed.
- G. B. Medeiros, F. de A. Lima, D. S. de Almeida, V. G. Guerra and M. L. Aguiar, Modification and Functionalization of Fibers Formed by Electrospinning: A Review, Membranes, 2022, 12(9), 861 CrossRef CAS PubMed.
- P. Cazón and M. Vázquez, Mechanical and barrier properties of chitosan combined with other components as food packaging film, Environ. Chem. Lett., 2020, 18(2), 257–267 CrossRef.
-
A. R. Alias, M. K. Wan, and N. M. Sarbon, Emerging Materials and Technologies of Multi-Layer Film for Food Packaging Application: A Review, Food Control, 2022, p. 108875 Search PubMed.
- C. Du, H. Li, B. Li, M. Liu and H. Zhan, Characteristics and properties of cellulose nanofibers prepared by TEMPO oxidation of corn husk, BioResources, 2016, 11(2), 5276–5284 CAS.
- W. Wang, X. Zhang, C. Li, G. Du, H. Zhang and Y. Ni, Using carboxylated cellulose nanofibers to enhance mechanical and barrier properties of collagen fiber film by electrostatic interaction, J. Sci. Food Agric., 2018, 98(8), 3089–3097 CAS.
- B. N. Jung, H. W. Jung, D. Kang, G. H. Kim and J. K. Shim, Synergistic effect of cellulose nanofiber and nanoclay as distributed phase in a polypropylene based nanocomposite system, Polymers, 2020, 12(10), 2399 CrossRef CAS PubMed.
- L. Lin,
et al., Enhancing the properties of Litsea cubeba essential oil/peach gum/polyethylene oxide nanofibers packaging by ultrasonication, Food Packag. Shelf Life, 2022, 34, 100951, DOI:10.1016/j.fpsl.2022.100951.
- J. Xue, T. Wu, Y. Dai and Y. Xia, Electrospinning and electrospun nanofibers: methods, materials, and applications, Chem. Rev., 2019, 119(8), 5298–5415 CrossRef CAS PubMed.
- J. Pires, C. D. de Paula, V. G. L. Souza, A. L. Fernando and I. Coelhoso, Understanding the barrier and mechanical behavior of different nanofillers in chitosan films for food packaging, Polymers, 2021, 13(5), 721 CrossRef CAS PubMed.
- A. Riaz,
et al., Preparation and characterization of chitosan-based antimicrobial active food packaging film incorporated with apple peel polyphenols, Int. J. Biol. Macromol., 2018, 114, 547–555 CrossRef CAS PubMed.
- M. W. Ahmed,
et al., A review on active packaging for quality and safety of foods: current trends, applications, prospects and challenges, Food Packag. Shelf Life, 2022, 33, 100913 CrossRef CAS.
- C. G. Otoni, P. J. Espitia, R. J. Avena-Bustillos and T. H. McHugh, Trends in antimicrobial food packaging systems: emitting sachets and absorbent pads, Food Res. Int., 2016, 83, 60–73 CrossRef CAS.
- S. S. Ahmad, O. Yousuf, R. U. Islam and K. Younis, Silver nanoparticles as an active packaging ingredient and its toxicity, Packag. Technol. Sci., 2021, 34(11–12), 653–663 CrossRef CAS.
- W. Li, L. Li, Y. Cao, T. Lan, H. Chen and Y. Qin, Effects of PLA Film Incorporated with ZnO Nanoparticle on the Quality Attributes of Fresh-Cut Apple, Nanomaterials, 2017, 7(8), 207, DOI:10.3390/nano7080207.
- H. Wei, F. Seidi, T. Zhang, Y. Jin and H. Xiao, Ethylene scavengers for the preservation of fruits and vegetables: a review, Food Chem., 2021, 337, 127750 CrossRef CAS PubMed.
- P. C. Spricigo, M. M. Foschini, C. Ribeiro, D. S. Corrêa and M. D. Ferreira, Nanoscaled Platforms Based on SiO2 and Al2O3 Impregnated with Potassium Permanganate Use Color Changes to Indicate Ethylene Removal, Food Bioprocess Technol., 2017, 10(9), 1622–1630, DOI:10.1007/s11947-017-1929-9.
- M. Prakash Menon, R. Selvakumar, P. Suresh kumar and S. Ramakrishna, Extraction and modification of cellulose nanofibers derived from biomass for environmental application, RSC Adv., 2017, 7(68), 42750–42773, 10.1039/C7RA06713E.
- S. Cichosz and A. Masek, Cellulose Fibers Hydrophobization via a Hybrid Chemical Modification, Polymers, 2019, 11(7), 1174, DOI:10.3390/polym11071174.
- W. Li, S. Wang, W. Wang, C. Qin and M. Wu, Facile preparation of reactive hydrophobic cellulose nanofibril film for reducing water vapor permeability (WVP) in packaging applications, Cellulose, 2019, 26(5), 3271–3284, DOI:10.1007/s10570-019-02270-x.
- Y. C. Gorur, P. A. Larsson and L. Wågberg, Self-Fibrillating Cellulose Fibers: Rapid In Situ Nanofibrillation to Prepare Strong, Transparent, and Gas Barrier Nanopapers, Biomacromolecules, 2020, 21(4), 1480–1488, DOI:10.1021/acs.biomac.0c00040.
- S. Cichosz and A. Masek, Thermal Behavior of Green Cellulose-Filled Thermoplastic Elastomer Polymer Blends, Molecules, 2020, 25(6), 1279, DOI:10.3390/molecules25061279.
- P. Nechita and M. Roman (Iana-Roman), Review on Polysaccharides Used in Coatings for Food Packaging Papers, Coatings, 2020, 10(6), 566, DOI:10.3390/coatings10060566.
- S. Saedi, C. V. Garcia, J. T. Kim and G. H. Shin, Physical and chemical modifications of cellulose fibers for food packaging applications, Cellulose, 2021, 28(14), 8877–8897, DOI:10.1007/s10570-021-04086-0.
- L. Zhou, K. Ke, M.-B. Yang and W. Yang, Recent progress on chemical modification of cellulose for high mechanical-performance Poly(lactic acid)/Cellulose composite: a review, Compos. Commun., 2021, 23, 100548, DOI:10.1016/j.coco.2020.100548.
- W. Wang,
et al., Multilayer surface construction for enhancing barrier properties of cellulose-based packaging, Carbohydr. Polym., 2021, 255, 117431, DOI:10.1016/j.carbpol.2020.117431.
- A. Negi and K. K. Kesari, Chitosan Nanoparticle Encapsulation of Antibacterial Essential Oils, Micromachines, 2022, 13(8), 1265, DOI:10.3390/mi13081265.
- T. Aziz,
et al., A Review on the Modification of Cellulose and Its Applications, Polymers, 2022, 14(15), 3206, DOI:10.3390/polym14153206.
- R. R. Basaglia, S. Pizato, N. G. Santiago, M. M. Maciel de Almeida, R. A. Pinedo and W. R. Cortez-Vega, Effect of edible chitosan and cinnamon essential oil coatings on the shelf life of minimally processed pineapple (Smooth cayenne), Food Biosci., 2021, 41, 100966, DOI:10.1016/j.fbio.2021.100966.
- A. Erfani, M. Khalil Pirouzifard, H. Almasi, N. Gheybi and S. Pirsa, Application of cellulose plate modified with encapsulated Cinnamomum zelanicum essential oil in active packaging of walnut kernel, Food Chem., 2022, 381, 132246, DOI:10.1016/j.foodchem.2022.132246.
- M. Soofi, A. Alizadeh, H. Hamishehkar, H. Almasi and L. Roufegarinejad, Preparation of nanobiocomposite film based on lemon waste containing cellulose nanofiber and savory essential oil: a new biodegradable active packaging system, Int. J. Biol. Macromol., 2021, 169, 352–361, DOI:10.1016/j.ijbiomac.2020.12.114.
- T. M. Vieira, M. Moldão-Martins and V. D. Alves, Composite Coatings of Chitosan and Alginate Emulsions with Olive Oil to Enhance Postharvest Quality and Shelf Life of Fresh Figs (Ficus carica L. cv. ‘Pingo De Mel’), Foods, 2021, 10(4), 718, DOI:10.3390/foods10040718.
- N. Oladzadabbasabadi,
et al., Recent advances in extraction, modification, and application of chitosan in packaging industry, Carbohydr. Polym., 2022, 277, 118876, DOI:10.1016/j.carbpol.2021.118876.
- Y. Zhang, K. Yang, Z. Qin, Y. Zou, H. Zhong and H. Zhang, Cross-linked gluten/zein nanofibers via Maillard reaction with the loading of star anise essential oil/β-cyclodextrin inclusions for food-active packaging, Food Packag. Shelf Life, 2022, 34, 100950, DOI:10.1016/j.fpsl.2022.100950.
- M. A. S. S. Ali, D. N. Jimat, W. M. F. W. Nawawi and S. Sulaiman, Antibacterial, Mechanical and Thermal Properties of PVA/Starch Composite Film Reinforced with Cellulose Nanofiber of Sugarcane Bagasse, Arabian J. Sci. Eng., 2022, 47(5), 5747–5754, DOI:10.1007/s13369-021-05336-w.
- Y. Shi, X. Cao, Z. Zhu, J. Ren, H. Wang and B. Kong, Fabrication of cellulose acetate/gelatin-eugenol core–shell structured nanofiber films for active packaging materials, Colloids Surf., B, 2022, 218, 112743, DOI:10.1016/j.colsurfb.2022.112743.
- J. Li,
et al., Emerging Food Packaging Applications of Cellulose Nanocomposites: A Review, Polymers, 2022, 14(19), 4025, DOI:10.3390/polym14194025.
- H. Shi, L. Wu, Y. Luo, F. Yu and H. Li, A facile method to prepare cellulose fiber-based food packaging papers with improved mechanical strength, enhanced barrier, and antibacterial properties, Food Biosci., 2022, 48, 101729, DOI:10.1016/j.fbio.2022.101729.
- E. von Falkenstein, F. Wellenreuther and A. Detzel, LCA studies comparing beverage cartons and alternative packaging: can overall conclusions be drawn?, Int. J. Life Cycle Assess., 2010, 15(9), 938–945 CrossRef.
- J. Korhonen, A. Honkasalo and J. Seppälä, Circular economy: the concept and its limitations, Ecol. Econ., 2018, 143, 37–46 CrossRef.
- A. Polyportis, R. Mugge and L. Magnier, Consumer acceptance of products made from recycled materials: a scoping review, Resour. Conserv. Recycl., 2022, 186, 106533 CrossRef.
- H. Caglayan and H. Caliskan, Life cycle assessment based exergoenvironmental analysis of a cogeneration system used for ceramic factories, Sustain. Energy Technol. Assessments, 2022, 52, 102078 CrossRef.
- L. Shen, E. Worrell and M. K. Patel, Environmental impact assessment of man-made cellulose fibres, Resour. Conserv. Recycl., 2010, 55(2), 260–274, DOI:10.1016/j.resconrec.2010.10.001.
- I. Leceta, P. Guerrero, S. Cabezudo and K. de la Caba, Environmental assessment of chitosan-based films, J. Clean. Prod., 2013, 41, 312–318, DOI:10.1016/j.jclepro.2012.09.049.
- F. Piccinno, R. Hischier, S. Seeger and C. Som, Life Cycle Assessment of a New Technology To Extract, Functionalize and Orient Cellulose Nanofibers from Food Waste, ACS Sustain. Chem. Eng., 2015, 3(6), 1047–1055, DOI:10.1021/acssuschemeng.5b00209.
- A. D. La Rosa and S. A. Grammatikos, Comparative Life Cycle Assessment of Cotton and Other Natural Fibers for Textile Applications, Fibers, 2019, 7(12), 101, DOI:10.3390/fib7120101.
- I. Bartolozzi, T. Daddi, C. Punta, A. Fiorati and F. Iraldo, Life cycle assessment of emerging environmental technologies in the early stage of development: A case study on nanostructured materials, J. Ind. Ecol., 2020, 24(1), 101–115, DOI:10.1111/jiec.12959.
- P. Gallo Stampino, L. Riva, C. Punta, G. Elegir, D. Bussini and G. Dotelli, Comparative Life Cycle Assessment of Cellulose Nanofibres Production Routes from Virgin and Recycled Raw Materials, Molecules, 2021, 26(9), 2558, DOI:10.3390/molecules26092558.
- E. Oliaei, T. Lindström and L. A. Berglund, Sustainable Development of Hot-Pressed All-Lignocellulose Composites—Comparing Wood Fibers and Nanofibers, Polymers, 2021, 13(16), 2747, DOI:10.3390/polym13162747.
- N. U. M. Nizam, M. M. Hanafiah and K. S. Woon, A Content Review of Life Cycle Assessment of Nanomaterials: Current Practices, Challenges, and Future Prospects, Nanomaterials, 2021, 11(12), 3324, DOI:10.3390/nano11123324.
- F. Foroughi, E. Rezvani Ghomi, F. Morshedi Dehaghi, R. Borayek and S. Ramakrishna, A Review on the Life Cycle Assessment of Cellulose: From Properties to the Potential of Making It a Low Carbon Material, Materials, 2021, 14(4), 714, DOI:10.3390/ma14040714.
- S. C. Carroccio, P. Scarfato, E. Bruno, P. Aprea, N. T. Dintcheva and G. Filippone, Impact of nanoparticles on the environmental sustainability of polymer nanocomposites based on bioplastics or recycled plastics – a review of life-cycle assessment studies, J. Clean. Prod., 2022, 335, 130322, DOI:10.1016/j.jclepro.2021.130322.
- N. Silva and H. Pålsson, Industrial packaging and its impact on sustainability and circular economy: a systematic literature review, J. Clean. Prod., 2021, 130165 Search PubMed.
- S. Otto, M. Strenger, A. Maier-Nöth and M. Schmid, Food packaging and sustainability – consumer perception vs. correlated scientific facts: a review, J. Clean. Prod., 2021, 298, 126733 CrossRef.
- Z.-C. Wang, Y.-X. Yin, H.-P. Ao, H. Yin, D.-F. Ren and J. Lu, The shelf-life of chestnut rose beverage packaged in PEN/PET bottles under long term storage: a comparison to packaging in ordinary PET bottles, Food Chem., 2022, 370, 131044 CrossRef CAS PubMed.
- Y. Biskri, M. Benzerara, L. Babouri, O. Dehas and R. Belouettar, Valorization and recycling of packaging belts and post-consumer PET bottles in the manufacture of sand concrete, Frat. Ed. Integrità Strutt., 2022, 16(62), 225–239 Search PubMed.
- S. Andreasi Bassi, D. Tonini, H. Saveyn and T. F. Astrup, Environmental and Socioeconomic Impacts of Poly (ethylene terephthalate)(PET) Packaging Management Strategies in the EU, Environ. Sci. Technol., 2021, 56(1), 501–511 CrossRef PubMed.
- S. Gerassimidou, O. V. Martin, S. P. Chapman, J. N. Hahladakis and E. Iacovidou, Development of an integrated sustainability matrix to depict challenges and trade-offs of introducing bio-based plastics in the food packaging value chain, J. Clean. Prod., 2021, 286, 125378 CrossRef CAS.
- S. Paidari, N. Zamindar, R. Tahergorabi, M. Kargar, S. Ezzati and S. H. Musavi, Edible coating and films as promising packaging: a mini review, J. Food Meas. Char., 2021, 15(5), 4205–4214 CrossRef.
- M. M. Jayakody, M. P. G. Vanniarachchy and I. Wijesekara, Seaweed derived alginate, agar, and carrageenan based edible coatings and films for the food industry: a review, J. Food Meas. Char., 2022, 16(2), 1195–1227 CrossRef.
Footnote |
† Equal contribution. |
|
This journal is © The Royal Society of Chemistry 2023 |
Click here to see how this site uses Cookies. View our privacy policy here.