Biological and postharvest interventions to manage the ethylene in fruit: a review
Received
3rd March 2023
, Accepted 28th June 2023
First published on 29th June 2023
Abstract
Ethylene, the gaseous ripening hormone, notably influences the postharvest physiology of the fruit. The pronounced effect of ethylene on postharvest physiology ranges from beneficial to unacceptable, with substantial commercial repercussions. Climacteric fruit undergoes an ethylene burst with the onset of ripening, and this autocatalytic ethylene production leads to accelerated ripening and senescence of fruit. Thus, the fruit becomes soft, mealy, and prone to pathogen attack that ultimately leads to high postharvest losses during handling, storage, and marketing. The key to appropriate handling, storage, and marketing of fruit lies largely in the successful management of ethylene. Biological interventions such as gene silencing, gene editing and recently the gene modifications through CRISPR/Cas9 are widely accepted and being used to regulate ethylene in fruit. Nevertheless, postharvest interventions and use of novel molecules such as 1-MCP, polyamines, salicylic acid, and ozone have received commercial status for controlling ethylene action in fruit. Use of metal-based catalysts at low temperature has been considered as a safer approach. This review focuses on the history, biosynthesis, action mechanism and techniques employed for useful modulation of ethylene biosynthesis and action in harvested fruit.
Sustainability spotlight
Ensuring safe food production with maximum quality retention through eco-friendly sustainable means is extremely important. Ethylene is a key compound hormone which accelerates the ripening process and triggers senescence. Managing the ethylene in the food supply line is crucial in order to maintain food safety and postharvest loss. Use of a single technology and approach is insufficient to combat the problems but managing ethylene using available sustainable technologies in an integrated manner could be worthwhile and provide a sustainable solution. In this context, we have presented the available biological and postharvest interventions to manage ethylene biosynthesis as well as action during handling. Use of novel biological tools such as gene editing, RNA sequencing, CRISPR/Cas9 and phytohormones could provide alternative long-term solutions. In addition to that, use of physical treatments, irradiation, oxidative materials and altering the physiology by means of certain agents are also equally important. Our work emphasizes the importance of certain sustainable technologies for food safety as mentioned in the UN sustainable goals of food safety and sustainable agriculture (SDG2) and climate change (SDG13).
|
1. Introduction
Ethylene (H2C
CH2) is a gaseous hydrocarbon. As a plant hormone, it is unique and spectacular in its structural simplicity but dramatic diverse physiological effects, especially in postharvest ripening and senescence of fruit.1–3 In addition, the ethylene level in the storage atmosphere is also augmented inadvertently due to rotting fruit, injured plant tissue, motor exhaust pollution, industrial activities and microbial sources.1,4
Ethylene action is the primary determinant of the rate of loss in quality and marketability of fruit, particularly of climacteric type. Yet, non-climacteric fruit and vegetables are also sensitive to ethylene exposure displaying quality loss and increased susceptibility to decay and physiological disorders.5
Ethylene plays a key role in escalating the rates of postharvest quality deterioration by affecting skin colour, cell turgor and structural changes leading to loss of texture, increasing respiration rate and cell wall degrading enzyme activities.1,2,6–8 The physiological activities in response to ethylene sensitivity are triggered at concentrations as low as ppm to ppb levels with threshold concentrations varying among different crops.9 However, the effects of ethylene on postharvest physiology and storage vary with several factors such as pre-harvest conditions, fruit species, cultivar, ethylene concentration, exposure duration, temperature and relative humidity of storage.10 Ethylene detection techniques such as gas chromatography, electrochemical methods, colorimetric and luminescence methods, laser-based sensors, organic field effect transistors and surface-enhanced Raman spectroscopy are being used to detect ethylene.11
Some biotechnological tools have also received significant attention and could be utilized. Use of gene editing, modifications and some of the latest CRISPR/Cas9 for gene modification has been employed in fresh produce.12 A novel technology to develop mutants at genome specific sites in a variety of fruit plants to regulate fruit colour, quality traits, aroma and shelf life, CRISPR/Cas9 generates novel mutants that affect RIN protein accumulation and fruit ripening phenotypes in tomato.13 Use of biological materials such as plant extracts, plant based novel biological molecules, phytohormones, edible coatings, micro-organisms and biofiltration could be effectively practiced in perishables to combat the negative effects associated with ethylene.
In addition to that, important pivotal approaches such as ethylene biosynthesis blocking agents (aminoethoxyvinyl glycine), ethylene action blocking agent (1-methylcyclopropene), ethylene scavenging agents (potassium permanganate, biofiltration, ozone), packaging scrubbers (titanium dioxide), adsorbents (zeolite) and plant based signaling molecules (brassinosteroids, salicylic acid, polyamines, etc.), and storage technologies are being commercially used.14–17
Thus, it is imperative to look upon postharvest physiology triggered/induced by ethylene, causing postharvest deterioration, rotting and quality changes. There is a need to incorporate comprehensive, practical, easy-to-adopt solutions in storage and supply chains with renewed interest and caution to encourage better management of perishable horticultural commodities during commerce. This review aims to compile, present and discuss the effects of ethylene in fruit after harvest and discuss recent studies and postharvest approaches to delay and inhibit ethylene action on horticultural commodities to slow down and prevent their deterioration by blocking biosynthesis, perception and/or scavenging, thus lowering the postharvest losses.
2. History of ethylene
In 1886, Dimitry Nikolayevich Neljubow, a graduate student, observed that abnormal growth of etiolated pea seedlings was due to ethylene released from lamps.18 This is referred to as ‘triple response’ due to ethylene causing inhibition of hypocotyl and root cell elongation, radial swelling of the hypocotyl and increased curvature of the plumular hook region. In 1910, H. H. Cousins reported that during storage, bananas senesced/over-ripened at faster rates due to a volatile product emanating from oranges. Notably, R. Gane in 1934, with the advent of the gas chromatography technique, identified and reported that the volatile substance was ethylene. Methionine as the precursor of ethylene was established by the pioneering work of M. Lieberman and L. W. Mapson in 1964. Later, D. Adams and F. Yang established that S-adenosyl methionine (SAM) and 1-aminocyclopropane-1-carboxylic acid (ACC) are the intermediates in ethylene biosynthesis (Fig. 1). In the following years, the research interest in ethylene rocketed with the realization of its dramatic influence on fruit ripening and senescence and the associated economic and humongous postharvest loss impacts.
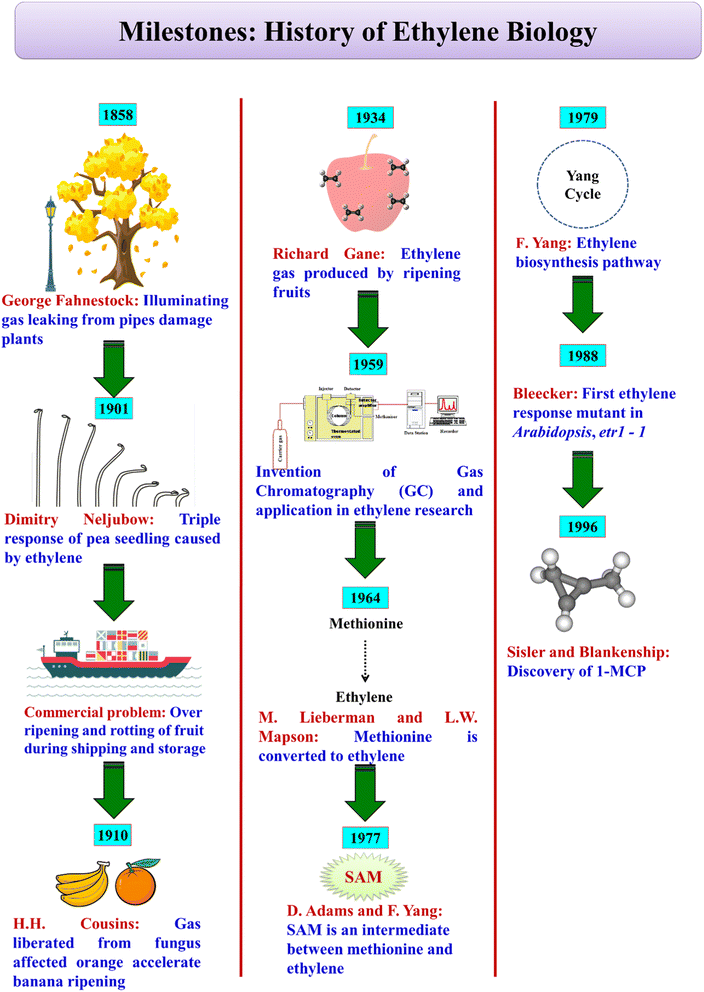 |
| Fig. 1 History of ethylene biology. | |
3. Ethylene biosynthesis and action
Ethylene is derived from amino acid L-methionine which is converted to S-adenosyl methionine (SAM) by SAM synthetase. SAM is a key methyl donor in plants and plays an important role in other biochemical pathways such as polyamine biosynthesis.19 SAM is then converted to the cyclic non-protein amino acid ACC (1-aminocyclopropane-1-carboxylic acid) by the ACC synthase (ACS) present in the cytoplasm.20 ACS also produces 5′-methylthioadenosine (MTA) which is recycled to methionine via a modified methionine cycle.21 In the final step, the synthesis of ethylene requires oxygen to oxidize ACC to ethylene by ACC oxidase (ACO) with subsequent production of carbon dioxide and cyanide. The produced cyanide is converted to β-cyanoalanine by the enzyme β-cyanoalanine synthase to reduce the toxic effect of the accumulated cyanide during increased ethylene production.22
The produced ethylene binds with the receptors embedded in cells. The receptors remain active in the absence of ethylene, allowing normal growth of fruit attached to the plant.23 However, with the onset of ripening, the produced ethylene binds with the receptors and switches them off. It has been suggested that two systems operate for ethylene regulation in climacteric fruit. System 1 produces a small amount of ethylene during the pre-climacteric period or early fruit growth stage in climacteric fruit and throughout the development and ripening in non-climacteric fruit. However, with the onset of fruit ripening system 2 operates in climacteric fruit that produces auto-stimulated massive ethylene evolution. However, exogenous application of ethylene to non-climacteric fruit during ripening may hasten the process in some cases.24 In tomato fruit, it has been observed that genes ACS6 and ACS1A are involved in system 1 which are down-regulated by ethylene, while ACS2 and ACS4 genes are involved in system 2 of ethylene production. Thus, the main reason behind discrete production of ethylene in pre-climacteric and climacteric phases is involvement of certain genes at a particular stage of fruit development.25
The ethylene signaling pathway includes both positive and negative regulators. The perception of ethylene takes place by a family of five membrane bound receptors: ETR1, ETR2, ERS1, ERS2 and EIN4.26 The binding of ethylene with its receptors takes place via a copper cofactor delivered by copper transporter RAN1. CTR1 (constitutive triple response 1) gene, encoding a Raf-like protein kinase, suppresses the positive regulator and downstream responses of EIN2 and EIN3/EIL transcription factors. When ethylene binds with the receptors, it does not activate CTR1 but activation of EIN2, EIN3, and EIL occurs, leading to the induction of the ripening signal in fruit.27 ERFs are transcription factors encoded by large families of plant transcription factors due to which a wide variation in ethylene response in climacteric and non-climacteric fruit exists.25 A study conducted on tomato revealed that most ERFs are ethylene inducible and show a positive response towards ripening, which suggests that ERFs may represent the connecting link between signaling of ethylene and its regulation during fruit development and ripening. Hence, ERFs could also be used as one of the strategies to control the ethylene during fruit storage.28 The transcriptional regulation of ethylene suggests a significant difference between climacteric and non-climacteric signaling of ethylene by varying the number of transcription factors. A higher number of ETRs are reported in climacteric fruit while non-climacteric fruit were characterized by the earlier expression peak of ETRs.29
4. Management strategies for ethylene
4.1. Biotechnological approaches
Several biotechnological interventions have been made so far to suppress ethylene production in fruit. Ethylene biosynthesis involves the activity of ACC synthase (ACS) and ACC oxidase (ACO), which play a regulatory role in converting SAM to ACC and ACC to ethylene, respectively. Delay in fruit ripening can be done by incorporation of silencing genes coding for ACS and ACO or expression of genes coding for enzymes associated with breakdown of the ethylene precursor. RNA antisense technology is also an effective tool to regulate the expression of fruit ripening genes.30,31 About 99.5% reduction in ethylene production by inhibition of ACC synthase mRNA was achieved in tomato by inserting a gene including the untranslated portions in an opposite orientation under the CaMV (cauliflower mosaic virus) 35S promoter.32 In another study, about 87% suppression of ethylene production was achieved in tomato fruit by inserting the antisense version of ACC oxidase and overexpressing mRNA in an antisense orientation.33 A similar approach of overexpressing an antisense ACC oxidase gene when used in Cantaloupe melon showed a 99% suppression in the ethylene level.34 During this period in 1994, FlavrSavr® tomato was developed by Calgene Corporation which was the first genetically engineered whole food approved for commercial sale by the Food and Drug Administration (FDA).35 In Cantaloupe Charentais melon, when antisense ACC oxidase gene was overexpressed, it showed more than 99% suppression in ethylene evolution.36 In a similar study, Silva et al.37 developed a transgenic melon that showed about 39-fold higher inhibition in ethylene production than that of wild-type by overexpression of an antisense ACC oxidase construct from apple. Application of antisense technology targeting ACC oxidase was also found to be effective in the suppression of ethylene by about 99.9% in muskmelon cv. Galia.38 Some researchers targeted ACC deaminase to control ethylene production in tomato. Klee et al.39 found 90% suppression in ethylene production and a delay in the climacteric peak by up to 3 days in tomato, in which the gene for ACC deaminase was inserted and overexpressed from Pseudomonas chloraphis 6G5.
Elevating the level of polyamines by biotechnological tools was also targeted by some researchers to reduce ethylene production as both the pathways are interlinked and SAM is the common precursor. The accumulation of polyamines (putrescine, spermidine and spermine) was enhanced in tomato by about 2-fold by overexpressing ADC (arginine decarboxylase) gene which showed about 44% reduction of ethylene production.40 A similar approach to increase the level of polyamines in tomato was also used by overexpressing S-adenosyl methionine decarboxylase (SAMdc), ornithine decarboxylase (ODC) and spermidine synthase (SpdSyn) genes to suppress ethylene production.1,41,42 Event 35-1-N in tomato was developed by Agritope Inc. in which a sam-k gene construct encoding SAM hydrolase was introduced leading to degradation of SAM and reduction in ethylene production.43 Tomato event 1345-4 (trade name: Endless Summer) was developed by DNA Plant Technology Corporation by inserting an ACS gene construct that suppressed conversion of SAM to ACC. Monsanto developed event 8338 in tomato by inserting the ACC deaminase (ACCd) gene responsible for ACC metabolism. In 1995, the cultivation of tomato event 1345-4 was approved in USA by the FDA and APHIS. Nevertheless, it was cultivated for a limited period while events 8338 and 35-1-N was deregulated in 1995 and 1996, respectively. Melon events A and B were developed with delayed ripening and approved in 1999 by the FDA; however, their cultivation was not approved.44 Likewise, tomato event Huafan No. 1 was developed in China by inserting an anti-efe (ethylene-forming enzyme) gene construct coding for an antisense RNA of ACO. It was approved in 1997 by the Ministry of Agriculture in China for cultivation, food and feed use.
Besides, CRISPR/Cas 9 gene editing technology has been used to regulate ripening and ethylene in climacteric fruit.45,46 It is now widely accepted that CRISPR/Cas9 has become a popular technology for gene manipulation and complete gene modification because of its high efficiency, simplicity and low cost.12 Many researchers used CRISPR/Cas9 for validation of functionality and inheritance of mutation of transcription factor RIPENING INHIBITOR (RIN) gene in tomato.13 It has been observed that CRISPR/Cas9 mediated mutations at the RIN locus inhibited ripening in tomato fruit and extended its shelf life. Moreover, CRISPR/Cas9 mediated mutagenesis of RIN gene, lycopene desaturase (PDS), pectate lyases (PL), SlMYB12, and CLAVATA3 (CLV3) regulated the ripening and biochemical traits in fruit.12
4.2. Postharvest interventions for ethylene control
4.2.1. Physical treatments.
There are several conventional and non-conventional ways to control ethylene evolution in fruit. Physical measures such as use of UV-C radiation, gamma irradiation, and hot water treatment are practiced to date in order to keep in check the ethylene evolution rate in harvested fruit (Table 1).
Table 1 Recent efforts in ethylene management by using physical treatments
Commodity – cultivar |
Treatments and dose |
Major research output |
Ref. |
Tomato |
Photocatalyst (TiO2) illuminated with UV light |
Complete oxidation of ethylene |
89
|
Green lime – ‘Paan’ |
Hot water immersion (50 °C for 5 min) |
Inhibited ethylene production and respiration |
48
|
Tomato – ‘Qirui F50’ |
Hot water treatment at (37 °C ± 0.5 °C) for 15 min |
Degenerated ACC synthase enzyme and suppressed LeERT10 and LeEIN3 |
49
|
Apples –‘Granny Smith’ |
X-ray irradiation @ 310 Gy and 1000 Gy |
Downregulated the ethylene biosynthesizing gene expression and reduced the ethylene evolution rate |
53
|
Ber – ‘Umran’ |
Gamma irradiation at 0, 0.4 and 1.0 kGy doses |
Ethylene peak was delayed by 7 days at 1.0 kGy dose |
52
|
Cherry tomato |
UV-C (4.2 kJ m−2) for 35 days |
Inhibited ethylene production |
57
|
Banana – ‘Berangan’ |
UVC irradiation (0.01–0.05 kJ m−2) |
Reduced ethylene production compared to control |
56
|
Kiwifruit – ‘Guichang’ |
Ozone fumigation (0, 100, 200, and 300 μL L−1 for 3 h) |
200 μL L−1 ozone blocks ethylene biosynthesis by preventing ACC synthesis |
175
|
In the last few years, hot water and vapor heat treatments have drawn potential interest for reducing ethylene evolution, thus delaying the ripening and senescence of fruit and vegetables. Use of such physical treatments is growing now-a-days as it is environment friendly and does not leave any residue in the fruit and vegetable.47 Earlier studies have shown that hot water treatment effectively reduces the production of ethylene in lime fruit by reducing the accumulation of ACC (1-aminocylocpropane-1-carboxylic acid) or eliminating the stimulation of ACS (1-aminocylocpropane-1-carboxylic acid synthase) and ACO (1-aminocylocpropane-1-carboxylic acid oxidase) involved in the biosynthesis of ethylene.48 Moreover, heat treatment above 37 °C causes degeneration of ACS enzyme and suppression of LeERT10 and LeEIN3, thus increasing the expression of SlERF-A1, hence delaying the accumulation of ethylene as found in tomato fruit.49 In contrast, vapor heat treatment (50 °C) for 5–10 min stabilized the metabolic activities of sweet orange fruit by reducing ethylene production and respiration rate, thereby retaining the fruit quality attributes.50
Irradiation is another physical treatment in which horticultural produce is exposed to a beam of γ rays or high energy electrons or X-rays for a stipulated time for extending the shelf life of the produce or reducing the ethylene production during storage. Irradiation treatment has been widely used to reduce the microbial load, inhibit sprouting of tubers and bulbs, delay ripening, extend shelf life and sterilize produce against insects and pests.51 One of the major uses of this irradiation treatment is to reduce the ethylene production and delay the senescence process which has been practiced in several fruit crops such as ber and apple.52,53 Irradiation hinders ATP production required for synthesis of ripening promoting enzymes. It also affects the carbohydrate metabolism and process of gluconeogenesis and therefore delays the action of ethylene and improves the shelf life of the commodity.54 Two major limitations in its use are the initial capital cost and slow consumer acceptance due to the perceived association with radioactive radiation. If an adequate volume of product is available for treatment, then the cost will be minimized and maximum benefits can be incurred from the technology.55
Another effective physical treatment in management of ethylene is the use of UV-C radiation. It has been applied in several fruits and vegetables such as banana and cherry tomatoes.56,57 UV-C irradiation at a wavelength of 254 nm is more effective in maintaining the physiological response by alleviating the stress responses with respect to production of defense related compounds such as malondialdehyde and proline as observed in bitter gourd fruit.58 UV-C treatment at 4.1 kJ m−2 postponed the onset of the ethylene evolution peak in wounded tomato fruit when it is dropped from a 1.5 m height and stored for 12 days at 20 °C. UV-C reduced the ethylene evolution rate due to decreased activity of ACO enzyme which is responsible for the ethylene biosynthesis.59 UV-C technology for ethylene reduction is a cost-effective and safe approach as mentioned by Pristijono et al.60 The limitation in using UV-C is that it does not penetrate the surface into much deeper regions, and thereby the source of UV-C for disinfection must be placed closer to the target in the processing system.61
4.3. Use of biosynthesis inhibitors
4.3.1. Aminoethoxyvinylglycine (AVG).
Aminoethoxyvinylglycine (AVG) impedes the conversion of S-adenosylmethionine to 1-aminocyclopropane carboxylase (ACC) by binding and thus inhibiting the ACC synthase enzyme activity hindering ethylene biosynthesis. It is a naturally occurring plant growth regulator. It is registered commercially and used for several crops like pome fruit (apple, pear), stone fruit (peach, plum and nectarine) and mandarins in both pre and postharvest stages under the trade name ReTain®.62 It was first patented in the United States in 1973. Also, US has permitted use of AVG in organic farming.63 Recently, it has been provided compliance with organic farming in Australia too (https://www.sumitomo-chem.com.au/retain). The effects of AVG, an off-white to pale yellow fine water-soluble powder, have been found to vary depending on the dose, time of application, species and cultivar.62 It is a natural fermentation metabolite obtained from Streptomyces spp.63 The remarkable effects of AVG application on delaying senescence are mainly attributed to the reduction in ethylene production and respiration rate, delaying colour change and loss of weight and texture, thus maintaining edible quality and storability over a longer period. In addition, several studies have reported a reduction in the incidence and severity of development of physiological disorders like superficial scald, water core and internal/core browning, and stem bowl cracking.62,64,65 Besides, beneficial influences on fruit such as delaying of tree fruit maturation and ripening often lead to better edible quality and malleable harvest management, as well as uniform ripening, leading to fewer pickings and reduced fruit drop/fall. However, some negative effects such as delay in uniform blush colour development, decrease in juiciness and lowered aroma volatiles synthesis have also been reported. Recent studies on the effects of different AVG treatments are presented in Table 2. The positive effects of AVG treatment have been reported in tomato, apple, pear, apricot, peach, nectarine and several others.62,66
Table 2 Recent efforts in ethylene management by using aminoethoxy vinyl glycine (AVG)
Commodity- cultivar |
Treatments and dose |
Major research output |
Ref. |
Persimmon – Sangjudungsi |
1-MCP (1 μL L−1) dipping in 75 mg L−1 AVG for 5 min |
1-MCP maintained maximum phenolics content. Overall, treatment of persimmon with 1-MCP and AVG lowered incidence of physiological disorders |
183
|
Apricot – Canino |
1-MCP (AgroFresh® 500, 1000 μg L−1 at 20 ± 2 °C for 12 h); AVG (50, 100 mg L−1 JA (50, 100 nmol) |
1-MCP at 1000 μg L−1 followed by AVG at 100 mg L−1 were reported most effective for maintaining fruit quality and delaying deterioration |
109
|
Banana – Cavendish |
AVG (1.25 ppm) and chitosan (2.5%) |
Combined AVG, chitosan, and low storage temperature increased shelf-life by four days over control |
184
|
Sweet Cherry – 0900 Ziraat |
Retain (pre-harvest- 50, 100, 150 mg L−1) |
AVG (100 mg L−1) delayed colour change, softening, acidity loss and maintained sensory quality |
64
|
Kiwifruit – Hayward |
MAP; AVG (225 mg L−1), AVG (225 mg L−1) + MAP |
Weight loss was delayed by MAP while AVG alone did not influence weight loss and quality |
185
|
Peach – Oz delight |
AVG (10 days before harvest); AVG pre and postharvest (10 μL L−1) AVG + SA (pre and postharvest respectively), NO; SA (2 mM) |
Pre-harvest AVG treatment with post-harvest SA reduced ethylene evolution, respiration, oxidative stress, softening and delayed changes in quality like colour, soluble solids and acids |
186
|
Plum – Friar |
AVG (0, 100, 200 and 300 mg L−1) |
AVG deferred ripening by lowering respiration rate, chilling injury, maintained fruit quality and enhanced antioxidant capacity and shelf life over control |
187
|
Pummelo – Tubtim siam |
1-MCP microbubble, AVG – (500 ppm) |
AVG significantly lowered respiration rate and ethylene evolution. AVG and 1-MCP-microbubbles maintained both external and internal postharvest quality |
188
|
4.3.2. Aminooxyacetic acid, norbornadiene and diazocyclopentadiene.
Aminooxyacetic acid (AOA) is an ethylene inhibitor. It has similar action to AVG, i.e., it inhibits ACC synthase activity. Norbornadiene (NBD), a cyclo-olefin, having structural similarity to ethylene, intercepts ethylene union to receptors, thereby suppressing the deterioration. DACP (diazocyclopentadiene) shows similar activity against ethylene. The action, however, is transient and temporary as they leave the receptors within a fortnight, allowing normal ripening henceforth.67 Nevertheless, the use of these compounds is considered objectionable due to the associated toxicity and manufacturing issues.68
4.4. Use of oxidative compounds
4.4.1. Potassium permanganate.
Potassium permanganate (KMnO4) is a coarse purplish-black coloured powder which dissolves in water to give intensely pink to purple-coloured solutions. It is generally held onto porous inert materials such as silica (SiO2), zeolite, montmorillonite, kaolinite, perlite, alumina (Al2O3), vermiculite and activated carbon to enable adsorption and oxidation of ethylene gas molecules effecting their removal from packaging and storage environments.69 A silica support is often used for its manifold benefits such as low cost, stability, high surface area, and easy availability, while alumina and vermiculite exhibit high adsorption capacity due to their high surface area and low-cost benefits.70 Activated carbon, particularly granular forms, produced by pyrolysis of carbonaceous materials is a non-crystalline porous support material frequently used for holding KMnO4.71 However, activated alumina and zeolite are most often used for commercial applications. Amongst powder, fibre and granular forms, frequently granular forms have been reported to show higher effectiveness. It is reported to be lethal at a dose of 142.9 mg per kg body weight.72,73 High surface area, shape and size of particles, adsorption ability, light weight, low cost, non-toxicity, stability, easy production and availability, hydrophobic nature, and KMnO4 dose are desired qualities in the support material required for holding KMnO4.71,74–76 In addition, the extent of adsorption and the surface area of the supporting/holding material also influence the scavenging activity.75,77 Ethylene is adsorbed onto the porous surface, followed by activation by moisture obtained from transpiration and respiration of fresh horticultural commodities, and oxidation of carbon–carbon double bonds by KMnO4 to produce CO2 and H2O as end products which is visibly indicated as a colour change from purple KMnO4 to brown MnO2.78 The colour change from purplish to brown, due to formation of MnO2, is an indicator of the end of active usage due to saturation and removal of ethylene by the oxidative action of KMnO4. It is commonly used as an effective, low-cost technology for scrubbing away ethylene and oxidizing it to carbon dioxide and water.77,79 The concentration of KMnO4 in commercial packages ranges between 4 and 6%. It is frequently used at the commercial level in active packaging in highly permeable sachets, during transportation and storage, and even at domestic levels in refrigerators for removal of ethylene from the surroundings and thus extends the storability of fresh horticultural produce.76 Moreover, it has also been suggested that the cost of KMnO4 is much less than that of ozone or irradiation and does not add additional cost to the produce.
However, Pathak80 rightly noted that a major limitation in KMnO4 embedded scrubbers is that they often need to be substituted with fresh permeable sachets to maintain effectiveness over longer storage periods. Moreover, KMnO4 cannot be used directly in contact with food as it is toxic and due to possible migration of purple colour to the food at high moisture conditions.79,81,82 Yet, due to its solubility in water (6.4 g per 100 mL at 20 °C), KMnO4 can be easily washed away from the surface of fresh whole fruit and vegetables. Some recent efforts in ethylene management using potassium permanganate are listed in Table 3 and commercial products for ethylene removal are listed in Table 4.
Table 3 Recent efforts in ethylene management by using potassium permanganate
Commodity- cultivar |
Treatments and dose |
Major research output |
Ref. |
Nectraine – Bayramiç Beyazi |
Ethylene sachets (10 g KMnO4) with MAP. Storage at and 90% RH for 40 days |
Maintained overall fruit quality for up to 40 days at 0–1 °C and 90% RH |
176
|
Guava – Baruipur |
Moisture scavenger sachet (46 g) ethylene scavenger sachet (3 g) |
Lowest chilling injury and highest L * value during 32 days storage period |
177
|
Blueberries – Duke |
KMnO4 with protonated montmorillonite clay |
Extended shelf life by 25 days and 14 days at 2 °C and 10 °C, respectively |
79
|
Apricot – Mirlonaranja |
KMnO4 with sepiolite |
No C2H4 concentration, reduced weight and decay loss |
178
|
Anona – Pinha |
Ethylene scavenging sachets ofKMnO4 (3 g) |
Treatments delayed ripening and preserved food value at 13 °C for 20 days |
179
|
Tomato |
KMnO4 based cotton absorber |
Delayed ripening, maintained texture and extended storability of tomato at room temperature |
72
|
Table 4 List of commercially available products for ethylene management
Product name |
Manufacturer |
Chemical component/description |
Mode of action |
Material type |
SmartFreshSM |
AgroFresh, Inc., USA |
1-Methyl cyclopropene |
Inhibition of ethylene action |
Sachets and other |
ReTain® |
Valet BioSciences Corporation, USA |
Aminoethoxy vinyl glycine |
Inhibition of ethylene action |
Powder |
Purafil |
Purafil Inc., GA, USA |
KMnO4 (≥8%) in activated alumina |
Ethylene oxidation |
Spherical pellet (3.2 mm dia.) |
Purafil Chemisorbant |
Purafil Inc., GEO, USA |
KMnO4 (≥4%) in activated alumina |
Ethylene oxidation |
Spherical pellet (3.2 mm dia.) |
Ethysorb® |
Molecular Products Ltd., UK |
KMnO4 (<6%) in activated alumina |
Ethylene oxidation |
Spherical particle (2.5–5.0 mm dia.) |
Sofnofil™ |
Molecular Products Ltd., UK |
KMnO4 (<6%) in activated alumina |
Ethylene oxidation |
Spherical particle (2.5–5.0 mm dia.) |
Multi-Mix® MM-1000 |
Circul-Aire Inc., Canada |
KMnO4 (4%) in activated alumina |
Ethylene oxidation |
Spherical particle (3.2 mm dia.) |
BrySorb™ 508 |
Bry-Air (Asia) Pvt. Ltd. India |
KMnO4 (conc. ns) in activated alumina |
Ethylene oxidation |
Spherical particle (2.5–3.5 mm dia.) |
Air repair™ ethylene gas absorber |
Delta Track Inc., CA, USA |
KMnO4 (conc. ns) in activated alumina |
Ethylene oxidation |
Spherical particle (dia. ns) |
Ozeano ETH |
Ozeano Urdina S.L., Spain |
KMnO4 (7.5%) in alumina |
Ethylene oxidation |
Spherical particle (3.0–5.0 mm dia.) |
Ryan® |
Sensitech Inc., MA, USA |
KMnO4 (conc. ns) in natural clays |
Ethylene oxidation |
Sachets, filters |
Bi-On® |
Bioconservacion S.A., Spain |
KMnO4 (12%) in zeolite |
Ethylene oxidation |
Cylindrical pellet (2.3–4.0 mm dia.) |
Super Fresh Media |
Ethylene Control Inc., CA, USA |
KMnO4 (4–6%) in zeolite |
Ethylene oxidation |
Granule (dia. ns) |
Extend-A-Life™ |
AgraCoTechologies International LLC., PE, USA |
KMnO4 (8%) in zeolite |
Ethylene oxidation |
Granule (dia. ns) |
ETI 25, 50 |
Fruit Control Equipentssrl, Italy |
ABSOTIL/purafil alumina pellets |
Ethylene oxidation |
Absorption system |
EC-3+ |
Ethylene Control Inc., USA |
Zeolite impregnated with KMnO4 |
Ethylene oxidation |
Sachets, filters, filtration system |
Air repair ethylene absorbers |
DeltaTRAK, Inc., USA |
Sachet of KMnO4 |
Ethylene oxidation |
Packet, blankets, tube |
Bio-Turbo |
Miatech, Inc., USA |
Ozone |
Ethylene oxidation |
Air filtration equipment |
Kuraray Coal™ |
Kuraray Co. Ltd., Japan |
Activated carbon/KMnO4 |
Ethylene oxidation |
Sachets |
Hatofresh |
Honshu Paper, Japan |
Activated carbon impregnated with bromine |
Ethylene oxidation |
Paper bag or corrugated fiber board |
Air-repair |
Cal Agri Co., USA |
KMnO4 |
Ethylene oxidation |
Sachets/blankets |
Bio-Kleen |
Kes Irrigations Systems, USA |
KMnO4 |
Ethylene oxidation |
Sachets/blankets |
Green Pack® |
Rengo Packaging Systems Co., Ltd. Japan |
KMnO4/silicon oxide |
Ethylene oxidation |
Sachets |
Delta Track® |
Delta Track Inc., USA |
KMnO4 |
Ethylene oxidation |
Sachets/blankets |
CJS® |
CJS Ethylene Filters, USA |
KMnO4 |
Ethylene oxidation |
Sachets, filters |
Retarder® |
Retarder S.R.L, Italy |
A mix of clays and KMnO4 |
Ethylene oxidation |
Sachet |
GKZ4 |
Greenkeeper Iberia, Spain |
Zeolite loaded with KMnO4 |
Ethylene oxidation |
Sachet/sheet |
BE fresh |
Befresh Technology, Spain |
Natural clays impregnated with KMnO4 |
Ethylene oxidation |
Sachet |
Purethyl |
Isolcell Spa, Italy |
Activated alumina granules with KMnO4 |
Ethylene oxidation |
Machine |
Mrs Green extra life |
Dennis Green Ltd., USA |
KMnO4 |
Ethylene oxidation |
Cartridge for refrigeration |
Extend-A-Life™, Produce Saver™ |
AgraCo Technologies International LLC, USA |
KMnO4 based absrober |
Absorption/adsorption |
Filters, sachets |
It's Fresh! |
It's Fresh! Ltd., UK |
Palladium impregnated zeolite |
Absorption/adsorption |
Sheet |
Prime Pro® |
DeltaTRAK, Inc., USA |
LLDPE plastic film |
Absorption/adsorption |
Sheet |
Peakfresh |
Peakfresh, Australia |
LDPE film impregnated with a naturally occurring mineral |
Absorption/adsorption |
Bags |
Green Bags™ |
Evert-Fresh Corporation, USA |
Clay |
Absorption/adsorption |
Bags |
KEEPFRESH® |
Teck Blue Systems, SL Keepfresh, Spain |
Zeolite and KMnO4 sachets/sheets |
Absorption/adsorption |
Sheets, bags |
Bi-On®, ETHYL STOPPER |
Bioconservacion S.A., Spain |
Porous clay and KMnO4 |
Absorption/adsorption |
Filtration system, sachets |
Sendo-Mate |
Mitsubishi Chemical Co. Japan |
Palladium catalyst on activated carbon |
Absorption/adsorption |
Sachets |
Orega |
Cho Yang Heung San Co., Korea |
PE film, filler includes pumice, zeolite, activated carbon and metallic oxides |
Absorption/adsorption |
Film |
Sendo Mate |
Mitsubishi Gas Chemical Co. Ltd., Japan |
Activated carbon with a metal catalyst |
Absorption/adsorption |
Sachet |
Neupalon |
Sekisui Jushi Corp., Japan |
Activated carbon with a metal catalyst |
Absorption/adsorption |
Sachet |
Keepcool |
Molina de Segura, Spain |
Sepiolite mixed with KMnO4 and activated carbon |
Absorption/adsorption |
Sachet |
Profresh |
E-I-A Warenhandels GmbH, Austria |
Minerals |
Absorption/adsorption |
Film |
Biofresh |
Groft Plastics, Israel |
Porous clay and potassium permanganate mixture |
Absorption/adsorption |
Zipper bag |
Swingtherm-BS |
Fruit Control Equipmentssrl, Italy |
Air filtration |
High temperature catalytic oxidation |
Air filtration equipment |
ECOscrub |
Absorger, France |
Titanium dioxide + UV light |
Photocatalytic oxidation |
Air filtration equipment |
AiroCide® |
KES Science & Technology, Inc |
Air filtration |
Photocatalytic oxidation |
— |
FRESH+™ |
Fresh Plus International, USA |
Catalyst + UV light |
Photocatalytic oxidation |
— |
Bo film |
Odja Shoji, Japan |
LDPE films extruded with finely divided crysburite ceramic |
— |
Film |
Evert-Fresh |
Evert-Fresh., USA |
LDPE films impregnated with Oya- stone |
— |
Film |
4.4.2. Ozone.
Ozone treatment is gaining popularity now-a-days as it is environment friendly, easy to use and does not leave any residue. Ozone was incorporated in 2001 in the GRAS list as a food additive by the United States Food and Drug Administration (FDA).83 It is a strong oxidizing agent, used for ethylene removal by conversion to carbon dioxide and water.82 Besides, it is also instrumental in the destruction of pathogenic microbes for fruit and vegetable preservation.84 Ozone is generated on site as it is a highly reactive free radical which decomposes readily to form oxygen.78,82 It is produced by illuminating ultraviolet (UV) radiation (285 nm wavelength) or passing a high voltage alternating current (corona discharge generator) in an oxygen or air stream. However, it can be used only with caution as the safe exposure level for human health is 0.1 μL L−1.85 Mahapatra et al.86 reported that the Occupational Safety and Health Administration (OSHA), USA has suggested a worker exposure limit of 1.5 minutes at a 0.3 μL L−1 level, whereas exposure for a few minutes can be lethal at >1700 μL L−1 levels. Ozone is also used for inhibiting the expression of ACS1 and ACO1 genes which further reduced the activities of ACS and ACO enzymes thereby reducing the biosynthesis of ethylene and thus delaying the ripening of fruit.87 It has been approved for use during food processing, treatment and storage in gas and aqueous states. Notably, the usefulness of ozone is high at high relative humidity due to enhanced solubility in the condensed surface moisture of fruit and vegetables.88
4.4.3. Photocatalytic oxidation by metals.
Recent advances in postharvest management of ethylene in fruit and vegetables include the use of photocatalytic materials such as titanium dioxide (TiO2) illuminated with ultraviolet light. Titanium dioxide is a chemically inert, environmentally friendly, highly photo-stable and least expensive photocatalyst. When titanium dioxide comes into contact with UV light it generates hydroxyl radicals and superoxide ions on its surface which act as oxidizing agents of ethylene.83 It has been demonstrated that ethylene could be completely oxidized into CO2 on a powdered TiO2 surface.89 Previous studies also showed that ethylene could be completely eliminated from tomato using TiO2-coated glass beads.90 Besides its advantages, some limitations such as persistent exposure of fruit to TiO2 agglomeration decreases the ethylene degradation efficiency and hence leads to excess emission of ethylene.91
This is channeled by the illumination of the catalyst with ultraviolet radiation (UV-A, around 380 nm) which consequently results in generation of reactive oxygen species such as hydroxyl and superoxide radicals (OH− and O2−) in the gaseous phase.80,92 Further, these free radicals oxidize ethylene to produce water and carbon dioxide.
TiO2 is hailed as a popular photocatalyst for ethylene degradation due to its distinct photochemical reactivity, high refractive index and resistance to colour change.92 The embedding of TiO2 in polypropylene film used for packaging tomatoes enhanced keeping quality.93 Similarly, nano-composites of TiO2/SiO2 showed significant improvement in enhancing the shelf life of tomato fruit by ethylene scavenging action.89 However, the ethylene degradation rate depends on the best possible process parameters, system design and proper holding material. Wei et al.92 reported that photocatalysis using TiO2 is an effective, clean and low-cost technology with potential for lowering postharvest losses. Kim et al.82 also observed that shelf life of fruit can be further enhanced by using both zeolite adsorbents and photocatalytic oxidation. Yuan and Li94 studied the effects of zeolite and KMnO4 ethylene scrubbers on banana fruit. However, the drawbacks are low efficiency, possible hazardous effects of ultraviolet radiation on fruit quality, formation of toxic intermediates to human health, easy deactivation and deterring electron–hole coupling.80,81,83
Besides, several low temperature catalysts have been employed as a promising tool to remove volatile organic compounds. Several metals such as Pt, Au, Ag and Ti have been used by researchers.73 Moreover, Wei et al.95 found that Pt/CeO2–O showed the highest low temperature C2H4 catalytic oxidation activity. In a separate study, it was noticed that CeO2-octahedron-supported Pt catalysts could be a better tool for catalytic oxidation of ethylene at low temperature. Wei et al.95 observed that a Pt/plasma-modified CeO2 catalyst (Pt/CeO2-P) exhibited significantly higher catalytic reactivity of ethylene oxidation at low temperature compared to Pt/pristine CeO2.
4.5. Use of action inhibitors
4.5.1. 1-Methylcyclopropene (1-MCP).
In 1973, Sisler and Pian reported that some olefin compounds (cyclopropenes) inhibit ethylene action which can be used for delaying the ripening and deterioration of fresh horticultural commodities by inhibition of ethylene perception. 1-MCP is a derivative of DACP (diazocyclopentadiene) products having biological use due to their lower volatility over cyclopropene. 1-Methylcyclopropene (C4H6) was identified for countering ethylene action in 1994, followed by its patenting by Sisler and Blankenship in 1996. It is a gas at standard temperature and pressure with a molecular weight of 54 which covalently binds with ethylene (C2H4) receptors at comparatively ten-times higher affinity levels.96,97 1-MCP is a very effective molecule even at very low concentrations due to its ten-fold higher affinity for ethylene binding receptors.97 The pioneering work for identifying 1-MCP as a potent ethylene inhibiting agent and its miraculous possibilities in extending the postharvest life of fruit and vegetables, especially climacteric ones, was done and holds the patent for its use to prevent ethylene action.98 The binding of 1-MCP with γ-cyclodextrin gives a stable powder which readily releases 1-MCP as gas when dissolved with water. This ensured ease in providing fumigation treatment and thus gave a significant advancement for commercialization of this technology. The action of 1-MCP is due to its binding with the ethylene receptors, thus blocking ethylene action which results in delayed, suppressed or in some cases complete inhibition of ripening in such fruit. It has been found useful for lowering physiological disorder and rotting incidence and severity.12,99 Ding et al.100 reported that 1-MCP blocked AFS1 enzyme transcription by blocking α-farnesene synthesis, and thus lowering superficial scald incidence. Currently, the general notion is that 1-MCP binds permanently to receptors, and ripening at later stages as a result of ethylene action is due to development of new receptors. The binding of 1-MCP to the receptors results in suppression of ethylene biosynthetic enzymes such as ACC synthase and ACC oxidase. Besides, expression of genes associated with ethylene signaling pathways such as ETR, CTR, ERF, etc. has also been reported to be significantly inhibited.101 It has been reported to be more effective at temperatures ranging between 20 and 25 °C and 12–24 h treatment exposure has been reported to be adequate to elicit complete response and inhibit ripening.
It is available commercially under several trade names such as SmartFresh™, EthylBlock™ and Fysium® which have 1-MCP as an active ingredient often in the form of cyclodextrin powders.102 1-MCP was included in Annexure I of Directive 91/414/EEC/2005 by the European Union thereby granting permission for use on commercially important climacteric fruit like apples and pears.103 The Acute Acceptable Operator Exposure Level (AOEL/AAOEL) is noted at 0.12 mg kg−1. The Acceptable Daily Intake (ADI) was increased to 0.02 mg per kg body weight over an earlier bar set at 0.0009 mg per kg body weight per day.104
The main advantages of using 1-MCP are its high effectiveness at very low concentrations, maintenance of firmness and quality even after removal from cold storage, and absence of hazardous or any other residues. The efficacy of 1-MCP depends on several factors such as species, cultivar, harvest stage, time of postharvest treatments, concentration, temperature, application methods and storage conditions.105–109 Pirrung et al.110 noted that the affinity of 1-MCP towards receptors is associated with chemical properties such as its strain, pyramidalization and enantiomeric selectivity.
Watkins111 has noted 1-MCP as the most significant and practical postharvest innovation in the last decade for inhibiting ethylene action. Notably, it is so effective that the “evergreen/stay green effect” due to irreversible, uneven ripening, less juiciness and/or lack of development of aroma volatiles such as alcohols and esters and incomplete sensory development have been observed in some crops like banana, pear, immature green tomato and guava. This consequently leads to hampering the commercial usage as the consumer demand wavers with non-uniform colour development, partial aroma volatiles or incomplete sensorial development. This further prompted research to induce proper ripening and revival of ethylene perception in fruit for ensuing final ripening.106,112–118 Some recent efforts in ethylene management using 1-MCP are listed in Table 5. Thompson et al.119 estimated that pre-storage 1-MCP treatment could lower the cost of fresh produce from $8.10 to $5.25 per ton by reducing the energy consumption and further meeting the challenges of reducing the CO2 output and cost.
Table 5 Recent efforts in ethylene management by using 1-methylcyclopropene (1-MCP)
Commodity- cultivar |
Treatments and dose |
Major research output |
Ref. |
Mango – Tainong |
1-MCP (1 μL L−1) |
1-MCP treatment-maintained quality, delayed firmness, colour change (pulp and peel both), respiration and ethylene evolution rates, lowered cell wall enzyme activities, and weight loss while increasing antioxidant enzyme activity |
116
|
Mango – Tainong 1 |
1-MCP (1 μL L−1) |
Delayed ripening, firmness and maintained acidity at room temperature |
117
|
Melon – Donatello |
Gaseous 1-MCP (650 nL L−1) and 1-MCP microbubbles for 10, 20 and 30 min |
1-MCP microbubbles (20 m) was effective over gaseous 1-MCP treatment |
180
|
New Queen melon – Xinmi No. 13 |
CaCl2 (0.045, 0.09, 0.18 and 0.36 mol L−1) for 25 min, along with 1-MCP fumigation (1.25, 0.5, 1.0, 2.0 μL L−1) at 20 °C for 24 hours |
The respiration rate, ethylene release and activities and gene expression of polygalacturonase, pectin methyl esterase and pectatelyase were significantly reduced in melons treated with 0.18 mol L−1 CaCl2 + 1 μL L−1 1-MCP |
106
|
Papaya- Golden |
Ethylene and 1-MCP |
Combined application retained firmness and chlorophyll content |
105
|
Pear – Blanquilla |
CA + 1-MCP |
1-MCP treated fruit completely inhibited ethylene production and fruit softening. The superficial scald disorder was totally controlled in fruit treated with 1-MCP |
113
|
Pear – D'Anjou |
1-MCP |
1-MCP inhibited ethylene production, respiration rate and scald development |
181
|
Pear – Bartlett and D'Anjou |
1-MCP (320 μL L−1) applied during pre-harvest stage |
Ethylene evolution, respiration, and disorder incidence were lowered, higher retention of flavonoids and antioxidants |
116
|
Pear – Nanguo |
1-MCP (0.5 μL L−1) |
Alleviated peel browning, maintained higher firmness, glutathione content, ATP concentration and energy charge value while lowering ethylene evolution, respiration rate, electrolyte leakage and malondialdehyde content in ‘Nanguo’ pear |
114
|
Pear – Laiyang |
1-MCP (fumigation with 0.5 μL L−1 1-MCP at 20 ± 1 °C for 12 h in sealed container) |
1-MCP treated fruit-maintained fruit firmness, lowered respiration and ethylene production and maintained storage quality of pear |
115
|
Pear – Huangguan |
1-MCP (1.0 μL L−1) |
1-MCP treatment lowered browning, malondialdehyde accumulation, electrolyte leakage, hydrogen peroxide content, PPO enzyme activity and enhanced total phenol, flavonoids, ascorbate peroxidase and phenylalanine ammonia lyase activities |
12
|
Pear – Wonhwang |
1-MCP (1 μL L−1) |
Higher firmness, delayed colour change, cortex and core browning |
108
|
Pear – Comice |
1-MCP (0.15 μL L−1, 0.3 μL L−1) |
Ethylene evolution and respiration were significantly inhibited by 1-MCP treatments |
107
|
Pecan |
1-MCP (1.0 μL L−1) |
1-MCP treated unshelled pecans-maintained luminosity and showed lower internal browning. 1-MCP treated pecans showed lower acidity, peroxide value and unwanted volatiles |
182
|
4.6. Use of plant signaling molecules
There are several signaling molecules that play a regulatory role in the biosynthesis and perception of ethylene in the plant system (Table 6). Association of these biomolecules like polyamines, salicylic acid, and nitric oxide with signaling responses triggered by ethylene led to interest among researchers to modulate postharvest ethylene responses in fruit by its exogenous application.120–123
Table 6 Effects of various treatments on ethylene evolution and shelf life of fruit during storage
Postharvest treatments |
Crop |
Major findings |
Ref. |
Hot water treatment (HWT)
|
HWT at 50 °C for 5 min + ethylene vapour pad |
Lime |
Ethylene production reduced up to 15 days and extended shelf life without impairing quality |
48
|
HWT (50 ± 1 °C) for 10 min |
Mango |
Reduced ethylene production at low temp storage as well as room temp with elevated catalase activity |
198
|
HWT at 50 °C, for 2 min |
Mandarin |
Suppressed ethylene evolution, decreased chilling injury, maintained high CAT activity |
199
|
HWT at 40 °C for 20 min |
Tomato |
Delayed ethylene, reduced chilling injury and extended shelf life about 3 days more than the control |
200
|
![[thin space (1/6-em)]](https://www.rsc.org/images/entities/char_2009.gif) |
Gamma irradiation
|
Gamma irradiation doses (0, 0.4, and 1.0 kGy) |
Jujube |
Extended storage life of jujube with higher nutritional value |
201
|
0.5, 1.0 or 2.0 kGy |
Raspberry |
Did not alter ethylene production but extended storage life by 8 days |
202
|
Gamma irradiation (0.08 kGy) |
Papaya |
Enhanced storage up to 13 days with reduced ripening rate |
203
|
![[thin space (1/6-em)]](https://www.rsc.org/images/entities/char_2009.gif) |
KMnO
4
|
KMnO4 levels (0, 10, 20, 30, 40, and 50 g) |
Mango |
30 g KMnO4 significantly extended shelf life with maximum biochemical parameters |
204
|
KMnO4 (20 g) |
Papaya |
Reduced about 2.2% ethylene |
205
|
Nano zeolite-KMnO4 |
Banana |
Extended shelf life of banana up to 23 days |
206
|
KMnO4 (0, 2, 4, 6 and 8 g) |
Banana |
Maximum shelf life (13.50 days) was recorded in 6 g KMnO4 |
207
|
![[thin space (1/6-em)]](https://www.rsc.org/images/entities/char_2009.gif) |
Ozone
|
Ozone (0.3 μL L−1) |
Kiwifruit |
Ethylene biosynthesis due to inhibited adacs1 and adaco1 expression |
208
|
Ozone fumigation (0, 1.5, 2.5, 3.5 and 5.0 μL L−1) |
Papaya |
Ozone (<5.0 μL L−1) also potently delays the papaya ripening with lower respiration rate and ethylene production |
209
|
Ozone concentrations (100 μL L−1, 200 μL L−1, 300 μL L−1 |
Kiwifruit |
200 μL L−1 delayed fruit senescence, ethylene concentration at 1 °C |
210
|
0.05 μmol mol−1 |
Tomato |
Reduced the expression of ethylene biosynthesis related enzymes |
211
|
![[thin space (1/6-em)]](https://www.rsc.org/images/entities/char_2009.gif) |
1-MCP
|
Immersion treatment in 0, 10, 25 or 50 μg L−1 1-MCP |
Banana |
1-MCP (25 μg L−1) delayed ripening up to 12 days |
212
|
1-MCP treatment |
Pear |
Downregulated ripening related genes and reduced enzymatic activity |
213
|
1-MCP treatment (0.14%) |
Apricot |
Slowed down the fruit senescence process and favored antioxidant activity |
214
|
1-MCP (500 ppb) |
Guava |
Reduced ethylene (5.02 μL kg−1 h) and extended shelf life |
215
|
1-Methylcyclopropene (1-MCP) (500 nl L−1) |
Mango |
Delayed the skin and flesh colour development with delayed ethylene formation |
216
|
![[thin space (1/6-em)]](https://www.rsc.org/images/entities/char_2009.gif) |
Polyamines
|
Spermidine (spd) |
Apricot |
Spd reduced ACC synthase and ACC oxidase activity. Reduced ethylene production |
127
|
Putrescine, spermidine and spermine 1 and 10 mm |
Kiwifruit |
Putrescine at 1 mM suppressed ethylene production |
217
|
Spermine (0.5, 1.0 and 1.5) mm] and spermidine (1.0, 1.5 and 2.0) mm |
Kiwifruit |
Spermine at 1.5 mM and spermidine at 2.0 mM showed extended shelf life and reduced ethylene amount |
218
|
Putrescine (1 mM) |
Plum |
Exogenous putrescine inhibited and delayed ethylene and CO2 production rates |
219
|
![[thin space (1/6-em)]](https://www.rsc.org/images/entities/char_2009.gif) |
Salicylic acid (SA) and NO
2
|
SA (0.5, 1.0, 1.5, 2.0, 2.5 mM) |
Plum |
Delayed the onset of the climacteric peak of respiration and inhibited the ethylene |
121
|
SA (0.2–1.2 mM) |
Tomato |
SA treated (1.2 mM) fruits exhibited maximum shelf life (32.75 days) |
134
|
SA (1, 2, 3 and 4 mmol L−1) |
Plum |
Delayed ripening with enhancing antioxidants |
122
|
SA at 0.4, 0.8 and 1.2 mmol L−1 |
Sugar apple |
Delay in the fruits ripening process was achieved after 10 days of storage |
144
|
Sodium nitro prusside (SNP) (1.0, 5.0 and 10.0 μmoL l−1) |
Strawberry |
Inhibited ethylene production, activity of ACC synthase |
150
|
SNP at 0.5 mM, 1.0 mM, 1.5 mM, and 2.0 mM |
Custard apple |
SNP (2.0 mM) extended the shelf life with maintain bioactive compounds |
151
|
SNP (0.5, 1.0, and 1.5 mM |
Guava |
SNP (1.0 mM) delayed ripening and senescence with minimum weight loss, MDA content and decay |
126
|
Sodium nitroprusside (at 1.0 mM or 1.5 mM) |
Mango |
Enhanced shelf life by downregulating softening enzymes and delayed ripening |
123
|
![[thin space (1/6-em)]](https://www.rsc.org/images/entities/char_2009.gif) |
Novel phytohormones
|
Brassinosteroids (BRs) |
Pear |
Suppressed fruit ripening via delaying ethylene biosynthesis |
220
|
24-Epibrassionolide (EBR) 5 μM |
Jujube |
Delayed senescence by reducing ethylene production |
152
|
24-Epibrassionolide (EBR) (0.25, 0.5, 0.75, and 1 mg L−1 |
Blueberry |
EBR (0.75 mg L−1) significantly delayed senescence and enhanced shelf life with maximum quality |
153
|
Melatonin (1 mmol L−1) |
Apple |
MT treated apples reduced ethylene production (28 days–56 d) by decreased expressions of MdACO1, MdACS1, MdAP2.4 and MdERF109 |
17
|
MT solution (100 μM) |
Pear |
Ethylene biosynthesis genes PcACS1 and PcACO1 completely inhibited by the MT and delayed the senescence and ripening process |
155
|
MT (0.5 mM) |
Mango |
Delayed ethylene biosynthesis and reduced ethylene production |
221
|
![[thin space (1/6-em)]](https://www.rsc.org/images/entities/char_2009.gif) |
Edible coatings
|
Chitosan/alginate based layer bilayer coating |
Japanese pear |
Reduced ethylene with preservation of food value |
222
|
Carboxymethyl cellulose (CMC) (1%), guar gum (GG) (2%) and gum acacia (GA) (2%) |
Mango |
Delayed senescence and maintained antioxidants properties with better shelf life |
156
|
Chitosan (CH) and guar gum (GG) based composite edible coating enriched with different starch |
Kinnow |
GG + TAM coating enhanced the shelf life of kinnow fruits without losing desirable traits |
223
|
4.6.1. Polyamines.
Polyamines (PAs) are aliphatic nitrogen containing polycations of low molecular weight. The major plant polyamines are putrescine (diamine), spermidine (triamine) and spermine (tetramine). They have been known to play a significant role in the regulation of ripening and senescence due to the contention for the common limited precursor S-adenosyl methionine (SAM).1,16,124 Moreover, endogenous polyamine levels have been observed to rise in response to injury levels. PAs have been hailed as anti-ethylene and anti-stress molecules. The polycationic nature of polyamines at cellular pH helps in stabilizing cell membranes and thus lowers lipid peroxidation. These are contained mainly in cell vacuoles.125 Several studies have reported the down-regulation of ethylene biosynthetic enzymes, stabilization of cell membranes, higher antioxidant capacity, lowered chilling injury and severity of physiological disorders and delayed senescence. Sahu et al.126 reported that guava fruits treated with spermine (1.5 mM) preserved biochemical composition and extended shelf life by 10 days. Similarly, Wang et al.127 observed that spermidine treated apricot fruits delayed ethylene production by reducing ACC synthase and ACC oxidase activity.
Some recent studies related to the effects of different polyamine treatments are presented in Table 7.
Table 7 Recent efforts in ethylene management by using polyaminesa
Commodity- cultivar |
Treatments and methodology |
Major research output |
Ref. |
Abbreviations. – [PUT-putrescine, SPD-spermidine and SPM-spermine].
|
Carambola – B10 |
Putrescine and spermidine (SPD) (0.1, 1.0 and 2.0 mM) |
PUT (2.0 mM) reduced weight loss, delayed peel colour change and firmness loss |
189
|
Apricot – Saimaiti |
0.1 mmol L−1 SPD, 0.1 mmol L−1D-arginine |
SPD treated apricot exhibited higher firmness, lowered ACC synthase and ACC oxidase activity, inhibition of relative gene expression of PaACS2 and PaACO, ethylene production and delayed senescence |
127
|
Mandarin – Murcott |
Salicylic acid (SA- 200 and 400 ppm) and PUT (50 and 100 ppm) |
SA (400 ppm) delayed weight loss and decay-maintained fruit firmness, total soluble solids (TSS), acidity, and ascorbic acid during storage |
190
|
Tomato – Pusa Ruby |
A. tumefaciens having recombinant plasmid pBin2A11ADC was used to transform cotyledonary explants. Such tomato fruits amassed PAs by over-expression of arginine decarboxylase (ADC) gene. Fully developed transformants were grown under transgenic green-house and nethouse growth conditions |
The AsADC over expressed transgenic tomatoes showed higher PUT, SPD and SPM content over control. These fruits showed better shelf-life due to decline in ethylene production and respiration rates |
40
|
Mango – Langra |
Chitosan solutions (0.5%, 1%, and 2%) and SPD (0, 0.5, 1, and 2 mM) |
Chitosan (2%) and SPD (2 mM) treated mangoes showed delay in weight and firmness loss, and rotting |
191
|
Peach – Florda king cultivar grafted on Peshawar local |
Aqueous solution of PUT (0, 1, 2 and 3 mM) |
PUT (2 mM) treatment lowered chilling injury, fruit softening, weight loss, sugars, acidity, vitamin C and delayed colour changes |
192
|
4.6.2. Salicylic acid.
Salicylic acid (SA) belongs to the group of phenolic compounds, is an endogenous signal molecule, and plays a crucial role in different plant physiological processes and stress responses. It is present in plant either as free phenolic acid or as a conjugate form that is produced by glucosylation, methylation or hydroxylation of the aromatic ring.128,129 The synthesis of SA occurs in plants via two pathways, in which chorismate is used as a primary metabolite. In one pathway, chorismate is converted to isochorismate by isochorismate synthase that is further catalyzed by isochrismate pyruvate lyase to salicylic acid. In another pathway, chorismate is converted to L-phenylalanine, which is later converted to cinnamic acid by phenylalanine ammonia lyase. Finally, salicylic acid is produced through a series of enzymatic reactions including synthesis of benzoate intermediates.130–132 Salicylic acid received attention from researchers due to its role in imparting resistance to plants against various biotic and abiotic factors.133 Later, investigation on harvested horticultural commodities revealed that SA also plays a regulatory role in delaying ripening by modulating ethylene production.121,134 Salicylic acid has been reported to interfere with the biosynthesis and action of ethylene in plants.135,136 It has been demonstrated that exogenous application of SA and acetyl salicylic acid (ASA) suppresses the activity of ACC synthase and ACC oxidase, thereby inhibiting ethylene production in fruit.137,138 Moreover, SA also suppresses the activity of lipoxygenase and generation of reactive oxygen species.139 This is also linked with reduced production of ethylene in fruit.140–143 Some recent studies related to the effects of salicylic acid treatments are presented in Tables 8 and 6. Mo et al.144 reported that pre-storage of SA delayed the ripening process in sugar apple. In case of tomato Mandal et al.134 found that SA treated (1.2 mM) fruits exhibited an extended shelf life (32.75 days).
Table 8 Recent efforts in ethylene management using salicylic acid and nitric oxide
Commodity- cultivar |
Treatments and methodology |
Major research output |
Ref. |
Peach – Jinqiuhongmi |
SA (1 μmol L−1) |
Reduced ethylene production, increased sucrose content and reduced chilling injury incidence |
193
|
Tomato – Hisar Arun, BSS-488 |
Immersion treatment with salicylic acid |
Delayed loss of firmness, weight loss, lycopene content and loss of TSS |
194
|
Jamun |
SA (1.0 mM and 1.5 mM) |
The treatment reduced weight loss, decay loss, delayed senescence and preserved higher anthocyanins and antioxidant capacity |
195
|
Blueberry – Powderblue |
Spray with 1.0 mmol L−1 salicylic acid |
SA treatment delayed fruit softening and deterioration |
196
|
Pistachionut cv. Ahmad Aghaei |
Fruit sprayed with 15, 30, 45 or 60 μM SNP (a NO donor) |
Nitric oxide treated fruit had reduced activity of polyphenol oxidase, phenylalanine ammonia lyase and peroxidase and had the maximum activity of superoxide dismutase |
197
|
Guava – Lucknow 49 |
SNP (0.5, 1.0-, and 1.5 mM) |
1.0 mM SNP treated fruit had lower physical quality loss, delayed loss of chlorophyll, ascorbic acid, phenolics, and flavonoids |
126
|
4.6.3. Nitric oxide.
Nitric oxide (NO) is a highly diffusible, membrane permeable signaling molecule that plays a multifaceted role in various plant physiological processes involving growth, development and responses to stress. Among the different effects of NO in plants, its role in fruit ripening via modulation of ethylene biosynthesis has become a matter of research interest among many scientists. Another landmark research by Leshem and Haramaty145 reports the antagonistic effect of NO on ethylene in growing plants. Inhibition of ethylene by NO involves two mechanisms, through direct stochiometric inhibition and suppressing activity of ethylene biosynthesis enzymes. NO suppresses autocatalytic ethylene production by binding with ACC oxidase and formation of an ACC oxidase–NO complex, which further combines with ACC to form stable ternary complex ACC–ACC oxidase–NO that reduces ethylene production.146 Moreover, reaction between NO and reactive oxygen species produces peroxynitrites that affect the co-factors required for catalysis of ACC by ACC synthase and ACC oxidase. Another possible mechanism of ethylene regulation by NO involves regulation of hydrogen peroxide that affects MAP kinase mediated downstream components of ethylene biosynthesis.147 In addition, inhibition of SAM turnover by S-nitrosylation of transcriptionally produced methionine adenosyl transferase (MAT) also suppresses biosynthesis of ethylene.148 Some recent studies related to the effects of different polyamine treatments are presented in Tables 8 and 6. Additionally, application of NO gas in fruit and vegetables has been considered as safe also.149 Sodium nitroprusside (SNP) is the most common commercial source of NO applied exogenously.123,150,151 Pre-storage application of SNP (1.0 mM or 1.5 mM) significantly delayed ethylene production in mango.123 Likewise, Sahu et al.126 reported in guava that SNP (1.0 mM) treatment significantly delayed the ripening and senescence and preserved the nutritional composition of the fruits during storage. In another study, Ghasil et al.151 revealed that SNP treated sugar apple fruits exhibited better nutritional properties with maximum shelf life.
4.6.4. Novel phytohormones.
In the past couple of years, use of melatonin and brassinosteroids showed their significant impacts on altering ethylene production. Previous studies on jujube and blueberry have shown delayed ethylene production during storage (Table 6).152,153 Exogenous application of melatonin has been found to be crucial in delaying ethylene production through decreased expression of MdACO1, MdACS1, MdAP2.4 and MdERF10 genes in apple.17 Moreover, Medina-Santamarina et al.154 reported delayed ethylene evolution in melatonin treated cherimoya fruits. In case of pear, ethylene biosynthesis genes PcACS1 and PcACO1 were significantly downregulated by the exogenous melatonin treatments and enhanced the shelf life.155 Likewise, application of brassinazole significantly delayed ripening and senescence by suppressing biosynthesis genes in tomato.14
4.7. Edible coatings
Edible coatings create a semipermeable membrane over the fruit surface, thus altering the physiology of the fruits. Several starch-based coatings such as carboxymethyl cellulose, guar gum, gum arabic, sago starch, chitosan, etc. have been reported to be fruitful in delaying ethylene content in fruits during storage.156,157 Nowadays, edible coatings based on polysaccharides are gaining commercial importance and have been found to be highly effective in extending shelf life (Table 6). In peach and plum fruits, Guillén et al.158 found that an aloe vera coating can significantly delay the ethylene evolution, thus reducing the harmful effects of ethylene on the fruits. Zapata et al.159 found delayed ethylene formation in tomato fruits during storage when coated with alginate and zein based coating materials.
4.8. Use of packaging technology
The postharvest quality is influenced mainly by storage temperature, relative humidity and gaseous composition.160,161 An ideal packaging needs to be tailored to the specific horticultural produce as they vary largely in respiration and ethylene production rates, with varying sensitivities to ethylene and CO2. Active packaging films with modified permeability have been developed with addition of zeolite and montmorillonite mineral powders and bromine type inorganic chemicals for ethylene scrubbing.78,92,162 The ethylene is held by physical adsorption and bound to activated carbon or zeolite structure by van der Waals force.163 The functionality is based on the removal of ethylene by absorption as well as by allowing higher diffusion rates outside. This reaction is aided by moisture released by transpiration and respiration of fresh commodities. The commercially available ethylene scrubbing films are mostly based on zeolite mineral due to its highly porous 3D structure with high adsorption. Ethylene scavenging using zeolite is being frequently used at the domestic scale in refrigerators with the aim of extending the keeping quality of fresh fruit and vegetables. However, the use of packaging films is limited by the film opacity and limited efficiency due to lower mineral concentration, decline in efficacy with the increase in saturation, incomplete decomposition, possible escape of ethylene and need for regular replacement.78,92 Zeolite incorporated with finely dispersed palladium was found more efficacious over KMnO4 based zeolite at lower concentrations.164 The use of a platinum catalyst heated to 250 °C and ultraviolet radiation (184 and 254 nm) to form reactive oxygen species (ROS) and oxidize ethylene to CO2 and H2O has also been reported. The use of different ethylene scrubbing agents depends on scavenging potential, degree, possible hazardous intermediates and/or final derivatives, mechanism of action and possible migration. In addition, cost, safety and acceptance among buyers are of paramount importance.
4.9. Use of storage technology
Effective removal of ethylene from the storage atmosphere by ventilation, getting rid of exhaust gases from combustion sources and eliminating rotten and over-ripened fruit is a simple way to exclude the detrimental senescence effects of ethylene. Low temperature in the storage atmosphere helps in reducing the respiration and ethylene evolution rates. Moreover, it also reduces the sensitivity of fresh produce towards exogenous ethylene sources. Carbon dioxide prevents ethylene action, thus delaying senescence responses. However, the CO2 inhibitory action engages till ethylene levels do not exceed 1 μL L−1.165 It works by displacing ethylene at the binding sites and inhibiting the ACC oxidase activity. Controlled atmosphere storage eliminates ethylene gas build up by enhancing CO2 concentration, thereby initiating competition for ethylene receptors. The elevated level of CO2 and lower level of oxygen with other gaseous compositions may be helpful in delaying ethylene biosynthesis and action of ethylene.166 Recently, dynamic controlled atmosphere (DCA) storage has come up which involves the reduction of oxygen to the lowest endured level by the fruit to the anaerobic compensation point. This has potential in extending the shelf life of fresh produce beyond modified atmosphere and controlled atmosphere storages.
Hypobaric storage results in ethylene elimination from the storage environment and lowers internal ethylene concentration. It is effective in reducing the respiration rate to a minimum due to the lowered partial pressure of oxygen.9
Several scrubber systems to remove ethylene in storage using activated charcoal, ozone and catalysts such as platinum have been patented and marketed. These may be portable or fixed to scrub ethylene and provide fresh treated air circulation. These generally use a swing system to first adsorb and oxidize ethylene gas from the storage, followed by its release by ventilation at higher temperature or movement of fresh air. Hydrated aluminium silicate or aluminium calcium silicate is used in such systems. The devices based on these technologies are marketed using the trade names “Swingtherm” and “Swingcat”.
4.10. Use of bio-filtration and microbes
In 1998, Elsgaard reported the pioneering studies on removal of ethylene, known as biofiltration based on catalysts of biological origin. He reported adequate operational scrubbing and efficacy to remove ethylene.167,168 This was based on isolated ethylene-oxidizing bacteria (RD-4 strain) incorporated on peat-soil. The efficiency for ethylene scrubbing was found to be 98.4% at varied temperatures (5, 10 and 20 °C). Later, Kim169 observed that ethylene scavenging using Bacillus or Pseudomonas embedded with activated carbon could reach up to 100%. This technology may be used for removing the ethylene level in storage due to its high scavenging potential (minimum 0.017 μL L−1 level), stability and efficiency at low temperatures (0 to 10 °C). In addition to that, plant rhizosphere contains several microbiomes and consumes them with the help of feeding roots. This association of plants and microbes has the ability to modulate the ethylene signaling in plants.170,171
It has been reported that microbes can inhibit the ethylene production by producing ACC deaminase.171 The microbiome can potentially alter the stress in direct and indirect ways, thus reducing the level of ethylene. The microbial population containing ACC deaminase is of the same magnitude as the one resulting from knocking out the ACS gene.172,173 Nonetheless, the ethylene modulating microbes can transmit vertically from one generation to another, thus allowing coevolution of microbes and the host as a cohesive unit of selection.174
Conclusion
A perishable horticultural supply chain involves the movement of fruit and vegetables from the harvest point to the ultimate consumer through handling, transportation and storage. The likelihood to maintain postharvest quality with minimum losses and waste can be augmented by managing ethylene in the surrounding atmosphere. The effective, practical and inexpensive means are imperative to block ethylene synthesis and/or perception and its removal from packaging and storage. Use of biotechnological interventions significantly combat the problem of ethylene. CRISPR/Cas9 is a novel approach that could be utilized for gene modification. Likewise, biomolecules such as polyamines, 1-MCP, salicylic acid, NO2, ozone, etc. can be applied to avoid the deleterious effects of ethylene. In summary, comprehensive solutions ranging from biosynthesis to inhibition of its action including alteration by application of efficacious cocktail postharvest technologies are the need of the hour. This shall go a long way in minimizing postharvest losses and wastage and optimize the maintenance of quality of horticultural produce. There are limited studies on stress induced deleterious cellular changes responsible for inducing the appearance of new receptor sites for effective ripening colour and aroma development. Also, there are hardly any studies on the various specific ethylene induced ripening requirements of different varieties within a crop. There is a need to develop a convenient in-packaging ethylene delivery mechanism, low-cost portable real time ethylene measuring sensors and intelligent packaging for enhancing the marketability of perishable horticultural produce.
Conflicts of interest
There are no conflicts to declare.
References
- S. Pandey, S. A. Ranade, P. K. Nagar and N. Kumar, Role of polyamines and ethylene as modulators of plant senescence, J. Biosci., 2000, 25, 291–299 CrossRef CAS PubMed.
- B. T. Ho, P. J. Hofman, D. C. Joyce and B. R. Bhandari, Uses of an innovative ethylene-α-cyclodextrin inclusion complex powder for ripening of mango fruit, Postharvest Biol. Technol., 2016, 113, 77–86 CrossRef CAS.
- N. Iqbal, N. A. Khan, A. Ferrante, A. Trivellini, A. Francini and M. I. R. Khan, Ethylene role in plant growth, development and senescence: interaction with other phytohormones, Front. Plant Sci., 2017, 4(8), 475 Search PubMed.
- D. Martínez-Romero, G. Bailén, M. Serrano, F. Guillén, J. M. Valverde, P. Zapata, S. Castillo and D. Valero, Tools to maintain postharvest fruit and vegetable quality through the inhibition of ethylene action: a review, Crit. Rev. Food Sci. Nutr., 2007, 47, 543–560 CrossRef PubMed.
- V. Paul and R. Pandey, Role of internal atmosphere on fruit ripening and storability—a review, J. Food Sci. Technol., 2014, 51, 1223–1250 CrossRef CAS PubMed.
- S. D. T. Maduwanthi and R. A. U. J. Marapana, Induced ripening agents and their effect on fruit quality of banana, Int. J. Food Sci., 2019, 2019, 1–8 CrossRef PubMed.
- J. Morales, A. Tárrega, A. Salvador, P. Navarro and C. Besada, Impact of ethylene degreening treatment on sensory properties and consumer response to citrus fruits, Food Res. Int., 2020, 127, 108641 CrossRef CAS PubMed.
- A. Ebrahimi, M. Zabihzadeh Khajavi, S. Ahmadi, A. M. Mortazavian, A. Abdolshahi, S. Rafiee and M. Farhoodi, Novel strategies to control ethylene in fruit and vegetables for extending their shelf life: a review, Int. J. Environ. Sci. Technol., 2022, 19, 4599–4610 CrossRef CAS.
-
S. P. Burg, Postharvest Physiology and Hypobaric Storage of Fresh Produce, CABI, 2004 Search PubMed.
- M. W. Ansari and N. Tuteja, Post-harvest quality risks by stress/ethylene: management to mitigate, Protoplasma, 2015, 252, 21–32 CrossRef CAS PubMed.
- B. Hu, D.-W. Sun, H. Pu and Q. Wei, Recent advances in detecting and regulating ethylene concentrations for shelf-life extension and maturity control of fruit: a review, Trends Food Sci. Technol., 2019, 91, 66–82 CrossRef CAS.
- F. Xu, K. Zhang and S. Liu, Evaluation of 1-methylcyclopropene (1-MCP) and low temperature conditioning (LTC) to control brown of Huangguan pears, Sci. Hortic., 2020, 259, 108738 CrossRef CAS.
- Y. Ito, A. Nishizawa-Yokoi, M. Endo, M. Mikami and S. Toki, CRISPR/Cas9-mediated mutagenesis of the RIN locus that regulates tomato fruit ripening, Biochem. Biophys. Res. Commun., 2015, 467, 76–82 CrossRef CAS PubMed.
- T. Zhu, W.-R. Tan, X.-G. Deng, T. Zheng, D.-W. Zhang and H.-H. Lin, Effects of brassinosteroids on quality attributes and ethylene synthesis in postharvest tomato fruit, Postharvest Biol. Technol., 2015, 100, 196–204 CrossRef CAS.
-
G. E. Schaller and B. M. Binder, Inhibitors of ethylene biosynthesis and signaling, in Methods in Molecular Biology, Humana Press Inc., 2017, pp. 223–235 Search PubMed.
-
S. Sharma, A. K. Singh, S. K. Singh, K. Barman and S. Kumar, Polyamines for preserving postharvest quality. In Emerging postharvest treatment of fruits and vegetables, ed. K. Barman, S. Sharma and M. W. Siddiqui, Apple Academic Press, New York, 1st edn, 2018, pp. 381-404 Search PubMed.
- J. C. Onik, S. C. Wai, A. Li, Q. Lin, Q. Sun, Z. Wang and Y. Duan, Melatonin treatment reduces ethylene production and maintains fruit quality in apple during postharvest storage, Food Chem., 2021, 337, 127753 CrossRef CAS PubMed.
-
W. G. Hopkins and N. P. A. Huner, Introduction to Plant Physiology, John Wiley & Sons, Inc., 4th edn, 2008 Search PubMed.
- S. Ravanel, B. Gakière, D. Job and R. Douce, The specific features of methionine biosynthesis and metabolism in plants, Proc. Natl. Acad. Sci, 1998, 95, 7805–7812 CrossRef CAS PubMed.
- S. F. Yang and N. E. Hoffman, Ethylene biosynthesis and its regulation in higher plants, Annu. Rev. Plant Physiol., 1984, 35, 155–189 CrossRef CAS.
- A. B. Bleecker and H. Kende, Ethylene: A gaseous signal molecule in plants, Annu. Rev. Cell Dev. Biol., 2000, 16, 1–18 CrossRef CAS PubMed.
- A. De Paepe and D. Van Der Straeten, Ethylene biosynthesis and signaling: an overview, Vitam. Horm., 2005, 72, 399–430 CrossRef CAS PubMed.
- P. K. Mehta, T. I. Hale and P. Christen, Aminotransferases: demonstration of homology and division into evolutionary subgroups, Eur. J. Biochem., 1993, 214, 549–561 CrossRef CAS PubMed.
- V. A. Bapat, P. K. Trivedi, A. Ghosh, V. A. Sane, T. R. Ganapathi and P. Nath, Ripening of fleshy fruit: molecular insight and the role of ethylene, Biotechnol. Adv., 2010, 28, 94–107 CrossRef CAS PubMed.
- M. Liu, J. Pirrello, C. Chervin, J.-P. Roustan and M. Bouzayen, Ethylene control of fruit ripening: revisiting the complex network of transcriptional regulation, Plant Physiol., 2015, 169(4), 2380–2390 CAS.
- J. Hua, H. Sakai, S. Nourizadeh, Q. G. Chen, A. B. Bleecker, J. R. Ecker and E. M. Meyerowitz, EIN4 and ERS2 are members of the putative ethylene receptor gene family in Arabidopsis, Plant Cell, 1998, 10, 1321–1332 CrossRef CAS PubMed.
- B. M. Binder, Ethylene signaling in plants, J. Biol. Chem., 2020, 295, 7710–7725 CrossRef CAS PubMed.
- M. Liu, G. Diretto, J. Pirrello, J. Roustan, Z. Li, G. Giuliano, F. Regad and M. Bouzayen, The chimeric repressor version of an Ethylene Response Factor (ERF) family member, Sl-ERF.B3, shows contrasting effects on tomato fruit ripening, New Phytol., 2014, 203, 206–218 CrossRef CAS PubMed.
- Y. Chen, J. Grimplet, K. David, S. D. Castellarin, J. Terol, D. C. J. Wong, Z. Luo, R. Schaffer, J.-M. Celton, M. Talon, G. A. Gambetta and C. Chervin, Ethylene receptors and related proteins in climacteric and non-climacteric fruits, Plant Sci., 2018, 276, 63–72 CrossRef CAS PubMed.
-
J. Gray, S. Picton, J. Shabbeer, W. Schuch and D. Grierson, in 10 Years Plant Molecular Biology, Springer, Dordrecht, Netherlands, 1992, pp. 69–87 Search PubMed.
- R. G. Fray and D. Grierson, Identification and genetic analysis of normal and mutant phytoene synthase genes of tomato by sequencing, complementation and co-suppression, Plant Mol. Biol., 1993, 22, 589–602 CrossRef CAS PubMed.
- P. W. Oeller, M.-W. Lu, L. P. Taylor, D. A. Pike and A. A. Theologis, Reversible inhibition of tomato fruit senescence by antisense RNA, Science, 1991, 254, 437–439 CrossRef CAS PubMed.
- A. J. Hamilton, G. W. Lycett and D. Grierson, Antisense gene that inhibits synthesis of the hormone ethylene in transgenic plants, Nature, 1990, 346, 284–287 CrossRef CAS.
- R. Ayub, M. Guis, M. Ben Amor, L. Gillot, J.-P. Roustan, A. Latché, M. Bouzayen and J.-C. Pech, Expression of ACC oxidase antisense gene inhibits ripening of cantaloupe melon fruits, Nat. Biotechnol., 1996, 14, 862–866 CrossRef CAS PubMed.
- A. Thayer, FDA gives go-ahead to bioengineered tomato, Chem. Eng. News, 1994, 72, 7–8 Search PubMed.
- M. Guis, R. Botondi, M. Ben-Amor, R. Ayub, M. Bouzayen, J.-C. Pech and A. Latché, Ripening-associated biochemical traits of Cantaloupe Charentais melons expressing an antisense ACC
oxidase transgene, J. Am. Soc. Hortic. Sci., 1997, 122, 748–751 CAS.
- J. A. Silva, T. S. da Costa, L. Lucchetta, L. J. Marini, M. R. Zanuzo, L. Nora, F. R. Nora, R. M. Twyman and C. V. Rombaldi, Characterization of ripening behavior in transgenic melons expressing an antisense 1-aminocyclopropane-1-carboxylate (ACC) oxidase gene from apple, Postharvest Biol. Technol., 2004, 32, 263–268 CrossRef CAS.
- H. G. Nuñez-Palenius, M. Gomez-Lim, N. Ochoa-Alejo, R. Grumet, G. Lester and D. J. Cantliffe, Melon fruits: genetic diversity, physiology, and biotechnology features, Crit. Rev. Biotechnol., 2008, 28, 13–55 CrossRef PubMed.
- H. J. Klee, M. B. Hayford, K. A. Kretzmer, G. F. Barry and G. M. Kishore, Control of ethylene synthesis by expression of a bacterial enzyme in transgenic tomato plants, Plant Cell, 1991, 3, 1187–1193 CAS.
- A. Gupta, R. Pandey, R. Sinha, A. Chowdhary, R. K. Pal and M. V. Rajam, Improvement of post-harvest fruit characteristics in tomato by fruit-specific over-expression of oat arginine decarboxylase gene, Plant Growth Regul., 2019, 88, 61–71 CrossRef CAS.
- S. Nambeesan, T. Datsenka, M. G. Ferruzzi, A. Malladi, A. K. Mattoo and A. K. Handa, Overexpression of yeast spermidine synthase impacts ripening, senescence and decay symptoms in tomato, Plant J., 2010, 63, 836–847 CrossRef CAS PubMed.
- P. Madhulatha, A. Gupta, S. Gupta, A. Kumar, R. K. Pal and M. V. Rajam, Fruit-specific over-expression of human S-adenosylmethionine decarboxylase gene results in polyamine accumulation and affects diverse aspects of tomato fruit development and quality, J. Plant Biochem. Biotechnol., 2014, 23, 151–160 CrossRef CAS.
- N. Yokotani, R. Nakano, S. Imanishi, M. Nagata, A. Inaba and Y. Kubo, Ripening-associated ethylene biosynthesis in tomato fruit is autocatalytically and developmentally regulated, J. Exp. Bot., 2009, 60, 3433–3442 CrossRef CAS PubMed.
-
V. R. Bommineni, H. Mathews, S. K. Clendennen, W. Wagoner, V. Dewey, J. Kellogg, S. Peters, W. Matsumura, W. Pieper, M. Kramer and D. R. Wagner, Genetic engineering of fruits and vegetables with the ethylene control gene encoding S-adenosylmethionine hydrolase (SAMase), Plant genetic engi.: Towards the third millennium.(Arencibia, ADed.)Elsevier Science,BV, 2000, pp. 206–214 Search PubMed.
- N. Kaur, A. Alok, Shivani, N. Kaur, P. Pandey, P. Awasthi and S. Tiwari, CRISPR/Cas9-mediated efficient editing in phytoene desaturase (PDS) demonstrates precise manipulation in banana cv. Rasthali genome, Funct. Integr. Genomics, 2018, 18, 89–99 CrossRef CAS PubMed.
- Z. Wang, S. Wang, D. Li, Q. Zhang, L. Li, C. Zhong, Y. Liu and H. Huang, Optimized paired-sgRNA/Cas9 cloning and expression cassette triggers high-efficiency multiplex genome editing in kiwifruit, Plant Biotechnol. J., 2018, 16, 1424–1433 CrossRef CAS PubMed.
- P. Opio, P. Jitareerat, N. Pongprasert, C. Wongs-Aree, Y. Suzuki and V. Srilaong, Efficacy of hot water immersion on lime (Citrus auranifolia, Swingle cv. Paan) fruit packed with ethanol vapor in delaying chlorophyll catabolism, Sci. Hortic., 2017, 224, 258–264 CrossRef CAS.
- P. Opio, P. Jitareerat, N. Pongprasert, C. Wongs-Aree, Y. Suzuki and V. Srilaong, Efficacy of hot water immersion on lime (Citrus auranifolia, Swingle cv. Paan) fruit packed with ethanol vapor in delaying chlorophyll catabolism, Sci. Hortic., 2017, 224, 258–264 CrossRef CAS.
- H. Pu, S. Shan, Z. Wang, W. Duan, J. Tian, L. Zhang, J. Li, H. Song and X. Xu, Dynamic changes of DNA methylation induced by heat treatment
were involved in ethylene signal transmission and delayed the postharvest ripening of tomato fruit, J. Agric. Food Chem., 2020, 68, 8976–8986 CrossRef CAS PubMed.
- I. Hussain, A. Rab, N. U. Khan, M. Sajid, N. Ali, S. M. Khan and S. Ali, Effect of chilling exposure and vapor heat treatment duration on the quality of sweet orange during simulated marketing, Acta Sci. Pol. Hortorum Cultus, 2017, 16, 39–47 CrossRef.
-
V. K. Mishra and T. V. Gamage, in Handbook of Food Preservation, ed. M. S. Rahman, CRC Press, 3rd edn, 2020, pp. 45–60 Search PubMed.
- L. Jat and S. S. Lakhawat, Effect of gamma irradiation treatment at phytosanitary dose level on physiological and consumer traits of Indian ber. Part 1. Cultivar Umran, Radiat. Phys. Chem., 2021, 179, 109209 CrossRef CAS.
-
B. Nyakundi, Effect of low dose irradiation on expression of genes involved in ethylene biosynthesis of 'Granny Smith' apples, MS thesis, Chapman University DOI:10.36837/chapman.000146.
- M. K. Yadav, N. L. Patel and S. R. Patel, Effect of irradiation and storage temperature on quality parameters of Kesar mango (Mangifera indica L.), Indian J. Plant Physiol., 2013, 18, 313–317 CrossRef.
- P. Loaharanu and M. Ahmed, Advantages and disadvantages of the use of irradiation for food preservation, J. Agric. Environ. Ethics, 1991, 4, 14–30 CrossRef.
- S. N. T. Mohamed, P. Ding, J. Kadir and H. M. Ghazali, Potential of UVC germicidal irradiation in suppressing crown rot disease, retaining postharvest quality and antioxidant capacity of Musa AAA Berangan during fruit ripening, Food Sci. Nutr., 2017, 5, 967–980 CrossRef PubMed.
- J. Bu, Y. Yu, G. Aisikaer and T. Ying, Postharvest UV-C irradiation inhibits the production of ethylene and the activity of cell wall-degrading enzymes during softening of tomato (Lycopersicon esculentum L.) fruit, Postharvest Biol. Technol., 2013, 86, 337–345 CrossRef CAS.
- U. Prajapati, R. Asrey, E. Varghese, A. K. Singh and M. Pal Singh, Effects of postharvest ultraviolet-C treatment on shelf-life and quality of bitter gourd fruit during storage, Food Packag. Shelf Life, 2021, 28, 100665 CrossRef CAS.
- H. Lu, L. Li, J. Limwachiranon, J. Xie and Z. Luo, Effect of UV-C on ripening of tomato fruits in response to wound, Sci. Hortic., 2016, 213, 104–109 CrossRef CAS.
- P. Pristijono, J. Golding and M. Bowyer, Postharvest UV-C treatment, followed by storage in a continuous low-level ethylene atmosphere, maintains the quality of ‘Kensington pride’mango fruit stored at 20 °C, Horticulturae, 2018, 5, 1 CrossRef.
- J. A. Guerrero-Beltran and G. V. Barbosa-C’novas, Advantages and limitations on processing foods by UV light, Food Sci. Technol. Int., 2004, 10, 137–147 CrossRef.
- P. Promkaew, S. Kondo, N. Pongprasert, C. Wongs-Aree, S. Kaewsuksaeng and V. Srilaong, Application of AVG or 1-MCP-MBs on postharvest quality of pummelo cv.“Tubtim Siam”(Citrus maxima Burm.), Food Appl. Biosci. J., 2019, 7, 55–71 Search PubMed.
- J. Streif, D. Kittemann, D. A. Neuwald, R. McCormick and H. Xuan, Pre-and post-harvest management of fruit quality, ripening and senescence, Acta Hortic., 2010, 55–68 CrossRef CAS.
- A. Guneyil, M. A. Koyuncu, C. E. Onursal, D. Erbas, M. Cetinbas, S. Butar and F. Koyuncu, Effects of pre-harvest retain treatments with map on cold storage quality of sweet cherry cv. 0900 Ziraat, Sci. Papers Ser. B Hortic., 2018, 153–158 Search PubMed.
- S. Lurie, Regulation of ethylene biosynthesis in fruits by aminoethoxyvinyl glycine and 1-methylcyclopropene, Acta Hortic., 2008, 31–41 CrossRef CAS.
- E. Bal, Effect of postharvest aminoethoxyvinylglycine (AVG) application on fruit quality of Asian pear (Pyrus pyrifolia cv. Hosui) during cold storage, Erwerbs-Obstbau, 2020, 62, 69–75 CrossRef.
- E. C. Sisler and M. Serek, Inhibitors of ethylene responses in plants at the receptor level: recent developments, Physiol. Plant., 1997, 100, 577–582 CrossRef CAS.
- X. Fan, S. M. Blankenship and J. P. Mattheis, 1-Methylcyclopropene inhibits apple ripening, J. Am. Soc. Hortic. Sci., 1999, 124, 690–695 CAS.
- K. Sadeghi, Y. Lee and J. Seo, Ethylene scavenging systems in packaging of fresh produce: A review, Food Rev. Int., 2021, 37, 155–176 CrossRef CAS.
- S. Mallakpour and E. Khadem, Recent development in the synthesis of polymer nanocomposites based on nano-alumina, Prog. Polym. Sci., 2015, 51, 74–93 CrossRef CAS.
- R. Ben-Mansour, M. A. Habib, O. E. Bamidele, M. Basha, N. A. A. Qasem, A. Peedikakkal, T. Laoui and M. Ali, Carbon capture by physical adsorption: materials, experimental investigations and numerical modeling and simulations – a review, Appl. Energy, 2016, 161, 225–255 CrossRef CAS.
- V. C. Wabali and A. Esiri, Effect of potassium permanganate on colour and textural characteristics of tomatoes at ambient temperature storage, European J. Agric. Food Sci., 2021, 3, 60–62 CrossRef.
- H. Wei, T. Wei, L. Li, T. Zhang, F. Seidi, Y. Jin and H. Xiao, Morphological effect of ceria-supported platinum catalyst on low-temperature ethylene oxidation, Appl. Catal., B, 2023, 324, 122242 CrossRef CAS.
- G. Awalgaonkar, R. Beaudry and E. Almenar, Ethylene-removing packaging: basis for development and latest advances, Compr. Rev. Food Sci. Food Saf., 2020, 19, 3980–4007 CrossRef PubMed.
- P. C. Spricigo, M. M. Foschini, C. Ribeiro, D. S. Corrêa and M. D. Ferreira, Nanoscaled platforms based on SiO2 and Al2O3 impregnated with potassium permanganate use color changes to indicate ethylene removal, Food Bioprocess Technol., 2017, 10, 1622–1630 CrossRef CAS.
- N. Keller, M.-N. Ducamp, D. Robert and V. Keller, Ethylene removal and fresh product storage: a challenge at the frontiers of chemistry. Toward an approach by photocatalytic oxidation, Chem. Rev., 2013, 113, 5029–5070 CrossRef CAS PubMed.
- R. B. H. Wills and M. A. Warton, Efficacy of potassium permanganate impregnated into alumina beads to reduce atmospheric ethylene, J. Am. Soc. Hortic. Sci., 2004, 129, 433–438 CAS.
- T. Janjarasskul and P. Suppakul, Active and intelligent packaging: the indication of quality and safety, Crit. Rev. Food Sci. Nutr., 2018, 58, 808–831 CrossRef PubMed.
- M. H. Álvarez-Hernández, F. Artés-Hernández, F. Ávalos-Belmontes, M. A. Castillo-Campohermoso, J. C. Contreras-Esquivel, J. M. Ventura-Sobrevilla and G. B. Martínez-Hernández, Current scenario of adsorbent materials used in ethylene scavenging systems to extend fruit and vegetable postharvest life, Food Bioprocess Technol., 2018, 11, 511–525 CrossRef.
-
N. Pathak, Photocatalysis and vacuum ultraviolet light photolysis as ethylene removal techniques for potential application in fruit storage, PhD thesis, Technischen Universität Berlin, 2019 DOI:10.14279/depositonce-8313.
- S. Yildirim, B. Röcker, M. K. Pettersen, J. Nilsen-Nygaard, Z. Ayhan, R. Rutkaite, T. Radusin, P. Suminska, B. Marcos and V. Coma, Active packaging applications for food, Compr. Rev. Food Sci. Food Saf., 2018, 17, 165–199 CrossRef PubMed.
- S. Kim, G. H. Jeong and S.-W. Kim, Ethylene gas decomposition using ZSM-5/WO3–Pt-Nanorod composites for fruit freshness, ACS Sustainable Chem. Eng., 2019, 7, 11250–11257 CrossRef CAS.
- N. Pathak, O. J. Caleb, M. Geyer, W. B. Herppich, C. Rauh and P. V. Mahajan, Photocatalytic and photochemical oxidation of ethylene: Potential for storage of fresh produce—A review, Food Bioprocess Technol., 2017, 10, 982–1001 CrossRef CAS.
- N. Tzortzakis and A. Chrysargyris, Postharvest ozone application for the preservation of fruits and vegetables, Food Rev. Int., 2017, 33, 270–315 CrossRef CAS.
-
J. L. Smilanick, in Washington Tree Fruit Postharvest Conference, 2003, pp. 1–10 Search PubMed.
- A. K. Mahapatra, K. Muthukumarappan and J. L. Julson, Applications of ozone, bacteriocins and irradiation in food processing: a review, Crit. Rev. Food Sci. Nutr., 2005, 45, 447–461 CrossRef CAS PubMed.
- I. S. Minas, A. R. Vicente, A. P. Dhanapal, G. A. Manganaris, V. Goulas, M. Vasilakakis, C. H. Crisosto and A. Molassiotis, Ozone-induced kiwifruit ripening delay is mediated by ethylene biosynthesis inhibition and cell wall dismantling regulation, Plant Sci., 2014, 229, 76–85 CrossRef CAS PubMed.
- L. Afsah-Hejri, P. Hajeb and R. J. Ehsani, Application of ozone for degradation of mycotoxins in food: a review, Compr. Rev. Food Sci. Food Saf., 2020, 19, 1777–1808 CrossRef CAS PubMed.
- M. L. V. de Chiara, S. Pal, A. Licciulli, M. L. Amodio and G. Colelli, Photocatalytic degradation of ethylene on mesoporous TiO2/SiO2 nanocomposites: effects on the ripening of mature green tomatoes, Biosyst. Eng., 2015, 132, 61–70 CrossRef.
- C. Maneerat, Y. Hayata, N. Egashira, K. Sakamoto, Z. Hamai and M. Kuroyanagi, Photocatalytic reaction of TiO2 to decompose ethylene in fruit and vegetable storage, Trans. ASAE, 2003, 46, 725–730 CAS.
- U. Siripatrawan and P. Kaewklin, Fabrication and characterization of chitosan–titanium dioxide nanocomposite film as ethylene scavenging and antimicrobial active food packaging, Food Hydrocolloids, 2018, 84, 125–134 CrossRef CAS.
- H. Wei, F. Seidi, T. Zhang, Y. Jin and H. Xiao, Ethylene scavengers for the preservation of fruits and vegetables: a review, Food Chem., 2021, 337, 127750 CrossRef CAS PubMed.
- C. Maneerat and Y. Hayata, Gas-Phase photocatalytic oxidation of ethylene with TiO2-coated packaging film for horticultural products, Trans. ASABE, 2008, 51, 163–168 CAS.
- R. Yuan and J. Li, Effect of sprayable 1-MCP, AVG, and NAA on ethylene biosynthesis, preharvest fruit drop, fruit maturity, and quality of delicious apples, Hort Sci., 2008, 43, 1454–1460 Search PubMed.
- H. Wei, L. Li, T. Zhang, F. Seidi, Q. Chen and H. Xiao, Surface-modified CeO2 -Octahedron-supported Pt nanoparticles as ethylene scavengers for fruit preservation, ACS Appl. Nano Mater., 2023, 6, 3738–3749 CrossRef CAS.
- E. C. Sisler, The discovery and development of compounds counteracting ethylene at the receptor level, Biotechnol. Adv., 2006, 24, 357–367 CrossRef CAS PubMed.
- S. M. Blankenship and J. M. Dole, 1-Methylcyclopropene: a review, Postharvest Biol. Technol., 2003, 28, 1–25 CrossRef CAS.
-
E. Sisler and M. Blankenship, Method of counteracting an ethylene response in plants, US Pat., 5518988, 1996 Search PubMed.
- X. Xie, T. Einhorn and Y. Wang, Inhibition of ethylene biosynthesis and associated gene expression by aminoethoxyvinylglycine and 1-Methylcyclopropene and their consequences on eating quality and internal browning of starkrimson pears, J. Am. Soc. Hortic. Sci., 2015, 140, 587–596 CAS.
- R. Ding, X. Che, H. Liu, B. Du, K. Dong and Y. Zhang, Effects of 1-MCP and storage temperature on transcription of mevalonate (MVA) enzyme genes of α-farnesene in White Winter Pearmain apples fruit, Sci. Hortic., 2020, 259, 108841 CrossRef CAS.
- M. Tatsuki, A. Endo and H. Ohkawa, Influence of time from harvest to 1-MCP treatment on apple fruit quality and expression of genes for ethylene biosynthesis enzymes and ethylene receptors, Postharvest Biol. Technol., 2007, 43, 28–35 CrossRef CAS.
- W. Rademacher, Plant growth regulators: Backgrounds and uses in plant production, J. Plant Growth Regul., 2015, 34, 845–872 CrossRef CAS.
- N. Falagán and L. Terry, 1-Methylcyclopropene maintains postharvest quality in Norwegian apple fruit, Food Sci. Technol. Int., 2020, 26, 420–429 CrossRef PubMed.
- M. Arena, D. Auteri, S. Barmaz, A. Brancato, D. Brocca, L. Bura, L. Carrasco Cabrera, A. Chiusolo, C. Civitella, D. Court Marques, F. Crivellente, L. Ctverackova, C. De Lentdecker, M. Egsmose, Z. Erdos, G. Fait, L. Ferreira, M. Goumenou, L. Greco, A. Ippolito, F. Istace, S. Jarrah, D. Kardassi, R. Leuschner, C. Lythgo, J. O. Magrans, P. Medina, D. Mineo, I. Miron, T. Molnar, L. Padovani, J. M. Parra Morte, R. Pedersen, H. Reich, C. Riemenschneider, A. Sacchi, M. Santos, R. Serafimova, R. Sharp, A. Stanek, F. Streissl, J. Sturma, C. Szentes, J. Tarazona, A. Terron, A. Theobald, B. Vagenende, J. Van Dijk and L. Villamar-Bouza, Peer review of the pesticide risk assessment of the active substance 1-methylcyclopropene, EFSA J., 2018, 16(7), 5308 Search PubMed.
- R. V. Façanha, P. C. Spricigo, E. Purgatto and A. P. Jacomino, Combined application of ethylene and 1-methylcyclopropene on ripening and volatile compound production of Golden papaya, Postharvest Biol. Technol., 2019, 151, 160–169 CrossRef.
- Q. Zhang, W. Dai, X. Jin and J. Li, Calcium chloride and 1-methylcyclopropene treatments delay postharvest and reduce decay of New Queen melon, Sci. Rep., 2019, 9, 13563 CrossRef PubMed.
- J. Zhao, X. Xie, S. Wang, H. Zhu, W. Dun, L. Zhang, Y. Wang and C. Fang, 1-Methylcyclopropene affects ethylene synthesis and chlorophyll degradation during cold storage of Comice pears, Sci. Hortic., 2020, 260, 108865 CrossRef CAS.
- H. P. Lwin, J.-H. Choi, J.-P. Chun, C. B. Watkins and J. Lee, 1-Methylcyclopropene treatment alters fruit quality attributes and targeted metabolites in Wonhwang pears during shelf life, Sci. Hortic., 2021, 284, 110125 CrossRef CAS.
- R. F. Satuor, M. M. Attia, H. A. Kassem and Y. S. Mostafa, Effect of postharvest aminoethoxyvinylglycine, 1-methylcyclopropene and jasmonic acid treatments on storability and quality maintenance of apricot fruit Cv. Canino, Alex. J. Agri. Sci., 2019, 64(1), 11–20 Search PubMed.
- M. C. Pirrung, A. B. Bleecker, Y. Inoue, F. I. Rodríguez, N. Sugawara, T. Wada, Y. Zou and B. M. Binder, Ethylene receptor antagonists: Strained alkenes are necessary but not sufficient, Chem. Biol., 2008, 15, 313–321 CrossRef CAS PubMed.
- C. B. Watkins, The use of 1-methylcyclopropene (1-MCP) on fruits and vegetables, Biotechnol. Adv., 2006, 24, 389–409 CrossRef CAS PubMed.
- L. Li, A. Lichter, D. Chalupowicz, D. Gamrasni, T. Goldberg, O. Nerya, R. Ben-Arie and R. Porat, Effects of the ethylene-action inhibitor 1-methylcyclopropene on postharvest quality of non-climacteric fruit crops, Postharvest Biol. Technol., 2016, 111, 322–329 CrossRef CAS.
- C. Larrigaudière, V. Lindo-García, D. Ubach and J. Giné-Bordonaba, 1-Methylcyclopropene and extreme ULO inhibit superficial scald in a different way highlighting the physiological basis of this disorder in pear, Sci. Hortic., 2019, 250, 148–153 CrossRef.
- D. Tao, J. Wang, L. Zhang, Y. Jiang and M. Lv, 1-Methylcyclopropene alleviates peel browning of Nanguo pears by regulating energy, antioxidant and lipid metabolisms after long term refrigeration, Sci. Hortic., 2019, 247, 254–263 CrossRef CAS.
- P. Shu, D. Min, J. Zhou, W. Ai, J. Li, Z. Li, X. Zhang, Z. Shi, Y. Sun, F. Li, X. Li and Y. Guo, The synergism of 1-methylcyclopropene and ethephon preserves quality of Laiyang pears with recovery of aroma formation after long-term cold storage, Front. Plant Sci., 2020, 25(11), 490 CrossRef PubMed.
- L. Li, C. Li, J. Sun, J. Sheng, Z. Zhou, M. Xin, P. Yi, X. He, F. Zheng, Y. Tang, J. Li and J. Tang, The effects of 1-methylcyclopropene in the regulation of antioxidative system and softening of mango fruit during storage, J. Food Qual., 2020, 2020, 1–11 Search PubMed.
- L. Li, L. Shuai, J. Sun, C. Li, P. Yi, Z. Zhou, X. He, D. Ling, J. Sheng, K. Kong, F. Zheng, J. Li, G. Liu, M. Xin, Z. Li and Y. Tang, The Role of 1-Methylcyclopropene in the regulation of ethylene biosynthesis and ethylene receptor gene expression in Mangifera indica L. (Mango Fruit), Food Sci. Nutr., 2020, 8, 1284–1294 CrossRef CAS PubMed.
- A. K. Baswal and A. Ramezanian, 1-methylcyclopropene potentials in maintaining the postharvest quality of fruits, vegetables, and ornamentals: a review, J. Food Process. Preserv., 2021, 45(1), e15129 CAS.
- J. F. Thompson, D. C. Mejia and R. P. Singh, Energy use of commercial forced-air coolers for fruit, Appl. Eng. Agric., 2010, 26, 919–924 Search PubMed.
- J. Jhalegar, R. R. Sharma and S. K. Singh, Effect of surface coatings on postharvest quality of Kinnow mandarin, Indian J. Hortic., 2015, 72, 267 CrossRef.
- Z. Luo, C. Chen and J. Xie, Effect of salicylic acid treatment on alleviating postharvest chilling injury of Qingnai plum fruit, Postharvest Biol. Technol., 2011, 62, 115–120 CrossRef CAS.
- G. H. Davarynejad, M. Zarei, M. E. Nasrabadi and E. Ardakani, Effects of salicylic acid and putrescine on storability, quality attributes and antioxidant activity of plum cv. Santa Rosa, J. Food Sci. Technol., 2015, 52, 2053–2062 CrossRef CAS PubMed.
- K. Barman, R. Asrey, R. K. Pal, S. K. Jha and K. Bhatia, Post-harvest nitric oxide treatment reduces chilling injury and enhances the shelf-life of mango (Mangifera indica L.) fruit during low-temperature storage, J. Hortic. Sci. Biotechnol., 2014, 89, 253–260 CrossRef CAS.
- V. Ziosi, A. M. Bregoli, C. Bonghi, T. Fossati, S. Biondi, G. Costa and P. Torrigiani, Transcription of ethylene perception and biosynthesis genes is altered by putrescine, spermidine and aminoethoxyvinylglycine (AVG) during ripening in peach fruit (Prunus persica), New Phytol., 2006, 172, 229–238 CrossRef CAS PubMed.
- A. D. Edreva Acad Kostov, Review polyamines in plants, Bulg. J. Plant Physiol., 1996, 22, 73–101 Search PubMed.
- S. K. Sahu, K. Barman and A. K. Singh, Nitric oxide application for postharvest quality retention of guava fruits, Acta Physiol. Plant., 2020, 42, 156 CrossRef CAS.
- Q. Wang, Z. Zhang, B. Wu and H. Zhang, Effect of spermidine and D-arginine treatments on ethylene synthesis in Xinjiang Saimaiti apricot, Sci. Hortic., 2019, 245, 289–293 CrossRef CAS.
- I. Raskin, Role of salicylic acid in plants, Annu. Rev. Plant Physiol. Plant Mol. Biol., 1992, 43, 439–463 CrossRef CAS.
- H. I. Lee, J. León and I. Raskin, Biosynthesis and metabolism of salicylic acid, Proc. Natl. Acad. Sci., 1995, 92, 4076–4079 CrossRef CAS PubMed.
- M. A. Strawn, S. K. Marr, K. Inoue, N. Inada, C. Zubieta and M. C. Wildermuth, Arabidopsis isochorismate synthase functional in pathogen-induced salicylate biosynthesis exhibits properties consistent with a role in diverse stress responses, J. Biol. Chem., 2007, 282, 5919–5933 CrossRef CAS PubMed.
- M. C. Verberne, R. Verpoorte, J. F. Bol, J. Mercado-Blanco and H. J. M. Linthorst, Overproduction of salicylic acid in plants by bacterial transgenes enhances pathogen resistance, Nat. Biotechnol., 2000, 18, 779–783 CrossRef CAS PubMed.
- M. C. Wildermuth, J. Dewdney, G. Wu and F. M. Ausubel, Isochorismate synthase is required to synthesize salicylic acid for plant defence, Nature, 2001, 414, 562–565 CrossRef CAS PubMed.
- M. Asghari and M. S. Aghdam, Impact of salicylic acid on post-harvest physiology of horticultural crops, Trends Food Sci. Technol., 2010, 21, 502–509 CrossRef CAS.
- D. Mandal, L. Pautu, T. K. Hazarika, B. P. Nautiyal and A. C. Shukla, Effect of salicylic acid on physico-chemical attributes and shelf life of tomato fruits at refrigerated storage, Int. J. Bio-Resour. Stress Manage., 2016, 7, 1272–1278 CrossRef.
- I. Raskin, H. Skubatz, W. Tang and B. J. D. Meeuse, Salicylic acid levels in thermogenic and non-thermogenic plants, Ann. Bot., 1990, 66, 369–373 CrossRef CAS.
- M. K. Srivastava and U. N. Dwivedi, Delayed ripening of banana fruit by salicylic acid, Plant Sci., 2000, 158, 87–96 CrossRef CAS PubMed.
- X. Fan, J. P. Mattheis and J. K. Fellman, Inhibition of apple fruit 1-aminocyclopropane-1-carboxylic acid oxidase activity and respiration by acetylsalicylic acid, J. Plant Physiol., 1996, 149, 469–471 CrossRef CAS.
- C. A. Leslie and R. J. Romani, Inhibition of ethylene biosynthesis by salicylic acid, Plant Physiol., 1988, 88, 833–837 CrossRef CAS PubMed.
- W. P. Xu, K. S. Chen, F. Li and S. L. Zhang, Regulation of lipoxygenase on jasmonic acid biosynthesis in ripening kiwifruit, Acta Phytophysiol. Sin., 2000, 26, 507–514 CAS.
- R. D. Marcelle, Relationships between mineral content, lipoxygenase activity, levels of 1-aminocyclopropane-1-carboxylic acid and ethylene emission in apple fruit flesh disks (cv. Jonagold) during storage, Postharvest Biol. Technol., 1991, 1, 101–109 CrossRef CAS.
- A. Kacperska and M. Kubacka-Zebalska, Formation of stress ethylene depends both on ACC synthesis and on the activity of free radical-generating system, Physiol. Plant., 1989, 77, 231–237 CrossRef CAS.
- J. F. Todd, G. Paliyath and J. E. Thompson, Characteristics of a membrane-associated Lipoxygenase in tomato fruit, Plant Physiol., 1990, 94, 1225–1232 CrossRef CAS PubMed.
-
M. Soleimani Aghdam, M. Asghari, M. Babalar and M. A. Askari Sarcheshmeh, In eco-friendly technology for postharvest produce quality, Elsevier, 2016, pp. 243–268 Search PubMed.
- Y. Mo, D. Gong, G. Liang, R. Han, J. Xie and W. Li, Enhanced preservation effects of sugar apple fruits by salicylic acid treatment during post-harvest storage, J. Sci. Food Agric., 2008, 88, 2693–2699 CrossRef CAS.
- Y. Y. Leshem and E. Haramaty, The characterization and contrasting effects of the nitric oxide free radical in vegetative stress and senescence of Pisum sativum Linn. Foliage, J. Plant Physiol., 1996, 148, 258–263 CrossRef CAS.
- G. Manjunatha, K. J. Gupta, V. Lokesh, L. A. Mur and B. Neelwarne, Nitric oxide counters ethylene effects on ripening fruits, Plant Signaling Behav., 2012, 7, 476–483 CrossRef CAS PubMed.
- M. Jakubowicz, H. Gałgańska, W. Nowak and J. Sadowski, Exogenously induced expression of ethylene biosynthesis, ethylene perception, phospholipase D, and Rboh-oxidase genes in broccoli seedlings, J. Exp. Bot., 2010, 61, 3475–3491 CrossRef CAS PubMed.
- C. Lindermayr, G. Saalbach, G. Bahnweg and J. Durner, Differential inhibition of arabidopsis methionine adenosyltransferases by Protein S-Nitrosylation, J. Biol. Chem., 2006, 281, 4285–4291 CrossRef CAS PubMed.
- Y.-B. Liu, Nitric oxide as a potent fumigant for postharvest pest control, J. Econ. Entomol., 2013, 106, 2267–2274 CrossRef CAS PubMed.
- S. Zhu and J. Zhou, Effect of nitric oxide on ethylene production in strawberry fruit during storage, Food Chem., 2007, 100, 1517–1522 CrossRef CAS.
- I. Ghasil, N. K. Meena, P. Bhatnagar, H. Singh, A. Kumar, S. K. Jain and M. Bhateshwar, Effect of postharvest treatments on biochemical and bioactive compounds of custard apple (Annona squamosa L.) Cv. Balanagar, Int. J. Fruit Sci., 2022, 22, 826–836 CrossRef.
- Z. Zhu, Z. Zhang, G. Qin and S. Tian, Effects of brassinosteroids on postharvest disease and senescence of jujube fruit in storage, Postharvest Biol. Technol., 2010, 56, 50–55 CrossRef CAS.
- Z. Min, L. Jiang, Y. Zhao, X. Wang, Q. Liu and Y. Zhang, Effects of 24-epibrassinolide on the postharvest quality and antioxidant activities of blueberry fruits, N. Z. J. Crop Hortic. Sci., 2022, 1–14 Search PubMed.
- J. Medina-Santamarina, F. Guillén, M. I. M. Ilea, M. C. Ruiz-Aracil, D. Valero, S. Castillo and M. Serrano, Melatonin treatments reduce chilling injury and delay ripening, leading to maintenance of quality in cherimoya fruit, Int. J. Mol. Sci., 2023, 24, 3787 CrossRef CAS PubMed.
- R. Zhai, J. Liu, F. Liu, Y. Zhao, L. Liu, C. Fang, H. Wang, X. Li, Z. Wang, F. Ma and L. Xu, Melatonin limited ethylene production, softening and reduced physiology disorder in pear (Pyrus communis L.) fruit during senescence, Postharvest Biol. Technol., 2018, 139, 38–46 CrossRef CAS.
- V. Gupta, N. K. Meena, Y. K. Sharma and K. Choudhary, Comparative study of different polysaccharide-based edible coatings on physicochemical attributes and bioactive compounds of mango cv. Dashehari fruits, eFood, 2023, 4(1), e55 CrossRef.
- C. Bhan, R. Asrey, N. K. Meena, S. G. Rudra, G. Chawla, R. Kumar and R. Kumar, Guar gum and chitosan-based composite edible coating extends the shelf life and preserves the bioactive compounds in stored Kinnow fruits, Int. J. Biol. Macromol., 2022, 222, 2922–2935 CrossRef CAS PubMed.
- F. Guillén, H. M. Díaz-Mula, P. J. Zapata, D. Valero, M. Serrano, S. Castillo and D. Martínez-Romero, Aloe arborescens and Aloe vera gels as coatings in delaying postharvest ripening in peach and plum fruit, Postharvest Biol. Technol., 2013, 83, 54–57 CrossRef.
- P. J. Zapata, F. Guillén, D. Martínez-Romero, S. Castillo, D. Valero and M. Serrano, Use of alginate or zein as edible coatings to delay postharvest ripening process and to maintain tomato (Solanum lycopersicon Mill) quality, J. Sci. Food Agric., 2008, 88, 1287–1293 CrossRef CAS.
- R. Sharma, P. Prajapati, H. Amin and Galib, Validation of standard manufacturing procedure of Guduci sattva (aqueous extract of Tinospora cordifolia (Willd.) Miers) and its tablets, Anc. Sci. Life, 2013, 33(1), 27–34 CrossRef PubMed.
- R. Sharma, R. Bolleddu, J. K. Maji, G. Ruknuddin and P. K. Prajapati,
In vitro α-amylase, α-glucosidase inhibitory activities and in vivo anti-hyperglycemic potential of different dosage forms of Guduchi (Tinospora Cordifolia [Willd.] Miers) prepared with Ayurvedic Bhavana process, Front. Pharmacol., 2021, 12, 642300 CrossRef CAS PubMed.
- C. Vilela, M. Kurek, Z. Hayouka, B. Röcker, S. Yildirim, M. D. C. Antunes, J. Nilsen-Nygaard, M. K. Pettersen and C. S. R. Freire, A concise guide to active agents for active food packaging, Trends Food Sci. Technol., 2018, 80, 212–222 CrossRef CAS.
- K. K. Gaikwad, S. Singh and Y. S. Negi, Ethylene scavengers for active packaging of fresh food produce, Environ. Chem. Lett., 2020, 18, 269–284 CrossRef CAS.
- L. A. Terry, T. Ilkenhans, S. Poulston, L. Rowsell and A. W. J. Smith, Development of new palladium-promoted ethylene scavenger, Postharvest Biol. Technol., 2007, 45, 214–220 CrossRef CAS.
- M. A. Opiyo and T. J. Ying, Advances in the management of ethylene in post-harvest handling of horticultural crops, Outlook Agric., 2006, 35, 51–55 CrossRef.
- D. R. Dilley, Controlled atmosphere storage – Chronology and technology, Acta Hortic., 2010, 493–502 CrossRef.
- L. Elsgaard, Ethylene removal at low temperatures under biofilter and batch conditions, Appl. Environ. Microbiol., 2000, 66, 3878–3882 CrossRef CAS PubMed.
- L. Elsgaard, Ethylene removal by a biofilter with immobilized bacteria, Appl. Environ. Microbiol., 1998, 64, 4168–4173 CrossRef CAS PubMed.
- J. Kim, Assessment of ethylene removal with Pseudomonas strains, J. Hazard. Mater., 2006, 131, 131–136 CrossRef CAS PubMed.
- F. X. Nascimento, M. J. Rossi, C. R. F. S. Soares, B. J. McConkey and B. R. Glick, New insights into 1-Aminocyclopropane-1-Carboxylate (ACC) deaminase phylogeny, evolution and ecological significance, PLoS One, 2014, 9, e99168 CrossRef PubMed.
- M. Ravanbakhsh, R. Sasidharan, L. A. C. J. Voesenek, G. A. Kowalchuk and A. Jousset, Microbial modulation of plant ethylene signaling: ecological and evolutionary consequences, Microbiome, 2018, 6, 52 CrossRef PubMed.
- M. Ravanbakhsh, R. Sasidharan, L. A. C. J. Voesenek, G. A. Kowalchuk and A. Jousset, ACC deaminase-producing rhizosphere bacteria modulate plant responses to flooding, J. Ecol., 2017, 105, 979–986 CrossRef CAS.
- T. E. Young, R. B. Meeley and D. R. Gallie, ACC synthase expression regulates leaf performance and drought tolerance in maize, Plant J., 2004, 40, 813–825 CrossRef CAS PubMed.
- E. Rosenberg and I. Zilber-Rosenberg, Microbes drive evolution of animals and plants: the hologenome concept, mBio, 2016, 7(2), 01395–15 CrossRef PubMed.
- P. Opio, S. Cao, L. Meng, C. Ma, L. Ba, J. Lei, N. Ji and R. Wang, Effect of ozone treatment on physicochemical parameters and ethylene biosynthesis inhibition in Guichang Kiwifruit, Food Sci. and Technol., 2022 DOI:10.1590/fst.64820.
- E. Bal, Extension of the postharvest life of nectarine using modified atmosphere packaging and potassium permanganate treatment, Turkish J. Agri.–Food Sci. Technol., 2018, 6, 1362–1369 CrossRef.
- S. B. Murmu and H. N. Mishra, Selection of the best active modified atmosphere packaging with ethylene and moisture scavengers to maintain quality of guava during low-temperature storage, Food Chem., 2018, 253, 55–62 CrossRef CAS PubMed.
- M. H. Álvarez-Hernández, G. B. Martínez-Hernández, F. Avalos-Belmontes, F. D. Miranda-Molina and F. Artés-Hernández, Postharvest quality retention of apricots by using a novel sepiolite–loaded potassium permanganate ethylene scavenger, Postharvest Biol. Technol., 2020, 160, 111061 CrossRef.
- A. G. Sanches, M. B. Da Silva, E. G. Silva Moreira, E. X. Dos Santos, K. R. P. Menezes and C. A. M. Cordeiro, Ethylene absorber (KMnO4) in postharvest quality of pinha (Anona squamosa L.), Emir. J. Food Agric., 2019, 31, 605 CrossRef.
- L. P. Le Nguyen, T. Zsom, M. S. Dam, L. Baranyai and G. Hitka, Evaluation of the 1-MCP microbubbles treatment for shelf-life extension for melons, Postharvest Biol. Technol., 2019, 150, 89–94 CrossRef.
- J. Guo, X. Wei, E. Lü, Y. Wang and Z. Deng, Ripening behavior and quality of 1-MCP treated d’Anjou pears during controlled atmosphere storage, Food Control, 2020, 117, 107364 CrossRef CAS.
- F. R. Thewes, V. Both, F. R. Thewes, A. Brackmann, R. Wagner, S. R. Ribeiro, V. Ludwig and F. P. Rossato, Pecan storage: Effects of 1-MCP on the overall quality and volatile compounds profile of shelled and unshelled pecans, LWT, 2021, 145, 111298 CrossRef CAS.
- N. M. Win, J. Yoo, H. P. Lwin, E. J. Lee, I.-K. Kang and J. Lee, Effects of 1-methylcyclopropene and aminoethoxyvinylglycine treatments on fruit quality and antioxidant metabolites in cold-stored ‘Sangjudungsi’ persimmons, Hortic. Environ. Biotechnol., 2021, 62, 891–905 CrossRef CAS.
-
N. Zulferiyenni, S. E. Widodo and A. Putri, in 6th Int’l Conference on Agriculture, Environment and Biological Sciences (ICAEBS’16), Kuala Lumpur, Malaysia, 2016 Search PubMed.
- B. Ozturk, S. Uzun and O. Karakaya, Combined effects of aminoethoxyvinylglycine and MAP on the fruit quality of kiwifruit during cold storage and shelf life, Sci. Hortic., 2019, 251, 209–214 CrossRef CAS.
- M. J. Tareen, Z. Singh, A. S. Khan, N. A. Abbasi and M. Naveed, Combined applications of aminoethoxyvinylglycine with salicylic acid or nitric oxide reduce oxidative stress in peach during ripening and cold storage, J. Plant Growth Regul., 2017, 36, 983–994 CrossRef CAS.
- E. Bal, Postharvest AminoethoxyVinylGlycine (AVG) treatment affects maturity and storage life of plum, J. Agri. Sci. Technol., 2019, 21, 1569–1579 Search PubMed.
- P. Promkaew, S. Kondo, N. Pongprasert, C. Wongs-Aree, S. Kaewsuksaeng and V. Srilaong, Application of AVG or 1-MCP-MBs on postharvest quality of pummelo cv. ‘Tubtim Siam’ (Citrus maxima Burm.), Food App. Biosci. J., 2019 Search PubMed.
- A. Ahmad and A. Ali, Improvement of postharvest quality, regulation of antioxidants capacity and softening enzymes activity of cold-stored carambola in response to polyamines application, Postharvest Biol. Technol., 2019, 148, 208–217 CrossRef CAS.
- H. A. Ennab, M. A. El-Shemy and S. M. Alam-Eldein, Salicylic acid and putrescine to reduce post-harvest storage problems and maintain quality of murcott mandarin fruit, Agron, 2020, 10, 115 CrossRef CAS.
- S. M. Zahedi, M. S. Hosseini, M. Karimi and A. Ebrahimzadeh, Effects of postharvest polyamine application and edible coating on maintaining quality of mango (Mangifera indica L.) cv. Langra during cold storage, Food Sci. Nutr., 2019, 7, 433–441 CrossRef CAS PubMed.
- N. Abbasi, I. Ali, I. Hafiz, M. Alenazi and M. Shafiq, Effects of putrescine application on peach fruit during storage,
Sustainability
, 2019, 11, 2013 CrossRef CAS.
- Y. Zhao, C. Song, D. A. Brummell, S. Qi, Q. Lin, J. Bi and Y. Duan, Salicylic acid treatment mitigates chilling injury in peach fruit by regulation of sucrose metabolism and soluble sugar content, Food Chem., 2021, 358, 129867 CrossRef CAS PubMed.
- N. Kumar, J. Tokas, M. Raghavendra and H. R. Singal, Impact of exogenous salicylic acid treatment on the cell wall metabolism and ripening process in postharvest tomato fruit stored at ambient temperature, Int. J. Food Sci. Technol., 2021, 56, 2961–2972 CrossRef CAS.
- V. Saurabh, K. Barman and A. K. Singh, Synergistic effect of salicylic acid and chitosan on postharvest life and quality attributes of jamun (Syzygium cumini Skeels) fruit, Acta Physiol. Plant, 2019, 41, 89 CrossRef.
- B. Jiang, R. Liu, X. Fang, C. Tong, H. Chen and H. Gao, Effects of salicylic acid treatment on fruit quality and wax composition of blueberry (Vaccinium virgatum Ait), Food Chem., 2022, 368, 130757 CrossRef CAS PubMed.
- S. Gheysarbigi, S. H. Mirdehghan, M. Ghasemnezhad and F. Nazoori, The inhibitory effect of nitric oxide on enzymatic browning reactions of in-package fresh pistachios (Pistacia vera L.), Postharvest Biol. Technol., 2020, 159, 110998 CrossRef.
- S. Yimyong, T. U. Datsenka, A. K. Handa and K. Seraypheap, Hot Water Treatment delays ripening-associated metabolic shift in ‘Okrong’ mango fruit during storage, J. American Soc. Horti. Sci., 2011, 136, 441–451 CAS.
- M. Ghasemnezhad, K. Marsh, R. Shilton, M. Babalar and A. Woolf, Effect of hot water treatments on chilling injury and heat damage in ‘satsuma’ mandarins: antioxidant enzymes and vacuolar ATPase, and pyrophosphatase, Postharvest Biol. Technol., 2008, 48, 364–371 CrossRef CAS.
- T. N. Tadesse and W. G. Abtew, Effect of hot water treatment on reduction of chilling injury and keeping quality in tomato (Solanum lycopersicum L.) fruits, J. Stored Products Postharvest Res., 2015, 7, 61–68 Search PubMed.
- L. Jat, S. S. Lakhawat, V. Singh, R. Meena, J. L. Choudhary and S. Gathala, Postharvest γ-irradiation treatment enhance nutritional and antioxidant potential of Indian jujube (Ziziphus mauritiana Lamk) fruit, Sci. Hortic., 2022, 301, 111127 CrossRef CAS.
- J. V. Tezotto-Uliana, N. D. Berno, F. R. Q. Saji and R. A. Kluge, Gamma radiation: An efficient technology to conserve the quality of fresh raspberries, Sci. Hortic., 2013, 164, 348–352 CrossRef.
- M. H. A. Rashid, B. W. W. Grout, A. Continella and T. M. M. Mahmud, Low-dose gamma irradiation following hot water immersion of papaya (Carica papaya linn.) fruits provides additional
control of postharvest fungal infection to extend shelf life, Rad. Physics Chem., 2015, 110, 77–81 CrossRef CAS.
- A. Basit, M. Younas, S. T. Shah, M. Sajid, I. Aziz and H. I. Mohamed, Trends in potassium permanganate (ethylene absorbent) management strategies: towards mitigating postharvest losses and quality of mango (Mangifera indica L) Fruit, Food Bioproc. Tech., 2023, 16, 2172–2183 CrossRef.
- S. F. Corrêa, M. B. Filho, M. G. da Silva, J. G. Oliveira, E. M. M. Aroucha, R. F. Silva, M. G. Pereira and H. Vargas, Effect of the potassium permanganate during papaya fruit ripening: Ethylene production, J. de Physique IV (Proceedings), 2005, 125, 869–871 CrossRef.
- K. Syamsua, E. Warsikib, S. Yulianic and S. M. Widayanti, Nano Zeolite-KMnO4 as ethylene adsorber in active packaging of horticulture products (Musa Paradisiaca), Int. J. Sci.: Basic App. Res., 2016, 30, 93–103 Search PubMed.
- S. Dhakal, S. Aryal, P. Khanal, B. Basnet and A. Srivastava, Effect of different concentrations of potassium permanganate (KMnO4) on shelf life and quality of banana (Musa paradisiaca L.), Funda. App. Agri., 2021, 1 Search PubMed.
- I. S. Minas, A. R. Vicente, A. P. Dhanapal, G. A. Manganaris, V. Goulas, M. Vasilakakis, C. H. Crisosto and A. Molassiotis, Ozone-induced kiwifruit ripening delay is mediated by ethylene biosynthesis inhibition and cell wall dismantling regulation, Plant Sci., 2014, 229, 76–85 CrossRef CAS PubMed.
- M. K. Ong, A. Ali, P. G. Alderson and C. F. Forney, Effect of different concentrations of ozone on physiological changes associated to gas exchange, fruit ripening, fruit surface quality and defence-related enzymes levels in papaya fruit during ambient storage, Sci. Hortic., 2014, 179, 163–169 CrossRef CAS.
- S. Cao, L. Meng, C. Ma, L. Ba, J. Lei, N. Ji and R. Wang, Effect of ozone treatment on physicochemical parameters and ethylene biosynthesis inhibition in Guichang Kiwifruit, Food Sci. Technol., 2022, 42, e64820 CrossRef.
- N. Tzortzakis, T. Taybi, R. Roberts, I. Singleton, A. Borland and J. Barnes, Low-level atmospheric ozone exposure induces protection against Botrytis cinerea with down-regulation of ethylene-, jasmonate- and pathogenesis-related genes in tomato fruit, Postharvest Biol. Technol., 2011, 61, 152–159 CrossRef CAS.
- L.-Y. Chang and J. K. Brecht, Responses of 1-methylcyclopropene (1-MCP)−treated banana fruit to pre− and post−treatment ethylene exposure, Sci. Hortic., 2023, 309, 111636 CrossRef CAS.
- A. H. Kazimi, O. W. Mitalo, A. Azimi, K. Masuda, C. Yano, T. Akagi, K. Ushijima and Y. Kubo, 1-Methylcyclopropene pretreatment in ‘La France’ pears to extend postharvest life and maximize fruit quality, Hort, J., 2023, 92, QH-012 Search PubMed.
- J. A. Salazar, D. Ruiz, P. Zapata, P. J. Martínez-García and P. Martínez-Gómez, Whole transcriptome analyses of apricots and Japanese plum fruits after 1-mcp (ethylene-inhibitor) and ethrel (ethylene-precursor) treatments reveal new insights into the physiology of the ripening process, Int. J. Mol. Sci., 2022, 23, 11045 CrossRef CAS PubMed.
- A. J. Sachin, D. V. S. Rao, K. Ravishankar, K. Ranjitha, C. Vasugi, C. K. Narayana and S. V. R. Reddy, 1-MCP treatment modulated physiological, biochemical and gene expression activities of guava during low-temperature storage, Acta Physiol. Plant, 2022, 44, 125 CrossRef CAS.
- D. Sivakumar, F. Van Deventer, L. A. Terry, G. A. Polenta and L. Korsten, Combination of 1-methylcyclopropene treatment and controlled atmosphere storage retains overall fruit quality and bioactive compounds in mango, J. Sci. Food Agric., 2012, 92, 821–830 CrossRef CAS PubMed.
- I. T. Petkou, T. S. Pritsa and E. M. Sfakiotakis, Effects of polyamines on ethylene production, respiration and ripening of kiwifruit, J. Hortic. Sci. Biotechnol., 2004, 79, 977–980 CrossRef CAS.
- Md. J. Jhalegar, R. R. Sharma, R. K. Pal and V. Rana, Effect of postharvest treatments with polyamines on physiological and biochemical attributes of kiwifruit (Actinidia deliciosa ) cv. Allison, Fruits, 2012, 67, 13–22 CrossRef CAS.
- A. Pérez-Vicente, D. Martínez-Romero, Á. Carbonell, M. Serrano, F. Riquelme, F. Guillén and D. Valero, Role of polyamines in extending shelf life and the reduction of mechanical damage during plum (Prunus salicina Lindl.) storage, Postharvest Biol. Technol., 2002, 25, 25–32 CrossRef.
- Y. Ji, Y. Qu, Z. Jiang, J. Yan, J. Chu, M. Xu, X. Su, H. Yuan and A. Wang, The mechanism for brassinosteroids suppressing climacteric fruit ripening, Plant Physiol., 2021, 185, 1875–1893 CrossRef CAS PubMed.
- S. Liu, H. Huang, D. J. Huber, Y. Pan, X. Shi and Z. Zhang, Delay of ripening and softening in ‘Guifei’ mango fruit by postharvest application of melatonin, Postharvest Biol. Technol., 2020, 163, 111136 CrossRef CAS.
- N. Hira, O. W. Mitalo, R. Okada, M. Sangawa, K. Masuda, N. Fujita, K. Ushijima, T. Akagi and Y. Kubo, The effect of layer-by-layer edible coating on the shelf life and transcriptome of ‘Kosui’ Japanese pear fruit, Postharvest Biol. Technol., 2022, 185, 111787 CrossRef CAS.
- C. Bhan, R. Asrey, N. K. Meena, S. G. Rudra, G. Chawla, R. Kumar and R. Kumar, Guar gum and chitosan-based composite edible coating extends the shelf life and preserves the bioactive compounds in stored Kinnow fruits, Int. J. Biol. Macromol., 2022, 222, 2922–2935 CrossRef CAS PubMed.
|
This journal is © The Royal Society of Chemistry 2023 |
Click here to see how this site uses Cookies. View our privacy policy here.