DOI:
10.1039/D3DT01357J
(Paper)
Dalton Trans., 2023,
52, 7558-7563
Simple synthetic access to [Au(IBiox)Cl] complexes†
Received
8th May 2023
, Accepted 9th May 2023
First published on 10th May 2023
Abstract
Green and sustainable access to chiral and achiral gold-IBiox complexes is reported. The gold complexes were synthesized using a simple, air-tolerant, weak base protocol carried out in a green solvent. Their catalytic activity was examined in the hydroamination of alkynes. The steric protection afforded the gold center by these ligands was quantified using the %Vbur model and compared with the most commonly encountered NHCs.
Introduction
The vast potential of N-heterocyclic carbenes (NHCs) as ancillary ligands in transition metal catalysis has been demonstrated and thoroughly explored during the last two decades.1–3 However, some families of NHC ligands, specifically the IBiox series, first reported by Glorius,4–7 have been much less explored when compared to the extensive work performed with classical N-heterocyclic carbenes, such as IPr (IPr = [1,3-bis (diisopropylphenyl(imidazolyl-2-ylidene)]).8 The reason behind this less frequent use is surely associated with the multistep synthetic sequence required to achieve their assembly. Consequently, only a limited number of reports have dealt with the synthesis of IBiox metal complexes.4,7,9–14 For instance [Pd(IBiox)Cl2]2 complexes were synthesized using IBiox·HOTf salts, Pd(OAc)2 and LiCl, in THF at 100 °C for 16 h.4,9 Another complex, [Pd(IBioxiPr)2I2], one where two IBiox ligands are bound to the metal was obtained using the IBiox triflate salt and Pd(OAc)2, with KOtBu as base in THF as solvent.7 [Pd(IBiox)(π-allyl)Cl] complexes have also been synthesized using a strong base, NaOtBu, in dimethoxyethane (DME).10 There are also several examples of various rhodium complexes such as [Rh(IBiox)(COE)Cl]2 which were obtained via the free carbene route in pentane from [Rh(COE)2Cl]2.11 In addition, complexes with the general formula [Rh(IBiox)(L)Cl] (L = COE, NBD, COD) are also reported again via free carbene route with the use of strong bases (KOtBu) in benzene.11,12 Other examples of [M(IBiox)(CO)2Cl] (M = Rh, Ir) complexes can be found in the literature, synthesized via the transmetallation pathway from [Ag(IBiox)Br] in the case of rhodium,13 and via the free carbene route for the Ir complex.4 To the best of our knowledge, there is only one example of gold-IBiox complex, which was synthesized from IBiox·HOTf salt with AuCl in the presence of NaOtBu in THF under inert atmosphere. The desired complex was obtained in a very low yield (42%) (Scheme 1).14
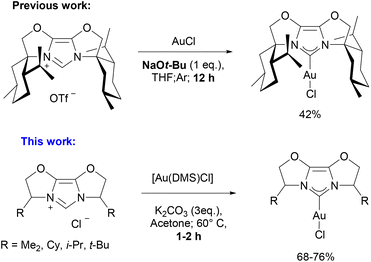 |
| Scheme 1 Comparison of a previously reported synthetic procedures to [Au(IBiox)Cl] complexes with the “weak base” route. | |
In spite of the difficult access to this family of NHCs, metal complexes (predominantly with palladium and rhodium) of IBiox ligands have found multiple uses in catalysis.4,9,11,14–16 Despite the fact that these compounds are of great interest, synthetic routes towards them are quite complicated and usually involve strong bases, toxic solvents and require manipulations under an inert atmosphere.
Therefore, the development of a more user-friendly and efficient synthetic pathway towards M-IBiox complexes would be highly desirable to help democratise their use in catalysis.
Results and discussion
Synthesis of [Au(IBiox)Cl] complexes
Since 2013, when Nolan17 and Gimeno18 independently reported simple and efficient approaches to Au–NHC complexes using weak bases, this “weak base route”19,20 has been extended to numerous metal–NHC syntheses.21–25 We now extend the range of NHC ligands compatible with this weak base route to the IBiox ligand family with a focus on Au-IBiox complexes (Scheme 1). The classical synthetic route employed to access IBiox ligands usually leads to the isolation of triflate IBiox salts.4–7 Therefore, our initial attempts to synthesize Au-IBiox complexes using the weak base route made direct use of these IBiox·HOTf salts. Unfortunately, no reaction was observed in this instance when a weak base was used. The concerted mechanism involving the weak base easily rationalises this failure as the nature of the counterion is crucial in dictating the feasibility of product formation. The approach works best with halides as counterion.19 We next converted the triflate IBiox salts to their chloride congeners (IBiox·HCl) as illustrated in Scheme 2. In the present series, we have targeted for synthesis and further metalation, two chiral (IBioxiPr, IBioxtBu) and two achiral (IBioxMe4, IBiox6) NHCs. In all cases, the desired IBiox·HCl salts were easily isolated in high yields in microcrystalline forms (Scheme 2).
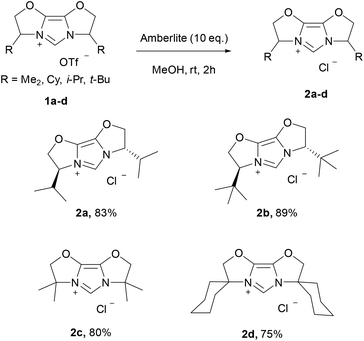 |
| Scheme 2 Synthesis of IBiox·HCl salts. | |
Having 2a–d in hand, we next turned our attention to the metalation (auration) using our weak base route. Gratifyingly, gold complexes bearing IBiox ligands were synthesized in high yields under much greener reaction conditions, namely by using green acetone as solvent at 60 °C with potassium carbonate as the base (Scheme 3). Reaction times of only one hour were needed to achieve full conversion of IBioxMe4 and IBiox6 salts to the desired Au-IBiox complexes. Slightly longer reaction times of 1.5 and 2 hours were needed for IBioxiPr and IBioxtBu salts respectively.
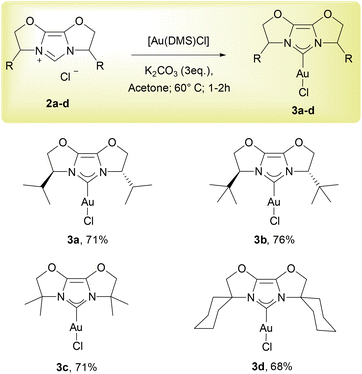 |
| Scheme 3 Synthesis of gold-IBiox complexes via weak base route. | |
To the best of our knowledge, a reported reaction time of 12 hours was needed to reach full conversion for [Au(IBiox[(−)-menthyl]Cl] complex.14 Au-IBiox complexes 3a–d were successfully isolated and fully characterized following this simple protocol and a facile workup (see ESI†).
The molecular structures of the obtained gold complexes (3a–d) were unambiguously determined by diffraction studies on single crystals (Fig. 1). Crystals suitable for X-ray diffraction were grown by slow vapour diffusion of pentane into saturated CH2Cl2 solutions of the complexes.
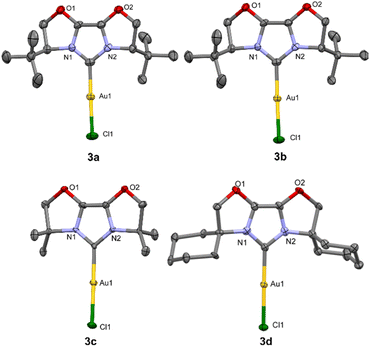 |
| Fig. 1 X-ray molecular structures of complexes 3a–d are presented (2238228–2238230, 2253267†) showing thermal displacement ellipsoids at the 30% probability level (3a) and at the 60% probability level (3b–d), hydrogen atoms are omitted for clarity. | |
Selected structural data for these compounds are presented in Table 1. Due to various conformation of cyclohexane rings, two non-equivalent molecules in the crystal unit cell were observed for 3d. Overall, metrical parameters, (bond lengths and angles) for the synthesized series of IBiox complexes are very similar. Minor deviations from linear geometry are observed in all complexes. The longest Au–CNHC bond is observed for complex 3b.
Table 1 Selected bond lengths (Å) and angles (°) for each complex
Complex |
CNHC–Au |
Au–Cl |
CNHC–Au–Cl |
Metrical parameters are the average values for two independent molecules found in the unit cell.
|
3a
|
1.970(7) |
2.2833(19) |
179.0(2) |
3b
|
1.985(5) |
2.2831(15) |
178.16(16) |
3c
|
1.979(9) |
2.284(2) |
178.7(3) |
3d
|
1.97(2) |
2.276(5) |
178.1(6) |
Interestingly, when compared with the classical [Au(IPr)Cl] complex, the Au–CNHC bond length in [Au(IPr)Cl] is significantly shorter (1.942(3) Å) than those reported here.26 However, the same bonds in [Au(IMes)Cl], [Au(It-Bu)Cl] and [Au(ICy)Cl] are longer than in the IBiox complexes.26 Complexes 3b and 3c have similar Au–CNHC bond lengths as [Au(SIPr)Cl], [Au(SIMes)Cl], [Au(IMe)Cl] and [Au(IAd)Cl]. Au–CNHC bond lengths in complexes 3a and 3d are shorter than in the reported classical NHCs with the exception of [Au(IPr)Cl].26 The Au–Cl bond lengths in 3d are similar to the ones found in [Au(SIMes)Cl], [Au(IMes)Cl], [Au(SIPr)Cl], [Au(IPrMe)Cl] and [Au(IAd)Cl]. The Au–Cl bond lengths in [Au(IBiox)Cl] complexes 3a–3c are similar to the ones found in [Au(ICy)Cl] and [Au(IMe)Cl].26
Percent buried volume (%Vbur) of [Au(IBiox)Cl] complexes
To understand the origin of these variations, we next focused on evaluating the steric pressure (or protection) afforded by the IBiox ligands to the gold center. To permit such an analysis, the percent buried volume27,28 (%Vbur), a steric descriptor initially conceived to addressed the non-cone angle depiction of NHC ligands, was used. The large database available for [Au(NHC)Cl] molecular structures make these a convenient scaffold for comparison with other NHC ligand classes.29 Although percent buried volumes of IBiox ligands have already been reported for [PdL(π-allyl)Cl] compounds, these were determined from DFT-optimized geometries instead of X-ray structures.10 To the best of our knowledge, there is only one gold-IBiox complex described in the literature, namely [Au(IBiox[(−)-menthyl]Cl], for which a %Vbur value was calculated from X-ray molecular structural data.14,30 Therefore, we next calculated %Vbur values for the presented IBiox ligands in [Au(IBiox)Cl] complexes in order to compare them with previously reported NHC %Vbur values (Fig. 2).29,30
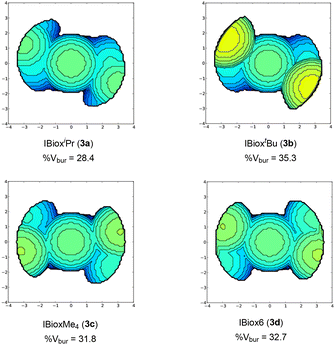 |
| Fig. 2 Steric maps and buried volumes of IBiox ligands in [Au(IBiox)Cl] complexes: NHC structures were extracted from crystal structures (r = 3.5 Å, d = 2.0 Å, Bondi radii scaled by 1.17) to perform the calculations. | |
The steric maps and %Vbur for [Au(IBiox)Cl] complexes were computed both from X-ray molecular structural data and DFT-optimized geometries (Fig. S1, ESI†) using the SambVca 2.1 developed by Cavallo group.31 Despite small variation of %Vbur between X-ray and DFT-optimized structures, among the studied IBiox ligands IBioxtBu (3b) has the largest %Vbur value (35.3), which is still significantly small compared with the previously reported [Au(IBiox[(−)-menthyl]Cl] (47.7).30
IBioxiPr (3a) has the smallest %Vbur value among the NHCs examined in the [Au(IBiox)Cl] series. Notably, all ligands under investigation display two more occupied and two less occupied quadrants (Fig. 2). Percent buried volumes of the IBiox ligands in gold complexes (3a–3d) are smaller compared with commonly used NHCs such as IPr (44.5), SIPr (47.0), SIMes (36.9), IMes (36.5) and IAd (39.8).29,30 However, values of %Vbur for (3a–3d) are larger than ICy (27.4) ligand.26,29,32
Hydroamination of phenylacetylene
We next turned our attention to potential uses of these Au-IBiox complexes in catalysis. During the last decade a vast number of gold catalysts, bearing both phosphines and NHC ligands, have successfully been deployed as catalysts in the hydroamination of C–C unsaturated bearing compounds.33–36 We, therefore, thought this a good model reaction to further examine the steric influence of the IBiox architecture but this time taking as an observable catalytic reactivity of the Au-IBiox complexes in the hydroamination of phenylacetylene. Our initial screening makes use of the reaction conditions developed by the Gessner group36 for this reaction, namely the use of aniline (1 eq.), phenylacetylene (1 eq.), LAuCl
:
NaBArF = 1
:
1, 50 °C, in the absence of solvent (neat) (Table 2). Reactions reached full conversion when complexes 3b and 3d, bearing the bulkiest ligands, were used as pre-catalysts. In the case of [Au(IBioxiPr)Cl], the lowest NMR yield was observed (84%) and this after a reaction time of 24 hours.
Table 2 [Au(IBiox)Cl] complexes in hydroamination of phenylacetylene
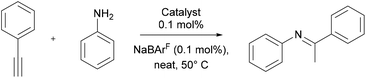
|
|
NMR yield (%) |
Time (h)/catalysts |
3a
|
3b
|
3c
|
3d
|
0.5 |
34 |
47 |
32 |
47 |
1 |
47 |
58 |
42 |
57 |
1.5 |
52 |
67 |
52 |
65 |
2 |
57 |
69 |
53 |
70 |
3 |
64 |
75 |
59 |
76 |
24 |
84 |
99 |
91 |
98 |
Notably, all tested [Au(IBiox)Cl] complexes displayed better performance than the conversions obtained with phosphine ligated [Au(L)Cl] complexes, where L = Y
PhPCy
2, Y
pOMePCy
2, Y
pCF3PCy
2, PPh
3.
36 For all the phosphine-bearing catalysts full conversion was not reached after 24 hours. The best results were observed in the case of [Au(PPh
3)Cl] and [Au(Y
pOMePCy
2)Cl], 39% and 42% NMR yields respectively, after 24 h. However, [Au(
CyJohnPhos)Cl] together with [Au(Y
oTolPCy
2)Cl] and [Au(Y
MesPCy
2)Cl] showed higher activity than the Au-IBiox complexes: 95%, 93% and 92% NMR yields were obtained after 2 hours. Nevertheless, initial rates of reaction for
3b and
3d are faster than for [Au(IPr)Cl] – one of the most commonly used gold catalyst. A 93% NMR yield was observed after 24 h with the latter.
36
Conclusions
In summary, we have synthesized gold complexes, bearing various chiral and achiral IBiox ligands via a simple and sustainable synthetic route. The molecular structures of 3a–d complexes were determined by X-ray diffraction. The obtained structural data were compared with previously reported NHC–Au congeners. Percent buried volumes for the Au-IBiox complexes 3a–d were calculated for the first time from XRD data and compared with the other reported IBiox ligand and with commonly used NHCs. IBioxtBu proves the bulkiest ligand among the studies series. Moreover, catalytic activity of the obtained gold–NHC complexes was examined in the hydroamination of phenylacetylene. Complexes 3b and 3d with the bulkiest ligands showed better results than the other IBiox congeners. It is worth noting that all tested complexes proved more active in the examined reaction than previously reported complexes with ylide-functionalized phosphines or PPh3 as ancillary ligand. Implementation of the described synthetic method to other transition metals and further exploration of properties of the IBiox family are ongoing in our laboratories.
Experimental
General information
All reactions were carried out in air, unless otherwise noted. Solvents and all other reagents were purchased and used as received without further purification unless otherwise stated. All IBiox·HOTf salts were synthesized according to known procedures.4–7 Unless otherwise noted, HPLC grade methanol, reagent grade acetone, DCM, pentane, Et2O and freshly crushed, anhydrous K2CO3 were used. Amberlite IRA-400 was used as supplied by Sigma-Aldrich. 1H, 13C–{1H} Nuclear Magnetic Resonance (NMR) spectra were recorded on a Bruker Avance 400 Ultrashield or Bruker Avance 300 Ultrashield spectrometer at 298 K using the residual solvent peak as reference (CDCl3: δH = 7.26 ppm, δC = 77.16 ppm; CD2Cl2: δH = 5.32 ppm, δC = 54.00 ppm). Peaks are assigned as: s (singlet), d (doublet), t (triplet), h (septet), dd (doublet of doublets), td (triplet of doublets) and m (multiplet). Elemental analyses were performed at Université de Namur, rue de Bruxelles, 55 B-5000 Namur, Belgium.
Experimental procedures
General procedure for the synthesis of IBiox·HCl salts.
A 20 mL scintillation vial equipped with a septum cap and a stirring bar was charged with IBiox·HOTf, amberlite (10 mass eq.) in MeOH (0.07 M). The reaction mixture was stirred at room temperature for 2 h. The mixture was filtered through the frit, then washed with DCM (3 times). Evaporation of the solvent, washing with pentane (3 × 3 mL) or recrystallization in the following mixtures of solvents DCM/pentane or in MeOH/Et2O, followed with drying under high vacuum afforded the products as powders.
Synthesis of (3S,7S)-3,7-diisopropyl-2,3,7,8-tetrahydroimidazo[4,3-b:5,1-b′]bis(oxazole)-4-ium chloride (IBioxiPr·HCl) (2a).
Complex 2a was synthesized according to general procedure from IBioxiPr·HOTf (0.42 mmol, 0.161 g), amberlite (10 mass eq., 1.6 g) in 5 ml of MeOH. Recrystallization in MeOH/Et2O. White powder, 94.4 mg (83%).
1H NMR (300 MHz, chloroform-d) δ 10.39 (s, 1H, CH), 5.09–4.91 (m, 4H), 4.88–4.79 (m, 2H), 2.62–2.47 (m, 2H, CH–CH3), 1.08 (d, J = 6.9 Hz, 6H, CH3), 1.00 (d, J = 6.9 Hz, 6H, CH3).
13C NMR (75 MHz, chloroform-d) δ 125.5 (C–O), 118.6 (CH), 78.9 (O–CH2), 64.1(CH2–CH), 31.2 (CH–CH3), 18.2 (CH3), 16.8 (CH3).
HRMS (ESI-TOF): calcd for C13H21ClN2O2+ [M]+: 237.1603; found: 237.1588.
Synthesis of (3S,7S)-3,7-di-tert-butyl-2,3,7,8-tetrahydroimidazo[4,3-b:5,1-b′]bis(oxazole)-4-ium chloride (IBioxtBu·HCl) (2b).
Complex 2b was synthesized according to general procedure from IBioxtBu·HOTf (0.51 mmol, 0.209 g), amberlite (10 mass eq., 2 g) in 6.4 ml of MeOH. Recrystallization in DCM/pentane. White powder, 136.6 mg (89%).
1H NMR (400 MHz, chloroform-d) δ 9.75 (s, 1H, CH), 5.10–4.98 (m, 2H), 4.95–4.84 (m, 4H), 1.12 (s, 18H, CH3).
13C NMR (101 MHz, chloroform-d) δ 125.7 (C–O), 119.2 (CH), 79.0 (O–CH2), 68.3 (CH2–CH), 34.3 (C–(CH3)3), 25.8 (CH3).
HRMS (ESI-TOF): calcd for C15H25ClN2O2+ [M]+: 265.1916; found: 265.1901.
Synthesis of 3,3,7,7-tetramethyl-2,3,7,8-tetrahydroimidazo[4,3-b:5,1-b′]bis(oxazole)-4-ium chloride (IBioxMe4·HCl) (2c).
Complex 2c was synthesized according to general procedure from IBioxMe4·HOTf (0.49 mmol, 0.174 g), amberlite (10 mass eq., 1.7 g) in 5.4 ml of MeOH. Recrystallization in DCM/pentane. White powder, 115.6 mg (97%).
1H NMR (300 MHz, chloroform-d) δ 11.06 (s, 1H, CH), 4.64 (s, 4H, CH2), 1.80 (s, 12H, CH3).
13C NMR (101 MHz, chloroform-d) δ 124.5 (C–O), 116.8 (CH), 88.5 (O–CH2), 64.6 (C–(CH3)2), 26.0 (CH3).
HRMS (ESI-TOF): calcd for C11H17ClN2O2+ [M]+: 209.1290; found: 209.1275.
Synthesis of 2′H,8′H-dispiro[cyclohexane-1,3′-imidazo[4,3-b:5,1-b′]bis(oxazole)-7′,1′′-cyclohexan]-4′-ium chloride (IBiox6·HCl) (2d).
Complex 2d was synthesized according to general procedure from IBiox6·HOTf (0.44 mmol, 0.192 g), amberlite (10 mass eq., 1.9 g) in 6 ml of MeOH. Recrystallization in DCM/pentane. White powder, 110.7 mg (75%).
1H NMR (300 MHz, chloroform-d) δ 11.18 (s, 1H, CH), 4.77 (s, 4H, O–CH2), 2.64–2.32 (m, 4H, CH2), 2.11–1.82 (m, 8H, CH2), 1.66–1.54 (m, 4H, CH2), 1.43–1.23 (m, 4H, CH2).
13C NMR (101 MHz, chloroform-d) δ 124.1(C–O), 117.3 (CH), 85.8 (O–CH2), 67.4 (C–CH3), 35.1(CH2), 23.7 (CH2), 23.4 (CH2).
HRMS (ESI-TOF): calcd for C17H25ClN2O2+ [M]+: 289.1916; found: 289.1903.
General procedure for the synthesis of [Au(IBiox)Cl] complexes
A 4 mL scintillation vial equipped with a septum cap and a stirring bar was charged with IBiox·HCl (1 eq.), [Au(DMS)Cl] (1 eq.), K2CO3 (3 eq.) and acetone (0.5–0.8 mL). The reaction mixture was stirred at 60° C for 1.5–2 h. The solvent was removed under vacuum and purification of the product was carried out by filtration through a millipore membrane filter with DCM (4 mL). Evaporation of the solvent and drying under high vacuum afforded the products as powders.
Synthesis of [Au(IBioxiPr)Cl] (3a).
Complex 3a was synthesized according to general procedure from IBioxiPr·HCl (100 mg, 0.367 mmol), [Au(DMS)Cl] (108 mg, 0.367 mmol), K2CO3 (152.1 mg, 1.101 mmol) and acetone (0.8 mL), 1.5 h. White powder, 122.1 mg (71%).
1H NMR (400 MHz, chloroform-d) δ 4.91–4.82 (m, 2H), 4.78–4.69 (m, 2H), 4.47–4.36 (m, 2H), 2.89–2.80 (m, 2H, CH–CH3), 1.02 (d, J = 7.0 Hz, 6H, CH3), 0.87 (d, J = 6.8 Hz, 6H, CH3).
13C NMR (101 MHz, chloroform-d) δ 124.9 (C–Au), 76.5, 62.2, 30.8 (CH–CH3), 18.8 (CH3), 14.9 (CH3).
Elemental analysis calcd (%) for C13H20AuClN2O2: C, 33.31; H, 4.30; N, 5.98; found: C, 33.41; H, 4.49; N, 5.94.
Synthesis of [Au(IBioxtBu)Cl] (3b).
Complex 3b was synthesized according to general procedure from IBioxtBu·HCl (50 mg, 0.166 mmol), [Au(DMS)Cl] (49 mg, 0.166 mmol), K2CO3 (69 mg, 0.498 mmol) and acetone (0.5 mL), 2 h. White powder, 63.1 mg (76%).
1H NMR (400 MHz, chloroform-d) δ 4.92–4.76 (m, 4H, O–CH2), 4.20 (dd, J = 6.2, 2.2 Hz, 2H, CH2–CH), 1.18 (s, 18H, CH3).
13C NMR (101 MHz, methylene chloride-d2) δ 151.5, 125.5, 79.1 (O–CH2), 68.0 (CH2–CH), 35.4, 27.5 (CH3).
Elemental analysis calcd (%) for C15H24AuClN2O2: C, 36.27; H, 4.87; found: C, 36.29; H, 4.73.
Synthesis of [Au(IBioxMe4)Cl] (3c).
Complex 3c was synthesized according to general procedure from IBioxMe4·HCl (50 mg, 0.204 mmol), [Au(DMS)Cl] (60.2 mg, 0.204 mmol), K2CO3 (84.7 mg, 0.613 mmol) and acetone (0.5 mL), 1 h. White powder, 63.8 mg (71%).
1H NMR (300 MHz, chloroform-d) δ 4.55 (s, 4H, O–CH2), 1.75 (s, 12H, CH3).
13C NMR (101 MHz, methylene chloride-d2) δ 143.2, 124.5, 88.4 (O–CH2), 61.6, 26.5 (CH3).
Elemental analysis calcd (%) for C11H16AuClN2O2: C, 29.98; H, 3.66; N, 6.36; found: C, 29.83; H, 3.69; N, 6.81.
Synthesis of [Au(IBiox6)Cl] (3d).
Complex 3d was synthesized according to general procedure from IBiox6·HCl (50 mg, 0.154 mmol), [Au(DMS)Cl] (45.4 mg, 0.154 mmol), K2CO3 (63.9 mg, 0.462 mmol) and acetone (0.5 mL), 1 h. White powder, 54.5 mg (68%).
1H NMR (400 MHz, chloroform-d) δ 4.67 (s, 4H, O–CH2), 2.59–2.37 (m, 4H), 1.93 (d, J = 11.6 Hz, 8H), 1.81–1.64 (m, 2H), 1.46–1.08 (m, 6H).
13C NMR (101 MHz, chloroform-d) δ 142.4, 123.6, 84.7 (O–CH2), 35.7 (CH2), 24.1 (CH2), 23.7 (CH2).
Elemental analysis calcd (%) for C17H24AuClN2O2: C, 39.21; H, 4.65; N, 5.38; found: C, 39.37; H, 4.55; N, 5.34.
Conflicts of interest
There are no conflicts to declare.
Acknowledgements
We are grateful to the BOF (starting and senior grants to SPN), as well as the iBOF C3 project for financial support. The Research Foundation – Flanders (FWO) is acknowledged for support. KVH and SB thank the FWO (projects AUGE/11/029 and 1275221N) for funding.
References
- Q. Zhao, G. Meng, S. P. Nolan and M. Szostak, Chem. Rev., 2020, 120, 1981–2048 CrossRef CAS PubMed.
- J.-N. Levy, C. M. Latham, L. Roisin, N. Kandziora, P. D. Fruscia, A. J. P. White, S. Woodward and M. J. Fuchter, Org. Biomol. Chem., 2012, 10, 512–515 RSC.
- S. Díez-González, N. Marion and S. P. Nolan, Chem. Rev., 2009, 109, 3612–3676 CrossRef PubMed.
- G. Altenhoff, R. Goddard, C. W. Lehmann and F. Glorius, J. Am. Chem. Soc., 2004, 126, 15195–15201 CrossRef CAS PubMed.
- S. Würtz, C. Lohre, R. Fröhlich, K. Bergander and F. Glorius, J. Am. Chem. Soc., 2009, 131, 8344–8345 CrossRef PubMed.
-
F. Glorius, Method for Producing Imidazolium Salts, US 2005/0240025A1, 2005.
- F. Glorius, G. Altenhoff, R. Goddard and C. Lehmann, Chem. Commun., 2002, 2704–2705 RSC.
- J. Huang and S. P. Nolan, J. Am. Chem. Soc., 1999, 121, 9889–9890 CrossRef CAS.
- G. Altenhoff, S. Würtz and F. Glorius, Tetrahedron Lett., 2006, 47, 2925–2928 CrossRef CAS.
- R. Melot, M. Zuccarello, D. Cavalli, N. Niggli, M. Devereux, T. Bürgi and O. Baudoin, Angew. Chem., Int. Ed., 2021, 60, 7245–7250 CrossRef CAS PubMed.
- A. B. Chaplin, Organometallics, 2014, 33, 3069–3077 CrossRef CAS.
- B. Ramasamy, A. P. Prakasham, M. K. Gangwar and P. Ghosh, ChemistrySelect, 2019, 4, 8526–8533 CrossRef CAS.
- J. Bexrud and M. Lautens, Org. Lett., 2010, 12, 3160–3163 CrossRef CAS PubMed.
- C. Lohre, C. Nimphius, M. Steinmetz, S. Würtz, R. Fröhlich, C. G. Daniliuc, S. Grimme and F. Glorius, Tetrahedron, 2012, 68, 7636–7644 CrossRef CAS.
- G. Altenhoff, R. Goddard, C. W. Lehmann and F. Glorius, Angew. Chem., Int. Ed., 2003, 42, 3690–3693 CrossRef CAS PubMed.
- R. Melot, M. Zuccarello, D. Cavalli, N. Niggli, M. Devereux, T. Bürgi and O. Baudoin, Angew. Chem., Int. Ed., 2021, 60, 7245–7250 CrossRef CAS PubMed.
- A. Collado, A. Gómez-Suárez, A. R. Martin, A. M. Z. Slawin and S. P. Nolan, Chem. Commun., 2013, 49, 5541–5543 RSC.
- R. Visbal, A. Laguna and M. Concepción Gimeno, Chem. Commun., 2013, 49, 5642–5644 RSC.
- E. A. Martynova, N. V. Tzouras, G. Pisanò, C. S. J. Cazin and S. P. Nolan, Chem. Commun., 2021, 57, 3836–3856 RSC.
- T. Scattolin and S. P. Nolan, Trends Chem., 2020, 2, 721–736 CrossRef CAS.
- E. A. Martynova, T. Scattolin, E. Cavarzerani, M. Peng, K. V. Hecke, F. Rizzolio and S. P. Nolan, Dalton Trans., 2022, 51, 3462–3471 RSC.
- S. G. Guillet, G. Pisanò, S. Chakrabortty, B. H. Müller, J. G. de Vries, P. C. J. Kamer, C. S. J. Cazin and S. P. Nolan, Eur. J. Inorg. Chem., 2021, 2021, 3506–3511 CrossRef CAS.
- V. A. Voloshkin, N. V. Tzouras and S. P. Nolan, Dalton Trans., 2021, 50, 12058–12068 RSC.
- N. V. Tzouras, E. A. Martynova, X. Ma, T. Scattolin, B. Hupp, H. Busen, M. Saab, Z. Zhang, L. Falivene, G. Pisanò, K. Van Hecke, L. Cavallo, C. S. J. Cazin, A. Steffen and S. P. Nolan, Chem. – Eur. J., 2021, 27, 11904–11911 CrossRef CAS PubMed.
- X. Ma, S. G. Guillet, Y. Liu, C. S. J. Cazin and S. P. Nolan, Dalton Trans., 2021, 50, 13012–13019 RSC.
- P. de Frémont, N. M. Scott, E. D. Stevens and S. P. Nolan, Organometallics, 2005, 24, 2411–2418 CrossRef.
- A. Poater, B. Cosenza, A. Correa, S. Giudice, F. Ragone, V. Scarano and L. Cavallo, Eur. J. Inorg. Chem., 2009, 2009, 1759–1766 CrossRef.
- A. C. Hillier, W. J. Sommer, B. S. Yong, J. L. Petersen, L. Cavallo and S. P. Nolan, Organometallics, 2003, 22, 4322–4326 CrossRef CAS.
- H. Clavier and S. P. Nolan, Chem. Commun., 2010, 46, 841–861 RSC.
- T. Dröge and F. Glorius, Angew. Chem., Int. Ed., 2010, 49, 6940–6952 CrossRef.
- L. Falivene, Z. Cao, A. Petta, L. Serra, A. Poater, R. Oliva, V. Scarano and L. Cavallo, Nat. Chem., 2019, 11, 872–879 CrossRef CAS PubMed.
- M. R. Fructos, T. R. Belderrain, P. de Frémont, N. M. Scott, S. P. Nolan, M. M. Díaz-Requejo and P. J. Pérez, Angew. Chem., Int. Ed., 2005, 44, 5284–5288 CrossRef CAS PubMed.
- Y. Tang, I. Benaissa, M. Huynh, L. Vendier, N. Lugan, S. Bastin, P. Belmont, V. César and V. Michelet, Angew. Chem., Int. Ed., 2019, 58, 7977–7981 CrossRef CAS PubMed.
- C. Schwarz, J. Handelmann, D. M. Baier, A. Ouissa and V. H. Gessner, Catal. Sci. Technol., 2019, 9, 6808–6815 RSC.
- V. Lavallo, J. H. Wright II, F. S. Tham and S. Quinlivan, Angew. Chem., Int. Ed., 2013, 52, 3172–3176 CrossRef CAS PubMed.
- J. Handelmann, C. Naga Babu, H. Steinert, C. Schwarz, T. Scherpf, A. Kroll and V. H. Gessner, Chem. Sci., 2021, 12, 4329–4337 RSC.
|
This journal is © The Royal Society of Chemistry 2023 |