Utilization of button mushroom (Agaricus bisporus) powder to improve the physiochemical and functional properties of cookies
Received
18th November 2022
, Accepted 6th February 2023
First published on 24th February 2023
Abstract
The objective of the current research was to develop nutritionally enriched cookies by combining protein-rich mushroom flour (MF) with barley flour (BF). In this study, BF was substituted with various concentrations of MF (10%, 20%, 30%, 40%, and 50%) to produce nutritional gluten-free cookies. All samples were analyzed for physicochemical, color, antioxidant activity, microbiological, and sensory properties of the developed cookies. Additionally, the effect of MF on the texture, temperature, crystallinity, and microstructure of the cookies was studied. The results showed that the MF-enriched cookies had higher crude protein (T5) and ash levels than the control cookies (only BF). The spread ratio decreased and the breaking strength increased as the percentage of MF increased. The inclusion of MF significantly increased the total phenolic content and antioxidant activity of the cookies, improving their bio-functional quality. The L* value of the cookies decreased, while the a* and b* values were observed to increase significantly with the incorporation of MF. Besides this, the antimicrobial properties of the cookies increased with increasing MF concentration. However, higher sensorial properties were reported at 10% MF concentration. Thereafter, increasing the MF concentration negatively affected the sensorial properties of the cookies. The texture analysis revealed that the increasing amount of MF decreased the hardness and fracturability of the cookies. The thermal characteristics showed that the degree of gelatinization decreased with increasing MF, while some large starch granules were observed on the outer surface. In addition, the increasing MF concentration increases the crystalline formation. The study showed that MF and BF can be used as functional ingredients to develop a protein-rich confectionery product with high textural and functional quality attributes.
Button mushroom (Agaricus bisporus) is mostly consumed worldwide due to its edibility, taste, and medicinal properties. Mushrooms are one of the most delicious vegetables, and contain between 86.5 and 94.5 percent moisture. They are high in polyphenol oxidase, peroxidase, catalase, and protease, as well as all of the essential amino acids. Unlike most fresh fruits and vegetables, they lack the protective layer of suberin. Thus, they are susceptible to rapid deterioration due to moisture loss at ambient temperature. With the passage of time, their rapid rate of respiration accelerates the rate of deterioration, resulting in shriveling, loss of texture, discoloration, development of off-flavor, loss of marketable quality, and decreased consumer acceptability.1,2 Mushrooms can be used in cereal-based products to improve the nutritional quality and functionality. With the advancement of living standards and lifestyles, people do not consume foods that merely provide essential nutrients but instead prefer foods that have both functional and nutraceutical benefits.3,4 Cookies are popular sweet bakery items and globally consumed.5 Cookies may be an ideal product for meeting nutritional needs that promote health.6 Bioactive compounds are mostly used to produce novel cookies with higher nutritive value. Because of the increased demand for higher nutrition bakery items, the use of mushrooms in cookies necessitates research attention.7
Barley (Hordeum vulgare L.) is an important grain as it comprises β-glucan, B-complex vitamins, tocopherols, tocotrienols, and has higher antioxidant activity.8 It is mostly used in brewing and malting, as well as animal feed, but it is also becoming more popular as a bakery and extruded ingredient in chapattis, cookies, breads, and extruded snacks.9 Barley contains a larger level of phenolic compounds and antioxidant activity.10 Phenolic components in barley include cinnamic and benzoic acid derivatives, flavonols, quinines, proanthocyanidins, flavones, chalcones, flavanones, and amino phenolic components. The risk due to the ingestion of free radicals and oxidants can be reduced by consuming dietary phenolic components.11 Thermal-treated foods have the potential to increase or decrease phenolic chemicals and antioxidant activities.12 The barley bioactive component supplement via baked foods such as cookies could be an efficient strategy to provide the bioactive components in barley.13 Barley is rich in bioactive compounds and inexpensive source of plant protein that may fulfill the nutritional requirement of at-risk populations, particularly poor and low-income groups. In comparison to wheat and other grains, it is regarded as the most useful grain because it is easily digestible. As a high gluten content is not required for cookies, BF can be a possible raw ingredient to prepare cookies with higher bioactive value. Thus, this study aims to produce gluten-free BF cookies fortified with various concentrations of MF (10–50%). All prepared samples were analyzed to evaluate the physico-chemical, antioxidant, antimicrobial, sensory, textural, and microstructural properties of cookies enriched with different concentrations of mushroom and BF.
Experimental method
Procurement of raw material
BF (Variety-VLB 118, India), sugar (White sugar), shortening (vegetable ghee, Dalda, India), and salt were purchased from the local market of Jammu, J&K, India, while dextrose and sodium bicarbonate were procured from Sigma-Aldrich (Steinheim, Germany) and used without any modifications.
Mushroom powder
Button mushrooms (Agaricus bisporus) were purchased from a local market. Upon arrival to the laboratory, the mushrooms were sorted out for any damage. The selected mushrooms were then washed and cut into thin slices. Mushroom slices were frozen using a freezer, and kept in a freeze-drier (SP Scientific VirTis Sentry 2.0, USA) at −80 °C for 24 h and 5 mTorr (0.666 Pa) pressure until a constant weight was attained, followed by grinding by a grinder (Philips, India).14
Preparation of cookies
Cookies were developed as per AACC method.15 All ingredients, such as composite flour (BF + MF) (225 g), dextrose solution (33 ml), sugar (130 g), sodium chloride (2.1 g) shortening (64 g), sodium bicarbonate (2.5 g), and distilled water (16 ml), were mixed properly to make the dough. The dough was then sheeted to the thickness of 10 mm, and cut into round shapes of 60 mm diameter. Baking was done at 105 °C for 10 min in the baking oven (Fig. 1). The samples were then packed in plastic bags (LDPE) (low density polyethylene). The cookies were prepared using composite flour in the following ratios (Table 1).
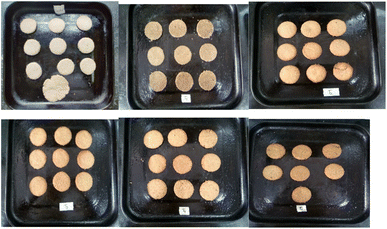 |
| Fig. 1 Development of BF cookies enriched with different concentrations of MF. | |
Table 1 Various concentrations of BF and MF are used to prepare composite flour for nutritional gluten-free cookies production
Treatments |
Barley four (%) |
Button mushroom flour (%) |
Tc (control) |
100 |
— |
T1 |
90 |
10 |
T2 |
80 |
20 |
T3 |
70 |
30 |
T4 |
60 |
40 |
T5 |
50 |
50 |
Determination of the physical characteristics of cookies
Diameter.
The diameter (D) was determined by stacking four cookies and calculating the total diameter. Cookies were then rotated by 90° and the new diameter was again calculated. The mean diameter was measured as the final diameter of the cookies.
Weight.
The weight of the cookies was measured as per the method given by the AACC method.15 The weight in grams was calculated using a weighing balance (Wensar, PGB 1030, India).
Thickness.
The total thickness (T) was measured by stacking four cookies. After restacking them in varying order, the height was calculated again and the mean height was taken as the final thickness of the cookies.
Spread ratio (D/T).
The spread ratio was measured by taking the ratio of the diameter and thickness of cookies.
Breaking strength.
The breaking strength was measured as per the technique mentioned by Okaka and Isieh.16 Cookies were kept between 2 parallel metal plates and weight was applied until the cookies snapped. The minimum weight necessary to break the cookies was measured as the breaking strength of the prepared cookies.
Proximate composition of the prepared cookies
The moisture, crude protein, fat, ash, crude fibre, total carbohydrate and energy content of the prepared sample were determined according to the AOAC methods.17
Water activity (wa).
The instrument (Aqua lab Pre, Decagon Device, USA) was first calibrated, followed by putting the sample (3/4th) in the water activity meter cup until a constant reading was attained.
Hunter color value
The color was evaluated using parameters such as L*, a*, and b* values, where L* indicates the lightness or whiteness, a* represents the redness or greenness, and b* indicates the yellowness or blueness. The instrument was calibrated with a standard black reference, followed by white tile. The sample was put in a handling dish and kept on an analyzing port to record L*, a*, and b* values.
Bioactive properties of developed cookies
Total phenolic content (TPC).
The TPC of the samples was calculated by the Folin–Ciocalteu method given by Xiao et al.18 TPC values were determined using the gallic acid standard curve, and the obtained absorbance values were represented as mg of gallic acid equivalents (GAE) per gram of the dry weight of the cookie.
DPPH radical scavenging activity.
The DPPH (2,2-diphenyl-1-picrylhydrazyl) radical scavenging activity was calculated as per the method given by Liu et al.19 The extract (0.2 ml) was uniformly dissolved with 3.8 ml DPPH solution (0.1 mM DPPH dissolved in methanol). The prepared solution was placed under dark conditions for incubation at ambient temperature for 30 minutes. The sample absorbance was recorded at 517 nm using a UV-visible spectrophotometer. The DPPH radical scavenging activity was measured using the following given formula (eqn (1)): | (% DPPH radical scavenging) = (Ac – A)/Ac × 100 | (1) |
where Ac = absorbance of the control, A = absorbance of the sample.
The IC50 value (sample extract concentration necessary to inhibit 50% DPPH activity) was obtained from the graph plotted between each concentration and percentage inhibition.
Reducing power activity assay.
The reducing power of the cookie samples was measured by the procedure given by Xiao et al.18 The standard curve was drawn at various concentrations of ascorbic acid. The reducing power was represented as μg ascorbic acid per g dry weight of the cookie.
Sensory evaluation
All cookie samples were evaluated by 25–30 semi-trained panelists aged between 25 to 45 years-old for its color, taste, texture, and overall acceptability using the 9-point hedonic rating, where 9 represented ‘extremely like’ and 1 represented ‘extremely dislike’.
Microbial analysis (total plate count)
The microbial growth was evaluated using the spread plate technique, given by Pelczar and Chan.20 A 1 g sample was put into a test tube with 9 ml of sterile water and mixed uniformly. A 1 ml volume of the mixture was then again put into a test tube having 9 ml sterile water for further dilution. The procedure was continued until 6th diluents (10−6). Potato dextrose agar was inoculated with 0.1 ml of the diluted sample (10−6) and incubated for 24 h at 37 °C. Colonies were then counted and multiplied by the dilution factor, as per given formula (eqn (2)). | 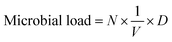 | (2) |
where N = no. of the colonies counted, V = volume of the inoculum, D = dilution factor.
Texture analysis of cookies
The texture of the cookies was checked by determining the peak breaking force (N) by a texture analyzer (TA HD-plus, Stable Micro Systems Ltd, Surrey, UK). A three-point bending rig probe was used. The cookie was kept on a loading cell and compressed. The process parameters on the texture analyzer were as follows: test speed of 2 mm s−1, pretest speed of 1.0 mm s−1, and post-test speed of 10.0 mm s−1 with a distance of 3 mm. It was represented as Newton (N) for force and Joule (J) for energy. The force time plots were measured for peak breaking force (N).21
Differential scanning calorimetry
The thermal properties of the sample were estimated by a differential scanning calorimeter (DSC-7, PerkinElmer, and Norwalk, CT) using the method described by Gonzalez et al.22 A 2 g sample was put into an aluminum pan, and mixed with distilled water using a Hamilton micro syringe to attain an aqueous suspension having 70% water, then sealed and equilibrated for 1 hour at ambient temperature before analysis. The instrument was calibrated with indium, and an empty aluminum pan was kept as a reference. The sample pan was then heated at the rate of 10 °C min−1 from 20–160 °C. The parameters, including onset (To), peak (Tp), and conclusion temperatures (Tc), and the enthalpy of gelatinization (ΔH) were recorded automatically by the software of the instrument.
Scanning electron microscopy (SEM)
The cookie samples were split crosswise using a blade. The cross-sections were then mounted on specimen holders using colloidal graphite, sputter-coated with the gold, and recorded at a magnification of 2000× using a scanning electron microscope (Make: Jeol, Model: JSM7610F-plus). The number and size of pores in the samples were compared after dehydration. SEM images were then processed by MATLAB 7.0 image analysis software (MathWorks, USA).
Structural characterization by X-ray diffraction
The X-ray diffraction (XRD) patterns of the cookie samples were prepared using an X-ray diffractometer (Panalytical, The Netherlands) fitted with Cu-Kα radiation at a wavelength of 1.5406 Å. The recordings were observed at ambient temperature with 0.016° step size, 20 s scanning rate per step, and 10 to 100° (2θ° range) diffraction angle range, where θ represents the angle of incidence of the X-ray beam on each sample. The diffraction pattern was analyzed using EVA software.
Statistical analysis
The samples were made at different concentrations and analyzed in triplicate. The obtained results are shown in the form of means and standard deviations. The significant difference between the samples was analyzed by post hoc ANOVA. All samples were statistically analyzed using SPSS software. The various statistical parameters such as the root mean square error (RMSE), coefficient of determination (R2), and chi-square (χ2) values were estimated using eqn (3)–(5). | 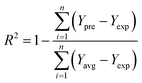 | (3) |
| 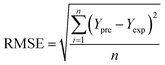 | (4) |
| 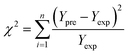 | (5) |
where Yexp is the observed value or experimental values, Ypre is the predicted value from the model, Yavg is the average response value, and n is the number of experiments.
Results and discussion
Physical characteristics of cookies
Diameter.
A higher cookie diameter is considered as one of the desirable quality parameters.23 The data pertaining to the diameter of the button mushroom and BF based cookies in Table 2 indicated that the diameter of the prepared cookies increased with the addition of mushroom powder and decrease in BF concentration. The lower diameter of 53.65 mm was observed in treatment Tc (control), followed by diameters of 54.30, 54.89, 55.27 and 56.45 mm in treatments T1, T2, T3 and T4, respectively. Meanwhile, the higher diameter of 58.63 mm was found in treatment T5. The diameter of the cookies increased significantly with the incorporation of button mushroom and BF, which might be due to the quantity of fat added to the flour during preparation. The increasing pattern in the diameter of the cookies with increasing mushroom powder (plant material substitution level) concentration was also explained by Chauhan et al.,24 who found that the diameter and protein content have an inverse correlation. The glass transition of gluten protein may occur during the heat treatment of flour. Thus, the increasing mobility allows it to relate and results in the formation of protein matrix in the form of web like structure. Kumar and Barmanray25 also observed an increase in diameter for button mushroom-fortified biscuits. Similarly, Abdul et al.26 found an increase in diameter for cookies with decreasing concentration of oat bran and vice versa. Bello et al.27 also observed that with an increase in the substitution levels of mushroom powder, the diameter of the biscuits increased. Similar results were also found by Giwa and Ikujenlola,28 who stated that increasing the concentration of quality protein maize increased the diameter of biscuits. Abou-Zaid et al.29 and Dhalagade et al.30 also found the increase in diameter of biscuits and cookies with an increase in MF concentration, respectively.
Table 2 Physical and color properties of button mushroom and BF-based cookiesa
Treatments (BF : BMP) |
Diameter (mm) |
Weight (g) |
Thickness (mm) |
Spread ratio |
Breaking strength (g) |
L* |
a* |
b* |
BF: barley flour, BMP: button mushroom powder. Different letters (a, b, c, d, e) show the significantly different means of observed data (p ≤ 0.05), according to the post hoc Tukey's HSD test.
|
Tc (100 : 0) |
53.65 ± 0.02a |
16.04 ± 0.09a |
6.54 ± 0.06a |
8.20 ± 0.04a |
160.09 ± 0.24a |
70.13 ± 0.29a |
6.34 ± 0.08a |
18.72 ± 0.37a |
T1 (90 : 10) |
54.30 ± 0.04b |
16.82 ± 0.06a |
6.71 ± 0.05a |
8.08 ± 0.03a |
169.01 ± 0.18b |
68.03 ± 0.31b |
6.91 ± 0.07a |
19.66 ± 0.43b |
T2 (80 : 20) |
54.89 ± 0.05b |
17.33 ± 0.11b |
6.84 ± 0.08a |
8.02 ± 0.05a |
178.02 ± 0.26c |
64.20 ± 0.31c |
7.60 ± 0.23b |
20.59 ± 0.13c |
T3 (70 : 30) |
55.27 ± 0.03c |
18.74 ± 0.03c |
6.95 ± 0.07a |
7.94 ± 0.03a |
183.19 ± 0.36d |
62.33 ± 0.46d |
8.35 ± 0.18c |
21.83 ± 0.31d |
T4 (60 : 40) |
56.45 ± 0.02d |
20.37 ± 0.07d |
7.16 ± 0.06a |
7.87 ± 0.09a |
201.16 ± 0.24e |
60.05 ± 0.42e |
8.91 ± 0.14d |
22.99 ± 0.12e |
T5 (50 : 50) |
58.63 ± 0.07e |
21.84 ± 0.04d |
7.44 ± 0.06a |
7.87 ± 0.04a |
265.27 ± 0.61f |
58.41 ± 0.42f |
9.44 ± 0.19e |
24.04 ± 0.42f |
Weight.
The data pertaining to the weight of button mushroom and BF-based cookies in Table 2 show that the weight of the prepared cookies increased with the addition of MF and decrease in BF concentration. The minimum weight of 16.04 g was recorded in treatment Tc (control), while the maximum weight of 21.84 g was observed in treatment T5. Bello et al.27 also found an increase in the weight of the prepared cookies with the incorporation of mushroom powder concentration. The increase in the weight of the composite biscuit with increasing incorporation of mushroom can be due to the higher bulk density of mushroom powder than wheat flour.27 Treatment Tc (control) had the lowest weight, which can be attributed to the higher water holding capacity (WHC) compared to the treated samples.31 Different cookies possess different weights due to varying levels of water holding tendencies (WHC). Okpala et al.5 found an increasing pattern in biscuit weight prepared from plant material. However, an increase in weight with a decrease in soy bean and wheat flour concentration and vice versa was also reported by Ayo et al.32
Thickness.
The data in Table 2 show that the thickness of the prepared cookies increased with the incorporation of mushroom powder and decrease in BF concentration. The lowest thickness of 6.54 mm was found in treatment Tc (control), while the highest thickness of 7.44 mm was observed in treatment T5. Bello et al.27 also observed an increasing pattern in the thickness of the biscuits prepared from MF. Thongram et al.33 stated that the thickness of the biscuits can also be influenced by various baking attributes, such as higher or lower temperature, and high moisture absorption of dough (due to water binding compounds). The incorporation of MF affected the thickness of biscuits as the gluten network was weakened.34 Dhalagade et al.30 also found an increase in the thickness of cookies with the addition of the MF concentration. Chinma and Gernah35 prepared cookies from soyabean/cassava/mango composite flour and observed an increase in thickness, and this was due to the hydrophilic nature of the composite flour.
Spread ratio.
The spread ratio is an essential parameter responsible for the shape of cookies, and is indicative of their quality. The data (Table 2) showed that the spread ratio of the prepared cookies decreased with the incorporation of mushroom powder and decrease in BF concentration. A higher spread ratio of 8.20 was found in treatment Tc (control), while a lower spread ratio of 7.87 was found in treatment T5. Dhalagade et al.30 reported a decrease in the spread ratio in cookies prepared from MF. Similarly, McWatters36 found a decrease in the spread ratio with incorporation of non-wheat flours. Usually, protein and fibre can absorb and retain more water, thereby increasing viscosity and thus influencing the cookie spread ratio. Fuhr37 stated that flour or other ingredients, which absorb moisture during the dough preparation, reduces the spread ratio. Prodhan, et al.34 also reported that the variation in the diameter and thickness indicated the spread ratio, which consistently decreased with the incorporation of MF. Similarly, Giwa and Ibrahim38 showed that with the incorporation of the MF ratio, there is corresponding decrease in the spread factor. Ikuomola et al.39 also found a decrease in the spread ratio with decreasing BF concentration and vice versa. The decrease in the size of the barley-fortified cookies may also be attributed to the low spread factor due to the high protein content present in the dough.13 Prodhan et al.34 also found that the spread ratio of the biscuits was consistently reduced with the incorporation of mushroom levels. Chinma and Gernah35 found that the cookies prepared from soyabean/mango/cassava composite flour showed a similar trend. This might be attributed to the hydrophilic nature of the flour used in the preparation of the products, leading to the reduction in the spread ratio. Chen et al.7 also found that the spread ratio of cookies decreased with the addition of the MF concentration.
Breaking strength.
The breaking strength is defined as the force required to break cookies. The data in Table 2 show that the breaking of the prepared cookies increased with the incorporation of mushroom powder and decrease in BF concentration. The minimum breaking strength of 160.09 g was recorded in treatment Tc (control), while the maximum breaking strength of 265.27 g was observed in treatment T5. Ikuomola et al.39 reported that the breaking strength was significantly increased with the decrease in barley bran levels and vice versa. This can be due to the carbohydrate or starch concentration of barley bran. Adebowale et al.40 observed that the increase in rigidity is attributed to the rise in the carbohydrate or starch content, resulting in a gel/structure formation in baked products. Gupta et al.41 also observed the rise in breaking strength with the decrease in BF concentration and vice versa.
Color profile
Color is an essential attribute to determine consumer acceptability and desirability. Cauvain42 observed that the development of color of baked goods mostly occurs during the later stages of the baking process, and it can also be used to determine the baking process completion. The color values of button mushroom and BF-based cookies were depicted in terms of hunter L* (lightness), a* (redness) and b* (yellowness). Table 2 shows that the L* value of the cookies decreased, while the a* and b* values increased significantly with the incorporation of mushroom powder and decrease in BF. The higher L* value of 70.13 was recorded in treatment Tc, and the lower L* value of 58.41 was found in treatment T5. Meanwhile, the lowest a* and b* values of 6.34 and 18.72 were recorded in treatment Tc, respectively, and the highest a* and b* values of 9.44 and 24.04 were observed in treatment T5, respectively. A similar pattern was also obtained by Chen et al.,7 who found that the incorporation of MF into the cookies caused an increase in the a* and b* values and decrease in the L* values. The lightness of the biscuits was recorded between 41.39 to 48.86 L* values, while the control cookies had an L* value of 51.29 (lightness intensity). Meanwhile, the a* and b* values increased from 6.09 to 8.31 and 26.51 to 27.87, respectively. Irakiza et al.43 also found the same pattern of increase in the a* and b* values and decrease in L* in bread incorporated with MF. Sharma and Gujral44 also observed the decrease in L* values and increase in a* and b* values with a decrease in BF concentration and vice versa. Baking can result in a decrease in L* values and increase in a* and b* values, as compared to the corresponding dough. Caramelization of sugar and Maillard browning is responsible for the production of brown pigments during the baking process.45 Similarly, Bashir46 reported the increase in a* and b* values and decrease in L* values of meat analog nuggets incorporated with MF.
Proximate composition of prepared cookies
Moisture content.
Moisture content is an essential attribute to determine quality characteristics, acceptability, and shelf-stability of sugar and fat-based baked foods.47Table 3 shows that the moisture content of the prepared cookies increased with increasing mushroom powder and decreasing BF concentration. The minimum moisture content of 3.81% was observed in treatment Tc (control), followed by moisture contents of 3.99, 4.19, 4.74 and 4.96% in treatments T1, T2, T3 and T4, respectively. A higher moisture content of 5.63% was observed in treatment T5. Dhalagade et al.30 also observed an increase in the moisture content of cookies with the incorporation of mushroom powder concentration. The increase in moisture content with the increasing MF concentration could be related to the increase in protein and fibre content enhancing water absorption and high retention in cookies despite heat treatment. Mushrooms have the tendency to increase the water absorption and water holding capacity due to the presence of amino acids (polar), leading to the increase in moisture content.48 Ikuomola et al.39 also found that the moisture content of the cookies increased with decreasing concentration with malted barley bran flour and vice versa. Bello et al.27 and Prodhan et al.34 also reported on the rise in moisture content with increased mushroom powder concentration. Cauvain49 found that proteins can absorb their own weight in water, which is responsible for the increased moisture absorption by the high-protein flour compared to the low-protein flour. The moisture content of the cookies was low enough to inhibit the microbial growth, and consequently enhanced the storage stability.32 The variation in moisture content in the cookie samples may also be attributed to the variation in the water holding capacity of different ingredients.50
Table 3 Physico-chemical properties of button mushroom and BF-based cookiesa
Treatments (BF : BMP) |
Moisture content (%) |
Crude dat content (%) |
Crude protein content (%) |
Crude fibre content (%) |
Ash content (%) |
Carbohydrate content (%) |
Energy content (kcal/100 g) |
Water activity |
BF: barley flour, BMP: button mushroom powder. Different letters (a, b, c, d, e) that show significantly different means of observed data (p ≤ 0.05), according to post hoc Tukey's HSD test.
|
Tc (100 : 0) |
3.81 ± 0.03a |
33.06 ± 0.25a |
12.23 ± 0.30a |
2.76 ± 0.08a |
1.52 ± 0.09a |
49.37 ± 0.61a |
544.00 ± 1.05a |
0.402 ± 0.006a |
T1 (90 : 10) |
3.99 ± 0.04b |
30.42 ± 0.37b |
18.33 ± 0.31b |
2.57 ± 0.11b |
1.65 ± 0.12a |
45.50 ± 0.76b |
529.12 ± 1.53b |
0.421 ± 0.009b |
T2 (80 : 20) |
4.19 ± 0.15c |
29.37 ± 0.42c |
22.14 ± 0.22c |
2.35 ± 0.08c |
2.43 ± 0.11b |
41.94 ± 0.58c |
520.77 ± 2.26c |
0.463 ± 0.009c |
T3 (70 : 30) |
4.74 ± 0.07d |
26.20 ± 0.24d |
25.29 ± 0.40d |
2.15 ± 0.10d |
2.64 ± 0.21b |
41.12 ± 0.78c |
501.47 ± 0.64d |
0.497 ± 0.006d |
T4 (60 : 40) |
4.96 ± 0.07e |
25.46 ± 0.40e |
28.26 ± 0.34e |
1.93 ± 0.10e |
2.93 ± 0.12c |
38.38 ± 0.80d |
495.71 ± 1.81e |
0.526 ± 0.004e |
T5 (50 : 50) |
5.63 ± 0.22f |
24.24 ± 0.32f |
29.94 ± 0.55f |
1.63 ± 0.11f |
3.26 ± 0.22d |
36.92 ± 0.86e |
485.63 ± 1.61f |
0.543 ± 0.009f |
Crude fat.
Fat is an essential attribute. A higher fat content indicates a higher energy value, and can act as a lubricating agent that helps in enhancing the texture, rheology, and overall acceptability of the prepared product.51Table 3 shows that the highest crude fat content of 33.06% was observed in treatment Tc (control), while the lower crude fat content of 24.24% was observed in treatment T5. It was observed that the fat content of the developed cookies was reduced significantly with the addition of mushroom powder and decrease in BF concentration. Ojinnaka et al.52 reported a decrease in crude fat content in wheat–cocoyam flour blended cookies fortified with mushroom. Bello et al.27 also found a reduction in the fat content of biscuits with the addition of MF. A high fat concentration is undesirable in foods as it can cause rancidity, causing the formation of unpleasant or off odors.52 Irakiza et al.43 also found a reduction in fat content in bread incorporated with mushroom powder. Ikuomola et al.39 reported that the fat content of cookies decreased significantly with the incorporation of barley bran and vice versa. Similarly, Abdelazim et al.53 found a decrease in the fat content of biscuits with a decrease in BF and vice versa.
Crude protein.
Protein is an essential attribute responsible for proper body functioning, as it provides energy when digested and metabolized in the body. Table 3 illustrates that a lower crude protein content of 12.23% was observed in treatment Tc (control), while a higher crude protein content of 29.94% was observed in treatment T5. It was observed that the crude protein content of the developed cookies was increased significantly with the incorporation of mushroom powder and decrease in BF concentration. Prodhan et al.34 also found that the protein content increased with increasing concentration of MF to the wheat flour in the biscuit formulation. Similarly, Kumar and Barmanray25 found an increase in the crude protein content of mushroom-fortified biscuits. The rise in protein content was mostly due to the higher protein content of mushroom powder than BF. Ibrahium and Hegazy54 also found that the crude protein content of biscuits was gradually increased with increasing concentration of MF/sweet potato flour. Similarly, Bello et al.27 found that the crude protein content of biscuits increased with the incorporation of mushroom powder. Irakiza et al.43 also observed that the incorporation of MF positively influenced the crude protein content of breads. Gupta et al.41 found that the crude protein content of the cookies increased with decreasing substitution levels of barley and vice versa.
Crude fibre.
Table 3 shows that a higher crude fibre content of 2.76% was observed in treatment Tc (control), followed by crude fibre contents of 2.63, 2.57, 2.35 and 2.15% in treatment T5, T1, T2 and T3, respectively, while a lower crude fibre content of 1.93% was observed in treatment T4. It was found that the crude fibre content of the developed cookies decreased significantly with the increase in mushroom powder and decrease in BF concentration. Ikuomola et al.39 also found a decrease in the crude fibre content of cookies with a decrease in the BF concentration and vice versa. BF has a comparatively higher crude fibre content than mushroom powder, justifying the results obtained for the cookies. The decreasing pattern in the crude fibre content of cookies with decreasing concentration of barley can be a reflection of its composition.55 Similarly, Abdelazim et al.,53 Frost et al.13 and Alu'datt et al.56 observed the decrease in the crude fibre content with a decrease in the BF concentration and vice versa for biscuits, cookies and bread, respectively. The further increasing level of mushroom increased the crude fibre content of the cookie samples, as the MF used in the composite cookie preparation is also a rich source of dietary fibre. A similar increasing trend in the crude fibre content with increasing concentration of MF was found by Bello et al.,27 Dhalagade et al.30 and Kumar and Barmanray.25
Ash.
The ash content of any food product indicates the presence of minerals. It represents the inorganic matter present after removing water and organic material by heat treatment in the presence of an oxidizing agent.57 The ash content increases the metabolism of other constituents like protein, carbohydrate and fat.58 It was observed in Table 3 that a lower ash content of 1.52% was found in treatment Tc (control), while a higher ash content of 3.26% was found in treatment T5. It was observed that the ash content of the developed cookies was increased significantly with the incorporation of mushroom powder and decrease in BF concentration. Bello et al.27 found that the ash content of biscuits was increased with increasing mushroom powder concentration. The higher ash content of composite biscuits can be attributed to the fact that the mushrooms are a rich source of minerals. Prodhan et al.34 and Kumar and Barmanray25 also found an increase in the ash content of biscuits with increasing mushroom powder concentration. Similarly, Ibrahium and Hegazy54 found that the ash content of biscuits gradually increased with increasing level of sweet potato/MF mixture. Okafor et al.59 and Irakiza et al.43 also found an increase in the ash content with the incorporation of mushroom powder in fortified bread samples.
Carbohydrate.
Table 3 shows that a higher carbohydrate content of 49.37% was observed in treatment Tc, while a lower carbohydrate content of 36.92% was reported in treatment T5. It was found that the total carbohydrate content of the developed cookies was decreased with the incorporation of mushroom powder and decrease in BF concentration. Prodhan et al.34 found a reduction in the carbohydrate content in biscuits fortified with mushroom powder. Similarly, Kumar and Barmanray25 found a reduction in the carbohydrate content of biscuits with an increase in the mushroom powder levels, which can be attributed to the lower carbohydrate content of mushroom powder. The decrease in carbohydrate contents (70.45–23.71%) and (73.46–46.20%) of cookies prepared from whole wheat full-fat soya composite flour and wheat-brewers spent grain composite flour were also observed by Joel et al.60 and Gernah et al.,61 respectively. Adebayo-Oyetoro et al.47 and Ibrahium and Hegazy54 also observed a decrease in the total carbohydrate content in cookies and biscuits upon increasing the MF incorporation level. The low carbohydrate content of biscuits possesses numerous health-promoting effects, as it can improve the colon digestion and provide relief from constipation.62 Zienab et al.63 also found that the total carbohydrate content was decreased in cake blends prepared with the incorporation of BF, mushroom and turmeric powder. Ojinnaka et al.52 found that the carbohydrate content of the cookies was decreased with the incorporation of wheat–cocoyam–mushroom blends.
Total energy content.
Food energy represents the total caloric value available from food by oxidation. Table 3 shows that the highest energy content of 544.00 kcal/100 g was found in treatment Tc, while the lowest energy content of 485.63 kcal/100 g was reported in treatment T5. It was shown that the energy content of developed cookies was reduced with the incorporation of MF and decrease in BF concentration. Kumar and Barmanray25 observed the decrease in the total calorific value with increasing MF concentration. Gupta et al.41 also found a decrease in the total energy content in cookies with a decrease in BF concentration and vice versa. Similarly, Ojinnaka et al.52 observed a decrease in the total energy value in wheat–cocoyam cookies enriched with mushroom powder. Similarly Patyal64 reported a decreasing trend in energy value with the incorporation of MF. The decrease in energy value can be due to the reduced gluten in MF. A decrease in energy content (443.89–431.95 kcal/100 g) of cookies prepared from wheat and protein maize was also observed by Giwa and Ikujenlola.28 The protein, carbohydrate and fat content contributed to the total energy content of cookies. Cookies are energy-providing foods that are eaten mainly in-between meals by people.
Water activity.
Table 3 shows that the lower water activity of 0.402 was observed in treatment Tc (control), while the higher water activity of 0.543 was observed in treatment T5. It was found that the water activity of the developed cookies was increased significantly with an increase in the mushroom powder and decrease in BF concentration. Abdelazim et al.53 observed an increase in the water activity of biscuits with decreasing BF concentration and vice versa. The results were in agreement with those by Hassan et al.65 Frost et al.13 also observed that the water activity of cookies was increased with decreasing substitution levels of BF and vice versa.
Bioactive properties of developed cookies
Total phenolic content (TPC).
Table 4 shows that the lower TPC of 49.23 mg GAE g−1 was observed in treatment Tc, and the higher TPC of 71.12 GAE g−1 was found in treatment T5. It was found that TPC was increased with button mushroom. A similar trend of increase in the total phenolic content in biscuits with the incorporation of MF was reported by Tu et al.66 The increase in the total phenols of mushroom biscuits can be attributed to the mushroom dietary fibres preventing the release of bound phenolic components. The barley biscuit phenolic components may be attached to mushroom dietary fibres during the development of cookies/biscuits via non-covalent bonding. This binding can lead to an increase in the bound phenolic components in the cookies/biscuits.67 Similarly, Zienab et al.63 found an increase in the total phenols in cake blends incorporated with BF, turmeric and mushroom powder. Ojinnaka et al.52 also found an increase in the total phenol content in wheat–cocoyam cookies enriched with the incorporation of mushroom powder.
Table 4 Bioactive, sensory and microbial properties of button mushroom and BF-based cookiesa
Treatments (BF : BMP) |
Total phenol content (mg GAE g−1) |
DPPH scavenging activity (IC50; mg ml−1) |
Reducing power (EC50; mg ml−1) |
Overall acceptability |
Total plate count (× 103 cfu g−1) |
BF: barley flour, BMP: button mushroom powder. Different letters (a, b, c, d, e) show the significantly different means of observed data (p ≤ 0.05), according to the post hoc Tukey's HSD test.
|
Tc (100 : 0) |
49.23 ± 1.08a |
1.98 ± 0.09a |
4.44 ± 0.11a |
7.55 ± 0.53a |
3.15 ± 0.50a |
T1 (90 : 10) |
53.36 ± 1.06b |
1.88 ± 0.04a |
4.00 ± 0.07b |
8.06 ± 0.59a |
2.88 ± 0.60a |
T2 (80 : 20) |
56.20 ± 1.58c |
1.82 ± 0.02a |
3.93 ± 0.04b |
7.41 ± 0.60a |
2.84 ± 0.85a |
T3 (70 : 30) |
58.50 ± 1.37d |
1.78 ± 0.03a |
3.82 ± 0.06c |
7.15 ± 0.65a |
2.65 ± 0.71a |
T4 (60 : 40) |
65.08 ± 1.05e |
1.69 ± 0.03b |
2.59 ± 0.05d |
6.95 ± 0.66b |
2.54 ± 0.42a |
T5 (50 : 50) |
71.12 ± 1.03f |
1.57 ± 0.04c |
2.45 ± 0.05d |
6.46 ± 0.61b |
2.47 ± 0.81a |
Antioxidant activity.
Antioxidants are vital components possessing the ability to scavenge free radicals, which can cause several degenerative diseases. The antioxidant activity of button mushroom and BF-based cookies was measured in terms of DPPH scavenging activity and reducing power, and was expressed in terms of IC50 (mg ml−1) and EC50 (mg ml−1), respectively.14 Low IC50 and EC50 values correspond to highest DPPH scavenging activity and reducing power, respectively. The data pertaining to the DPPH scavenging activity of cookies in Table 4 revealed that the cookies prepared with a higher concentration of mushroom exhibited the lowest IC50 value for DPPH scavenging activity, and consequently higher antioxidant activity in comparison to the cookies prepared with BF. The lowest IC50 value of 1.57 mg ml−1 was recorded in treatment T5, while the highest IC50 value of 1.98 mg ml−1 was observed in treatment Tc. The perusal data in Table 4 showed that the cookies prepared with a higher concentration of mushroom exhibited the lowest EC50 value for DPPH scavenging activity, and consequently higher antioxidant activity as compared to the cookies prepared with BF. As depicted in Table 4, the lowest EC50 value of 2.45 mg ml−1 was recorded in treatment T5, while as the highest EC50 value of 4.44 mg ml−1 was observed in treatment Tc. This might be due to the higher amounts of antioxidants, such as total phenols, total flavonoids and total ascorbic acid, present in mushroom relative to BF. The rise in antioxidant activity can also be due to the Mallaird reaction, leading to the brown pigment formation during the baking process. These pigments are considered to have antioxidant potential.68 Zienab et al.63 also found that the antioxidant activity of cake blends increased steadily as the concentration of BF, mushroom and turmeric powders increased. Bashir46 found an increase in the antioxidant activity of meat analog nugget with an increase in mushroom powder concentration.
Sensory evaluation of developed cookies.
On sensory evaluation basis, an overall acceptability (color, texture, taste) score of button mushroom and BF-based cookies of 8.06 was found in the T1 treatment, while the lowest overall acceptability score of 6.46 was found in the T5 treatment. The variation of the overall acceptability score of prepared cookies might be due to the color, texture and taste associated with the BF and MF. The sensory score indicated that there was no significant difference in the surface color and appearance of the control samples and the biscuits developed from 10% of MF (T1). It was also observed that in treatments T2, T3, T4 and T5, the incorporation of MF inclusion led to the relatively darker color. This might be attributed to enzymatic browning, which may result in products being considered overbaked and dissatisfactory to some panelists. Biscuits were found to be harder with increasing concentration of MF. This can be attributed to the high water absorption capacity.25 However, with increasing concentration of mushroom powder, the cookies had a slightly bitter taste, which can be due to the high polyphenols content.69 Irakiza et al.43 observed a decrease in the sensory score in breads as the mushroom powder concentration increased. Majeed et al.70 also found a highly significant difference in the sensory score of breads prepared with MF. Abdelazim et al.53 reported that the overall acceptability (taste and texture) of biscuits was decreased with a decrease in BF and vice versa. Kulkarni et al.71 also observed an increase in the overall acceptability of mushroom-fortified cookies up to 10% level of substitution. However, further addition adversely influenced the overall acceptability of cookies. Thus, it was found that the substitution of 10% mushroom powder can produce better cookies that are highly acceptable compared to the reference sample with improved attributes.
Microbial analysis.
The microorganisms present in developed cookies were indicators of the sanitary quality of the raw materials, processing and storage conditions.46 The results in Table 4 revealed that a higher total plate count of 3.15 × 103 cfu g−1 was recorded in treatment Tc, and a lower total plate count of 2.47 × 103 cfu g−1 was observed in treatment T5. The total plate count decreased significantly with increasing concentration of MF and BF. This decrease might be due to lower moisture content, water activity, and secondary metabolites associated with MF. Similarly, Bashir46 also observed a decrease in the total plate count of meat analog nuggets fortified with mushroom, flaxseed and amaranth flour. Desayi et al.1 also found a reduction in the total plate count of biscuits fortified with mushroom powder.
Textural properties of cookies.
The textural characteristics of the cookies are important for quality evaluation. One of the primary characteristics that determines the textural characteristics of cookies is hardness, which is assessed as the peak force required to puncture the cookies. The control sample exhibited the maximum peak force (24.749 ± 0.539 N), whereas the 10% mushroom powder-augmented cookies had a hardness value of 21.353 ± 0.891 N (Fig. 2(a)). The 50% mushroom powder-augmented cookies yielded the lowest peak force (14.312 ± 0.503 N). The results showed that with increasing levels (10, 20, 30, 40, 50%) of MF incorporated into the cookie mix, the mean peak force value decreased significantly (p < 0.05). MF cookies required a substantially lower force to break than control cookies. Because of the degradation of macromolecules and lower bulk density of the flour blends, the hardness of wheat flour cookies is higher than the hardness of blended cookies. Furthermore, the high dietary fiber content of the MF-blended flour boosts the viscosity of cookies, while delaying the starch granule accessibility to digesting enzymes in the human digestive system. The same pattern was also reported by Salehi et al.,72 who observed that the hardness of cakes was decreased with the incorporation of increasing levels of MF. This was mainly related to the volume/density of the material. However, Brennan et al.73 also found a decrease in the hardness with the incorporation of mushroom powder concentration.
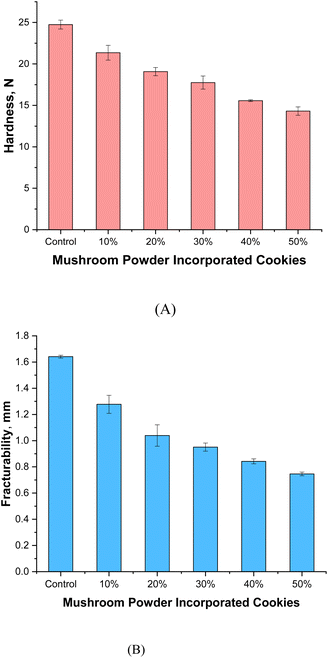 |
| Fig. 2 Textural properties of mushroom powder-incorporated cookies: (A) hardness (N), and (B) fracturability (mm). | |
The ease with which the cookies break is referred to as its fracturability. The fracturability of the mushroom powder-incorporated cookies varied between 0.341 and 0.644 mm (Fig. 2(b)). The lowest fracturability was found for cookies containing 50% mushroom powder, whereas the greatest fracturability was obtained for the control sample. The fracturability was shown to diminish with the incorporation of MF. As a result, the level of mushroom powder had a detrimental impact on the fracturability of cookies.
Effect on the thermal properties of mushroom powder-incorporated cookies
Differential scanning calorimetry was used to analyze the thermal characteristics of the cookies, and the findings are summarized in Table 5. The onset temperature (To) significantly decreased (p < 0.05) from 54.86 to 46.82 from the control sample to the 50% mushroom powder incorporated sample. In the current analysis, the endothermic peak of the control sample was measured at 61.87 °C, whereas the endothermic peak of the samples containing 10%, 20%, 30%, 40%, and 50% mushroom powder was detected at 59.51, 58.27, 57.69, 56.81, and 55.64 °C, respectively. The gelatinisation temperature (Tpeak or Tg) is a thermostability indicator; the greater the Tg, the more thermodynamically stable the product. This change in the glass transition temperature might be related to the decreased thermal stability of mushroom powder. As the percentage of MF in the cookies increased, the Tg value moved to a lower temperature due to its decreased thermostability. Integrating the region below the endothermic peak (the integral is determined by integrating the area under the peak) yields the enthalpy of the transition (ΔH). The data revealed only one endotherm, which corresponded to fat melting (Table 5). For 10% to 50% inclusion of mushroom powder in the cookies, the enthalpy value increased considerably (p < 0.05) from 3.711 to 6.654 J g−1. Because of the inclusion of mushroom powder in the cookies, the enthalpy of the transition ΔH was greater than that of the control sample. As a result, there was a positive association between the ΔH of the control cookies and the total mushroom powder concentration in the cookies. Similar results were observed by Lu et al.,74 who reported that with the increase in mushroom supplementation, the degree of gelatinization was also decreased, as some large starch granules were found to move towards the exterior surface of bread. However, the onset temperature (To) and enthalpy of the transition ΔH was observed to increase with the incorporation of MF concentration.74
Table 5 Thermal characteristics of the mushroom powder-incorporated cookiesa
Treatments (BF : BMP) |
Treatments |
Temperatures of onset (To) |
Gelatinisation temperature (Tpeak or Tg) |
Temperatures of endset (Te) |
Enthalpy of the transition (ΔH) J g−1 |
BF: barley flour, BMP: button mushroom powder.
|
Tc (100 : 0) |
1 |
54.86 |
61.87 |
68.45 |
3.711 |
T1 (90 : 10) |
2 |
52.71 |
59.51 |
67.82 |
4.161 |
T2 (80 : 20) |
3 |
51.18 |
58.27 |
66.03 |
4.081 |
T3 (70 : 30) |
4 |
49.39 |
57.69 |
68.53 |
5.404 |
T4 (60 : 40) |
5 |
48.26 |
56.81 |
69.62 |
6.119 |
T5 (50 : 50) |
6 |
46.82 |
55.64 |
69.81 |
6.654 |
Scanning electron microscopy (SEM)
Scanning electron microscopy of four types of cookies (with BF and mushroom powder ratios of 100
:
0, 90
:
10, 70
:
30, and 50
:
50) at a magnification of 2000× was used to demonstrate the effect of various components on the sample's structure. A continuous sheet of gelatinized starch was produced as a consequence of the starch granules becoming distorted and losing their identity (Fig. 3A–D). The gelatinized starch matrix may have formed as a result of steam produced during baking. The same pattern was also found by Ng et al.,75 where the starch granules were found to retain their granular identity. However, the starch granules were elongated and seemed to be fused with neighboring starch granules to constitute a continuous network. The microstructure was presented by an open structure with a larger starch-granule diameter, corresponding to the entrapment of gas bubbles that expanded during the baking process. This can increase the surface area exposed to enzymatic activity. The same pattern was also observed by Brennan and Tudorica76 while studying the effect of dietary fibre on the internal structures of pasta. Some of the starch granules retained the effects of heat treatment since there was not enough moisture in all of the cookies for gelatinization. In Fig. 3(B–D), the structure was compressed in contrast to the control cookie sample due to the higher fibre and protein content, resulting in a denser matrix. Fiber particles were shown adhering to starch granules in the photomicrographs. A network and irregular air gaps were seen in the control sample with no specific granule formation. Wu et al.77 also observed that the dietary fibres decreased the retention of integrity of the starch granules by decreasing the diameter of starch granules and interrupting the starch granule structure. These irregular air spaces grow narrower as the level of MF rises. It was shown in Fig. 3(A–D) that an increase in the percentage of MF reduces the amount of air bubbles in the final product.
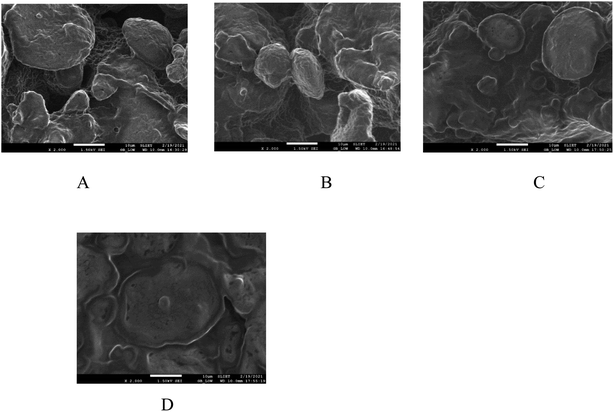 |
| Fig. 3 Scanning electron microscopy of four types of cookies formulated with BF and mushroom powder in the ratios of 100 : 0, 90 : 10, 70 : 30, and 50 : 50. A – (control: 100% BF); B – (90 BF and 10% mushroom powder-based cookies); C – (70% BF and 30% mushroom powder-based cookies); D – (50% BF and 50% mushroom powder-based cookies). | |
Structural characterization by X-ray diffraction (XRD)
By using XRD analysis, the crystalline characteristics of cookies made with BF and mushroom powder in the ratios of 100
:
0 and 50
:
50 were examined. The XRD pattern showed that the mushroom powder-incorporated sample had a peak intensity that was greater than the control sample. More crystalline formation was caused by the incorporation of mushroom powder, which increased the intensity of the long spacing peaks. Similar results were also obtained by Yadav and Mishra78 in mushroom-fortified cheese, and they reported that the material had a very broad humped peak. After baking, a V-type crystal structure was indicated by the peak that was visible between 20.366 and 20.552° (Fig. 4 and Table 6). The presence of an amylose–lipid complex is indicated by the “V” type starch peaks at 20.366° and 20.552°. Incorporated cookies with mushroom powder showed an amylose–lipid complex peak that was both deeper and sharper than control cookies. The peaks of cookies made with BF and mushroom powder in the proportions of 100
:
0 and 50
:
50 had full widths at half maximums (FWHM) of 13.557 and 14.353 rad, respectively.
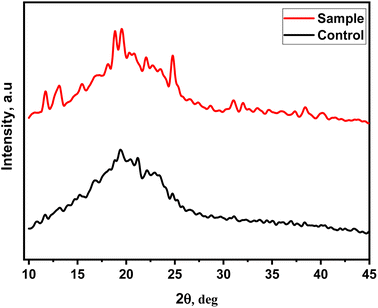 |
| Fig. 4 X-ray diffraction profile of cookies made with BF and mushroom powder in the ratios of 100 : 0 and 50 : 50. | |
Table 6 XRD analysis of cookies produced using BF and mushroom powder in the ratios of 100
:
0 and 50
:
50
Sample |
2θ, deg |
θ, deg |
θ, rad |
cos (θ), rad |
FWHM, rad |
Beta, rad |
D, rad |
Control (BF: mushroom powder ratio = 100 : 0) |
20.366 |
10.183 |
0.178 |
0.984 |
13.557 |
0.237 |
0.595 |
Sample (BF: mushroom powder ratio = 50 : 50) |
20.552 |
10.276 |
0.179 |
0.984 |
14.353 |
0.251 |
0.562 |
Conclusion
The cookies prepared by barley and MF were more rich in nutrients than cookies prepared with BF as control. The results indicated that it is possible and desirable to partially substitute BF with mushroom powder for cookie production. BF substitution of up to 50% MF resulted in improving the physicochemical, nutritional, bioactive and textural attributes. The addition of MF increased the total phenolic content and antioxidant activities of the cookies. Cookies developed using a 50
:
50 ratio of BF and mushroom powder were found to be better, having the highest crude protein content (29.94 percent), ash content (3.26 percent), phenolic content (71.12 mg GAE g−1), antioxidant activity (1.57 mg ml−1). The sensory evaluation revealed that the higher overall acceptability score of 8.06 was found in T1 (90
:
10 ratio of barley and MF). The supplementation of BF with MF affected the thermal, textural and structural properties of the prepared cookies. Utilization of mushroom powder for the formulation of cookies can be executed at the industrial scale in order to develop a value-added protein and fiber-rich product with potential bioactive properties. The incorporation of cookies with MF is thus an efficient tool to provide a nutritious and healthy alternative to people.
Abbreviations
AACC | American association of cereal chemists |
AOAC | Association of official analytical chemists |
BMP | Button mushroom powder |
D
| Diameter |
DPPH | 2,2-Diphenyl-1-picrylhydrazyl |
LDPE | Low density polyethylene |
T
| Thickness |
TPC | Total phenolic content |
WHC | Water holding capacity |
Author contributions
Conceptualization, R. S.; investigation, methodology, J. S., K. K. D.; and A. H. D.; writing original draft, R. S.; review and editing – R. P.
Conflicts of interest
There are no conflicts to declare.
Acknowledgements
The first author is thankful to the Indian Council of Medical Research, for the award of the ICMR-Fellowship 2019 in favour of Ms. Rafeeya Shams (vide grant no. ICMR/SRF-3/1/2/131/2019-(Nut)).
References
- D. B. Desayi, L. H. Kukanoor, C. P. Patil, M. Shirur and B. B. Shivakumar, Mushroom Res., 2012, 21(1), 67–71 Search PubMed.
- A. Gaurh, A. Kothakota, R. Pandiselvam, J. P. Pandey and N. C. Shahi, Agric. Eng., 2017, 41(2), 48–54 Search PubMed.
- M. Irakli, A. Mygdalia, P. Chatzopoulou and D. Katsantonis, Food Chem., 2019, 285, 231–239 CrossRef CAS PubMed.
- N. U. Sruthi, K. Josna, R. Pandiselvam, A. Kothakota, M. Gavahian and A. M. Khaneghah, Food Chem., 2022, 368, 130809 CrossRef CAS PubMed.
- L. Okpala, E. Okoli and E. Udensi, Food Sci. Nutr., 2013, 1(1), 8–14 CrossRef CAS PubMed.
- N. A. Bhat, I. A. Wani and A. M. Hamdani, Heliyon, 2020, 6(1), e03042 CrossRef PubMed.
- C. Chen, Y. Han, S. Li, R. Wang and C. Tao, CyTA–J. Food, 2021, 19(1), 137–145 CrossRef CAS.
- P. Sharma and H. S. Gujral, Food Chem., 2010, 120, 673–678 CrossRef CAS.
- P. Sharma and H. S. Gujral, Food Bioprocess Technol., 2013, 6, 1374–1389 CrossRef CAS.
- P. Sharma, H. S. Gujral and B. Singh, Food Chem., 2012, 131, 1406–1413 CrossRef CAS.
- H. S. Gujral, P. Sharma, R. Bajaj and V. Solah, Food Sci. Technol. Int., 2012, 18, 47–54 CrossRef CAS PubMed.
- R. Randhir, Y. I. Kwon and K. Shetty, Innovative Food Sci. Emerging Technol., 2008, 9, 355–364 CrossRef CAS.
- D. J. Frost, K. Adhikari and D. S. Lewis, J. Food Sci. Technol., 2011, 48(5), 569–576 CrossRef CAS PubMed.
- R. Shams, J. Singh, K. K. Dash and A. H. Dar, Appl. Food Res., 2022, 2(1), 100084 CrossRef CAS.
-
AACC. American Association of Cereal Chemists Approved Methods of American Association of Cereal Chemists, 10th edn, St Paul, MI, 2000, pp. 10–50 Search PubMed.
- J. C. Okaka and M. I. Isieh, Niger. Food J., 1997, 8(2), 56–62 Search PubMed.
-
AOAC. Official Methods of Analysis, 18th edn, Association of Official Analytical Chemists, 2006 Search PubMed.
- Y. Xiao, G. Xing, X. Rui, W. Li, X. Chen, M. Jiang and M. Dong, J. Funct. Foods, 2014, 10, 210–222 CrossRef CAS.
- J. Liu, J. Luo, H. Ye, Y. Sun, Z. Lu and X. Zeng, Carbohydr. Polym., 2009, 78(2), 275–281 CrossRef CAS.
-
M. J. Pelczar and E. C. S. Chan, in Black Dot, New York. 1991 Search PubMed.
- Y. Biao, X. Chen, S. Wang, G. Chen, D. J. Mcclements and L. Zhao, Food Sci. Nutr., 2020, 8(1), 361–370 CrossRef PubMed.
- R. Gonzalez, C. Carrara, E. Tosi, M. C. Anon and A. Pilosof, LWT–Food Sci. Technol., 2007, 40(1), 136–143 CrossRef CAS.
- H. Yamamoto, S. T. Worthington, G. Hou and P. Ng, Cereal Chem., 1996, 73, 215–221 CAS.
- A. Chauhan, D. C. Saxena and S. Singh, Cogent Food Agric., 2016, 2, 1125773 Search PubMed.
- K. Kumar and A. Barmanray, Proteins, 2007, 96, 325 Search PubMed.
- W. K. Abdul, A. Javid, M. Tariq, A. Muhammad, P. Mohammad and H. Said, World J. Dairy Food Sci., 2015, 10, 68–73 Search PubMed.
- M. Bello, M. O. Oluwamukomi and V. N. Enujiugha, Arch. Curr. Res., 2017, 9(3), 1–11 CrossRef.
- E. O. Giwa and A. V. Ikujenlola, Afr. J. Food Sci. Agric., 2010, 1(5), 116–119 Search PubMed.
- A. A. Abou-Zaid, A. Abd El-Hafez and H. Elsokary, Powder Blends, 2016, 1–10 Search PubMed.
- J. R. Dhalagade, D. N. Parab, R. C. Ranveer, N. B. Rathod and A. K. Sahoo, Am. J. Food Technol., 2020, 15, 28–34 CrossRef CAS.
- F. L. Kolawole, B. A. Akinwande and B. I. O. Ade-Omowaye, J. Saudi Soc. Agric. Sci., 2018, 19(2), 174–178 Search PubMed.
- J. A. Ayo, V. A. Ayo, I. Nkama and R. Adeworie, Niger. Food J., 2007, 25, 15–17 Search PubMed.
- S. Thongram, B. Tanwar, A. Chauhan and V. Kumar, Cogent Food Agric., 2016, 2, 1172389 Search PubMed.
- U. K. Prodhan, K. M. M. R. Linkon, M. F. Al-Amin and M. J. Alam, Emir. J. Food Agric., 2015, 542–547 CrossRef.
- C. E. Chinma and D. I. Gernah, J. Mater. Res., 2007, 4, 32–43 Search PubMed.
- K. H. McWatters, Cereal Chem., 1978, 55, 853–863 Search PubMed.
- F. R. Fuhr, Baker's digest, 1962, 36, 56–60 Search PubMed.
- O. E. Giwa and T. A. Ibrahim, J. Pharm. Biomed. Sci., 2012, 18, 1–15 Search PubMed.
- D. S. Ikuomola, O. L. Otutu and D. D. Oluniran, Cogent Food Agric., 2017, 3(1), 1293471 CrossRef.
- A. A. Adebowale, M. T. Adegoke, S. A. Sanni, M. O. Adegunwa and G. O. Fetuga, Am. J. Food Technol., 2012, 7, 372–379 CrossRef.
- M. Gupta, A. S. Bawa and N. Abu-Ghannam, Food Bioprod. Process., 2011, 89(4), 520–527 CrossRef CAS.
-
S. Cauvain, Biscuits, Cookies, Crackers and Wafers, Baking Problems Solved, 2nd edn, 2017, pp. 299–329 Search PubMed.
- P. N. Irakiza, G. B. Chuma, T. Z. Lyoba, M. A. Mweze, J. M. Mondo, P. K. Zihalirwa and G. N. Mushagalusa, Agric. Food Secur., 2021, 10(1), 1–11 CrossRef.
- P. Sharma and H. S. Gujral, Food Res. Int., 2011, 44, 235–240 CrossRef CAS.
- L. Laguna, A. Salvador, T. Sanz and S. M. Fiszman, LWT–Food Sci. Technol., 2011, 44, 737–746 CrossRef CAS.
-
N. Bashir, PhD. thesis, Sher-e Kashmir university of agricultural sciences and technology, Jammu, 2019.
- A. O. Adebayo-Oyetoro, O. O. Ogundipe and K. N. Adeeko, Food Sci. Nutr., 2015, 4(3), 364–369 CrossRef PubMed.
- A. B. Nordiana, W. W. Rosli and W. W. A. Nizam, Int. Food Res. J., 2019, 26(4), 1249–1257 CAS.
-
C. Cauvain, Technology of Bread Making, Thomson Publishing, Britain, 1998, DOI:10.1007/978-1-4615-2199-0.
- H. Rathnayake and S. Navaratne, Int. J. Sci. Res., 2017, 6(2), 339–344 Search PubMed.
-
P. K. Grewal, M. Res. thesis, Western Sydney University, 2018.
- M. C. Ojinnaka, N. E. Obasi and J. K. Owuala, Niger. Agric. J., 2018, 49(2), 46–54 Search PubMed.
- S. A. Abdelazim, T. E. Sohair and M. A. Kamel, Act. Sci. Nutr., 2019, 3(11), 114–123 CrossRef.
- M. Ibrahium and A. Hegazy, Curr. Sci. Int., 2014, 3(1), 26–33 Search PubMed.
- K. Satinder, S. Sativa and H. P. S. Nagi, Asian J. Food Agro-Ind., 2011, 4, 122–131 Search PubMed.
- M. H. Alu'datt, T. Rababah, K. Ereifej, I. Alli, M. A. Alrababah, A. Almajwal and M. N. Alhamad, Food Hydrocolloids, 2012, 26(1), 135–143 CrossRef.
- S. A. Sanni, A. A. Adebowale, I. O. Olayiwola and B. Maziya-Dixon, J. Food Agric. Environ., 2008, 6, 172–175 CAS.
-
J. C. Okaka and G. L. Ene, Food microbiology: method in food safety control, OCJANCO Academic, Enugu, 2005, p. 262 Search PubMed.
- J. N. C. Okafor, G. I. Okafor, A. U. Ozumba and G. N. Elemo, Pak. J. Nutr., 2012, 11(1), 5–10 CrossRef CAS.
- N. Joel, K. Fatima and F. Stephen, Eur. Food Res. Technol., 2014, 2, 19–28 Search PubMed.
- D. I. Gernah, I. A. Senger and J. O. Senger, Niger. Food J., 2010, 28(2), 440–447 Search PubMed.
- M. Elleuch, D. Bedigian, O. Roiseux, S. Besbes, C. Blecker and H. Attia, Food Chem., 2011, 124(2), 411–421 CrossRef CAS.
- A. A. Zienab, I. A. Hassan and E. R. Ibrahim, Middle East J. Appl. Sci., 2015, 5(4), 1257–1266 Search PubMed.
-
S. Patyal, Doctoral dissertation, UHF, NAUNI, 2014.
- A. A. Hassan, N. M. Rasmy, M. I. Foda and W. K. Bahgaat, World J. Dairy Food Sci., 2012, 7(1), 01–20 CAS.
- J. Tu, M. A. Brennan, G. Wu, W. Bai, P. Cheng, B. Tian and C. S Brennan, Foods, 2021, 10(8), 1812 CrossRef CAS PubMed.
- J. R. Taylor, P. S. Belton, T. Beta and K. G. Duodu, J. Cereal Sci., 2014, 59(3), 257–275 CrossRef CAS.
- L. S. Manzocco, S. Calligaris, D. Mastrocola, M. C. Nicoli and C. R. Lerici, Trends Food Sci. Technol., 2000, 11, 340–346 CrossRef CAS.
- C. M. Ajila, K. U. J. S. Leelavathi and U. P. Rao, J. Cereal Sci., 2008, 48(2), 319–326 CrossRef CAS.
- M. Majeed, M. U. Khan, M. N. Owaid, M. R. Khan, M. A. Shariati, P. Igor and G. N. Ntsefong, J. Microbiol., Biotechnol. Food Sci., 2021, 1221–1227 Search PubMed.
- S. K. Kulkarni, B. K. Sakhale, V. D. Pawar, U. G. Miniyar and B. M. Patil, Food Sci. Res. J., 2010, 1(2), 90–93 Search PubMed.
- F. Salehi, M. Kashaninejad, F. Asadi and A. Najafi, J. Food Sci. Technol., 2016, 53(3), 1418–1423 CrossRef CAS PubMed.
- M. A. Brennan, E. Derbyshire, B. K. Tiwari and C. S. Brennan, J. Agric. Food Chem., 2012, 60, 4396–4401 CrossRef CAS PubMed.
- X. Lu, M. A. Brennan, W. Guan, J. Zhang, L. Yuan and C. S. Brennan, Foods, 2021, 10(4), 731 CrossRef CAS PubMed.
- S. H. Ng, S. D. Robert, W. A. N. W. Ahmad and W. R. W. Ishak, Food Chem., 2017, 227, 358–368 CrossRef CAS PubMed.
- C. S. Brennan and C. M. Tudorica, Int. J. Food Sci. Technol., 2008, 43(12), 2151–2162 CrossRef CAS.
- N. J. Wu, F. J. Chiou, Y. M. Weng, Z. R. Yu and B. J. Wang, Int. J. Food Sci. Nutr., 2014, 65(4), 502–506 CrossRef PubMed.
- S. Yadav and S. Mishra, Act. Sci. Nutr., 2022, 6(7), 67–72 CrossRef.
|
This journal is © The Royal Society of Chemistry 2023 |