Sustainable plant protein: an up-to-date overview of sources, extraction techniques and utilization
Received
9th January 2023
, Accepted 12th April 2023
First published on 13th April 2023
Abstract
Protein is a vital macronutrient that helps in the survival of human beings. Its major functions include body building and promoting normal growth and maintenance. The two key sources of protein in the diet are animal and plant proteins. Vegetable/plant protein intake has several health benefits, while a greater intake of animal protein can be associated with a number of diseases. Besides, animal protein is one of the major causes of greenhouse gas emissions and subsequently a source of carbon footprint. In the context of plant proteins, there are three main categories namely leguminous proteins, cereal proteins, and oilseed proteins. Legumes play a crucial role in the human diet as they contain essential amino acids, calories, minerals, and vitamins. Cereals are vital for human nutrition, but their protein quality is poor due to a lack of amino acid lysine. Oilseed proteins can be used to provide good nutritive value and functional qualities to foods. Processing of plant proteins involves physico-chemical and thermal treatments that affect both the nutritional value and functional properties of the final product. With the advent of green chemistry, research is increasingly focusing on non-thermal/green methods to improve extraction efficiency and minimize proteolysis during extraction. The present review focuses on the recent literature about plant protein sources, isolation/extraction techniques, and application of plant proteins in the food sector.
1. Introduction
Proteins are high-molecular-weight organic components made up of amino acids that cannot be substituted by lipids, fibers, or other organic compounds. Furthermore, they constitute a fundamental building material for meeting the population's increasing demand for high-quality nutrition.1 Protein is a vital component of human nutrition since it is required for muscle mass maintenance, immunological responses, cell signaling, and cell repair.2 The amino acid profile, bioavailability, digestibility, anti-nutritional factors, and protein processing methods have a significant impact on protein nutritional value.3 Each amino acid plays a unique and crucial part in how the body works. Essential amino acids cannot be made by the human body and must be obtained from food. A high-quality protein should contain sufficient amounts of each of the nine essential amino acids.3,4 Besides, there are usually two types of proteins: complete proteins and incomplete proteins. Foods that contain all essential amino acids in optimal amounts for body building are called complete proteins. Incomplete proteins also contain essential amino acids, but not in sufficient amounts.5
In addition, an individual can obtain protein from two main sources, i.e. animal and plant sources.2 In comparison to plant proteins, animal proteins are easier to digest, have high biological value (BV), net protein utilization (NPU), and protein digestibility-corrected amino acid score (PDCAAS). In contrast, animal proteins were observed to have a lower number of α-helices and a higher number of β-sheet structures than plant proteins, making them much more susceptible to enzymatic degradation. Also, animal foods are a source of increased carbon footprint. Concerning the market cost, land requirement, environmental impact, and phenomenon of water eutrophication, animal proteins are in the spotlight. Furthermore, consumers' trust in animal proteins has been eroded as a result of food safety concerns caused by diseases like bovine spongiform encephalopathy.6,7
In contrast, plant proteins contain good amounts of essential amino acids (Fig. 1). Besides, they have poor proteolytic digestibility because of the presence of a higher proportion of fibers in plant foods. Some plant proteins contain anti-nutritional elements, such as trypsin inhibitors, which can inhibit protein digestion and reduce the overall efficiency of the proteins' final utilization.4 Additionally, they have a larger concentration of antinutritional components, which is another factor contributing to their reduced digestibility. Processing methods can boost the digestibility of these proteins by reducing the amount of these antinutritive components.7 Because of their renewable and biodegradable nature, as well as functional qualities such as emulsifying ability, fibrogenic capabilities, and water-solubilizing action, plant proteins have been intensively investigated in recent years.
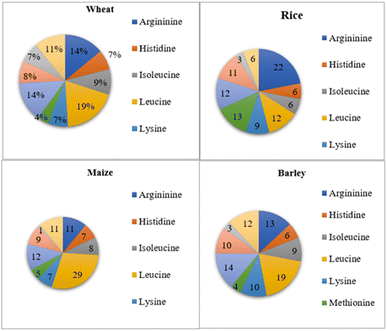 |
| Fig. 1 Amino acid content of plant protein. | |
Plant proteins are excellent microencapsulation wall material that can be used in meals, pharmaceuticals, and cosmetics.8 As functional ingredients in food preparations, plant proteins are an economical and versatile alternative to animal proteins. However, the successful replacement of animal protein requires technological advances. To be able to make these advances effective and efficient, understanding the relationship is essential. The study of the relationship between protein structure and functional characteristics is important.9
The most important step in utilizing plant proteins is the use of suitable extraction techniques based on plant matrices. Different researchers have worked on conventional and novel ways of extracting protein. The conventional methods are some of the common traditional techniques used for protein extraction such as aqueous-based protein extraction, alkali, solvent, and use of detergent while novel or non-conventional extraction techniques offer effective recovery of protein with energy-saving effects such as the use of microwaves, ultrasound, high-intensity pulses, and enzyme assisted treatment. The conventional ways of extracting protein led to lower protein yields with greater degradation of proteins. This decrease in the yield of plant protein depends on many aspects, viz. extraction time, solvent, pH, and temperature. Therefore, scientists today are focusing on non-thermal green technologies to improve extraction efficiency and reduce proteolysis during extraction. These novel techniques have no detrimental impact on the ecological system and the resulting proteins are safe for human use with only small quantities of toxic chemicals and solvents being used.10
Moreover, with increasing consumer awareness about the disadvantages of animal proteins, today's research needs to focus on the efficient handling and use of plant proteins for better applicability. Furthermore, the food industry as well as commercial players should be interested in the challenges and opportunities of investing in the plant protein trend.11 A literature search revealed that information about the sources of plant protein and its better applicability in the food sector is scarce. Therefore, this review provides an overview of the various plant food sources that can be used as protein sources, conventional and novel protein extraction methods, and their applications, and development, as well as the future prospects of plant protein.
2. Different types of plant proteins
In recent times, there has been a growing trend of using plant proteins as a cost-effective and adaptable substitute for animal-based sources in human diets. Plant-based ingredients are also being utilized as functional components in product development. Numerous plant-based proteins including leguminous proteins (viz. soybean, pea, chickpea, mung bean, and kidney bean), cereal protein (such as oat, rice, wheat, and corn), and oilseed protein (such as peanut protein, flaxseed, sesame, and sunflower) have been extensively researched and incorporated as protein supplements.12
Leguminous protein: legumes are another widely consumed food grain in the human diet, after cereals. These legumes are considered a cheap source of protein as well as other vital nutrients. Pea seeds contain two important globulin proteins namely vicilin and legumin. Besides, they are also a rich source of lysine and contain a balanced amino acid profile.13 Furthermore, chickpeas also contain significant amounts of essential amino acids with high bioavailability and, on the other hand, anti-nutritional factors in low amounts. In addition, numerous bioactivities of chickpea protein hydrolysates have been reported, e.g. reduced antigen effect and inhibition of angiotensin I converting enzyme.14
Cereal protein: cereal grains such as rice, wheat, oat, barley, etc. are used as a plant protein source. Among cereals, oat protein is found to be valuable. The total protein content of oats is 12–24% with a high proportion of globulin and albumin. In particular, globulins account for 70–80% of total oat protein.15 The oat globulin protein has three major fractions, 3S, 7S, and 11S (molecular weight of 54 kDa with a basic subunit of 22 kDa and an acidic subunit of 32 kDa). The amino acid content present in 100 g of oat protein shows the presence of amino acids viz. valine (5.2–5.7 g), tryptophan (0.8–0.9 g), threonine (3.3–3.7 g), lysine (4.1–4.5 g), leucine (7.4–7.7 g), isoleucine (3.8–4.1 g) and histidine (2.1–2.9 g).16 Oat protein concentrate is also poorly soluble, and several chemical modifications have been employed to improve protein solubility, including succinylation, acetylation, and enzymatic hydrolysis.15 Furthermore, wheat protein i.e. gluten is distinctive from other plant proteins and cereals in its capacity to create a cohesive mixture with viscoelastic properties after it is made malleable.17 Cereals constitute a major source of energy and protein, but they are deficient in the critical amino acid lysine. In contrast, legumes are likewise high in protein and amino acids, but they are also deficient in sulphur-containing amino acids. Hence, adding legumes to cereals would increase the protein and nutritional density of the resulting food products.18
Oilseed protein: more than 200 species of oilseeds are cultivated worldwide. The most common oilseed crops are soybeans, sunflowers, coconuts, rapeseeds, olives, and peanuts while oilseeds such as sesame, flax, and cotton seeds are utilized at a lower level. Furthermore, some less common oilseeds have gained wide popularity in current times such as chia seeds, primrose, pumpkin, hemp seeds, nigella, and milk thistle. Oilseed processing by-products are good sources of protein having excellent nutritional and bioactive effects. These oilseeds are exciting raw materials that offer the possibility of developing healthy products or functional foods.19
2.1. Leguminous proteins
Legumes belong to the Leguminosae family often known as Fabaceae. They play a substantial part in human health and nutrition since they hold a high profile of proteins, calories, vitamins, and minerals. Legumes are considered a good source of protein as they provide an adequate amount of amino acids, and thus utilization of legumes in the food industry is currently increasing.20 As compared with animal proteins, the nutritional value of plant proteins is lower, although they retain a high protein content. The digestibility of proteins and essential amino acids, as well as the quantity of essential amino acids concerning dietary requirements, helps to assess the quality of protein in a food. For the past 20 years, the Protein Digestibility Corrected Amino Acid Score (PDCAAS) has been employed as a tool to evaluate protein quality. The PDCAAS values for most legumes are below 100 (e.g., for peas they are 50–68, for lentils 51–63, for chickpeas 52, for beans 53–67 and for peanuts 30–75), but these values may vary based on variety, cultivar, and processing technology.21 Besides, the high levels of naturally occurring anti-nutritive substances, such as trypsin and tannin inhibitors in legumes, are responsible for lowering the digestibility of proteins and amino acids.22 Phytic acids, phenols, and tannins may act as potential antioxidants with health-promoting properties. The persistence or removal of these molecules varies based on the preferences of the consumer. Phytic acid has lately been shown to have significant antioxidant, hypoglycemic, and anticarcinogenic effects.23 Legumes exhibit certain groups of anti-nutritional factors that inhibit protein digestibility by suppressing enzyme activity, which can occasionally result in the formation of protein-ANF complexes. Some grain legumes that contain a high percentage of galactosides in a carbohydrate component cause flatulence.21 By neutralizing anti-nutritional elements and increasing the bioavailability of nutrients, common food preparation methods including soaking, sprouting, boiling, and fermentation enhance the flavor and palatability of legumes. They also facilitate the absorption and digestion of protein and carbohydrates.23 The usage of legume proteins in food formulation is mainly dependent on their functional attributes such as the physical as well as chemical functions of protein, which affect their behavior in the food system in the course of processing, storage, cooking and utilization.
According to the mechanism of action, the functional properties of proteins are divided into three key units: (i) hydration-related characteristics (water/oil absorption capacity, thickening, solubility, & wettability), (ii) properties related to the structure of protein and rheological features (aggregation, elasticity, viscosity, adhesiveness, gelification, etc.) and (iii) attributes linked with protein surface characteristics (emulsification and foaming capacity, formation of protein-lipid films, whipability etc.). However, the most crucial characteristics of plant proteins are the solubility, ability to bind to fat and water, ability to form gels and exhibit rheological behaviors, and ability to emulsify, foam, and whip, all of which are influenced by environmental factors, processing conditions, and the molecular size/structure and charge distribution of the protein molecules.24
2.1.1. Soy protein.
Soybeans (Glycine max) are legumes rich in high-quality protein and edible oils. Soybeans are about 36% protein, 15% soluble carbohydrates, 15% insoluble carbohydrates, and 18% oil. Depending on the type, region, and weather, these values can vary. Because of their distinctive chemical makeup, they are a rich source of nutritional components. As compared to other varieties of beans and grains they have high protein content25 (Table 1).
Table 1 Protein amino acid composition of different leguminous proteins (g/100 g protein)
Leguminous protein |
Amino acids |
Ref. |
Arginine |
Histidine |
Isoleucine |
Leucine |
Lysine |
Methionine |
Cystine |
Phenylalanine |
Tyrosine |
Threonine |
Tryptophan |
Valine |
Soy isolate |
8.5 |
2.6 |
4.9–5.0 |
7.9–8.2 |
5.7–6.4 |
1.3 |
1.0–1.3 |
5.2–5.9 |
3.9 |
3.7–3.8 |
1.0–1.4 |
5.1–5.2 |
35 and 38 |
Pea |
8.4–9.8 |
2.4 |
3.4–4.4 |
7.3–7.6 |
6.7–8.4 |
0.9 |
0.6–1 |
4.6–5.7 |
3.7–4 |
3.8–4 |
0.4–0.9 |
3.9–4.9 |
107 and 108 |
Kidney bean |
6.9 |
3 |
3.7 |
7.2 |
7 |
1.7 |
1.2 |
4.6 |
3.1 |
3.4 |
— |
4.1 |
109
|
Mung bean |
4.5–6.7 |
3.2–3.5 |
4.3 |
6.9–9.77 |
4.1–8.1 |
0.9–1.2 |
6.34 |
4.6–6.2 |
2.2–3.4 |
2.7–4.74 |
1.2 |
5.1 |
52 and 110 |
Soybeans originally provide abundant dietary proteins and peptides found in Asian foods for a wide range of uses. Natto, miso, and other soy-based food products are some examples of fermented soy-based foods. Soy protein contains all essential amino acids and many bioactive nutritional components, as well as exhibiting emulsifying capacity and water–oil holding capacity. It is perhaps the most economically important bean in the world and a potential source of bioactive peptides, providing plant-based proteins and compounds to millions of people.26 Currently, rather than for their nutritional benefits, the various soy protein types are still predominantly used for their functional impacts such as effectiveness against chronic cancer, cardiovascular disease, and type 2 diabetes. However, a number of businesses are currently developing foods where soy proteins are the primary protein source, and they are utilizing the functional qualities of the proteins to successfully manufacture these products.27 As it has great gelation capabilities, superior nutritional content, and low cost, it has been acknowledged as a promising alternative for application in the development of meat substitutes made from plant proteins.28 The primary drawback of soy protein is its noticeable unpleasant taste in the resulting products. Two types of unpleasant tastes exist: the first is a grassy and beany taste, which results from the presence of lipoxygenases in soybeans; the second is a bitter and puckering taste, which is due to the presence of saponins and isoflavones.17
2.1.1.1 Tofu.
The most well-known meat substitute is probably soy-based product “tofu”. Tofu is made from soybeans and contains substantial amounts of calcium, iron, and protein. It is found commonly in block form, and despite being tasteless on its own, it rapidly absorbs the flavors of marinades, sauces, and other dressings.29 On a fresh weight basis, tofu includes roughly 8% of the total protein, 4–5% fats, and about 2% carbohydrates. Due to its lack of cholesterol and small amount of dietary fiber (approximately 1%), tofu has unique nutritional value. The rich vitamin and mineral content of tofu also contributes to its physiological significance.30 Generally, meat alternatives should taste like meat. Additionally, meat substitutes should have a fibrous muscle structure that closely resembles real meat, and comparable moisture content, bite resistance, and texture.28
2.1.1.2 Textured vegetable protein (TVP).
These are animal meat alternatives, usually made from soybeans. Despite the fairly time-consuming production process, the finished product has a fibrous consistency that is extremely comparable to meat.30 However, a newly developed procedure called high-moisture extrusion generates products with high moisture content that do not require rehydration and have a fibrous structure that more closely resembles animal meat, evading the unpleasant mouthfeel and texture quality that occurs when chewing low-moisture products.28 TVP is made by hot extruding defatted soy proteins into enlarged, high-protein chunks, grains, strips, nuggets, and other shapes. TVP has the texture of meat because of the denaturized proteins used in its production.30
2.1.2. Pea protein.
Peas (Pisum sativum L.) are the most widely cultivated and eaten legumes in the world. They are an excellent source of bioactive peptides with antioxidant and angiotensin-I converting enzyme (ACE) inhibitory properties that have beneficial effects on health.31
Their minimal allergenicity, non-transgenic variety, high nutrient content, easy availability, and origin as a sustainable crop make pea protein a viable alternative to conventional protein ingredients such as animal protein and soy protein. Being a potent supplier of essential amino acids such as phenylalanine, arginine, lysine, leucine, and isoleucine, which are not normally found in grains, pea protein is considered a high-quality protein. However, sulfur-containing amino acids, especially methionine as well as cysteine, are deficient in pea protein. Pea protein isolates' amino acid scores (AAS) (1.56) are marginally lower than those of soy isolates (1.69) but greater than those of egg white (1.19).32
Besides, a variety of peas namely field peas also contain substantial quantities of protein (23.1–30.9%), fat (1.5–2.0%), and other minor components based on the variety, harvest maturity, and growing environment. Albumins and globulins are the two major types of protein present in peas, which make up up to 10–20% and 70–80% of the total pea protein, respectively. When compared to globulins, albumins in peas have higher quantities of important amino acids such as lysine, tryptophan, methionine, and threonine. Albumins are thought to be water-soluble metabolic proteins. Legumin and vicilin proteins, along with convicilin in trace levels, make up the majority of globulins, which are classified as salt-soluble storage proteins.46
Pea protein is a novel and useful alternative to other proteins in functional food applications owing to its accessibility, low cost, nutrient quotient, and therapeutic potential. Pea protein isolates, in contrast to conventional cereal proteins, are gluten-free and can be a valuable ingredient in the creation of gluten-free products because of their exceptional physicochemical characteristics, which include outstanding water as well as oil absorption capacity, gelation ability, and gel clarity.34
Applications of pea protein in the food industry include its usage in beverages, sauces, salad dressings, baked goods, and other food products that could all benefit from the prepared pea protein isolates. The use of pea protein as a binder in meat applications is based on its lower water-holding capacity. In a recent study, pea protein isolates were created, where their functionality was found to be acceptable; however, the isolates' flavor and use in a variety of food products were not studied, which could pose significant challenges for product developers.35
2.1.3. Kidney bean protein.
Kidney bean (Phaseolus vulgaris L.) is the most commonly grown and eaten legume in Africa, India, Latin America, and Mexico. These beans offer a range of health-related advantages, involving reducing the risk of heart and kidney diseases, a lower glycemic index for diabetics, greater satiation, and cancer prevention. In addition, kidney beans are a valuable supplier of protein as well as minerals for animal feed production, as well as being a potential raw material for human food processing. On the other hand, kidney beans have a low protein digestibility due to the occurrence of antinutrients, some of which also reduce trace elements and protein bioavailability. Anti-nutrients and α-galactosides (e.g., stachyose, raffinose, and verbascose) are two unfavorable components of beans that may hinder protein and carbohydrate consumption.36 Kidney beans typically contain protein (20–30%), with a significantly high amino acid content but a minimal content of sulfur-containing amino acids, particularly tryptophan, and methionine. Furthermore, kidney bean and field pea proteins have been proven to be adequate for making gluten-free muffins and have similar properties to those prepared with wheat gluten.34 Ammonium sulfate precipitation of protein from kidney bean seeds can yield two main components, albumin and globulin. These components are rich in lysine and arginine, making them suitable for use in fortifying foods. While kidney bean powder outperforms soybean powder in terms of gelation ability, its emulsifying activity and foam stability are comparable. Additionally, the gelation ability of kidney bean protein isolate (KPI) is better than that of mung bean protein isolate.37
2.1.4. Mung bean protein.
Mung beans (Vigna radiata (L.)) have a quick growth cycle of approximately 70–90 days. Many Asian countries, as well as drier regions of Southern Europe and warmer regions of Canada and the United States abundantly grow mung beans. Mung beans are well recognized for their detoxifying bioactivities as a major plant-based food source. It has also been used to treat a variety of ailments, ranging from improving the human cognitive role to preventing heat stroke. Mung bean has a high nutritional content, especially in the seed part.38 Humans and other animals use it as a source of food and feed. Mung beans have a protein content of about 20.97–31.32%, compared to 18–22% and 20–30% for soybeans and kidney beans, respectively. In addition, the protein content of mung beans is about twice that of cereal grains like maize, which have a smaller protein content (7–10%), and much higher than that of traditional root-based crops.38 Mung beans are a type of leguminous plant. They have a more than 2000 year history of cultivation in China. Owing to their biological properties, which include detoxification, cholesterol reduction, and anti-tumor and anti-inflammatory properties, mung beans are one of the most common foods in China. They contain an abundance of vitamins, minerals, and other essential nutrients.39 Mung bean seeds are exceptionally high in protein, with a protein level of 20.97–31.32 percent. As per the Food and Agriculture Organization of the United Nations (FAO)/World Health Organization (WHO) criteria, mung bean received a chemical score of 76 percent. Consuming mung bean seeds along with cereal grains is recommended due to their high protein content and digestibility that significantly improve the quality of protein intake as part of a vegetarian diet.38
2.2. Cereal proteins
Cereals are members of the monocotyledon family Poaceae. They are particularly significant because they are the principal staple food in different regions of the globe. Cereals are dietary staples as they contain an abundance of essential nutrients viz. vitamins, dietary fiber, proteins, and carbohydrates.40,41 Wheat (Triticum aestivum), maize (Zea mays), sorghum (Sorghum vulgare), rice (Oryza sativa), and millets like pearl millet (Pennisetum glaucum) and finger millet (Eleusine coracana) are common types of cereal grains.18 Cereal grains satisfy over 60% of the world's food supply and are grown on over 73% of the world's harvested land. Cereals can be used as a fermentable substrate for the growth of probiotic microbial cultures, especially Lactobacilli and Bifidobacteria, or as dietary fiber promoting numerous beneficial physiological impacts in functional food compositions (Fig. 2). The most popular cereal-based functional foods and nutraceuticals are those made from wheat, buckwheat, oat, barley, flaxseed, psyllium, and brown rice.42
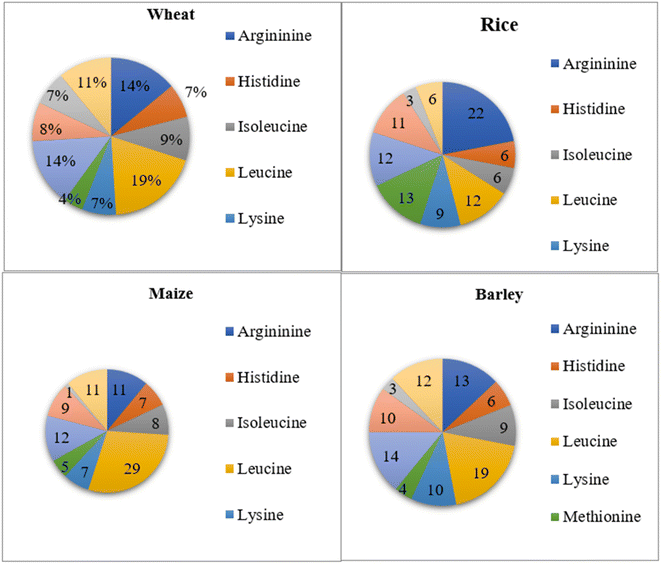 |
| Fig. 2 Percentage distribution of essential amino acids in cereals. | |
The protein quality of cereals is reduced due to their amino acid content. Cereals do not contain sufficient quantities of essential amino acids such as lysine, tryptophan and threonine. On the other hand, they are a rich source of sulfur-containing amino acids, such as methionine and cystine, so they complement lysine-rich, low-sulfur-containing legume proteins very well. However, owing to the low levels of essential amino acids, these proteins have lower net protein utilization and protein efficiency ratio (PER). In general, the PER for cereal protein varies from 0.8 to 2.0 in comparison to 2.5 for milk casein. In the context of cereal grains, rice and oat proteins are considered to be the most available and high-quality proteins.18,43
The modernized society and the expansion of the cereal food industry created a need for highly efficient processing technologies, especially for flour production.44 Cereal proteins possess significant potential for various industrial applications, whether in their original form or modified form. Wheat glutens are particularly promising for use in coatings and films due to their exceptional film-forming characteristics. However, additional research is necessary to examine the proteins of maize and sorghum for this specific purpose. These coatings and films can be utilized in the food industry and packaging sectors to regulate barrier properties for gases, flavors, and water vapor. In baking processes, glutenins and gliadins, which are the storage proteins of cereals, are essential in providing dough elasticity and gas retention. The unique properties of wheat glutens make them crucial in bread-making, as they improve dough strength and gas retention, control its expansion, extend dough life, enhance water absorption, and impart natural flavors and colors while preserving the structure and quality of the baked products.45
2.2.1. Wheat protein.
Wheat is one of the common cereal grains consumed in different parts of the world. Wheat flour is used to make leavened as well as unleavened loaves of bread, noodles, pasta, biscuits, etc. Wheat flour ingredients play an important role in determining the functionality of the wheat grain. The quality of wheat protein is a complicated term that necessitates application-specific specifications (for example, breadmaking requires a different functionality than pasta making).46
The protein content of wheat grains is an important indicator of the quality of wheat for human consumption and is sometimes neglected in attempts to improve crops. Grain and protein yields are expected to be lower and more unpredictable due to reduced growth stimulation from high CO2 emissions. Introducing a warming-adapted genotype (taking into account changes in CO2 and precipitation) could increase global wheat production by 7% and protein yield by 2%, but grain protein concentrations decreased by 1.1%, suggesting a relative shift of 8.6%. Adaptations to climate change that increase cereal yields are not necessarily good for cereal quality and put further pressure on global wheat supplies.34 Hexaploid Triticum aestivum wheat varieties are used to make wheat flour for bread. There are significant variances in the bread-making quality of the flour. The link between the protein composition of Triticum aestivum and bread-making quality is examined. The details of the properties (structure, size, & content) of the glutenin protein fraction and how they affect its function are also discussed. A more in-depth look at the features of the glutenin protein fraction (structure, size, and content) and how they affect its functionality is also provided.46,47
2.2.2. Corn protein.
Corn, also known as maize, is one of the popular cereal grains, followed by rice and wheat. In 2013, global maize production surpassed 1 billion metric tons, with the United States accounting for around 35% of the world's total production. Corn is a staple cereal grain for many people in Latin America, Africa, and Asia, where it is eaten as “corn on the cob” or corn kernels and used to make a variety of traditional dishes. Corn is a very significant cereal grain in the food business in the United States. Corn is milled either wet or dry to develop a range of food preparations. Corn starch and oil are the main products of corn wet milling, whereas corn gluten, seed coat, and steeping solids are secondary products.48 Zein is a key storage protein found in corn. Commercial attention has focused on the ability of zein and related resins to form tough, lustrous, hydrophobic, and oleophobic coatings that are resistant to microbiological attacks. Zein has also been utilized to make microspheres that delay medication release until it reaches the gut, protect pharmaceuticals from stomach acid, and offer a system for continuous drug release in the stream. Biodegradable films and plastics have also been made from zein.49 Dry milling, alkali treatment, wet milling, and dry milling processes for ethanol production are the four main ways of processing corn. Alkali-treated and dried corn is for direct human consumption.50 On a dry basis, the protein present in various varieties of corn ranges from 6 to 12%. The endosperm tissue contains around 75% of the protein. The rest is split between the germ and the bran. The hardness of corn endosperm is decided solely by the protein zein. The solubility of the four major protein types in corn is mainly explained by their solubility in specific solvents.
The solubility of four primary kinds of protein in corn is principally described by their solubility in specific solvents. Zein is a member of the prolamin protein family, which is found exclusively in cereals. John Gorham initially detected it in 1821 by infusing water in Zea, often recognized as ‘Indian corn’ in the United States. The endosperm contains almost all of the zein, while the glutelin is distributed between the endosperm and the germ. Albumin and globulin are mainly found in the germ part of the grain corn. Endosperm proteins are mainly found in CGM while germ proteins are mainly found in CGF among wet-milled protein co-products.50
2.2.3. Rice protein.
Rice (Oryza sativa L.) is a monocotyledonous plant belonging to the grass family, and is an important food crop with an annual production of about 480 million tons worldwide. Rice is cultivated in more than 100 nations, with countries like China and India alone producing over half of the global rice. Only the caryopsis is edible, thus the mature rice grain is stored as rough rice, with the caryopsis enclosed in a thick siliceous hull that must be treated before being ingested by humans. After loosening the rice husk and removing impurities, it is separated from the husk. Bran is composed of the pericarp, seed coat, nucellus, aleurone, pulverized embryo, and several starch pieces of endosperm and husk, which are removed by grinding or abrasion processes, or a combination of both, to make milled rice or white rice obtained from the endosperm.51 Milled rice and regular rice flour have a low protein content (about 7–9%). Rice protein, on the other hand, is nutritious, hypoallergenic, and particularly healthy for human consumption. It has been determined that the alkaline extraction of rice flour followed by pH adjustment to the isoelectric point to precipitate the protein can produce relatively pure rice protein. Rice proteins are commonly isolated for food use by enzymatically removing non-protein sections from sources such as conventional rice flour. The protein content of products from these processes varies between 65 and 90%, based on parameters such as variety or cultivar of rice and degree of milling.52
Rice bran protein concentrate (RBPC) exhibits emulsifying and foaming properties that make it a promising ingredient for food proteins. RBPC has been combined with soy as a protein supplement in cookies, resulting in improved cookie quality and protein content. The addition of RBPC increased the protein content of the cookies regardless of the level of substitution.53 Rice is widely acknowledged as a hypoallergenic food and is typically one of the first solid foods introduced to infants. It is also commonly included in elimination diets for both children and adults undergoing food allergy diagnostic programs. For children with allergies to cow's milk, formulae based on rice protein offer a viable alternative. Additionally, rice protein supplements are gaining popularity in sports nutrition as a substitute for casein, whey, and soy proteins that are typically used.54
2.2.4. Oat protein.
Avena sativa, commonly known as oats, is a highly sought cereal grain for human consumption. Recently, their exceptional health-related benefits have sparked greater interest in their consumption.55 Compared to other major cereal grains, oat groats have a relatively higher protein content, ranging from 15 to 20%. Oat proteins consist mainly of globulins (70–80%), with smaller amounts of albumins (1–12%), prolamins (4–15%), and glutenin.56
The digestibility of proteins derived from oats is quite high, ranging from 90.3 to 94.2%, with a biological value of 74.5–79.6% and a net protein utilization rate of 69.1–72.4. Additionally, the oat protein efficiency ratio is comparable to that of casein, ranging from 2.25 to 2.38. When assessing protein quality, the protein digestibility-corrected amino acid score (PDCAAS) is a crucial factor. Oat protein has a higher PDCAAS value than wheat and almond proteins, although it is lower than that of soy and pea proteins. Furthermore, compared to most cereals, oat protein has a relatively superior amino acid profile, with a high content of lysine and threonine, making it more nutritious than other cereal grains.57
Oat protein isolates exhibit favorable emulsifying activity and binding properties. Given their amino acid composition, oat protein isolates have the potential to create value-added products for the food industry.58
As oat protein is free of gluten, it is safe for individuals with celiac disease to consume oat protein-based products. However, solid products such as bakery items, cereal bars, and meat substitutes, as well as semi-solid products like yogurt, and liquid products such as milk and beverages made exclusively from oat flour tend to have low protein content due to the low protein content of oat flour overall. To create oat-based products as an alternative to meat substitutes, dairy, and bakery products that have a similar protein content or texture, additional protein must be added. Oat protein isolates and concentrates may provide an additional source of oat protein.57
2.3. Oilseed protein
Oilseeds are seeds and fruits that are rich in oils and other dietary fats. These oilseeds are also a good source of calories and protein. Cold-pressed oil production involves pressing the oil from the seeds to create a protein- and fiber-rich by-product, cake, which is characterized by an abundant composition of oils and a high protein content. It also includes balanced amounts of carbohydrates, lipids, and proteins. By applying the right technology, oil can be extracted from seeds, which can then be utilized to make biodiesel. This meal can be improved and can provide nourishment for people or animals. When the seeds are first roasted before the oil is pressed and organic solvents are used to extract the oil from the seeds, the finished product is called a post-extraction meal. Meal and oil cake are used as part of cattle feed.59,60
Efficient oil seed processing can be achieved by developing and implementing innovative technologies that provide environmentally sustainable agricultural and agri-industrial solutions, with applications in the food and pharmaceutical industries, from the industrial by-products of oilseed processing.59 The protein content of defatted oilseed meal from dehulled oilseeds is 35–60%, which varies depending on the seed (Table 2).
Table 2 Amino acid composition of oilseed protein (g/100 g protein)
Oilseed protein |
Amino acids |
Ref. |
Ile |
Leu |
Lys |
Met |
Cys |
Phe |
Tyr |
Thr |
Trp |
Val |
Flaxseed |
0.87 |
1.18 |
0.75 |
0.32 |
0.32 |
0.95 |
0.53 |
0.72 |
0.30 |
1.07 |
60
|
Rapeseed |
1.25 |
2.51 |
2.04 |
0.47 |
0.59 |
1.44 |
0.99 |
1.59 |
0.43 |
1.55 |
Chia seed |
0.73 |
1.35 |
0.98 |
0.80 |
0.53 |
1.10 |
0.58 |
0.76 |
0.79 |
0.93 |
Sunflower seed |
0.92 |
1.40 |
0.86 |
0.53 |
0.38 |
1.05 |
0.57 |
0.81 |
0.35 |
1.11 |
Oilseed meals generally contain some anti-nutritional components such as trypsin inhibitors, oligosaccharides, phytates and tannins, and low protein solubility that may restrict their food usage. Aqueous processing whether assisted by enzymes that hydrolyze structural cell wall polysaccharides for oil extraction can further improve the nutritional and functional properties of proteins.61 Substituting animal proteins with plant-based proteins can enhance the nutritional benefits of meat products while also aligning with the desires of numerous scientists, activists, and government agencies who seek to decrease meat production for ethical and environmental reasons. Along with legume proteins, oilseed proteins are employed as alternatives to meat proteins.59
2.3.1. Peanut protein.
Peanuts (Arachis hypogaea L.) are a popular crop in China and around the globe. Since 1993, China's overall yield of peanuts has been the greatest in the world. In 2007, China exported 637.4 million kilograms of peanuts. Most peanuts cultivated in China are employed to make edible oil. The remaining oilseed meal also known as defatted peanut flour is a high-protein, inexpensive peanut industry by-product containing 50–55% high-quality protein. Despite its excellent amino acid profile, defatted peanut flour has been used in a limited number of food applications due to its poor functional properties. As a result, the development of new protein-based products, such as peanut protein concentrate from defatted peanut flour, is becoming more important as it can provide the food industry with new protein-rich (more than 70 g protein per 100 g) food ingredients for product formulation and protein enrichment. Animal protein is more costly and out of reach for a lot of people in underdeveloped nations, hence an alternative is desperately needed in many of them. Peanuts' abundant proteins make it a less expensive source of protein that is widely available.62 Peanuts (Arachis hypogeae L.) are also known as earthnut, manilanut, monkeynut, and groundnut. They are the fourth most vital supplier of vegetable cooking oil and the thirdmost extremely crucial source of vegetable protein in the world. Three outstanding evaluations on peanut protein and peanut culinary usage were published about a decade ago.63 Peanut protein concentrate was separated from fermented and unfermented defatted peanut flour using isoelectric precipitation and physical separation methods. Spray drying or vacuum drying was used to eliminate moisture from peanut protein concentrate. The proximate principles and functional attributes viz. water/oil binding ability, protein solubility, emulsifying ability, foaming ability, etc. of the peanut protein concentrate from each drying process were evaluated. Besides, the defatted peanut flour, as well as soy protein isolate, were used as the control sample.64 Peanuts are unique among the major oilseed crops in that they can be used in a variety of food forms. Peanuts may be transformed into goods using a simple roasting and grinding process. Roasting and salting are the most popular ways of consuming peanuts among the world's peanut eaters. Roasted nuts are one of the common ready-to-eat (RTE) dishes made from peanuts. In addition, peanuts are also offered in a fresh form as a vegetable, canned or frozen form, roasted along with the shell, toasted and salted, and used in over 50% of candies, cakes and other confectionary items. However, it is also ground in butter for application in over 100 recipes.63
2.3.2. Flaxseed protein.
Flaxseeds (Linum usitatissimum L.) are one of the classes of oilseeds that are rich in lignans, lipids, proteins, fiber, carbs, and micronutrients. Flaxseed has gained a reputation as a food source of high-value functional ingredients due to its well-known nutritional quotient. These key elements in flaxseeds can be made accessible for a variety of purposes, including nutraceuticals, cosmetics, and the food sector, using a variety of bioprocessing processes. However, despite their health-promoting and culinary properties, flaxseeds contain significant amounts of phytotoxic chemicals such as linatin, phytic acid, protease inhibitors, and cyanogenic glycosides.65 It is a major oilseed crop that contains nutrients such as oil, protein, dietary fiber, and lignans. Flaxseed protein is an important nutrient, accounting for about 18 to 22% of the weight of the seed based on the variety as well as origin. It is made up of dual protein fractions: one that is salt soluble and has a high molecular weight, and another that is water soluble and has a low molecular weight. Amino acids such as aspartate, leucine, glutamate and arginine are found in abundance in flaxseed protein. Flaxseed protein may provide health benefits to malnourished people and people who are allergic to milk proteins because of its amino acid profile. Allergenic studies of flaxseed have been documented for flaxseed oil, which is applied as a laxative. There are no documented clinical trials that have shown flaxseed protein to cause allergic reactions when ingested as a portion of a balanced meal.66 Usually, flaxseeds are of two common types namely brown flaxseeds and golden/yellow flaxseeds. Both types of flaxseeds have similar nutritive value and contain an equivalent amount of short-chain fatty acids. However, there is an exceptional flaxseed variety known as solin, a yellow form of flax with a completely distinct oil composition and low omega-3 fatty acid content. Brown flax is more commonly used in paints, varnishes, fiber, and cow feed.
Whole flaxseed, milled type flaxseed, roasted flaxseed, and flaxseed oil are all commercially available forms of edible flaxseed. Flaxseed is a multi-component seed consisting of bioactive plant elements such as proteins, oils, dietary fibers, soluble polysaccharides, lignans, phenolic compounds, and micronutrients viz. vitamins (A, C, and E) and minerals depending on its physicochemical composition.67 Flaxseed oil and whole seeds are the only parts of flaxseed that are used in the food business. Flaxseed protein isolate (FPI) has not been widely utilized because flaxseed mucilage interferes with protein isolation and lacks appropriate extraction procedures. According to studies, the polysaccharides present in flaxseed husks interfere with the breakdown of proteins because they swell in water/aqueous medium. Removal of these mucilage-like polysaccharides prior to extraction of protein significantly improves protein recovery and purity.
Wanasundara68 utilized polysaccharide degrading enzymes to reduce mucilage interference in the retrieved protein. Although breakthroughs in protein extraction and isolation have been made in the last 20 years, extracting flaxseed protein from flaxseed remains difficult. The high concentration of water-soluble mucilage in the hull can raise the viscosity of the mixture and obstruct subsequent protein extraction. A group of researchers69 devised a continuous and scalable wet technique for extracting and fractionating mucilage from flaxseed in 2009. This technique, however, was time intensive, necessitated a large quantity of water, and caused low protein recovery.66
2.3.3. Sunflower protein.
The production and use of sunflower have expanded in various nations in current years and many studies have been conducted on its processing, nutritional, and functional aspects, as well as food applications. Characterization studies of sunflower proteins lack detail or use chemically treated proteins. In a study, ultracentrifugation, chromatography, gel filtration, and gel electrophoresis techniques were used to fractionate and characterize the sunflower protein produced by separating the albumin-containing fraction in water, the globular fraction in total salt and protein in an alkaline solution.38 Sunflower seeds are planted mainly for their great oil yield (50 percent). Mechanical, thermal, and chemical treatments are used in the oil extraction process, which may alter the nutritive value of sunflower-based products. In most cases, oil extraction is done in two processes. The first process involves mechanically cracking the kernels (with centrifugal or pneumatic shellers or abrasion) in order to isolate the oil from sunflower seeds using screw presses or expellers.69 As a result, a sunflower deoiled cake with 15–20% oil is produced. Small farmers in developed as well as developing nations employ mechanical extraction techniques. Industrial-scale solvent extraction boosts oil output and produces a defatted sunflower seed meal byproduct in the second stage. The endosperm cell wall of sunflower seeds is completely disrupted by adding pressure followed by n-hexane extraction, which facilitates enzyme activity and thereby increases digestibility.70
3. Protein extraction techniques
In recent times, health, ethical, and/or religious priorities have increased public attention to the use of plant proteins as a preferred alternative to animal-based protein products.33 Therefore, the extraction and isolation of plant proteins are very important to meet the growing demand. However, the plant protein is strongly trapped in the cell wall of the filament and the intracellular matrix of the cotyledon, which accounts for its under-usage. Therefore, there is a need for efficient technologies capable of disrupting plant cell integrity and assisting in protein extraction.71 Traditional/conventional (water, salt, solvent, detergent, alkali) and non-traditional/non-conventional (microwave, pulsed electric field, enzyme assisted, high pressure, homogenization, ultrasound) techniques are used for extraction of proteins from plant sources (Fig. 3) (Table 3).
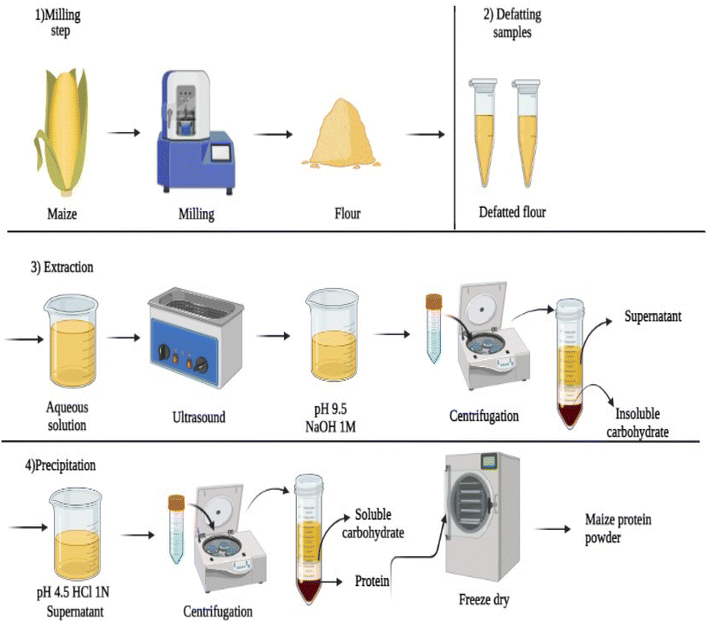 |
| Fig. 3 Methods for extraction of proteins. | |
Table 3 Different techniques for extraction of plant proteins
Types of plant protein |
Extraction techniques |
Benefits |
Ref. |
Soy protein |
•Enzyme assisted extraction |
•Protease may improve the solubility of soy proteins |
111
|
•Ultrasound-assisted extraction |
•Improvements in protein extraction yields |
•High pressure homogenization |
•Improved extraction yields from soybean processing materials |
Pea protein |
•Alkaline extraction or isoelectric precipitation |
•Simple step process |
33
|
•Salt extraction and micellization |
•Less cost |
Rice protein |
•Alkali extraction |
•Using alkali solution in rice bran protein extraction, protein yield increases with increasing pH |
112
|
•Enzymatic extraction |
Sunflower protein |
•Ultrasonic-assisted protein extraction |
•Reduce power consumption, shortened extraction time and improved protein extractability and functionality |
113
|
Pumpkin protein |
•Microwave assisted extraction |
•Extraction yield 93.95% |
114
|
•Polyethylene glycol (PEG) 200-based deep eutectic solvent (DES) concentration |
Peanut protein |
•High pressure assisted extraction |
•Peanut protein isolate extraction yield of 39.86% as compared to 16.84% yield in control |
115
|
Mushroom protein |
•PEF assisted protein extraction |
•61% increase in comparison to only pressure extraction |
116
|
Watermelon protein |
•Ultrasound-assisted extraction method |
•87% with enhanced functional properties and amino acid profile |
117
|
Conventional methods may occasionally cause reduced extraction yields because of protein degradation. Several variables, including extraction time, solvents, pH, and temperature affect the yield of extraction of protein from the source. As a result, researchers are concentrating increasingly on non-thermal green methods to improve extraction efficiency and minimize protein degradation during extraction (Table 3).
While few hazardous chemicals and solvents are used, these processes have no negative environmental effects, and the produced proteins are also safe to consume. Utilizing cutting-edge protein extraction methods can improve protein production and its nutritionally and technologically useful characteristics. But since they are economically viable, many sectors continue to use traditional approaches. However, due to economic sustainability, many industries still depend on conventional methods.72
3.1. Alkaline extraction/isoelectric precipitation technique
The alkaline extraction/isoelectric precipitation technique seems to be the most popular approach for extracting protein from plant sources because it is uncomplicated and inexpensive. Alkaline solubilization and subsequent isoelectric precipitation is a frequently used technique to recover proteins from low-value food sources. This technique is efficient, provides maximum recovery, and produces functional, stable proteins that are lipid-free.73 The alkaline extraction based isoelectric precipitation technique depends on the dissolution of proteins in an alkaline medium. Proteins are then precipitated at an isoelectric point (pH 4.5). Globulin is mainly extracted by this method because globulin and albumin have different isoelectric points.74
Previous studies have shown that the yield of isolated proteins increases with an increase in pH levels to 10.0 and above. The protein extraction capacity of pigeon pea and cowpea increased from 35.1 to 58.1% and 36.4 to 53.5%, respectively, when the extraction pH was adjusted from 8.5 to 12.5.75 In another study, it was observed that pea protein recovery increased from 49.20% to 57.56% when the alkaline extraction pH was increased from 8.5 to 9.5. However, the increase in extraction pH facilitated the formation of protein aggregates which reduced the percentage of solubility of pea protein but did not affect the secondary structure of the protein.76 Nonetheless, during protein extraction, highly alkaline conditions caused significant protein denaturation. Therefore, a balance between increased protein yields and the degree of denaturation must be taken care of. On the other hand, the increased temperature and longer extraction times help proteins to be more easily extracted. However, a rise in temperature may also bring about precipitation and thermal denaturation of proteins. Thus, room temperature or much higher temperatures are advisable for protein extraction.76–78 According to a study carried out by Tanger et al.,74 the precipitation step mainly affects the protein composition of pea protein. In a recent research on hemp protein isolate it was found that the composition of protein subunits and structural properties of proteins are greatly affected by the technique used for extraction. Alkaline extraction of hemp protein isolate resulted in a greater percentage of β-sheets (54.46%) as compared to the salt extraction method (52.65%). In contrast, the salt-extracted hemp protein isolate showed better functionality as compared to the alkaline-extracted protein. Besides, the salt extraction of hemp protein is beneficial in the context of solubility, heat stability, and emulsion activity.79
3.2. Ultrasound-assisted extraction (UAE)
The ultrasound waves applied to improve the extraction efficiency are mainly in the range of 20 to 1000 kHz. Ultrasound waves are muscle waves that travel by the target matrix through compression and are rare. The propagation/transmission of these waves leads to negative pressure in the solvent, and when the higher intensity sound wave pressure propagates through the solvent, the formation of microscopic pores or bubbles arises. When these spaces/voids or bubbles are packed with gas or water vapor, bubble growth and contraction occur until they collapse, causing cavitation.78,80 It has recently been acknowledged that UAE is an environment-friendly, clean, and significantly novel technology in the food science area. It can be used either as a pretreatment method before more traditional ones or merged with other innovative methods such as microwave-assisted techniques, vacuum-based techniques, or enzymatic extraction methods. The main goals of ultrasonic protein extraction are to minimize extraction time, energy costs, and solvent consumption, produce more homogeneous mixtures, increase energy transfer rates, reduce temperature gradients, provide selective extraction, reduce device size, and enable faster response and better process control. UAE is commonly used in this context to extract proteins from plant and animal sources, increasing extraction yield by approximately 20% compared to traditional methods. Additionally, studies have observed the influence of the UAE on the technological functional aspects of isolated proteins. Hydrogen bonds and hydrophobic interactions across proteins are impacted by ultrasound cavitation. As a result, the techno-functional characteristics are improved.81 Nevertheless, depending on the plant source, longer and stronger sonication strength may result in lower protein yields.10
However, if ultrasound is applied to support protein extraction, there are certain disadvantages such as its ability to change the structure of the protein, cause denaturation of protein and affect the protein's functional effect (reduced emulsification as well as foaming), particularly in the context of high power and prolonging the sonication time. Alternatively, amino acids can be modified with sulfhydryl and phenolic residues to develop new covalent bonds between proteins.82,83 A recent study found that sonication pretreatment did not significantly increase the hydrolysis of defatted wheat germ protein. Changes in protein structure were recorded after sonication pretreatment, demonstrating that ultrasonication degrades proteins and affects the exposure of hydrophobic amino acid residues in proteins.78
Despite protein extraction, some studies showed that high-intensity ultrasound technology successfully reduced the allergenic potential of soy protein isolates by 18.9%. Usually, the allergenic potential of the soy protein can be minimized by using procedures that modify the structure of allergens, causing them to become less accessible to antibody receptors.84 Ultrasound can induce modifications in the physical and chemical properties of proteins, including changes in their secondary and tertiary structure, particle size, solubility, hydrophobicity, emulsifying and foaming properties, water absorption capacity (WAC), oil absorption capacity (OAC), viscosity, and gelation. An improvement in the solubility of vegetable proteins may present promising opportunities for the food industry as this can positively affect other functional properties. Soy protein is the most widely studied regarding ultrasound extraction, while there is a lack of research on the use of ultrasound for extracting proteins from other vegetable sources. Additionally, possible negative effects of ultrasound extraction on the quality of extracted proteins must be investigated to ensure their suitability as raw materials or food ingredients.81
3.3. Enzyme-assisted extraction (EAE)
EAE is a reliable method for economically recuperating good quality plant-based proteins and increasing protein extraction yield. In this process, a rigid plant cell wall behaves as a hindrance to the cell's protein extraction. Essentially, EAE focuses on disrupting cell wall integrity by the enzymatic breaking of components of the cellular wall (hemicellulose, cellulose, and pectin).85 It provides different enzymes such as protease for the extraction to boost the yield of proteins extracted and minimize protein degradation. Protease increases the yield of protein by separating protein from the polysaccharidal membrane matrix. Cell wall disruption facilitates the release of cellular proteins. After releasing these proteins, proteases break down high molecular weight proteins into smaller, more soluble fractions, creating favorable extraction conditions. Additionally, proteases work at an optimal pH to avoid protein denaturation. A typical dose of 1–5% g of protease or ml enzyme per g substrate dose is considered optimal for the extraction process. Furthermore, the use of enzymes can also prevent the formation of complexes between released proteins and various cellular substances such as carbohydrates and phytic acid under precise physiological conditions.86
Protease works at ideal pH to prevent protein denaturation. For many extraction techniques, a typical concentration of 1–5% g or mL of enzyme per g protease substrate is optimal. Under specific physiological conditions, these enzymes can also prevent the formation of complexes between the produced proteins and various cell-based components such as carbohydrates and phytates.10,75 Furthermore, the combination of EAE with mechanical treatments such as ultrasound and microwave improves yield as well as the quality of protein extracts. Commercially available protein concentrates usually consist of proteins liquefied in an aqueous environment. Hence, water is the preferred solvent to improve extraction yield in enzyme-assisted aqueous protein extraction.10
3.4. Microwave-assisted extraction (MAE)
Microwave-assisted extraction (MAE) is the other non-conventional/green method of protein extraction.10 Generally, it is the most commonly utilized technique for solid-liquid-based extraction in microwave treatment because of its effectiveness, practicality, and affordability. Less requirement of solvent and minimum extraction time are the major advantages of this technology. The process produces a lot of thermal energy, which degrades heat-labile bioactive chemicals and renders them ineffective for extracting proteins. To effectively extract the plant proteins, another option is to use short microwave pulses or to optimize the microwave's input characteristics.10,77
Microwaves are typically non-ionizing electromagnetic radiation with frequencies in the range of 300 MHz to 300 GHz. Microwaves heat the sample through the interaction of dipole rotation and ionic conduction, breaking the hydrogen bonds present in the cell walls of the plant matrix. This reaction increases cell wall porosity and facilitates solvent penetration into the cell, allowing the effective release of intracellular plant constituents into the solvent system.7,87
MAE has numerous benefits over conventional thermal protein extraction methods, such as uniform flow of heat, faster extraction rate, reduced solvent consumption, and shorter extraction time.88 Minimal time of extraction and low solvent requirements are the main advantages of the microwave method. The continued use of microwaves with different physical or biochemical methods can enhance the efficiency of protein extraction. Based on the scientific literature, researchers recommend using microwaves to extract proteins from biological samples with hard structures that are difficult to digest by enzymes and ultrasound.10 A study done by Choi et al.89 showed an around 58% upsurge in protein yield from soybean using microwave heating at a frequency, temperature, time, and water to solid ratio of 2450 MHz, 60.1 °C, 30 min and 12.6 mL g−1, respectively in comparison to the hot water extraction process. Furthermore, Phongthai et al.77 reported 1.54-fold greater protein yield by microwave extraction at a power of 1000 W, time of 90 s, and solid-to-liquid ratio of 0.89 g rice bran/10 mL of distilled water as compared to the alkaline extraction process. The possible benefits of using microwave-assisted extraction (MAE) to extract soymilk from soybeans have been explored. Compared to traditional extraction methods, MAE resulted in a significant increase (p < 0.05) in both the extraction yield (24%) and protein content (44.4%) of the soymilk. In addition, the protein characteristics of the extracted soymilk, such as protein solubility and digestibility, also exhibited significant improvement (p < 0.05) over traditional methods.90
3.5. Pulsed electric field (PEF)-assisted extraction
Pulsed electric field processing (PEF) is a completely new technique that does not use heat to extract proteins from plant membranes. This new protein extraction technique involves exposing the plant material to a number of intense pulses of electric fields between 10 and 80 kV cm−1 for short periods of time from a few microseconds to several milliseconds. During this process, a vegetative matrix is received between the two electrodes, and a transmembrane voltage is generated across the cell membrane, which depends on the electric field strength, the radius of the cell, and the position of the membrane compared to the direction vector of the electric field.91
Basically, PEF is used for the preservation of food, inactivation of microbes and enzymes, chemical reaction enhancement, and protein extraction from cellular membranes. Utilizing rapeseed biomass such as stems to extract proteins as well as polyphenols making use of a hydraulic press magnified the yield of protein from the extracted juice after PEF pretreatment in comparison to the untreated sample.92 On a comparative note, this approach is less effective than other non-traditional ways of producing higher protein yields. More proteins are recovered during PEF extraction at low temperatures, longer pulse durations, and stronger electric fields. To extract proteins in their natural condition, it is further necessary to modify the PEF input variables. In comparison to traditional thermal treatments, it is a promising method since the quality of the protein is mostly unaffected both during processing and storage time.10
4. Application of vegetable proteins in the food sector
Proteins including texture proteins, concentrates, isolates, or hydrolysates are a group of food ingredients with many applications in the food industry, especially those of plant origin.81 The variety of plant protein sources provides the food industry with a wide variety of proteins to choose from to achieve desired structural, organoleptic, and nutritional properties. Plant proteins have been used for a variety of reasons, including providing the specific essential properties and structural basis to the products to which they are added, in addition to their apparent nutritional value in food products. Proteins are often expected to offer physical stability and attractive usability properties such as desired texture, consistency or viscosity when it comes to desirable properties provided to food systems.6 Microbial transglutaminase is used to texture and modify vegetable proteins for food applications. For several years now, microbial transglutaminase (MTG) isolated from Streptomyces mobaraensis has been marketed. MTG induces intramolecular and intermolecular cross-links between glutamine-carboxyl amide groups and lysine-amino groups in proteins. MTG is mainly used to improve softness, stability, and water-binding capacity due to its remarkable ability to improve many functional aspects of proteins. MTG's use of plant-based protein meals, including tofu, noodles, bread and pies, remains limited to the raw ingredients of soybeans and wheat. This review aims to demonstrate the true potential of MTG in plant protein food processing to open new doors for the application of MTG. New plant protein sources suitable for cross-linking with MTG have received special attention.93
There is a combination of existing and alternative technologies to promote oilseed and pulse proteins in food applications. The constant growth of the world's population leads to an increase in the total demand for protein, which is mainly met by plant sources. Existing and new wet and dry fractionation methods will have to be integrated to better value the vegetable protein fractions of legumes and oilseeds to meet these global nutritional needs. The intrinsic properties of soy protein, coupled with ongoing R&D efforts since the mid-20th century, have contributed to the worldwide success of soy protein isolates.
Accordingly, soybean protein can be used to develop protein isolates. Besides, a variety of legumes and oilseed meal, such as rapeseed, have been recognized as a novel food protein. Legumes like mung bean seeds contain approximately 20.97–31.32% protein, while soybeans and kidney beans have protein contents of 18–22% and 20–30%, respectively.38 The effective functional and thermal mungbean peptide properties can be applied to enhance functionality in food processing applications. For instance, the emulsifying properties of proteins help stabilize emulsions, beverages, or foams to extend the shelf life of foods.94 In addition to the high level of protein content, the antioxidant activity of kidney bean hydrolysate was observed to be greater than that of ascorbic acid, which is used commercially in the food sector. The digestate was able to inhibit oxidant development in yogurt at a usage rate of 3 g L−1 for the period of storage at ambient room temperature for 3 days.95
Agribusinesses and universities need to merge their skills to improve the supply of plant-based protein. However to explore the possibilities of usage of legumes and oil-based proteins, innovative and determined R&D projects must be initiated, especially (i) the development of oil extraction processes that preserve the natural structure of proteins; (ii) development of new protein extraction processes from the laboratory to the industrial pilot scale; (iii) production of plant protein isolates with foaming, emulsifying or gelling functions equivalent to those of animals; and (iv) generation of highly digestible hydrolyzed proteins. It is also essential to initiate research programs to innovate in the wet and dry fractions of plants or to design in vitro models to analyze the digestibility and allergenicity of proteins.96
For more than 25 years, membrane technology has been an important tool in food processing. The food industry accounts for a significant portion of the global film industry's revenue. The dairy industry (whey protein concentration, milk protein standardization, etc.) is the most common application of membrane operation, followed by beverages (wine, beer, fruit juices, etc.) from eggs. Among the many industrial-scale applications, several major separation methods represent the latest breakthroughs in food processing. Microfiltration or ultrafiltration of fruit, vegetable and sugary juices can simplify flows, make the process cleaner and increase the quality of the final product. Vegetable proteins can be converted into beverages by enzymatic hydrolysis and selective ultrafiltration. The maturation and fermentation tank bottom recovery has been used industrially in the beer industry. Microfiltration membranes have made significant progress in raw beer clarification over the past decade, which is the biggest challenge in technology. Cascade microfiltration (pore diameter 0.2 mm) is an electrolytic method used in the wine industry to ensure clarity, microbiological stability and tartar. Bacterial removal and globule fat fractionation from milk using microfiltration for the production of drinking milk and cheese milk have been described in the dairy sector.97
Cereal proteins are utilized for a number of reasons in the food industry. Wheat is a commonly consumed cereal grain that contains gluten protein which is used for its excellent viscoelastic activity.46 Additional wheat proteins are less commonly utilized as a base for making meat substitutes. Rice is also utilized for enhancing the firmness of meat-based foods such as sausages while increasing consumer acceptance.98 Some pseudocereals are also rich sources of proteins. Amaranth, a pseudocereal containing high protein content of 14%, is effectively applied as a binder in the formulation of sausages. Quinoa is another pseudocereal utilized in meat-based foods.99 It contains about 8% protein; quinoa is highly nutritious and contains all nine essential amino acids. Quinoa is used in meat-based foods for improving their water retention properties and decreasing toughness, which had a positive impact on the organoleptic attributes of meat-based foods.100
With the advanced research, several oilseeds are utilized as a protein source in the food sector. Both the seeds and meal of the oilseed contain good amounts of protein with balanced content of essential and sulfur-containing amino acids. Usually, the oil plants that are utilized as sources of protein include soybeans, flaxseed, pumpkin seeds, chia seeds, milk thistle, hemp seed, sesame, cottonseed, nigella seeds, safflower seeds, evening primrose etc.101 Additionally, the proteins obtained from oilseeds add to the functional attributes when combined with specific foods. This relates to the viscosity, whipping ability, emulsifying ability, and water–oil binding ability. Among the oilseed proteins, soybean protein has high emulsifying ability. The emulsifying ability of protein obtained from thermally treated oilseed equals and is sometimes considered superior to that of animal-based proteins.102,103
5. Challenges of vegetable proteins in the food sector
The food industry is increasingly searching for plant protein ingredients that are acceptable to consumers and can replace animal-derived proteins.104 Plant-based protein is commonly acknowledged to have lower nutritional value compared to animal protein. This is due to differences in their amino acid composition, digestibility, and capacity to convey essential nutrients like calcium and iron. Furthermore, animal proteins' technological attributes, like its ability to gel, emulsify, and foam, which contributes to the texture and sensory qualities of food, are generally deemed to be better than those of plant-based protein.105 The factors that impact the digestibility of protein can be divided into two main groups: exogenous factors, such as the presence of antinutritional factors, and endogenous factors, including cross-linking, hydrophobicity, and changes in protein secondary structure. Heat treatments can help reduce the content of certain compounds that are heat labile, thus improving the nutritional value of plant protein.106
The plant protein field faces two significant obstacles: finding sources with nutritional value comparable to animal-based proteins and developing innovative food processing methods to improve the nutritional quality of conventional plant protein sources. The ultimate goal is to create tasty, nutritious, healthy, affordable, and convenient alternative protein products that appeal to consumers in terms of cultural and sensory attributes such as appearance, taste, texture, and flavor.106 Plant proteins are often limited in their use in food products due to their restricted physicochemical properties. For instance, their low solubility makes it challenging to include them in beverages, and their tendency to be compacted, aggregated, and inflexible restricts their ability to stabilize interfaces.105 To improve the functionality of plant proteins, various methods can be used, including enzymatic modification and physical food processing technologies like extrusion, high-pressure processing, and high-power ultrasound treatment. By controlling the hydrolysis conditions, limited hydrolysis of proteins can be achieved using protease enzymes, which catalyze the hydrolytic break at peptide linkages and produce smaller peptide units that are more water-soluble and have increased amphiphilicity. Commercially, extrusion technology is used to create fibrous structures from soy proteins, gluten, and mixtures of plant proteins. During the extrusion process, the alignment of protein molecules allows them to form thin filaments or microfibrils that combine to create a macroscopic fibrous structure. When the fibrous strands are hydrated, they give a laminated, fleshy appearance that resembles the texture of meat.107
6. Conclusion
Plant proteins are an economical and versatile alternative to animal proteins because of their eco-friendly nature. Nowadays, leguminous, cereal and oilseed proteins are utilized as a source of plant-based proteins. Plant protein-based food products rich in plant protein help in body and tissue development for those who follow a vegetarian dietary regime. In addition, due to the growing consumer awareness of the shortcomings of animal proteins, today's research is engrossed in the cost-effective utilization of plant proteins so that they can be better applied. For effective use of plant proteins, energy-efficient protein extraction techniques are required. Scientists today are aiming at non-thermal green technologies (microwave, ultrasound, pulsed electric field, enzyme assisted etc.) to improve extraction efficiency and reduce proteolysis during extraction. These novel techniques have no detrimental impact on the ecological system and the resulting proteins are safe for human use with only small quantities of toxic chemicals and solvents being used. Moreover, not only are food products being developed by using plant proteins, but edible and biodegradable packaging materials are also being developed using protein.
Author contributions
PC conceptualized this work, and SS and ASC wrote the manuscript. Furthermore, PC reviewed the manuscript. All the authors have read and agreed to the published version of the manuscript.
Conflicts of interest
The authors declare that they have no known competing financial interests or personal relationships that could appear to influence the reported work.
References
- Y. Abdushaeva, The problem of vegetable protein and its solutions, IOP Conf. Ser.: Earth Environ. Sci., 2020, 613, 1–5 Search PubMed
.
- D. Lin, W. Lu, A. L. Kelly, L. Zhang and B. Zheng, Trends in Food Science & Technology Interactions of vegetable proteins with other polymers: structure- function relationships and applications in the food industry, Trends Food Sci. Technol., 2017, 68, 130–144 CrossRef CAS
.
- A. G. A. Sá, Y. M. F. Moreno and B. A. M. Carciofi, Plant proteins as high-quality nutritional source for human diet, Trends Food Sci. Technol., 2020, 97, 170–184 CrossRef
.
-
F. Mariotti, 35 – Plant Protein, Animal Protein, and Protein Quality, Elsevier, 2017 Search PubMed
.
- J. Nehete, R. Bhambar, M. Narkhede and S. Gawali, Natural proteins: sources, isolation, characterization and applications, Pharmacogn. Rev., 2013, 7, 107–116 CrossRef PubMed
.
-
S. González-Pérez and J. B. Arellano, Vegetable protein isolates, in, Handbook of hydrocolloids, Woodhead Publishing, 2009, pp. 383–419 Search PubMed
.
- M. Kumar, M. Tomar, S. Punia, J. Dhakane-Lad, S. Dhumal, S. Changan, M. Senapathy, M. K. Berwal, V. Sampathrajan and A. A. S. Sayed,
et al., Plant-based proteins and their multifaceted industrial applications, LWT, 2022, 154, 112620 CrossRef CAS
.
- A. Nesterenko, I. Alric, F. Violleau, F. Silvestre and V. Durrieu, The effect of vegetable protein modifications on the microencapsulation process, Food Hydrocolloids, 2014, 41, 95–102 CrossRef CAS
.
-
S. González-Pérez, and J. B. Arellano, Vegetable protein isolates, in Handbook of Hydrocolloids, Elsevier, 2009, pp. 383–419 Search PubMed
.
- M. Kumar, M. Tomar, J. Potkule, R. Verma, S. Punia, A. Mahapatra and J. F. Kennedy, Advances in the plant protein extraction: Mechanism and recommendations, Food Hydrocolloids, 2021, 115, 106595 CrossRef CAS
.
- J. Aschemann-Witzel, R. F. Gantriis, P. Fraga and F. J. Perez-Cueto, Plant-based food and protein trend from a business perspective: Markets, consumers, and the challenges and opportunities in the future, Crit. Rev. Food Sci. Nutr., 2021, 61(18), 3119–3128 CrossRef CAS PubMed
.
- A. G. A. Sá, Y. M. F. Moreno and B. A. M. Carciofi, Plant proteins as high-quality nutritional source for human diet, Trends Food Sci. Technol., 2020, 97, 170–184 CrossRef
.
- C. Graça, A. Raymundo and I. de Sousa, Rheology changes in oil-in-water emulsions stabilized by a complex system of animal and vegetable proteins induced by thermal processing, LWT, 2016, 74, 263–270 CrossRef
.
- T. Zhang, Y. Li, M. Miao and B. Jiang, Purification and characterisation of a new antioxidant peptide from chickpea (Cicer arietium L.) protein hydrolysates, Food Chem., 2011, 128(1), 28–33 CrossRef CAS PubMed
.
- A. Nesterenko, I. Alric, F. Silvestre and V. Durrieu, Vegetable proteins in microencapsulation: A review of recent interventions and their effectiveness, Ind. Crops Prod., 2013, 42, 469–479 CrossRef CAS
.
-
O. E. Mäkinen, N. Sozer, D. Ercili-Cura and K. Poutanen, Protein from oat: structure, processes, functionality, and nutrition, in Sustainable Protein Sources, Academic Press, 2017, pp. 105–119 Search PubMed
.
- M. A. Asgar, A. Fazilah, N. Huda, R. Bhat and A. A. Karim, Nonmeat protein alternatives as meat extenders and meat analogs, Compr. Rev. Food Sci. Food Saf., 2010, 9(5), 513–529 CrossRef CAS PubMed
.
- M. C. Temba, P. B. Njobeh, O. A. Adebo, A. O. Olugbile and E. Kayitesi, The role of compositing cereals with legumes to alleviate protein energy malnutrition in Africa, Int. J. Food Sci. Technol., 2016, 51(3), 543–554 CrossRef CAS
.
- K. Kotecka-Majchrzak, A. Sumara, E. Fornal and M. Montowska, Oilseed proteins–properties and application as a food ingredient, Trends Food Sci. Technol., 2020, 106, 160–170 CrossRef CAS
.
- M. M. N. Qayyum, M. S. Butt, F. M. Anjum and H. Nawaz, Composition analysis of some selected legumes for protein isolates recovery, J. Anim. Plant Sci., 2012, 22, 1156–1162 CAS
.
- M. C. Vaz Patto, R. Amarowicz, A. N. A. Aryee, J. I. Boye, H. J. Chung, M. A. Martín-Cabrejas and C. Domoney, Achievements and Challenges in Improving the Nutritional Quality of Food Legumes, Crit. Rev. Plant Sci., 2015, 34, 105–143 CrossRef CAS
.
- G. S. Gilani, C. W. Xiao and K. A. Cockell, Impact of antinutritional factors in food proteins on the digestibility of protein and the bioavailability of amino acids and on protein quality, Br. J. Nutr., 2012, 108(S2), S315–S332 CrossRef PubMed
.
- R. Kamboj and V. Nanda, Proximate composition, nutritional profile and health benefits of legumes – A review, Legume Res., 2018, 41, 325–332 Search PubMed
.
- K. Shevkani, N. Singh, A. Kaur and J. C. Rana, Structural and functional characterization of kidney bean and field pea protein isolates: a comparative study, Food Hydrocolloids, 2015, 43, 679–689 CrossRef CAS
.
-
M. Thrane, P. V. Paulsen, M. W. Orcutt and T. M. Krieger, Soy Protein: Impacts, Production, and Applications, Elsevier Inc., 2017 Search PubMed
.
- T. J. Ashaolu, Applications of soy protein hydrolysates in the emerging functional foods: a review, Int. J. Food Sci. Technol., 2020, 55, 421–428 CrossRef CAS
.
- P. Singh, R. Kumar, S. N. Sabapathy and A. S. Bawa, Functional and edible uses of soy protein products, Compr. Rev. Food Sci. Food Saf., 2008, 7, 14–28 CrossRef CAS
.
- T. Zhang, W. Dou, X. Zhang, Y. Zhao, Y. Zhang, L. Jiang and X. Sui, The development history and recent updates on soy protein-based meat alternatives, Trends Food Sci. Technol., 2021, 109, 702–710 CrossRef CAS
.
- O. P. Malav, S. Talukder, P. Gokulakrishnan and S. Chand, Meat Analog: A Review, Crit. Rev. Food Sci. Nutr., 2015, 55, 1241–1245 CrossRef CAS PubMed
.
- S. Kumar, Meat Analogs “Plant based alternatives to meat products: Their production technology and applications.”, Crit. Rev. Food Sci. Nutr., 2016, 8398 DOI:10.1080/10408398.2016.1196162
.
- T. G. Burger and Y. Zhang, Recent progress in the utilization of pea protein as an emulsifier for food applications, Trends Food Sci. Technol., 2019, 86, 25–33 CrossRef CAS
.
- F. Boukid, C. M. Rosell and M. Castellari, Trends in Food Science & Technology Pea protein ingredients : A mainstream ingredient to (re) formulate innovative foods and beverages, Trends Food Sci. Technol., 2021, 110, 729–742 CrossRef CAS
.
- A. C. Y. Lam, A. Can Karaca, R. T. Tyler and M. T. Nickerson, Pea protein isolates: Structure, extraction, and functionality, Food Rev. Int., 2018, 34, 126–147 CrossRef CAS
.
- Z. X. Lu, J. F. He, Y. C. Zhang and D. J. Bing, Composition, physicochemical properties of pea protein and its application in functional foods, Crit. Rev. Food Sci. Nutr., 2020, 60, 2593–2605 CrossRef CAS PubMed
.
- A. K. Stone, A. Karalash, R. T. Tyler, T. D. Warkentin and M. T. Nickerson, Functional attributes of pea protein isolates prepared using different extraction methods and cultivars, Food Res. Int., 2015, 76, 31–38 CrossRef CAS
.
- E. A. Shimelis and S. K. Rakshit, Effect of processing on antinutrients and in vitro protein digestibility of kidney bean (Phaseolus vulgaris L.) varieties grown in East Africa, Food Chem., 2007, 103, 161–172 CrossRef CAS
.
- R. Yang, L. Zhu, D. Meng, Q. Wang, K. Zhou, Z. Wang and Z. Zhou, Proteins from leguminous plants: from structure, property to the function in encapsulation/binding and delivery of bioactive compounds, Crit. Rev. Food Sci. Nutr., 2021, 1–22, DOI:10.1080/10408398.2021.1883545
.
- Z. Yi-Shen, S. Shuai and R. Fitzgerald, Mung bean proteins and peptides: Nutritional, functional and bioactive properties, Food Nutr. Res., 2018, 62, 1–11 Search PubMed
.
- M. Du, J. Xie, B. Gong, X. Xu, W. Tang, X. Li, C. Li and M. Xie, Extraction, physicochemical characteristics and functional properties of Mung bean protein, Food Hydrocolloids, 2018, 76, 131–140 CrossRef CAS
.
- M. Nadeem, M. Anjum, R. M. Amir, R. Khan, S. Hussain and M. S. Javed, An overview of anti-nutritional factors in cereal grains with special reference to wheat-A review, Pak. J. Food Sci., 2010, 20, 54–61 Search PubMed
.
- M. C. Temba, P. B. Njobeh, O. A. Adebo, A. O. Olugbile and E. Kayitesi, The role of compositing cereals with legumes to alleviate protein energy malnutrition in Africa, Int. J. Food Sci. Technol., 2016, 51, 543–554 CrossRef CAS
.
- A. Das, U. Raychaudhuri and R. Chakraborty, Cereal based functional food of Indian subcontinent: a review, J. Food Sci. Technol., 2012, 49, 665–672 CrossRef CAS
.
- A. Panghal and B. S. S. U. Khatkar, Cereal proteins and their role in food industry, Indian Food Ind., 2006, 25, 58 Search PubMed
.
- A. S. M. Saleh, P. Wang, N. Wang, S. Yang and Z. Xiao, Technologies for enhancement of bioactive components and potential health benefits of cereal and cereal-based foods: Research advances and application challenges, Crit. Rev. Food Sci. Nutr., 2019, 59(2), 207–227 CrossRef CAS
.
- A. Panghal, B. S. Khatkar and U. Singh, Cereal proteins and their role in food industry, Indian Food Ind., 2006, 25(5), 58 Search PubMed
.
- W. S. Veraverbeke, J. A. Delcour and F. Bekes, Critical Reviews in Food Science and Nutrition Wheat Protein Composition and Properties of Wheat Glutenin in Relation to Breadmaking Functionality Wheat Protein Composition and Properties of Wheat Glutenin in Relation to Breadmaking Functionality, Crit. Rev. Food Sci. Nutr., 2002, 42, 179–208 CrossRef CAS PubMed
.
- S. Asseng, P. Martre, A. Maiorano, R. P. Rötter, G. J. O'Leary, G. J. Fitzgerald, C. Girousse, R. Motzo, F. Giunta and M. A. Babar,
et al., Climate change impact and adaptation for wheat protein, Global Change Biol., 2019, 25, 155–173 CrossRef PubMed
.
- S. Gorinstein, E. Pawelzik, E. Delgado-Licon, R. Haruenkit, M. Weisz and S. Trakhtenberg, Characterisation of pseudocereal and cereal proteins by protein and amino acid analyses, J. Sci. Food Agric., 2002, 82, 886–891 CrossRef CAS
.
- J. Dong, Q. Sun and J. Y. Wang, Basic study of corn protein, zein, as a biomaterial in tissue engineering, surface morphology and biocompatibility, Biomaterials, 2004, 25, 4691–4697 CrossRef CAS PubMed
.
- R. Shukla and M. Cheryan, Zein: The industrial protein from corn, Ind. Crops Prod., 2001, 13, 171–192 CrossRef CAS
.
- L. Amagliani, J. O'Regan, A. L. Kelly and J. A. O'Mahony, Composition and protein profile analysis of rice protein ingredients, J. Food Compos. Anal., 2017, 59, 18–26 CrossRef CAS
.
- F. F. Shih and K. W. Daigle, Preparation and characterization of rice protein isolates, J. Am. Oil Chem. Soc., 2000, 77, 885–889 CrossRef CAS
.
- R. B. Yadav, B. S. Yadav and D. Chaudhary, Extraction, characterization and utilization of rice bran protein concentrate for biscuit making, Br. Food J., 2011, 113(9), 1173–1182 CrossRef
.
- L. Amagliani, J. O’Regan, A. L. Kelly and J. A. O’Mahony, The composition, extraction, functionality and applications of rice proteins: a review, Trends Food Sci. Technol., 2017, 64, 1–12 CrossRef CAS
.
- C. Klose, B. D. Schehl and E. K. Arendt, Fundamental study on protein changes taking place during malting of oats, J. Cereal Sci., 2009, 49(1), 83–91 CrossRef CAS
.
- F. Boukid, Oat proteins as emerging ingredients for food formulation: where we stand?, Eur. Food Res. Technol., 2021, 247(3), 535–544 CrossRef CAS
.
- L. Kumar, R. Sehrawat and Y. Kong, Oat proteins: A perspective on functional properties, LWT, 2021, 152, 112307 CrossRef CAS
.
- C. Klose and E. K. Arendt, Proteins in Oats; their Synthesis and Changes during Germination: A Review, Crit. Rev. Food Sci. Nutr., 2012, 52(7), 629–639 CrossRef CAS PubMed
.
- I. M. Rodrigues, J. F. J. Coelho and M. G. V. S. Carvalho, Isolation and valorisation of vegetable proteins from oilseed plants: Methods, limitations and potential, J. Food Eng., 2012, 109, 337–346 CrossRef CAS
.
- K. Kotecka-Majchrzak, A. Sumara, E. Fornal and M. Montowska, Oilseed proteins – Properties and application as a food ingredient, Trends Food Sci. Technol., 2020, 106, 160–170 CrossRef CAS
.
- A. Moure, J. Sineiro, H. Domínguez and J. C. Parajó, Functionality of oilseed protein products: a review, Food Res. Int., 2006, 39, 945–963 CrossRef CAS
.
- H. Wu, Q. Wang, T. Ma and J. Ren, Comparative studies on the functional properties of various protein concentrate preparations of peanut protein, Food Res. Int., 2009, 42, 343–348 CrossRef CAS
.
- B. Singh and U. Singh, Peanut as a source of protein for human foods, Plant Foods Hum. Nutr., 1991, 41, 165–177 CrossRef CAS PubMed
.
- J. Yu, M. Ahmedna and I. Goktepe, Peanut protein concentrate: Production and functional properties as affected by processing, Food Chem., 2007, 103, 121–129 CrossRef CAS
.
- C. K. O. Dzuvor, J. T. Taylor, C. Acquah, S. Pan and D. Agyei, Bioprocessing of functional ingredients from flaxseed, Molecules, 2018, 23, 1–18 CrossRef CAS PubMed
.
- P. Kaushik, K. Dowling, S. McKnight, C. J. Barrow, B. Wang and B. Adhikari, Preparation, characterization and functional properties of flax seed protein isolate, Food Chem., 2016, 197, 212–220 CrossRef CAS PubMed
.
- A. Goyal, V. Sharma, N. Upadhyay, S. Gill and M. Sihag, Flax and flaxseed oil: an ancient medicine & modern functional food, J. Food Sci. Technol., 2014, 51, 1633–1653 CrossRef CAS
.
- J. P. D. Wanasundara, Proteins of Brassicaceae oilseeds and their potential as a plant protein source, Crit. Rev. Food Sci. Nutr., 2011, 51, 635–677 CrossRef CAS PubMed
.
- W. Zhang, S. Xu, Z. Wang, R. Yang and R. Lu, Demucilaging and dehulling flaxseed with a wet process, LWT–Food Sci. Technol., 2009, 42, 1193–1198 CrossRef CAS
.
- Y. A. Ditta and A. J. King, Recent advances in sunflower seed meal as an alternate source of protein in broilers, World's Poult. Sci. J., 2017, 73, 527–542 CrossRef
.
- S. Melchior, S. Calligaris, G. Bisson and L. Manzocco, Understanding the impact of moderate-intensity pulsed electric fields (MIPEF) on structural and functional characteristics of pea, rice and gluten concentrates, Food Bioprocess Technol., 2020, 13, 2145–2155 CrossRef CAS
.
- M. Kumar, M. Tomar, J. Potkule, R. Verma, S. Punia, A. Mahapatra, T. Belwal, A. Dahuja, S. Joshi and M. K. Berwal,
et al., Advances in the plant protein extraction: Mechanism and recommendations, Food Hydrocolloids, 2021, 115, 106595 CrossRef CAS
.
- A. Sasidharan and V. Venugopal, Proteins and co-products from seafood processing discards: Their recovery, functional properties and applications, Waste Biomass Valorization, 2020, 11, 5647–5663 CrossRef CAS
.
- C. Tanger, J. Engel and U. Kulozik, Influence of extraction conditions on the conformational alteration of pea protein extracted from pea flour, Food Hydrocolloids, 2020, 107, 105949 CrossRef CAS
.
- M. Hadnadjev, T. Dapcevic-Hadnadjev, M. Pojic, B. Saric, A. Misan, P. Jovanov and M. Sakac, Progress in vegetable proteins isolation techniques: A review, Food Feed Res., 2017, 44, 11–21 CrossRef
.
- Z. Gao, P. Shen, Y. Lan, L. Cui, J.-B. Ohm, B. Chen and J. Rao, Effect of alkaline extraction pH on structure properties, solubility, and beany flavor of yellow pea protein isolate, Food Res. Int., 2020, 131, 109045 CrossRef CAS PubMed
.
- S. Phongthai, S. T. Lim and S. Rawdkuen, Optimization of microwave-assisted extraction of rice bran protein and its hydrolysates properties, J. Cereal Sci., 2016, 70, 146–154 CrossRef CAS
.
- A. S. Sengar, N. Thirunavookarasu, P. Choudhary, M. Naik, A. Surekha, C. K. Sunil and A. Rawson, Application of power ultrasound for plant protein extraction, modification and allergen reduction – a review, Appl. Food Res., 2022, 2, 100219 CrossRef CAS
.
- B. Fang, L. Chang, J. B. Ohm, B. Chen and J. Rao, Structural, functional properties, and volatile profile of hemp protein isolate as affected by extraction method: Alkaline extraction–isoelectric precipitation vs. salt extraction, Food Chem., 2023, 405, 135001 CrossRef CAS PubMed
.
- K. S. Ojha, R. Aznar, C. O'Donnell and B. K. Tiwari, Ultrasound technology for the extraction of biologically active molecules from plant, animal and marine sources, TrAC, Trends Anal. Chem., 2020, 122, 115663 CrossRef CAS
.
- S. Bernardi, A. L. Lupatini-Menegotto, D. L. Kalschne, É. L. Moraes Flores, P. R. S. Bittencourt, E. Colla and C. Canan, Ultrasound: a suitable technology to improve the extraction and techno-functional properties of vegetable food proteins, Plant Foods Hum. Nutr., 2021, 76, 1–11 CrossRef CAS PubMed
.
-
R. Mawson, M. Gamage, N. S. Terefe, and K. Knoerzer: Ultrasound Technologies for Food and Bioprocessing. 2011 Search PubMed
.
- Z. Zhang, J. M. Regenstein, P. Zhou and Y. Yang, Effects of high intensity ultrasound modification on physicochemical property and water in myofibrillar protein gel, Ultrason. Sonochem., 2017, 34, 960–967 CrossRef CAS PubMed
.
- H. Li, K. Zhu, H. Zhou, W. Peng and X. Guo, Comparative study of four physical approaches about allergenicity of soybean protein isolate for infant formula, Food Agric. Immunol., 2016, 27, 604–623 CrossRef CAS
.
- S. Jung, B. P. Lamsal, V. Stepien, L. A. Johnson and P. A. Murphy, Functionality of soy protein produced by enzyme-assisted extraction, J. Am. Oil Chem. Soc., 2006, 83, 71–78 CrossRef CAS
.
- F. Zhan, M. Shi, Y. Wang, B. Li and Y. Chen, Effect of freeze-drying on interaction and functional properties of pea protein isolate/soy soluble polysaccharides complexes, J. Mol. Liq., 2019, 285, 658–667 CrossRef CAS
.
- B. Kaufmann and P. Christen, Recent extraction techniques for natural products: microwave-assisted extraction and pressurised solvent extraction, Phytochem. Anal., 2002, 13, 105–113 CrossRef CAS PubMed
.
- S. Y. Lee, P. L. Show, T. C. Ling and J.-S. Chang, Single-step disruption and protein recovery from Chlorella vulgaris using ultrasonication and ionic liquid buffer aqueous solutions as extractive solvents, Biochem. Eng. J., 2017, 124, 26–35 CrossRef CAS
.
- I. L. Choi, S. J. Choi, J. K. Chun and T. W. Moon, Extraction yield of soluble protein and microstructure of soybean affected by microwave heating, J. Food Process. Preserv., 2006, 30, 407–419 CrossRef CAS
.
- T. Varghese and A. Pare, Effect of microwave assisted extraction on yield and protein characteristics of soymilk, J. Food Eng., 2019, 262, 92–99 CrossRef CAS
.
- A. Golberg, M. Sack, J. Teissie, G. Pataro, U. Pliquett, G. Saulis, T. Stefan, D. Miklavcic, E. Vorobiev and W. Frey, Energy-efficient biomass processing with pulsed electric fields for bioeconomy and sustainable development, Biotechnol. Biofuels, 2016, 9, 1–22 CrossRef PubMed
.
- X. Yu, T. Gouyo, N. Grimi, O. Bals and E. Vorobiev, Pulsed electric field pretreatment of rapeseed green biomass (stems) to enhance pressing and extractives recovery, Bioresour. Technol., 2016, 199, 194–201 CrossRef CAS PubMed
.
-
M. Dube, C. Sch, S. Neidhart and R. Carle, Texturisation and Modification of Vegetable Proteins for Food Applications Using Microbial Transglutaminase, 2007, DOI:10.1007/s00217-006-0401-2
.
- H. Liu, H. Liu, L. Yan, X. Cheng and Y. Kang, Functional properties of 8S globulin fractions from 15 mung bean (Vigna radiata (L.) Wilczek) cultivars, Int. J. Food Sci. Technol., 2015, 50(5), 1206–1214 CrossRef CAS
.
- A. Sarker, S. Chakraborty and M. Roy, Dark red kidney bean (Phaseolus vulgaris L.) protein hydrolysates inhibit the growth of oxidizing substances in plain yogurt, J. Agric. Food Res., 2020, 2, 100062 Search PubMed
.
- D. Chéreau, P. Videcoq, C. Ruffieux, L. Pichon, J. C. Motte, S. Belaid, J. Ventureira and M. Lopez, Combination of existing and alternative technologies to promote oilseeds and pulses proteins in food applications, OCL: Oilseeds Fats, Crops Lipids, 2016, 41, D406 CrossRef
.
- G. Daufin, J. P. Escudier, H. Carrére, S. Bérot, L. Fillaudeau and M. Decloux, Recent and emerging applications of membrane processes in the food and dairy industry, Food Bioprod. Process., 2001, 79, 89–102 CrossRef CAS
.
- J. Pereira, G. H. Zhou and W. G. Zhang, Effects of rice flour on emulsion stability, organoleptic characteristics and thermal rheology of emulsified sausage, J. Food Nutr. Res., 2016, 4(4), 216–222 CrossRef CAS
.
- C. A. Manassero, M. C. Añón and F. Speroni, Development of a high protein beverage based on amaranth, Plant Foods Hum. Nutr., 2020, 75, 599–607 CrossRef CAS PubMed
.
- J. Fernández-López, R. Lucas-González, M. Viuda-Martos, E. Sayas-Barberá, J. Ballester-Sánchez, C. M. Haros and J. A. Pérez-Álvarez, Chemical and technological properties of bologna-type sausages with added black quinoa wet-milling coproducts as binder replacer, Food Chem., 2020, 310, 125936 CrossRef PubMed
.
- K. Kotecka-Majchrzak, A. Sumara, E. Fornal and M. Montowska, Oilseed proteins–properties and application as a food ingredient, Trends Food Sci. Technol., 2020, 106, 160–170 CrossRef CAS
.
- G. M. Sharma, M. Su, A. U. Joshi, K. H. Roux and S. K. Sathe, Functional properties of select edible oilseed proteins, J. Agric. Food Chem., 2010, 58(9), 5457–5464 CrossRef CAS PubMed
.
- M. Singh, N. Trivedi, M. K. Enamala, C. Kuppam, P. Parikh, M. P. Nikolova and M. Chavali, Plant-based meat analogue (PBMA) as a sustainable food: a concise review, Eur. Food Res. Technol., 2021, 247, 2499–2526 CrossRef CAS
.
- T. G. Burger and Y. Zhang, Recent progress in the utilization of pea protein as an emulsifier for food applications, Trends Food Sci. Technol., 2019, 86, 25–33, DOI:10.1016/j.tifs.2019.02.007
.
- L. Day, J. A. Cakebread and S. M. Loveday, Food proteins from animals and plants: Differences in the nutritional and functional properties, Trends Food Sci. Technol., 2022, 119, 428–442, DOI:10.1016/j.tifs.2021.12.020
.
- A. G. A. Sá, Y. M. F. Moreno and B. A. M. Carciofi, Food processing for the improvement of plant proteins digestibility, Crit. Rev. Food Sci. Nutr., 2019, 1–20, DOI:10.1080/10408398.2019.1688249
.
- J. Liu, M. Klebach, M. Visser and Z. Hofman, Amino acid availability of a dairy and vegetable protein blend compared to single casein, whey, soy, and pea proteins: A double-blind, cross-over trial, Nutrients, 2019, 11, 1–13 Search PubMed
.
- M. Claessens, W. H. M. Saris and M. A. Van Baak, Glucagon and insulin responses after ingestion of different amounts of intact and hydrolysed proteins, Br. J. Nutr., 2008, 100, 61–69 CrossRef CAS PubMed
.
- S. S. Audu and M. O. Aremu, Effect of processing on chemical composition of red kidney bean (Phaseolus vulgaris L.) flour, Pak. J. Nutr., 2011, 10, 1069–1075 CrossRef CAS
.
- P. K. Dahiya, A. R. Linnemann, M. A. J. S. Van Boekel, N. Khetarpaul, R. B. Grewal and M. J. R. Nout, Mung Bean: Technological and Nutritional Potential, Crit. Rev. Food Sci. Nutr., 2015, 55, 670–688 CrossRef CAS PubMed
.
- K. E. Preece, N. Hooshyar and N. J. Zuidam, Whole soybean protein extraction processes: A review, Innovative Food Sci. Emerging Technol., 2017, 43, 163–172 CrossRef CAS
.
- C. Fabian and Y. H. Ju, A review on rice bran protein: Its properties and extraction methods, Crit. Rev. Food Sci. Nutr., 2011, 51, 816–827 CrossRef CAS PubMed
.
- M. Dabbour, H. Jiang, B. K. Mintah, H. Wahia and R. He, Ultrasonic-assisted protein extraction from sunflower meal: Kinetic modeling, functional, and structural traits, Innovative Food Sci. Emerging Technol., 2021, 74, 102824 CrossRef CAS
.
- R. L. Liu, P. Yu, X. L. Ge, X. F. Bai, X. Q. Li and Q. Fu, Establishment of an aqueous PEG 200-based deep eutectic solvent extraction and enrichment method for pumpkin (Cucurbita moschata) seed protein, Food Anal. Methods, 2017, 10, 1669–1680 CrossRef
.
- X. Dong, M. Zhao, B. A. O. Yang, X. Yang, J. Shi and Y. Jiang, Effect of high-pressure homogenization on the functional property of peanut protein, J. Food Process Eng., 2011, 34(6), 2191–2204 CrossRef CAS
.
- O. Parniakov, N. I. Lebovka, E. Van Hecke and E. Vorobiev, Pulsed electric field assisted pressure extraction and solvent extraction from mushroom (Agaricus bisporus), Food Bioprocess Technol., 2014, 7, 174–183 CrossRef CAS
.
- S. M. Gadalkar and V. K. Rathod, Extraction of watermelon seed proteins with enhanced functional properties using ultrasound, Prep. Biochem. Biotechnol., 2020, 50(2), 133–140 CrossRef CAS PubMed
.
|
This journal is © The Royal Society of Chemistry 2023 |