Widespread presence of chlorinated paraffins in consumer products†
Received
28th November 2022
, Accepted 31st January 2023
First published on 11th April 2023
Abstract
Short-chain chlorinated paraffins (SCCPs) were listed for elimination under the Stockholm Convention in 2017 due to their persistence and toxicity. Although Canada and other Stockholm signatories have prohibited the manufacture, usage and import of SCCPs since 2013, they can still be detected at high concentrations in indoor dust. To identify the sources of the SCCPs in the Canadian indoor environment, short-, medium- and long-chain chlorinated paraffins (SCCPs, MCCPs, LCCPs, respectively) were measured using a sensitive LC-ESI-orbitrap method. SCCPs were detected in 84 of the 96 products purchased in Canada after 2013 (87.5%) including electronic devices, clothing, plastics (toys), and paintings. Concentrations of SCCPs were up to 0.93% (9.34 mg g−1). SCCPs were also detected in newly purchased toys at 0.005–2.02 mg g−1, indicating the potential for children's exposure. Profiles of chlorinated paraffins differed among categories of products. For example, C13-SCCPs were most common in toys, while electronic devices like headphones showed comparable concentrations of SCCPs and MCCPs. Additionally, four new carboxylate derivatives of CPs were detected in an electronic device sample. These are the first data to show the ubiquitous occurrences of SCCPs in a wide range of products currently marketed in Canada, suggesting continuing indoor exposure to SCCPs despite their prohibition.
Environmental significance
Prohibited short-chain chlorinated paraffins were widely detected in indoor consumer products. Short-chain chlorinated paraffins (SCCPs) were listed for elimination under the Stockholm Convention in 2017 due to their persistence and toxicity. Although Canada has prohibited the manufacture, usage and import of SCCPs since 2013, we herein reported the widespread detection of SCCPs in Canadian consumer products. SCCPs were detected in 84 of the 96 products purchased in Canada after 2013 including electronic devices, clothing, plastics (toys), and paintings. SCCPs were also detected in newly purchased toys, indicating the potential for children's exposure. These are the first data to show the ubiquitous occurrences of SCCPs in a wide range of currently marketed products, suggesting continuing indoor exposure to SCCPs despite their prohibition.
|
Introduction
Chlorinated paraffins (CPs) are a class of chlorinated n-alkanes widely used in many products and applications.1 CPs are categorized into three classes according to their carbon chain length, as short-chain (C10–C13, SCCPs), medium-chain (C14–C17, MCCPs), and long-chain (C>17, LCCPs) CPs. CPs have been widely detected in environmental matrices,2–5 wildlife,4,6,7 and human milk and blood.8 Due to the concerns regarding their persistence, bioaccumulation potential and toxicity, SCCPs were listed in 2017 as persistent organic pollutants (POPs) under the Stockholm Convention in Annex A (elimination).9 It is important to note that SCCPs are allowed for use at up to 1% by weight in other CP mixtures and that the listing includes numerous exemptions such as their use in the leather industry, adhesives and as secondary plasticizers in flexible polyvinyl chloride, but exemptions do not include toys and children's products.10 Canada prohibited the manufacture, sale, use, and import of CPs in Canada under the Canadian Environmental Protection Act in 2012. This regulation, which came into force in March 2013,11 pertains to CPs as a feedstock and in finished imported goods. They do not specify a “de minimus” or threshold value above which the prohibition pertains, although the regulation acknowledges that CPs could be present due to incidental presence.
SCCPs have been widely detected in recently collected Canadian indoor dust,12 a common human exposure route for indoor contaminants. This suggests continuing emissions of SCCPs indoors but the sources remain unknown. SCCPs have been used in plastics, rubber and textiles as flame retardants, in polyvinyl chloride (PVC), sealants, adhesives, paints, and varnishes as plasticizers and flame retardants, and in leather as a liquoring agent.13 Chen et al. estimated that the greatest use of SCCPs in China was in PVC-based products such as the sheathing of electrical cables, piping used in plumbing and in vinyl flooring.14 Two previous studies from Europe that focused on indoor products reported high concentrations of SCCPs in hand blenders and baking oven doors.15,16 Recently, relatively high concentrations of SCCPs were reported in hand wipes in a Norwegian cohort.17 While these studies highlight human exposure to SCCPs via indoor products, a systematic investigation of the occurrence of SCCPs in indoor products has not been conducted to the best of our knowledge. More importantly, it is critical to determine if SCCPs are continuously released from products purchased in Canada since its prohibition in 2013.
The goal of this study was to identify typical consumer products likely to contribute to indoor levels of SCCPs. A sensitive chloride-enhanced method with liquid chromatography electrospray ionization coupled to Orbitrap mass spectrometry (LC-ESI-Orbitrap) was used to investigate the occurrences of SCCPs, MCCPs, and LCCPs in 96 diverse indoor consumer products, purchased in Canada after 2013. Unexpectedly, SCCPs were widely detected in various products despite their prohibition in Canada since 2013. Since these products were largely manufactured overseas for an international market, they are likely to be an indoor source of SCCP exposure in other counties or regions (e.g., US and European) as well.
Experimental section
Chemicals and reagents
Six standard technical mixtures of CPs (C10–C13, 51.5% Cl; C10–C13, 63% Cl; C14–C17, 42% Cl; C14–C17, 57% Cl; C18–C20, 36% Cl; C18–C20, 49% Cl) were purchased from LGC Standards (Manchester, NH, USA). Mass labeled 13C10-antidechlorane plus (13C10-anti-DP) was purchased from Wellington Laboratories (Guelph, Ontario, Canada). Ultrapure water, methanol, dichloromethane and acetonitrile (HPLC grade) were purchased from Fisher Scientific (Ottawa, ON, CA). Formic acid (mass spectrometry grade) was purchased from EMO Millipore (St. Louis, MO, USA). Ammonium chloride (ACS grade) was purchased from Millipore Sigma (Oakville, ON, CA).
Sample collection and extraction
96 Indoor products including electric devices, plastic products, toys, personal care products, and indoor furniture were purchased in Toronto, Canada, or collected from Toronto homes. All products were newly purchased in 2019 or purchased at least one year after the prohibition of SCCPs in Canada in 2013 (see Table S1† for more information). We were not able to source most products with regard to whether they were domestically manufactured or imported. Only the newly purchased children's toys were confirmed to be imported. Solid products were cut into 1–2 mm slices with scissors or scalpel on a pre-cleaned metal surface in the lab. To avoid interference from potential surface contamination, samples were collected from at least 5 mm below the surface of each product, when possible. However, contamination might have occurred for fabrics and upholstery for which only surface samples could be collected.
A simple sample extraction method was used for the analysis of CPs due to the high sensitivity of the instrumental method. Approximately 0.05–0.1 g of product samples was loaded into 1.5 mL Eppendorf tubes and spiked with 10 μL of 13C10-anti-DP (1 mg L−1) as an internal standard. The samples were extracted by shaking with 1 mL of dichloromethane for 30 minutes.18 200 μL aliquots of extracts were then blown to dryness under a gentle stream of N2 and reconstituted in 1 mL of methanol for instrumental analysis.
Chloride-enhanced LC-ESI-orbitrap analysis
Analysis of CPs was conducted with using a Q Exactive mass spectrometry equipped with a Vanquish UHPLC system (Thermo Fisher Scientific, USA). Separation of CPs was achieved with Accucore Vanquish C18 column (50 × 2.1 mm, 1.5 μm, Thermo Scientific). The Injection volume was 2 μL with 0.1 mM ammonium chloride in ultrapure water (A) and 0.1 mM ammonium chloride in methanol (B) as mobile phases. Initially 10% B was increased to 50% in 2 min, then increased to 100% at 5 min and held static for 3 min, followed by a decrease to initial conditions of 10% B and held for 1.5 min to allow for equilibration. Flow rate was 0.15 mL min−1. The column and sample compartment temperatures were maintained at 40 °C and 4 °C, respectively. Data were acquired in full MS mode. Parameters for full scan (100–1500 m/z) switch mode recorded at resolution R = 70
000 (at m/z 200) with a maximum of 3 × 106 ions collected within 200 ms. Mass spectrometric settings for ESI mode were as follows: spray voltage, 2.7 kV (ESI−) and 3.0 kV (ESI+); sheath gas flow rate, 30 L h−1; auxiliary gas flow rate, 6 L h−1; capillary temperature, 300 °C; and probe heater temperature, 325 °C. Calibration curves were generated using a concentration series of 0.5, 1.0, 2.0, 3.9, 7.8, 15.6, 31.2, 62.5, 125, 250, and 500 ng mL−1, which showed strong linearity (R2 > 0.99).
Quality assurance/quality control
Contamination was minimized by rinsing all equipment with ethanol. Product samples were all extracted in a single batch. A total of six procedure blanks in the form of empty sampling containers were incorporated into the analytical procedure. Background signals detected in blanks were subtracted from all samples for subsequent data analysis. The mean concentrations of CPs detected in the blanks (n = 6) were 0.99, 3.4, and 0.77 ng g−1 for SCCPs, MCCPs, and LCCPs, respectively. Accordingly, the method detection limits (MDLs) of SCCPs (2.97 ng g−1), MCCP (10.2 ng g−1) and LCCPs (2.31 ng g−1) were defined as three times the mean concentration measured in the blanks.
Recoveries were determined for both toys (n = 3) and electronic wires (n = 3) which showed the highest concentrations of CPs. Recoveries were also determined for hand cream (n = 3), as a representative liquid sample. Specifically, recoveries were determined by spiking CP technical mixtures (10 μL of a 5 mg L−1 solution) and an internal standard 13C10-anti-DP into 0.1 g of representative samples (PVC wire covering and plastic children's toy) with relatively low levels of measured CPs. The spiking concentration was about 10 times the concentration measured in the samples. The averaged recoveries of SCCPs, MCCPs, and LCCPs across three types of samples were determined to be 84.1 ± 9.7%, 80.0 ± 11.1%, and 80.1 ± 21.2%, respectively (Table S3†). The recovery of the internal standard 13C10-anti-DP was 84.9 ± 21.5% across all 96 product samples.
Instrumental detection limits (IDL) for this study were calculated based on multiple runs (n = 9) of the lowest concentration of calibration standards (0.5 μg L−1) using eqn (1).
|  | (1) |
where
t(99%, n−1) is the one-sided Student's
t value at the 99% confidence level for
n − 1 observations, and RSD is the relative sample standard deviation in %.
Semi-quantification of CPs
As the only chemical standards commercially available for CPs are technical mixtures, a semi-quantitative strategy derived from the method of Reth et al. was employed.19 In brief, a response factor (RF) was calculated for each technical mixture based on the sum of normalized signals of individual CPs. This was plotted against the total chlorine content of the mixtures to make a calibration curve. The curves were then used to calculate the RF of each sample based on measured chlorine content. The details of the semi-quantification method can be found in the ESI.†
Results and discussion
Analytical method development
To facilitate the analysis of CPs across diverse indoor products consisting of different materials, a high-resolution mass spectrometry-based analytical method robust against matrix effects was established. While DCM-enhanced ionization has been employed as a sensitive LC-MS method for the analysis of CPs,17,20,21 a post-column pump is required to introduce dichloromethane if chromatography is used to separate CPs (Fig. 1A). Another method has used ammonium chloride as a mobile phase additive for the analysis of chlorinated paraffins with a Time-of-Flight (ToF) mass spectrometer.22 However, due to the complexity of CPs with hundreds of homologues, the resolving power of ToF is insufficient to discriminate isotopic peaks of CPs from co-eluting interferences. For instance, a MS resolution of >50
000 is required to discriminate isotopic peaks of CPs from unsaturated CPs.23 Thus, the halide-enhanced method was employed in this study together with the Orbitrap mass spectrometer operating at 70
000 resolving power.
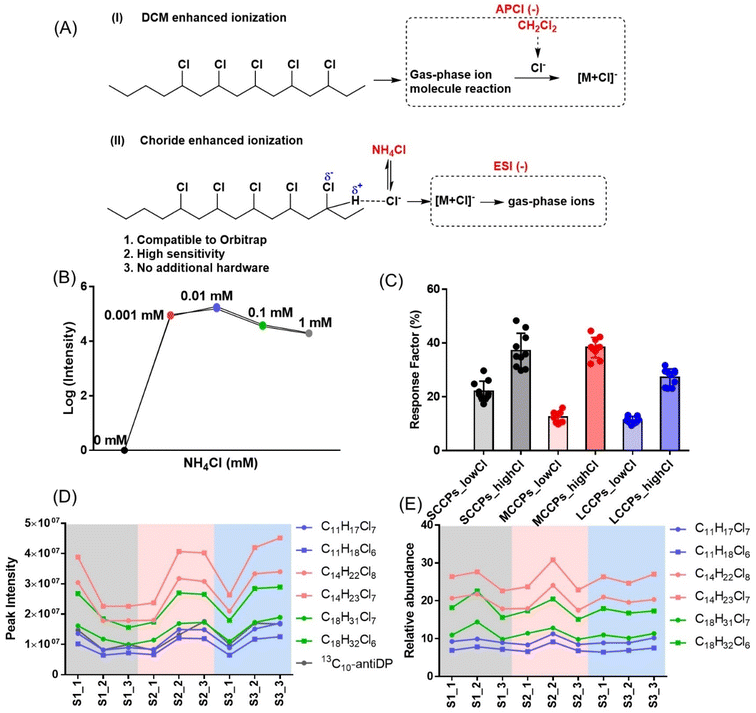 |
| Fig. 1 Development of a LC-ESI-Orbitrap method for the analysis of chlorinated paraffins. (A) Comparison of the schemes of DCM and chloride enhanced ionization. (B) The impacts of concentrations of NH4Cl in mobile phase on instrumental responses of chlorinated paraffins. Two replicates were tested. (C) The stronger response factors for chlorinated paraffins with high chlorine content (highCl) compared to low chlorine content (lowCl). (D) Variations of peak abundances of six representative CPs and internal standard (13C10-antiDP) across 3 spiked extracts and 9 injections. Sx_y indicates the yth injection of xth spiked extract. (E) Variations of abundances of six representative CPs after normalization by internal standard 13C10-antiDP. Relative abundance was calculated by dividing peak intensities of CPs by 13C10-antiDP. | |
Multiple halides including Cl−, F− and Br− were tested as additives, but ammonium chloride was found to produce the greatest improvement in sensitivity under ESI−. High sensitivity was observed even at low concentrations of ammonium chloride (0.001–0.1 mM, Fig. 1B), while signals were decreased at higher concentrations (>1 mM), likely due to the competition of chloride with analytes from ESI spray droplet surfaces. Similar to the DCM-enhanced method, stronger responses were observed for CPs with higher chlorine contents across SCCPs, MCCPs and LCCPs (Fig. 1C), probably due to the enhanced acidities of hydrogens connected to chlorocarbons. While significant fluctuations were observed across standard injections (e.g., CV = 29% for C11H18Cl6, Fig. 1D), good reproducibility was achieved when signals were normalized according to the internal standard 13C10-antiDP (CV = 10% for C11H18Cl6, Fig. 1E). The instrumental detection limits (IDLs) of this method are 0.52, 0.41 and 0.48 μg L−1 for SCCPs, MCCPs, and LCCPs respectively (Table S2†), 1–2 orders of magnitudes lower than those using DCM enhanced ionization methods (10–200 μg L−1).20 In order to take advantage of the high sensitivity, a ‘dilution and injection’ strategy was employed for the analysis of CPs in indoor products, which involved simply diluting sample extracts. Fortunately, no significant matrix effects were observed, and hence laborious SPE cleanup was not employed. This reduced the matrix effects from a wide range of product materials on CP ionizations under ESI−, thereby enhancing the accuracy of quantification. With this strategy, sufficient method detection limits (2.97, 10.2 and 2.31 ng g−1 for SCCPs, MCCPs and LCCPs) and recoveries (84.9 ± 21.5% 13C10-anti-DP across 96 product samples) were achieved to detect CPs from most investigated products (Tables S2 and S3†).
Ubiquitous detection of SCCPs in indoor products
After applying this method to indoor consumer products, SCCPs were detected in 84 of all 96 samples (representative chromatograms shown in Fig. 2). The total concentrations of SCCPs ranged from <MDL-9.34 mg g−1 across all products, with a median concentration of 0.61 μg g−1. These concentrations were generally lower than those of MCCPs (<MDL-18.7 mg g−1) but higher than LCCPs (<MDL-5.8 mg g−1) (ESI Data†). The relatively lower concentrations of SCCPs than MCCPs were consistent with a previous study on CPs in Canadian house dust.12 Among 84 products, total concentrations of CPs were detected above 10 μg g−1 for 27 of them (Fig. 3A), while the low concentrations of CPs (e.g., personal care products) in other products were likely the result of impurities or contamination from plastic containers rather than intentional ingredients. The highest concentrations of SCCPs, MCCPs and LCCPs were all detected in two electronic devices, namely the outer plastic sheath of a computer wire (product #21, 9.34 mg SCCPs per g, 18.7 mg MCCPs per g, and 5.79 mg LCCPs per g) and the outer plastic coating of a headphone wire (product #7, 3.01 mg SCCPs per g, 5.05 mg MCCPs per g, and 0.63 mg LCCPs per g) (product photos were shown in Fig. S2†). The concentrations of SCCPs and MCCPs in these two electronic devices were ∼1000 times higher than those of SCCPs (0.006 mg g−1) and MCCPs (0.019 mg g−1) measured in Canadian indoor dust,12 suggesting that they could be important indoor sources. These results are also consistent with the reports of relatively high concentrations and usage of CPs in PVC plastics collected in China.14,24 This type of usage, particularly in headphone cables, provides opportunities for human exposure directly through hand contact with the cables (followed by hand-to-mouth transfer and/or dermal transfer).
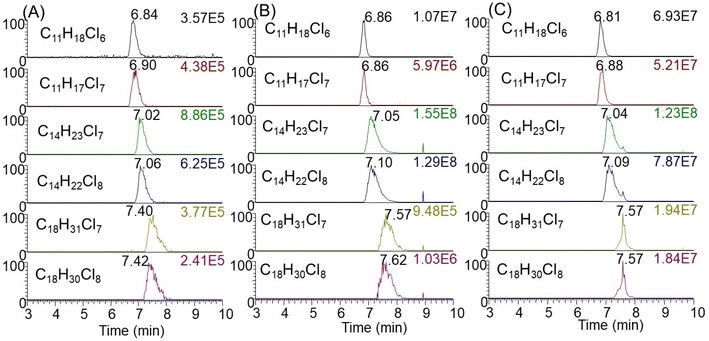 |
| Fig. 2 Representative chromatograms of SCCPs, MCCPs and LCCPs in standards (A, 10 μg L−1), extract of product #91 (B), and extract of product #21 (C). Molecular formulae are shown in corresponding chromatograms. | |
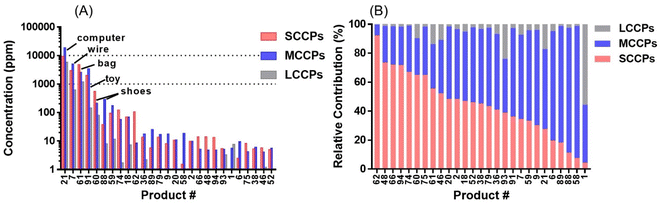 |
| Fig. 3 Concentrations and profiles of chlorinated paraffins in 27 indoor products with ΣCPs > 10 ppm. (A) Concentrations of CPs detected in products. The top 6 products are highlighted. (B) Relative contributions of SCCPs, MCCPs and LCCPs to ΣCPs in different products. | |
In addition to electronic devices, high concentrations of CPs were also detected in several toys, with the highest concentrations detected in a foam toy (product #91, Fig. S1†) at 2.02 mg SCCPs per g, 3.41 mg MCCPs per g and 0.14 mg LCCPs per g. CPs were also detected in toy packaging at relatively high concentrations (0.12 mg SCCPs per g, 0.06 mg MCCPs per g and 0.002 mg LCCPs per g). The results were surprising, as all toys were newly purchased in 2019, 7 years after the prohibition of use and/or importing of SCCPs in Canada. The finding of CPs in toys has direct implications for exposure to infants and toddlers by hand-to-mouth transfer and/or mouthing.25 Evidence indicates that indeed, toddlers (0–4 years old) had exposures to SCCPs (72 ng g−1 lipid weight in serum) that exceeded that of adults in Australia.26
Profiles of SCCPs, MCCPs and LCCPs are distinct across products
The relative contributions of SCCPs (4.4–92%), MCCPs (7.5–91%) and LCCPs (0.2–56%) varied across indoor products (Fig. 3B). The highest percentage of SCCPs relative to total CPs was detected at 92% in a shopping bag (#61), while the percentages in electronic devices were from 28% (computer wire covering, #21) to 35% (headphone wire covering, #7). This suggests that different formulations of CPs were used in the various products. This was further supported by correlation matrix analysis at the homologue level indicating that different products exhibited distinct profiles (Fig. 4A). While the CP profiles of two electronic devices (Fig. S2,† Product ID #21 and #7 as listed in Table S1†) covered a similarly broad range, C13-SCCPs and C14-MCCPs dominated the profile in a toy sample (#61). Generally, the same type of products showed stronger correlations between concentrations of CP homologues, as exemplified by two electronic devices: computer wire covering (#21) and headphone wire covering (#7) (r2 = 0.81, p < 0.001, Fig. 4B). Similarly, significant correlations were also observed between two products made of synthetic fibers (#60 and #61, r2 = 0.77, p < 0.001), or two toys (#38 and #91, r2 = 0.68, p < 0.001), while no significant correlation was observed between #7 and #61 (p = 0.98). This demonstrated that different formulations of CPs may be applied to distinct products. The discovery of product-specific CP patterns might be used in future to discriminate specific sources of CPs in indoor environments.
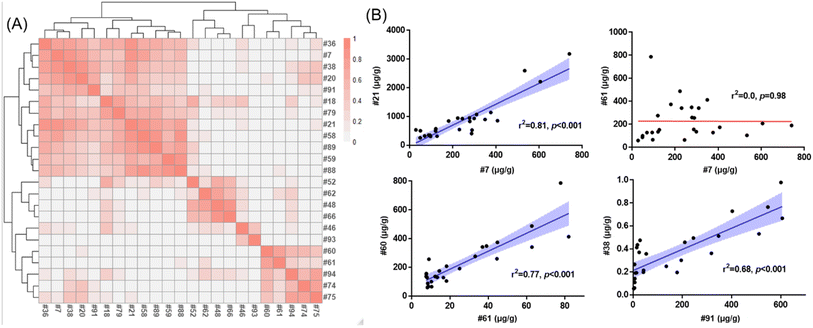 |
| Fig. 4 Clustering of 27 indoor products with ΣCPs > 10 ppm. (A) Correlation matrix of products according to concentrations of chlorinated paraffins. Only Homologues exceeding 1% of total concentrations were used for correlation analysis. The color is proportional to r2. (B) Correlation analysis of 6 representative products. Note that the same type of products generally show stronger correlations. | |
Detection of a new class of carboxylate derivates of CPs
Inspired by the high concentrations of CPs detected in electronic devices including the computer wire covering (#21), we decided to perform nontargeted analysis to check if other chlorinated chemical additives might be detected in the same samples. To achieve this, we employed an in-house R script to screen chlorinated compounds by filtering peak features with characteristic chlorine isotopic peaks.27,28 Four chlorinated compounds were tentatively identified with this strategy, with similar retention times between 7.08–8.23 min (Fig. 5A). Their m/z values differed by 33.9602, which indicated that they were homologues with different numbers of chlorine atoms. The formulae of these compounds were predicted as C16HxCl4−7O2 within a mass error of 2 ppm, by constraining the number of chlorine atoms according to their isotopic peak patterns. For instance, the most abundant compound detected at retention time 7.45 min and m/z 391.0776, was determined to contain four chlorines according to its isotopic peak pattern (0.77
:
1
:
0.49
:
0.11
:
0.01, Fig. 5B). Accordingly, the formula of this compound was predicted as C16H27Cl4O2 (0.64 ppm). To double check if these compounds are carboxylates, alcohol or carbonyl compounds, MS2 spectra were collected for these compounds. Unfortunately, no informative fragments were detected to support their structure assignment. We then checked the MS1 spectra in ESI+ mode, but these compounds were not detected. As carbonyls including ketones or aldehydes typically showed good ionization efficiency in ESI+, the absence of signals in ESI+ excluded these compounds as ketones or aldehydes. In addition, when pure methanol and water were used as the mobile phase, the instrumental signals of these compounds remain unchanged. This supported the class of compounds as carboxylates, rather than chloride/acetate adducts, or alcohols.
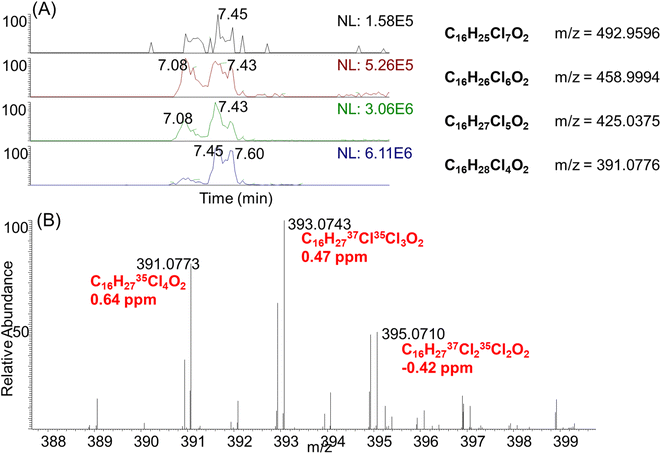 |
| Fig. 5 Detection of a novel class of carboxylic acid derivatives of chlorinated paraffins in product #21. (A) Chromatograms of four representative carboxylic acid derivatives and their proposed formulae. (B) High-resolution MS1 spectra of chemical C16H27Cl4O2. | |
Using retention time and exact mass, we also manually inspected the mass spectra of other indoor products but these carboxylates were only detected in the computer wire covering (#21). This product was purchased five years before the sample extraction, and thus carboxylate derivatives might be formed through oxidation from CPs in the indoor environment. Indeed, the autooxidation of both unsaturated and saturated fatty acids have been well documented through radical reactions.29 Alternatively, as carboxylates were only detected for the C16 homologues, these compounds might be produced as impurities of chlorinated paraffins. It is well known that linear carboxylates such as per- and polyfluoroalkyl substances (PFAS), can mimic endogenous fatty acids by binding to peroxisome proliferator-activated receptors (PPARs) and lipid-binding proteins.30,31 Indeed, a previous study has reported the PPARα activities of SCCPs by using a reporter assay.32 Future studies are warranted to investigate the potential contribution of carboxylate derivatives to the PPARα activities of CP mixtures.
Conclusion
While CPs were prohibited from manufacture, sale, use or in imported finish goods in Canada under the Canadian Environmental Protection Act which came into force in 2013, this study revealed the ubiquitous detection of SCCPs, MCCPs, and LCCPs in a wide range of indoor products purchased after 2013. While lower concentrations (<100 mg g−1) of CPs in some indoor products might be from impurities, higher concentrations of SCCPs, MCCPs, and LCCPs were detected in toys and electronic devices of up to 0.93%, 1.9%, and 0.58% by weight, which would seem to indicate intentional use. The presence of SCCPs in these products is of particular concern as infants, toddlers and young children are vulnerable to exposures from such sources.
While Canada has legislative controls for CPs as noted above, the presence of CPs (and possibly other “toxic” compounds) in products clearly shows the challenge of ensuring compliance with the regulation which requires on-going product testing followed by punitive action against those contravening the regulation such as importers. However, the analysis of CPs is technically challenging, which poses difficulties to the implementation of a regulatory testing program. The results presented in this study demonstrate an urgent need for on-going product testing (followed by enforcement) which could be enabled by coordination between scientific communities and government.
The source of these products was not clear, except for the toys that were manufactured overseas. However, many of these types of products purchased in Canada are manufactured for international markets. As such, we believe that these would be available in the US and European markets and worldwide. The ubiquity of CPs in toys and electronic devices commonly used worldwide could benefit from concerted and coordinated international efforts to stem their production and international trade.
Author contributions
Steven Kutarna: investigation, methodology, writing; Xuan Du, methodology; Miriam L. Diamond, supervision, review & editing; Arlene Blum, review & editing; Hui Peng, supervision, funding acquisition, writing.
Conflicts of interest
There are no conflicts to declare.
Acknowledgements
Hui Peng was supported by the Great Lakes Protection Initiatives (GLPI, GCXE19P017) from Environment and Climate Change Canada, and the National Sciences and Engineering Research Council (NSERC) Discovery Grant. The authors acknowledge the support of instrumentation grants from the Canada Foundation for Innovation, the Ontario Research Fund, the NSERC Research Tools and Instrument Grant. We thank Lorrie Hayes and Luke Cayley of Environment and Climate Change Canada for information on Canadian SCCPs regulations.
References
-
G. Filyk, L. Lander and M. Eggleton, Short Chain Chlorinated Paraffins (SCCPs) Substance Dossier (Final Draft II), Environment Canada, Canada, 2002 Search PubMed.
- C. X. Zhang, H. Chang, H. P. Wang, Y. R. Zhu, X. L. Zhao and Y. He,
et al., Spatial and temporal distributions of short-, medium-, and long-chain chlorinated paraffins in sediment cores from nine lakes in China, Environ Sci Technol, 2019, 53(16), 9462–9471 CrossRef CAS PubMed.
- H. P. Wang, H. Chang, C. X. Zhang and F. C. Wu, Occurrence and mass balance of medium- and long-chain chlorinated paraffins in a municipal sewage treatment plant: Comparison to short-chain compounds, Environ Int, 2019, 133, 105273 CrossRef CAS PubMed.
- D. D. Cao, W. Gao, J. Wu, K. Lv, S. Z. Xin and Y. W. Wang,
et al., Occurrence and human exposure assessment of short- and medium-chain chlorinated paraffins in dusts from plastic sports courts and synthetic turf in Beijing, China, Environ Sci Technol, 2019, 53(1), 443–451 CrossRef CAS PubMed.
- H. Chen, J. C. W. Lam, M. S. Zhu, F. Wang, W. Zhou and B. B. Du,
et al., Combined effects of dust and dietary exposure of occupational workers and local residents to short- and medium-chain chlorinated paraffins in a mega e-waste recycling industrial park in south China, Environ Sci Technol, 2018, 52(20), 11510–11519 CAS.
- H. J. Li, D. Bu, J. J. Fu, Y. Gao, Z. Y. Cong and G. S. Zhang,
et al., Trophic dilution of short-chain chlorinated paraffins in a plant-plateau pika-eagle food chain from the Tibetan Plateau, Environ Sci Technol, 2019, 53(16), 9472–9480 CrossRef CAS PubMed.
- X. Y. Du, B. Yuan, Y. H. Zhou, Z. Y. Zheng, Y. Wu and Y. L. Qiu,
et al., Tissue-specific accumulation, sexual difference, and maternal transfer of chlorinated paraffins in black-spotted frogs, Environ Sci Technol, 2019, 53(9), 4739–4746 CrossRef CAS PubMed.
- M. Aamir, S. S. Yin, F. J. Guo, K. Liu, C. Y. Xu and W. P. Liu, Congener-specific mother-fetus distribution, placental retention, and transport of C10–13 and C14–17 chlorinated paraffins in pregnant women, Environ Sci Technol, 2019, 53(19), 11458–11466 CrossRef CAS PubMed.
-
UNEP, Short-Chain Chlorinated Paraffins (SCCPs): Draft Risk Management Evaluation, 2016 Search PubMed.
- Y. Guida, R. Capella and R. Weber, Chlorinated paraffins in the technosphere: a review of available information and data gaps demonstrating the need to support the Stockholm Convention implementation, Emerging Contaminants, 2020, 6, 143–154 CrossRef.
-
https://gazette.gc.ca/rp-pr/p2/2011/2011-10-12/html/sor-dors212-eng.html
.
- H. Shang, X. Fan, C. Kubwabo and P. E. Rasmussen, Short-chain and medium-chain chlorinated paraffins in Canadian house dust and NIST SRM 2585, Environmental science and pollution research international, 2019, 26(8), 7453–7462 CrossRef CAS PubMed.
- J. Glüge, Z. Wang, C. Bogdal, M. Scheringer and K. Hungerbühler, Global production, use, and emission volumes of short-chain chlorinated paraffins – a minimum scenario, The Science of the total environment, 2016, 573, 1132–1146 CrossRef PubMed.
- C. Chen, A. Chen, L. Li, W. Peng, R. Weber and J. Liu, Distribution and Emission Estimation of Short- and Medium-Chain Chlorinated Paraffins in Chinese Products through Detection-Based Mass Balancing, Environ Sci Technol, 2021, 55(11), 7335–7343 CrossRef CAS PubMed.
- B. Yuan, A. Strid, P. O. Darnerud, C. A. de Wit, J. Nystrom and A. Bergman, Chlorinated paraffins leaking from hand blenders can lead to significant human exposures, Environ Int, 2017, 109, 73–80 CrossRef CAS PubMed.
- C. Gallistl, J. Sprengel and W. Vetter, High levels of medium-chain chlorinated paraffins and polybrominated diphenyl ethers on the inside of several household baking oven doors, Sci Total Environ, 2018, 615, 1019–1027 CrossRef CAS PubMed.
- B. Yuan, J. H. Tay, E. Papadopoulou, L. S. Haug, J. A. Padilla-Sanchez and C. A. de Wit, Complex Mixtures of Chlorinated Paraffins Found in Hand Wipes of a Norwegian Cohort, Environ Sci Technol Lett, 2020, 7(3), 198–205 CrossRef CAS PubMed.
- C. Chaemfa, Y. Xu, J. Li, P. Chakraborty, J. Hussain Syed and R. Naseem Malik,
et al., Screening of atmospheric short- and medium-chain chlorinated paraffins in India and Pakistan using polyurethane foam based passive air sampler, Environ Sci Technol, 2014, 48(9), 4799–4808 CrossRef CAS PubMed.
- M. Reth, Z. Zencak and M. Oehme, New quantification procedure for the analysis of chlorinated paraffins using electron capture negative ionization mass spectrometry, Journal of chromatography A, 2005, 1081(2), 225–231 CrossRef CAS PubMed.
- T. Li, Y. Wan, S. X. Gao, B. L. Wang and J. Y. Hu, High-throughput determination and characterization of short-, medium-, and long-chain chlorinated paraffins in human blood, Environ Sci Technol, 2017, 51(6), 3346–3354 CrossRef CAS PubMed.
- B. Yuan, T. Alsberg, C. Bogdal, M. MacLeod, U. Berger and W. Gao,
et al., Deconvolution of soft ionization mass spectra of chlorinated paraffins to resolve congener groups, Anal Chem, 2016, 88(18), 8980–8988 CrossRef CAS PubMed.
- L. Zheng, L. Lian, J. Nie, Y. Song, S. Yan and D. Yin,
et al., Development of an ammonium chloride-enhanced thermal-assisted-ESI LC-HRMS method for the characterization of chlorinated paraffins, Environ. Pollut., 2019, 255(2), 113303 CrossRef CAS PubMed.
- T. Li, S. Gao, Y. Ben, H. Zhang, Q. Kang and Y. Wan, Screening of Chlorinated Paraffins and Unsaturated Analogues in Commercial Mixtures: Confirmation of Their Occurrences in the Atmosphere, Environ Sci Technol, 2018, 52(4), 1862–1870 CrossRef CAS PubMed.
- C. Wang, W. Gao, Y. Liang, Y. Wang and G. Jiang, Concentrations and congener profiles of chlorinated paraffins in domestic polymeric products in China, Environ. Pollut., 2018, 238, 326–335 CrossRef CAS PubMed.
- L. Shi, Y. Gao, H. Zhang, N. Geng, J. Xu and F. Zhan,
et al., Concentrations of short- and medium-chain chlorinated paraffins in indoor dusts from malls in China: implications for human exposure, Chemosphere, 2017, 172, 103–110 CrossRef CAS PubMed.
- L. M. van Mourik, L. L. Toms, C. He, A. Banks, P. Hobson and P. E. G. Leonards,
et al., Evaluating age and temporal trends of chlorinated paraffins in pooled serum collected from males in Australia between 2004 and 2015, Chemosphere, 2020, 244, 125574 CrossRef CAS PubMed.
- S. Kutarna, S. Tang, X. Hu and H. Peng, Enhanced Nontarget Screening Algorithm Reveals Highly Abundant Chlorinated Azo Dye Compounds in House Dust, Environ Sci Technol, 2021, 55(8), 4729–4739 CrossRef CAS PubMed.
- H. Peng, C. L. Chen, D. M. V. Saunders, J. X. Sun, S. Tang and G. Codling,
et al., Untargeted identification of organo-bromine compounds in lake sediments by ultrahigh-resolution mass spectrometry with the Data-Independent Precursor Isolation and Characteristic Fragment method, Anal Chem, 2015, 87(20), 10237–10246 CrossRef CAS PubMed.
- J. Wang and X. Zhang, Free-Radical-Initiated Phospholipid Oxidations at the Air–Water Interface: The Oxidation of Unsaturated and Saturated Fatty Acid Chains, The journal of physical chemistry A, 2021, 125(4), 973–979 CrossRef CAS PubMed.
- D. W. Yang, J. J. Han, D. R. Hall, J. X. Sun, J. Fu and S. Kutarna,
et al., Nontarget Screening of Per- and Polyfluoroalkyl Substances Binding to Human Liver Fatty Acid Binding Protein, Environ Sci Technol, 2020, 54(9), 5676–5686 CrossRef CAS PubMed.
- A. E. Kurtz, J. L. Reiner, K. L. West and B. A. Jensen, Perfluorinated Alkyl Acids in Hawaiian Cetaceans and Potential Biomarkers of Effect: Peroxisome Proliferator-Activated Receptor Alpha and Cytochrome P450 4A, Environ Sci Technol, 2019, 53(5), 2830–2839 CrossRef CAS PubMed.
- Y. Gong, H. Zhang, N. Geng, X. Ren, J. P. Giesy and Y. Luo,
et al., Short-chain chlorinated paraffins (SCCPs) disrupt hepatic fatty acid metabolism in liver of male rat via interacting with peroxisome proliferator-activated receptor α (PPARα), Ecotoxicol Environ Saf, 2019, 181, 164–171 CrossRef CAS PubMed.
|
This journal is © The Royal Society of Chemistry 2023 |
Click here to see how this site uses Cookies. View our privacy policy here.