Decelerated degradation of a sulfonylurea herbicide in four fungicide-treated soils†
Received
4th October 2021
, Accepted 16th January 2022
First published on 20th January 2022
Abstract
Soil microbial communities which are essential for soil ecosystems can be disturbed by a manifold of environmental variables, including pesticides. Among others, fungicides are frequently applied as effective crop protection chemicals in modern agriculture. Due to their wide killing and inhibitory capabilities, broad-spectrum fungicides play a dominant role in alteration of the soil microflora. Alteration of the soil microbial composition due to the presence of e.g., fungicides affects the environmental fate of co-existing chemicals. Ergo, the degradation rate of such compounds can be inhibited and their dissipation half-life in the environment can be prolonged. Nonetheless, current international guidelines for testing of chemicals are designed for the examination and risk assessment of a single chemical compound in their respective test system. While mixtures have been thoroughly studied concerning their agronomic efficacy, possible cumulative or synergistic effects and interactions between individual mixed compounds in the environment, and indirect side-effects such as alteration of microbial communities in soil, water and sediment were only rarely investigated. Therefore, we investigated the potential environmental impact of tank mixtures containing broad spectrum fungicides tebuconazole and prothioconazole on the degradation of the herbicide iodosulfuron-methyl-sodium in US American and German soils under controlled laboratory conditions. We showed that the biodegradation of the herbicide iodosulfuron-methyl-sodium in four different soils is decelerated in the presence of the fungicides tebuconazole and prothioconazole. This effect was observed from the inhibited biotic transformation of the herbicide into its main soil metabolite. Except for one soil, the adverse fungicidal effect was transient and mitigated towards the end of the respective incubation periods. Half-lives (DT50) and DT90 values of the herbicide in the four fungicide-treated soils increased 1.5 to 2.9-fold and 1.3 to 2.2-fold, respectively when compared to those in the untreated control soils.
Environmental significance
Concurrent application of pesticides leads to pollution of the soil environment and significant alteration of the abundance and diversity of microbial communities in soil. This can result in retarded biodegradation of individual parent compounds. Therefore, the route and rate of degradation of the herbicide iodosulfuron-methyl-sodium in four different soils treated with fungicides tebuconazole and prothioconazole under controlled laboratory conditions were investigated. Environmentally realistic doses were applied. The study results reveal that the presence of the fungicides did adversely impact the biodegradation of the herbicide in all four soils. These findings demand for more environmentally realistic laboratory research and risk assessment on pesticide mixtures.
|
1. Introduction
Over the past 20 years, the human population has increased by about 1 billion per decade, reaching nearly 8 billion in 2021.1 Apart from increasing population, a diet that comprises more meat and dairy products emphasizes the importance of agricultural production. Livestock is largely nourished on grain, whereas agricultural land to grow crops is critically endangered by increasing urbanization, erosion, pollution, and climate change. This raises the necessity to produce more nutritious and safe foods on less arable land with less resources, such as water and fertilizers. Especially the enhanced use of fertilizers stimulates higher levels of pathogenic fungi infestation on crops, whereas the extensive use of pesticides allows for even higher input of fertilizers to boost yields. Consequently, fungicides have been playing a major role in pest control.2 Fungicides capture more than one third of the worldwide pesticide market share with Europe as the capital market and their major use is on grains, cereals, fruits, and vegetables. As a side-effect, crops can develop resistance to frequently applied fungicides. Hence, despite fierce exploitation of fungicides, fungal pathogens cause up to 24% of yield losses in field crops.3 Multiple applications per season to target crops effect incremental fungicide use, which is anymore reason for concern due to increasing pesticide residues in commodities, such as wine products and drinking water.4 Moreover, agrochemicals can reach the soil environment and may negatively interact with soil microflora and disturb its biological activities.5
The prevalent group of antifungal chemicals applied on inter alia cereals in various European countries is obtained from conazole fungicides, comprising triazoles and imidazoles, whose residues are regularly detected in arable soils.6 One of these fungicides is tebuconazole (Fig. 1), a broad-spectrum systemic triazole fungicide for controlling soil-borne and foliar fungal pests.7,8 Tebuconazole is persistent in soil and is widely used on turf, cereals and various fruit crops, such as grapes and bananas.9,10 In Europe and the USA, tebuconazole is distributed individually and together with the broad-spectrum systemic triazole fungicide prothioconazole (Fig. 1) in the mixed formulation Prosaro.11 Prothioconazole controls proliferation of fungi on barley, wheat, and peanuts. Both fungicides, tebuconazole and prothioconazole, disturb growth of various plant pathogenic fungi, such as ascomycetes, basidiomycetes, and deuteromycetes. The mode of action is based on obstruction of ergosterol biosynthesis through inhibition of sterol 14α‐demethylase cytochrome P450 (CYP51), which is responsible for the morphology and functionality of the fungal cell membrane.12–16 The use of the fungicide formulation Prosaro is recommended in combination with iodosulfuron-methyl-sodium (Fig. 1),11 a sulfonylurea post-emergence herbicide, which controls weeds amongst corn17 and cereals, such as winter wheat and barley.18 Iodosulfuron-methyl-sodium interferes with acetolactate synthase (ALS), which regulates the synthesis of amino acids that are necessary for plant development.19
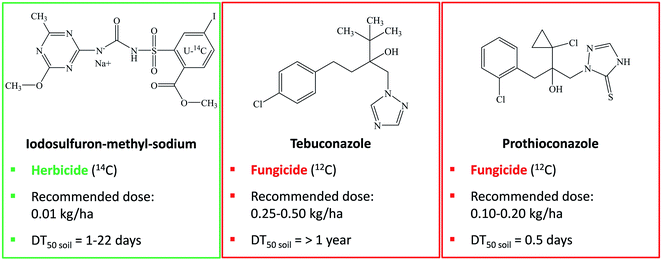 |
| Fig. 1 Structural formulae of the herbicide iodosulfuron-methyl-sodium and the fungicides tebuconazole and prothioconazole, with recommended field rates and half-lives in soil (DT50).8,10,14–19 | |
In the production of agricultural crops, the successive application of distinct pesticides, or mixtures of them, is a frequent practice. Tank mixtures including two or more individual chemical compounds are preferred by farmers, as they save fuel and labor hours, decrease soil compaction due to less agricultural traffic and lead to a larger range and efficacy in pest control, when compared to the applications of single chemical compounds. Simultaneous application of dissimilar pesticides, such as iodosulfuron-methyl-sodium, tebuconazole and prothioconazole, results in pollution of the soil environment by a multitude of different plant protection agents and their residues,20 which can have numerous effects on soil life. The impact of individual and combined chemicals on soil microbial biomass, enzymatic reactions and physicochemical parameters has widely been studied. Yet, literature on the influence of chemical combinations on the degradation of parent compounds is scarce.21 Consequently, we have studied the route and rate of degradation of the radiolabeled herbicide iodosulfuron-methyl-sodium in four different soils treated with the fungicide combination tebuconazole and prothioconazole under controlled conditions. This research elucidates potential effects of both fungicides and their residues on the degradation potential of soils.
2. Materials and methods
2.1 Soils
Four soils differing in geographies and characteristics were selected to assess the aerobic degradation rate of the radiolabeled herbicide iodosulfuron-methyl-sodium in soil, in the presence of fungicides tebuconazole and prothioconazole. The characteristics of the individual soils are presented in Table 1.
Table 1 Soil characteristics
Soil Name |
Am Fischteich |
Bellevue |
Speyer 2.1 |
Pavillion |
Geography |
Crimmitschau Germany |
Iowa USA |
Speyer Germany |
Wyoming USA |
Texture (USDA) |
Silt loam (1) |
Silt loam (2) |
Loamy sand |
Sandy loam |
Particle size analyses
|
Clay (<0.002 mm) [%] |
27.0 |
23.0 |
4.1 |
13.0 |
Silt (0.002–0.05 mm) [%] |
57.0 |
63.0 |
9.3 |
11.0 |
Sand (>0.05 mm) [%] |
16.0 |
14.0 |
86.6 |
76.0 |
![[thin space (1/6-em)]](https://www.rsc.org/images/entities/char_2009.gif) |
Water holding capacity
|
|
|
|
|
At pF 2 [g water per 100 g soil] |
27.5 |
37.0 |
7.3 |
13.6 |
pH (0.01 M CaCl2) |
5.6 |
6.7 |
4.7 |
8.1 |
Organic carbon [%] |
2.1 |
2.6 |
0.6 |
0.9 |
CEC [mmol/100 g soil] |
13.2 |
13.7 |
3.7 |
17.6 |
Biomass [mg Corg per 100 g soil] – SIR |
83.8 |
68.0 |
11.6 |
21.1 |
The soils were stored at about 4 °C in the dark upon usage, followed by biomass determination with the substrate induced respiration method (SIR).22 Soil samples were prepared by filling 100 g of dry-weight soil into all-glass flasks, with a volume of approximately 1 liter and an inner diameter of about 10.6 cm. The soil moisture content was adjusted to the respective pF 2 (gravimetrically by adding purified water, if needed) prior to acclimatization in the dark for one week, in an environmental chamber at a controlled temperature of 20 ± 2 °C and under constant aeration with moist air.
2.2 Chemicals
The herbicide iodosulfuron-methyl-sodium (Izotop, Budapest, Hungary) was [14C]-uniform-labeled in the phenyl moiety of the molecule with a specific activity of 4.359 MBq per mg. The radiochemical purity was determined to be above 95% by high-performance liquid chromatography (HPLC-UV/RAM) before the first and after the final applications. The unlabeled fungicides tebuconazole and prothioconazole (Sigma Aldrich, Buchs, Switzerland) had a chemical purity of 99.3% and 99.9%, respectively.
2.3 Application scenario
Each soil sample (100 g) was individually treated with 13 µg of [14C]-iodosulfuron-methyl-sodium. For each soil type, one set of triplicate individual samples per interval was treated with the radiolabeled herbicide only (controls) and one set of triplicate individual samples per interval was subjected to a fungicidal application scenario simulating agricultural field practice of combined treatment. This fungicidal application scenario represented three sequential treatments, each with unlabeled fungicides tebuconazole and prothioconazole, dissolved in acetonitrile/acetone (1/1; v/v), per sample: the first fungicidal treatment was performed at the end of the one-week acclimation period, respectively 14 days prior to the second treatment on the same day as the herbicide treatment (day 0) and the final (third) treatment 5 days after application of radiolabeled iodosulfuron-methyl-sodium. The fungicides were dosed in accordance with field rates as recommended by regulatory US EPA and EU EFSA, or by FAO (Food and Agriculture Organization), WHO. In each treatment, tebuconazole and prothioconazole were applied at 0.500 kg ha−18 and 0.200 kg ha−1,14,16 corresponding to 67 µg and 27 µg per soil sample (100 g), respectively. To ensure higher analytical reliability, the herbicide iodosulfuron-methyl-sodium was applied at a ten-fold exaggerated target rate of 0.100 kg ha−1 (ref. 18) in the controls and fungicidal application scenario, corresponding to 13 µg per 100 g of soil, when assuming an even distribution of the chemical in the top 5 cm soil layer, and a soil bulk density of 1.5 g cm−3. This dose rate did not affect the degradation velocity of iodosulfuron-methyl-sodium in the tested soil types as shown in preliminary tests using the recommended dose (data not shown). At each fungicide treatment interval, the soils of the control samples received an equivalent amount of organic solvent (0.3 mL of acetonitrile/acetone (1/1; v/v)). After dosing the soil surface, the treated soils were mixed thoroughly allowing the organic solvent to evaporate and the soil moisture content was monitored and adjusted to pF 2, if needed. The total amount of organic solvent added to each soil sample throughout the complete application procedure was 1.0 mL per 100 g of dry-weight soil, corresponding to 1.0% (v/w) and in accordance with regulatory guidelines.23,24
2.4 Incubation
The soil samples were incubated for up to 77 days, depending on the soil type, in an environmental chamber at a temperature of 20 ± 2 °C in the dark. Each flask was individually equipped with an inlet and outlet for gases and was maintained under aerobic conditions by constant aeration with moist air. The exhaust air was passed through an individual trapping system for each sample equipped with two absorption traps containing ethylene glycol and 2 N NaOH (in this sequence) allowing organic volatiles and 14CO2 to be trapped, respectively.
2.5 Sample processing
Three individual samples per soil and condition were taken for exhaustive extraction and analysis at eight different time points until 30 to 77 days of incubation, depending on the degradation velocity of [14C]-iodosulfuron-methyl-sodium in the various soils. Samples were extracted four times with acetonitrile/water (4/1; v/v), until less than 5% of the applied radioactivity (AR) could be extracted in a single extraction step. If more than 10% AR remained unextractable after these polar extractions, the soils were subsequently extracted once each with acetone, ethyl acetate and hexane. If still more than 10% AR remained unextractable after the polar and less polar extractions, Soxhlet extraction with acetonitrile/water (4/1; v/v) was performed for 4 hours. The recovery of each extraction step was determined by duplicate liquid scintillation counting (LSC) measurements. For each sample, all four initial acetonitrile/water (4/1; v/v) soil extracts were combined, the resulting pool was measured by LSC for recovery, and subsequently an aliquot was concentrated under a gentle stream of nitrogen at about 35 °C. Aliquots of the Soxhlet extracts recovering more than 5% AR were also concentrated, initially by use of a rotary evaporator, followed by concentration under a gentle stream of nitrogen, both at about 35 °C, and LSC measurements were performed. Procedural recoveries consistently were > 90% AR. Additionally, radioactivity in the absorption traps containing ethylene glycol or 2 N NaOH was measured by LSC after recording the liquid volumes.
2.6 Quantification of [14C]-iodosulfuron-methyl-sodium and transformation products
The concentrates (final constitution: acetonitrile/water (1/10; v/v)) were subjected to HPLC-UV/RAM for determination of iodosulfuron-methyl-sodium and potential degradation products. For selected samples, the chromatography results obtained by HPLC were confirmed by high-performance thin-layer chromatography (HPTLC) analysis.
2.7. Data evaluation and statistical analysis
Collected data were processed using Microsoft Excel® (Microsoft® Office 365, USA), GraphPad Prism (version 8.0.2, GraphPad Software, USA) and R (version 3.0.0, USA). Outliers were identified by Grubbs's test (α = 0.05). Raw data were tested for normal distribution with the Shapiro–Wilk test (α = 0.05). The Holm-Sidak25 method (α = 0.05) was performed on the datasets for iodosulfuron-methyl-sodium recovered from the four soils at each sampling interval, in order to determine the significance of the difference in abundance of both substances in the two different test systems (herbicide-only and herbicide & fungicides). The datasets for iodosulfuron-methyl-sodium were further subjected to degradation kinetics evaluation using a step-wise approach according to the FOCUS Guidance26 on estimating persistence and degradation kinetics from environmental fate studies. Evaluations were performed by using the computer assisted kinetic evaluation (CAKE) software tool.27 Three different kinetic models, namely single first-order (SFO), first-order multi compartment (FOMC) and double first-order in parallel (DFOP) were fitted to the degradation data. Endpoints could only be found by an iterative procedure, which is implemented in the CAKE software27 and used for the calculations.
3. Results
3.1 Distribution of radioactivity
For the samples treated with the fungicides tebuconazole and prothioconazole, total mass balances were 103.3 ± 1.8% AR in silt loam 1 (Am Fischteich), 103.2 ± 1.5% AR in silt loam 2 (Bellevue), 103.5 ± 2.6% AR in loamy sand (Speyer 2.1) and 100.9 ± 2.7% AR in sandy loam (Pavillion) soils. The corresponding values for the herbicide-only soils (controls) were 103.1 ± 2.0%, 103.0 ± 1.4%, 103.3 ± 2.2% and 101.2 ± 2.4% AR. For the 192 individual soil samples, total mass balances ranged from 96.1% to 107.8% AR and were generally comparable (per interval) for the samples dosed with the fungicides tebuconazole and prothioconazole and those without fungicidal treatment. Mineralization was relatively low in both silt loam (Am Fischteich and Bellevue) and the loamy sand (Speyer 2.1) soils, with radioactivity trapped in the 2 N NaOH absorption bottles peaking at mean amounts of 3.2%, 2.7% and 0.9% AR, respectively, whereas in the sandy loam soil (Pavillion), an increased mineralization of up to 8.6% AR was observed towards the end of the 62 day incubation period. Radioactivity recovered from the ethylene glycol traps, representing volatile organic compounds, was negligible and remained < 0.1% AR for all samples throughout incubation. Non-extractable residues increased throughout incubation, reaching means of 15.9%, 13.4%, 14.1% and 9.5% AR in the fungicide-treated soils, and 16.9%, 15.5%, 15.3% and 9.3% AR in the herbicide-only silt loam 1 (Am Fischteich), silt loam 2 (Bellevue), loamy sand (Speyer 2.1) and sandy loam (Pavillion) soils, respectively. The mass balance results are shown in Fig. 2.
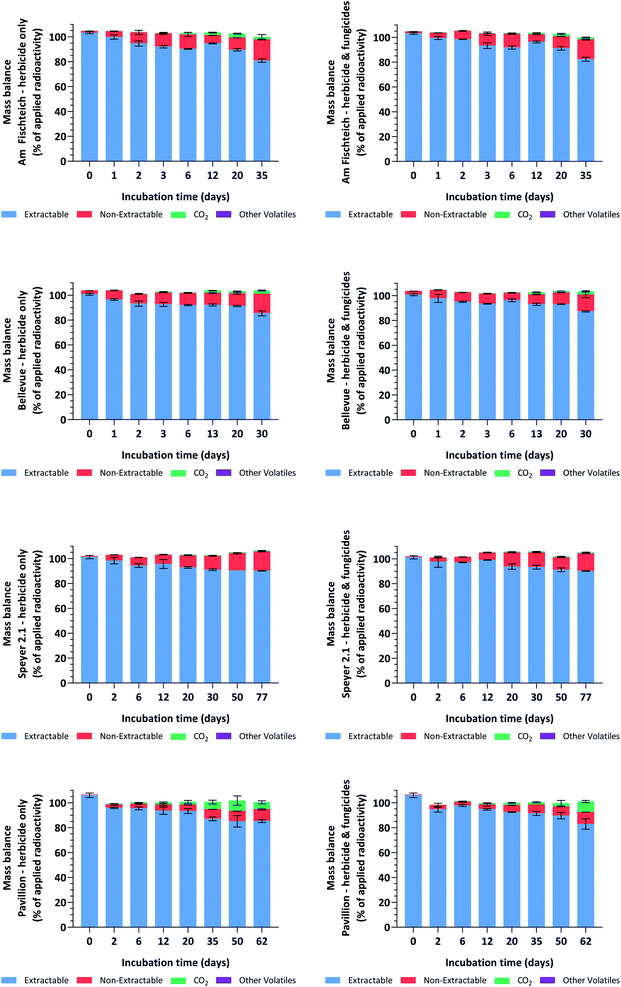 |
| Fig. 2 Mass balances of [14C]-iodosulfuron-methyl-sodium in soil samples treated with iodosulfuron-methyl-sodium only (left), or with tebuconazole and prothioconazole (right) 14 days prior to (day -14; dose 1), then on the same day as the herbicide treatment (day 0; dose 2), and again 5 days after application of radiolabeled iodosulfuron-methyl-sodium (day 5; dose 3). Mean values and standard deviations were derived from triplicates (n = 3) and are expressed as the percentage of applied radioactivity (%AR). | |
The limit of detection (LOD) and limit of quantification (LOQ) values for LSC and HPLC were estimated to be less than 1% AR. Details are provided in Table 2.
Table 2 Estimated LOD and LOQ values of radiolabeled iodosulfuron-methyl-sodium in soil
Method |
LOD (% AR) |
LOQ (% AR) |
LSC
|
Acetonitrile/water (4/1; v/v) extracts |
0.10 |
0.15 |
Soxhlet extracts |
0.21 |
0.32 |
Non-extractables |
0.24 |
0.36 |
14CO2 and other volatiles |
0.05 |
0.08 |
HPLC
|
0.48 |
0.96 |
3.2 Route of degradation
In all four soils, degradation of iodosulfuron-methyl-sodium proceeded via biotic reductive elimination of iodine from the phenyl ring28 to metsulfuron-methyl as the main degradation product, or to AE F145740 by hydrolysis of the phenyl based carboxylate ester. Metsulfuron-methyl and AE F145740 were thereafter metabolized further, either by hydrolysis of the phenyl based carboxylate ester, or via biotic elimination of iodine from the phenyl ring, respectively to AE 0014966 (Fig. 3) along with minor degradation products, and ultimately mineralized to carbon dioxide.29,30
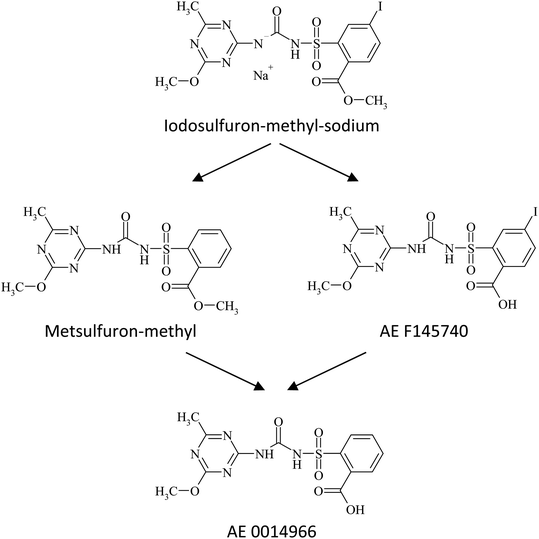 |
| Fig. 3 Formation pathway of major transformation products30 of iodosulfuron-methyl-sodium in silt loam 1 (Am Fischteich), silt loam 2 (Bellevue), sandy loam (Speyer 2.1) and loamy sand (Pavillion) soils incubated at 20 ± 2 °C in the dark under aerobic conditions. | |
For detailed amounts of iodosulfuron-methyl-sodium and its metabolites metsulfuron-methyl, AE F145740 and AE 0014966 recovered from the soils at each sampling interval, see ESI Table S1.†
3.3 Degradation kinetics
Degradation of iodosulfuron-methyl-sodium occurred fastest in herbicide-only silt loam soils (Am Fischteich and Bellevue), followed by the sandy loam soil (Pavillion), with half-lives of 2.2, 3.0 and 6.9 days, respectively (Fig. 4). In the loamy sand soil (Speyer 2.1), a retarded degradation velocity (DT50 = 29 days) of the herbicide was observed and contrary to the other three soils, DT90 was not reached during the 77 day incubation period. Datasets were evaluated and plotted by non-linear regression (one-phase decay in combination with least squares fit). Since iodosulfuron-methyl-sodium showed rapid degradation in silt loam 1 (Am Fischteich) and silt loam 2 (Bellevue) soils, the work-up time of these samples was considered and consequently, for kinetic evaluation, the test item purity (>95%) pattern was assessed as time 0 data, whereas the chromatography day 0 results were set to 0.10 days.
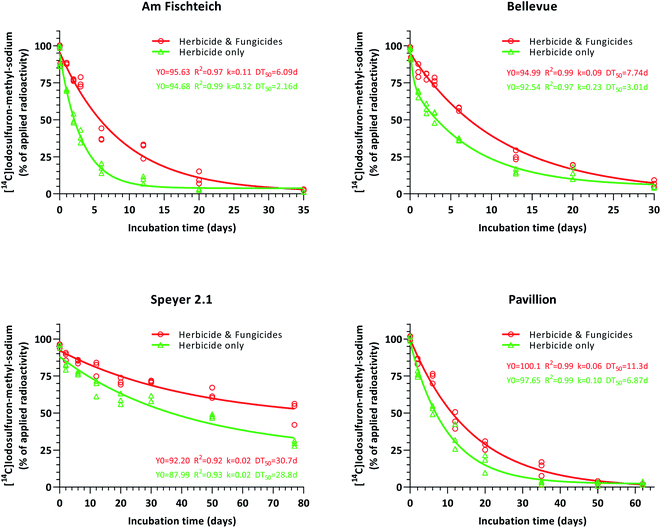 |
| Fig. 4 Recovery (in %AR) of [14C]-iodosulfuron-methyl-sodium from soil samples (n = 3). Triangular data in green depict soil samples treated with iodosulfuron-methyl-sodium only (controls), circular data in red describe soil samples treated with tebuconazole and prothioconazole 14 days prior to (day -14; dose 1), then on the same day as the herbicide treatment (day 0; dose 2), and again 5 days after application of radiolabeled iodosulfuron-methyl-sodium (day 5; dose 3). | |
In comparison to the untreated control soils (green datasets), degradation of iodosulfuron-methyl-sodium was impeded in all four soils treated with the fungicides tebuconazole and prothioconazole (red datasets). Exclusive of the loamy sand soil Speyer 2.1, where the contrast in the degradation velocity of the herbicide between treated and untreated soil samples intensified throughout the 77 day incubation period, this distance was offset towards the end of incubation in the remaining three soils (silt loam 2 (Am Fischteich), silt loam 2 (Bellevue) and sandy loam (Pavillion)).
All datasets (Fig. 4) were further subjected to degradation kinetics evaluation as previously described in Section 2.7. For untreated silt loam 1 soil (Am Fischteich; herbicide-only), the FOMC model resulted in improved visual and statistical results, when compared to the SFO model, and was chosen as the best-fit model, whereas the DFOP model returned invalid statistical output. Use of the SFO model on the silt loam 2 (Bellevue) soil untreated control sample (herbicide-only) dataset generated a poor visual fit, whereas both the FOMC and DFOP models produced good visual fits. The DFOP model returned optimal statistical output, hence was selected as the best-fit model. For the remaining datasets, the SFO model prompted superior visual and/or statistical output and was accepted as the best-fit model respectively. The respective persistence endpoints acquired by best fit kinetics models are given in Table 3.
Table 3 Persistence endpoints using Tessella CAKE (version 3.4) for iodosulfuron-methyl-sodium in soils treated with and in the absence of fungicides tebuconazole and prothioconazole. For each soil, the best-fit model in accordance with the FOCUS guidelines, along with half-lives (DT50) and dissipation times after 90 days (DT90), Chi2 (χ2-error in %) and r2 (observed vs. predicted data) are given
Soil |
Treatment |
Best-fit kinetic model |
DT50 |
DT90 |
χ
2-error |
r
2
|
Silt loam 1 |
Herbicide only |
FOMC |
2.2 |
10.2 |
5.1 |
0.992 |
Am Fischteich |
Herbicide & fungicides |
SFO |
6.3 |
20.9 |
7.3 |
0.972 |
Silt loam 2 |
Herbicide only |
DFOP |
3.5 |
19.5 |
4.0 |
0.992 |
Bellevue |
Herbicide & fungicides |
SFO |
7.9 |
26.3 |
3.9 |
0.988 |
Loamy sand |
Herbicide only |
SFO |
40.3 |
134 |
9.9 |
0.927 |
Speyer 2.1 |
Herbicide & fungicides |
|
89.2 |
296 |
3.2 |
0.904 |
Sandy loam |
Herbicide only |
SFO |
7.3 |
24.3 |
5.8 |
0.986 |
Pavillion |
Herbicide & fungicides |
|
11.1 |
36.9 |
4.4 |
0.990 |
3.4 Statistical data analysis
With the purpose to assess statistical significance of the observed contrasting degradation velocities of iodosulfuron-methyl-sodium in the fungicide-treated and control test systems of each of the four soils, a statistical multiple t-test using the Holm-Sidak25 method (alpha = 0.05) was performed on the datasets for iodosulfuron-methyl-sodium (Table 4). These multiple t-test results confirmed the visual results as deduced from Fig. 4. Significance contrast in amounts of iodosulfuron-methyl-sodium recovered from the fungicide-treated and control soils was statistically confirmed for all but the first interval in all four soils throughout incubation, and except for soil Speyer 2.1, redeemed towards the end of incubation.
Table 4 Significance results by the multiple t-test using the Holm-Sidak method (n = 3)
Interval (days) |
Significant? |
P value |
Mean of herbicide only |
Mean of herbicide & fungicides |
Difference |
Standard error of difference |
t ratio |
Degrees of freedom |
Adjusted P value |
Silt loam 1 – Am Fischteich
|
0 |
No |
>0.999999 |
86.9 |
86.9 |
0.0 |
2.281 |
0.000 |
32 |
>0.999999 |
1 |
Yes |
<0.000001 |
70.2 |
88.1 |
−17.9 |
2.281 |
7.846 |
32 |
<0.000001 |
2 |
Yes |
<0.000001 |
50.4 |
76.8 |
−26.4 |
2.281 |
11.570 |
32 |
<0.000001 |
3 |
Yes |
<0.000001 |
38.6 |
75.1 |
−36.5 |
2.281 |
16.000 |
32 |
<0.000001 |
6 |
Yes |
<0.000001 |
17.4 |
39.2 |
−21.8 |
2.281 |
9.555 |
32 |
<0.000001 |
12 |
Yes |
<0.000001 |
9.5 |
29.9 |
−20.4 |
2.281 |
8.942 |
32 |
<0.000001 |
20 |
Yes |
0.002460 |
3.0 |
10.5 |
−7.5 |
2.281 |
3.287 |
32 |
0.007362 |
35 |
No |
0.602527 |
1.4 |
2.6 |
−1.2 |
2.281 |
0.526 |
32 |
0.842015 |
![[thin space (1/6-em)]](https://www.rsc.org/images/entities/char_2009.gif) |
Silt loam 2 – Bellevue
|
0 |
No |
>0.999999 |
91.6 |
91.6 |
0.0 |
2.088 |
0.000 |
32 |
>0.999999 |
1 |
Yes |
<0.000001 |
67.7 |
83.0 |
−15.3 |
2.088 |
7.328 |
32 |
<0.000001 |
2 |
Yes |
<0.000001 |
57.5 |
79.8 |
−22.3 |
2.088 |
10.680 |
32 |
<0.000001 |
3 |
Yes |
<0.000001 |
52.6 |
76.1 |
−23.5 |
2.088 |
11.260 |
32 |
<0.000001 |
6 |
Yes |
<0.000001 |
36.7 |
57.4 |
−20.7 |
2.088 |
9.915 |
32 |
<0.000001 |
13 |
Yes |
0.000014 |
15.1 |
25.8 |
−10.7 |
2.088 |
5.125 |
32 |
0.000055 |
20 |
Yes |
0.012881 |
14.0 |
19.5 |
−5.5 |
2.088 |
2.634 |
32 |
0.038146 |
30 |
No |
0.222044 |
4.3 |
6.9 |
−2.6 |
2.088 |
1.245 |
32 |
0.394785 |
![[thin space (1/6-em)]](https://www.rsc.org/images/entities/char_2009.gif) |
Loamy sand – Speyer 2.1
|
0 |
No |
>0.999999 |
95.4 |
95.4 |
0.0 |
2.715 |
0.000 |
32 |
>0.999999 |
2 |
Yes |
0.011281 |
81.4 |
88.7 |
−7.3 |
2.715 |
2.689 |
32 |
0.022435 |
6 |
Yes |
0.006524 |
77.0 |
84.9 |
−7.9 |
2.715 |
2.910 |
32 |
0.019445 |
12 |
Yes |
0.000050 |
67.7 |
80.4 |
−12.7 |
2.715 |
4.678 |
32 |
0.000303 |
20 |
Yes |
0.000118 |
59.3 |
71.2 |
−11.9 |
2.715 |
4.384 |
32 |
0.000530 |
30 |
Yes |
0.000106 |
59.2 |
71.2 |
−12.0 |
2.715 |
4.421 |
32 |
0.000530 |
50 |
Yes |
0.000005 |
48.0 |
62.8 |
−14.8 |
2.715 |
5.452 |
32 |
0.000037 |
77 |
Yes |
<0.000001 |
29.5 |
51.0 |
−21.5 |
2.715 |
7.920 |
32 |
<0.000001 |
![[thin space (1/6-em)]](https://www.rsc.org/images/entities/char_2009.gif) |
Sandy loam – Pavillion
|
0 |
No |
>0.999999 |
100.8 |
100.8 |
0.0 |
2.973 |
0.000 |
32 |
>0.999999 |
2 |
Yes |
0.004846 |
76.4 |
85.4 |
−9.0 |
2.973 |
3.027 |
32 |
0.019243 |
6 |
Yes |
<0.000001 |
52.4 |
73.8 |
−21.4 |
2.973 |
7.198 |
32 |
<0.000001 |
12 |
Yes |
0.000611 |
33.4 |
44.7 |
−11.3 |
2.973 |
3.801 |
32 |
0.003659 |
20 |
Yes |
0.000382 |
16.5 |
28.3 |
−11.8 |
2.973 |
3.969 |
32 |
0.002672 |
35 |
Yes |
0.002010 |
3.0 |
13.0 |
−10.0 |
2.973 |
3.364 |
32 |
0.010007 |
50 |
No |
0.867503 |
2.4 |
2.9 |
−0.5 |
2.973 |
0.168 |
32 |
0.993706 |
62 |
No |
0.815362 |
2.8 |
2.1 |
0.7 |
2.973 |
0.235 |
32 |
0.993706 |
Overall, in soils that received fungicidal treatment with tebuconazole and prothioconazole, degradation of iodosulfuron-methyl-sodium was significantly slower when compared to the soils lacking fungicidal treatment (DT50 1.5 to 2.9-fold, and DT90 1.3 to 2.2-fold).
4. Discussion
Soils are crucial in the environment through impelling biological processes and acting as the buffer zone to preserve groundwater and plants against contaminants.31 Through screening more than three hundred European agricultural topsoils in the application of over seventy distinct pesticide residues by Silva et al.,32 the conclusion was drawn that more than 80% of the soils were polluted and over 50% of the soils contained 166 different mixtures. Tebuconazole, representing one of a few dominant contaminants, was found at elevated concentrations, frequently higher than its predicted environmental concentrations. Hvězdová et al.33 detected tebuconazole in 27 out of 75 agricultural Central European soils, at concentrations regularly surpassing 0.01 mg kg−1. Similarly, Riedo et al.20 screened 100 agricultural fields with contrasting farming systems (conventional with and without tillage, organic) in Switzerland with 46 pesticides. They showed that pesticides, such as tebuconazole, and transformation products were present in all sites, however with higher abundance in conventional than in organic fields. Additionally, Riedo et al.20 found that microbial biomass and the presence of arbuscular mycorrhizal fungi, representing soil-borne fungi able to enhance plant nutrient uptake and resistance to several abiotic stressors,34 were increasingly harmed by elevated number and concentrations of pesticides in soils.
We could show that in soils that received fungicidal treatment through tebuconazole and prothioconazole according to good agricultural practice, the aerobic degradation of iodosulfuron-methyl-sodium was significantly slower when compared to that of the soils lacking fungicidal treatment (DT50 1.5 to 2.9-fold, and DT90 1.3 to 2.2-fold). Except for the sandy soil Speyer 2.1, where the discrepancy in the degradation velocity of the herbicide between treated and untreated soil samples intensified throughout 77 days of incubation, the negative fungicidal effect mitigated until the end of the respective incubation periods in the remaining silt loam soils (Am Fischteich and Bellevue), and in the sandy loam soil (Pavillion) (Fig. 4 and Table 4). This fading adverse effect might be related to the recovery of microbial biomass in respective soils as demonstrated by Cycón et al.35 They documented that adverse effects of tebuconazole on soil fungi were transient at concentrations of 2.7, 13.5 and 270 mg kg−1, which are considerably higher than the concentration (3 × 0.67 mg kg−1, or a total of 2.0 mg kg−1) applied in our experiment, although the fungicide is persistent in soil (Fig. 1). The fungal population in tebuconazole treated soil decreased by 1 day and recovered over time by 14 and 28 days after treatment. Cycón et al. showed that the level of decrease on days 1 and 14 was correlated with the fungicidal concentration. Soil microorganisms could survive in soils through utilization of tebuconazole and/or its emulsion formulation as the substrate, which resulted in only short-term hostile impact of the fungicide, with successive increase of nitrification, ammonification, and microbial population, mainly bacteria. The latter could be related to extended supply of nutrients and sources discharged from deceased fungal hyphae. Since fungi, when compared to bacteria, offer a penurious source of nitrogen, stimulation of ammonifying bacteria following intensified yield of nitrogen might have resulted from other factors than the fungicide.35 Nevertheless, an inhibitory effect of tebuconazole on denitrification was noticed by the decreasing amount of denitrifying bacteria.35 The process of ammonification is typically unaffected by stress. Nitrification however, where only limited microbial communities are involved, is receptive to stress.36 Monkiedje and Spiteller could prove reversible inhibitory effects of the fungicides mefenoxam and metalaxyl on ammonifying and nitrifying bacteria in sand, clay, loam and sandy loam soils, following growth stimulation of both groups. Additionally, dehydrogenase activity, present in all living microbial cells and representing a marker for microbial respiratory processes37 was decreased, whereas phosphatase and β-glucosidase activities were enhanced in both fungicide-treated soils.
Contrary to Cycón et al.35 and Monkiedje & Spiteller,36 Muñoz-Leoz et al.38 demonstrated, that tebuconazole affects the microbial populations in soil at various concentrations, and that microbial biomass was not revitalized over time (90 days). Besides, adverse effects, that were concentration independent, on basal respiration were noticed. In our experiments, however, the negative effect of the fungicides on three of four soils was only transient and the soil degradation potentials were regained towards the end of the respective incubation periods, indicating recovery of the microbial population in these fungicide treated soils over time.
Zhang et al.39 showed that another widely used triazole fungicide, difenoconazole, adversely affected the soil bacterial diversity in five different soils incubated for up to 60 days. This effect, however, was correlated with the fungicide concentration (3.75 to 10 mg kg−1) and soil type.
Mainly involved in the biodegradation of pesticides in the soil and aquatic environment are bacteria, fungi, and algae. Common bacterial species involved are Pseudomonas, Bacillus, Alcaligenes, Flavobacterium, and actinomycetes Micromonospora, Actinomyces, Nocardia and Streptomyces. Fungi are represented by Aspergillus, Penicillium, Fusarium, Mucor, Trichoderma spp, Morteriella sp., Rhizopus, Cladosporium, and lignin degraders white-rot fungi. Biodegradation of pesticides is usually promoted by a combination of various microbes and consists of several dissimilar reactions, while proceeding via mineralization, and via co-metabolism as important drivers.40,41
The herbicide iodosulfuron-methyl-sodium supposably has an effect on the soil fungal biomass. Baćmaga et al.31 reported that a mixture of the herbicides diflufenican, mesosulfuron-methyl and iodosulfuron-methyl-sodium, at different concentrations, impact microbial and enzymatic activities in sandy loam soil. The prevalence of all tested microbial orders was affected: the mixture stimulated a number of oligotrophic bacteria, sporulating oligotrophic bacteria, organotrophic bacteria and actinomycetes. An adverse effect, however, was noted on Azotobacter bacteria, an essential marker of soil pollution, and fungi. Additionally, a shift in the soil microbial diversity detrimental to fungi did occur, as assessed by colony development and ecophysiological indices through colony counting. Distortions of microbial abundance and diversity were correlated with the mixture concentration. Furthermore, dehydrogenase, acid phosphatase, alkaline phosphatase and arylsulfatase activities were inhibited. Dehydrogenases were largely affected. Vazquez and Bianchinotti42 tested the capability of thirty fungi strains to effectively metabolize metsulfuron-methyl, the main degradation product of iodosulfuron-methyl-sodium in soils. Three strains, Mucor, Penicillium and Trichoderma, were able to utilize metsulfuron-methyl as an exclusive source of carbon and were isolated from Argentinian silt loam soils treated with the herbicide. Growth of Penicillium strains however, was significantly decelerated in the presence of metsulfuron-methyl, an effect that was also signalized by He et al.43 They could demonstrate that the addition of isolated Penicillium sp. significantly increased the degradation of metsulfuron-methyl in a liquid medium and soil. Per contra, metsulfuron-methyl initially induced a harmful effect on Penicillium sp., thereby hindering its metabolization in soil. Similar interactions with the fungal biomass in the four soils tested might proceed from the structural analog and parent molecule iodosulfuron-methyl-sodium.
Aerobic degradation of iodosulfuron-methyl-sodium occurred at different rates in the four soils. Silt loam 1 soil (Am Fischteich) was observed to be capable of degrading iodosulfuron-methyl-sodium most rapidly, followed by silt loam 2 (Bellevue), sandy loam (Pavillion) and loamy sand (Speyer 2.1) soils. The degradation velocity of the herbicide in respective soils was positively correlated with the soil texture, the clay fraction, and with the active microbial biomass, each being highest in the silt loam 1 soil (Am Fischteich; Table 1). Metabolization was constrained in sandier soils (Pavillion and Speyer 2.1) a typical characteristic of low water holding capacity and hence inferior biomass content. Whereas Swarcewicz and Gregorczyk21 suggested that the influence of soil moisture on the degradation behavior of chemical compounds is dependent on the soil type, soil moisture itself, in fact, plays a more dominant role than the soil texture.44,45 The soil moisture content at pF 2 and the amount of organic carbon, which contributes to dissipation of pollutants,46 were the highest in both fast-degrading silt loam soils (Am Fischteich and Bellevue). Therefore, these parameters might have played a significant role in the degradation of the herbicide as well. Iodosulfuron-methyl-sodium is hydrolytically stable under neutral and slightly alkaline pH conditions. Hence, the acidic pH had a lesser impact on the degradation of the herbicide in the loamy sand soil (Speyer 2.1).30,47,48
When comparing neutral silt loam 2 soil (Bellevue, pH 6.7) and alkaline sandy loam soil (PaviIlion, pH 8.1), ester hydrolysis in the side-chain of iodosulfuron-methyl-sodium and metsulfuron-methyl advanced via enzymatic activity, whereas abiotic hydrolysis was precluded. As a consequence of the acidic and relatively moist characteristics of the silt loam 1 soil (Am Fischteich, pH 5.6) however, degradation of iodosulfuron-methyl-sodium to AE F145740, and degradation of metsulfuron-methyl to AE 0014966, which was detected in major amounts greater than 10% AR in both silt loam soils (Am Fischteich and Bellevue), could additionally have proceeded via acid hydrolysis of the methyl ester.49 This was indicated by increasing proportions of the metabolite AE 0014966 in sandy loam (Pavillion), silt loam 2 (Bellevue) and silt loam 1 (Am Fischteich) soils throughout incubation, accounting for approximately 9%, 19% and 21% AR, respectively at the end of incubation. Formation of metabolite AE F145740 was observed in all soils and at major mean amounts of up to 11% and 14% AR in silt loam am Fischteich soil after 6 days and in loamy sand Speyer 2.1 soil after 50 days, respectively. In silt loam Bellevue and sandy loam Pavillion soils, AE F145740 remained less than 10% AR in every sample. Details are provided in Table S1 of the ESI.† Obstructed degradation of iodosulfuron-methyl-sodium was mainly expressed by significantly abated incidence of its main metabolite metsulfuron-methyl. Accordingly, tebuconazole and/or prothioconazole have an adverse effect on the biodegradation of iodosulfuron-methyl-sodium in the four tested soils by harming or altering their microbial composition accountable for the biotic transformation of iodosulfuron-methyl-sodium into metsulfuron-methyl via elimination of iodine from the phenyl ring.
The hampered degradation of rapidly biodegradable iodosulfuron-methyl-sodium in soil might not have any essential impact on regulatory risk assessment of this agrochemical since the trigger value for persistence in soil corresponds to a half-life of 120 days. For slower biodegrading compounds, where the half-life in soils reaches 120 days, refined risk assessments considering combined treatment of pesticides, however, might classify such molecules as persistent in the terrestrial environment. Consequently, respective agrochemicals would be restricted or banned for agricultural use. Accordingly, an additional experiment is ongoing, where the influence of fungicides tebuconazole and prothioconazole on the degradation of a herbicide with a regular half-life of about 100–120 days in alkaline soil, is evaluated.
Nevertheless, the potential impact of pesticide mixtures on non-target soil organisms should be highlighted. Gomes et al.50 investigated the reproduction effects of tebuconazole and prothioconazole individually, as well as the mixture in the formulation Prosaro on non-target soil oligochaete Enchytraeus crypticus. The concentration range of up to 250 mg kg−1 soil for both fungicides covered the concentrations used in our experiments. They revealed that the reproduction toxicity of Prosaro was explained based on tebuconazole rather than prothioconazole, whereas survival toxicity was predicted based on Prosaro being more harmful to the soil invertebrates than its individual chemicals. Besides, Tebuconazole, among selected herbicides and other fungicides, has a less harmful effect on the soil microbial communities when penetrating into the soil as a slow release formulation, respectively embedded in a biodegradable composite matrix such as polymer poly-3-hydroxybutyrate, rather than as a free pesticide, as demonstrated by Prudnikova et al.51 They tested the free and embedded pesticides at 0.1 mg per g of soil on various strains of polyhydroxyalkanoate degrading bacteria and fungi. Free tebuconazole extensively harmed the abundance and diversity of the microbial soil composition, which did not recover to the untreated control state for 3 months after application. In contrast, gradually released matrix-embedded pesticides did not significantly repress the microbial soil life and even invigorated the development of certain strains of microorganisms, maintaining the soil degradation potential.
New perceptions on pesticide and specifically fungicide effects in the natural environment can be incorporated in smarter regulatory frameworks on pesticide risk assessments, as suggested by Schäfer et al.,52 who advised a future triple stage evaluation of chemicals. Stage 1 would include short-term modifications of the current system with higher safety factors and estimation of the cost benefits by comparing agricultural against environmental implications. Stage 1, rather overprotective, would run to stage 2, which requires further adaptation of the regulatory context and would involve post-authorization monitoring of the active substance in real test landscapes and catchments. Subsequently, the final stage 3 would connect separate environmental policy frameworks towards an integrated pesticide regulation framework, including regional domain (landscapes and catchments) management and stakeholders (industry, authorities, farmers, and conservationists) sharing their responsibility for a sustainable environment and society.
Finally, soils act as the buffer zone to preserve groundwater and plants against pollutants.31 Therefore, reduction of agricultural fungicide use by promotion of more sustainable food production in combination with impeding progression of arable land into surrounding areas and minimizing agrochemical leakage into the aquatic environment is highly desirable.53
5. Conclusion
We showed that the fungicides tebuconazole and/or prothioconazole have a significant adverse effect on the biodegradation of the herbicide iodosulfuron-methyl-sodium in soil. This effect was illustrated by inhibited biotic transformation of the herbicide into its main soil metabolite metsulfuron-methyl, which proceeds through elimination of iodine from the phenyl ring. Except for one sandy soil, this adverse effect was transient and mitigated towards the end of the respective incubation periods. Half-lives (DT50) and DT90 values of iodosulfuron-methyl-sodium in the four fungicide-treated soils increased 1.5 to 2.9-fold and 1.3 to 2.2-fold, respectively when compared to those of the untreated control soils. It remains undefined, which interactions of the fungicides with the soil microflora are leading to the decelerated metabolization of the herbicide, and these processes demand further investigations.
Funding
This research received no external funding.
Author contributions
CW designed this study. CW and YW performed the laboratory experiments. CW evaluated the data and drafted the manuscript. AS, HH, KN, SH and YW revised the manuscript. All authors accepted to submit for publication. CW = Christiaan Wijntjes, YW = Yanik Weber, SH = Stefan Höger, KN = Kim Thu Nguyen, HH = Henner Hollert, and AS = Andreas Schäffer.
Conflicts of interest
The authors declare no conflict of interest.
Acknowledgements
We are grateful to Claudine Mattler, Fitore Krasniqi, Sebastian Müller and Pascal Luder for their relentless assistance in the lab.
References
-
Worldometers.info. Current World Population, 2021, available from: URL: https://www.worldometers.info/world-population/ Search PubMed.
-
R. Oliver and H. G. Hewitt, Fungicides in Crop Protection, Wallingford, CABI, 2014 Search PubMed.
- J. P. Zubrod, M. Bundschuh, G. Arts, C. A. Brühl, G. Imfeld and A. Knäbel,
et al. Fungicides: An Overlooked Pesticide Class?, Environ. Sci. Technol., 2019, 53(7), 3347–3365 CrossRef CAS PubMed.
- M. Syafrudin, R. A. Kristanti, A. Yuniarto, T. Hadibarata, J. Rhee and W. A. Al-Onazi,
et al. Pesticides in Drinking Water-A Review, Int. J. Environ. Res. Public Health, 2021, 18(2), 468 CrossRef CAS PubMed.
- M. V. Martinez-Toledo, V. Salmeron, B. Rodelas, C. Pozo and J. Gonzalez-Lopez, Studies on the effects of a chlorinated hydrocarbon insecticide, lindane, on soil microorganisms, Chemosphere, 1993, 27(11), 2261–2270 CrossRef CAS.
- M. Šudoma, N. Peštálová, Z. Bílková, P. Sedláček and J. Hofman, Ageing effect on conazole fungicide bioaccumulation in arable soils, Chemosphere, 2021, 262, 127612 CrossRef PubMed.
- M. Komárek, E. Čadková, V. Chrastný, F. Bordas and J.-C. Bollinger, Contamination of vineyard soils with fungicides: a review of environmental and toxicological aspects, Environ. Int., 2010, 36(1), 138–151 CrossRef PubMed.
-
FAO, Tebuconazole: Part I: Residues [Report of the Joint Meeting of the FAO Panel of Experts on Pesticide Residues in Food and the Environment], Food and Agriculture Organization of the United Nations, Rome, Italy, 1997, available from: URL: http://www.fao.org/fileadmin/templates/agphome/documents/Pests_Pesticides/JMPR/Evaluation97/Tebucon.PDF Search PubMed.
-
U.S. EPA, Tebuconazole: Ecological Risk Assessment for Section 3 Registration of Tebuconazole on Wheat, Cucurbits, Bananas, Turnips, Tree Nuts, Hops, and Sunflowers, U.S.A.: United States Environmental Protection Agency, Office of Prevention, Pesticides and Toxic Substances, Washington D.C. 20460, 2000 Search PubMed.
- European Food Safety Authority, Conclusion on the peer review of the pesticide risk assessment of the active substance tebuconazole, EFSA J., 2014, 12(1), 1 Search PubMed.
-
Bayer CropScience Limited, Tank-mix sheet: Prosaro, 2021, available from: URL: https://cropscience.bayer.co.uk/data/documents/fungicides/prosaro/prosaro-tank-mixes/ Search PubMed.
- I. M.-Y. Kwok and R. T. Loeffler, The biochemical mode of action of some newer azole fungicides, Pestic. Sci., 1993, 39(1), 1–11 CrossRef CAS.
- G. I. Lepesheva and M. R. Waterman, Sterol 14alpha-demethylase cytochrome P450 (CYP51), a P450 in all biological kingdoms, Biochim. Biophys. Acta, 2007, 1770(3), 467–477 CrossRef CAS PubMed.
- European Food Safety Authority, Conclusion regarding the peer review of the pesticide risk assessment of the active substance prothioconazole, EFSA J., 2007, 5(8), 106r CrossRef.
-
F. A. O. Prothioconazole, Pesticide Residues in Food – 2008 [Report of the Joint Meeting of the FAO Panel of Experts on Pesticide Residues in Food and the Environment]. FAO Plant Production and Protection Paper 193, Food and Agriculture Organization of the United Nations, Rome, Italy, 2009, available from: URL: http://www.fao.org/fileadmin/templates/agphome/documents/Pests_Pesticides/JMPR/Report08/Prothioconazole.pdf Search PubMed.
-
U.S. EPA, Prothioconazole: Pesticide Fact Sheet, U.S.A.: United States Environmental Protection Agency, Office of Prevention, Pesticides and Toxic Substances (7501 P), Washington D.C. 20460, 2007 Search PubMed.
-
U.S. EPA, Iodosulfuron-Methyl-Sodium Technical: Registration, U.S.A.: United States Environmental Protection Agency, Office of Pesticide Programs, Registration Division (7505C), Washington D.C. 20460, 2002 Search PubMed.
- European Food Safety Authority, Peer review of the pesticide risk assessment of the active substance iodosulfuron-methyl-sodium (approved as iodosulfuron), EFSA J., 2016, 14(4) Search PubMed.
-
U.S. EPA, Tebuconazole: Registration Review Label Mitigation for Iodosulfuron-Methyl-Sodium, U.S.A.: United States Environmental Protection Agency, Office of Chemical Safety and Pollution Prevention, Washington D.C. 20460, 2019 Search PubMed.
- J. Riedo, F. E. Wettstein, A. Rösch, C. Herzog, S. Banerjee and L. Büchi,
et al. Widespread Occurrence of Pesticides in Organically Managed Agricultural Soils-the Ghost of a Conventional Agricultural Past?, Environ. Sci. Technol., 2021, 55(5), 2919–2928 CrossRef CAS PubMed.
- M. K. Swarcewicz and A. Gregorczyk, The effects of pesticide mixtures on degradation of pendimethalin in soils, Environ. Monit. Assess., 2012, 184(5), 3077–3084 CrossRef CAS PubMed.
- J. Anderson and K. H. Domsch, A physiological method for the quantitative measurement of microbial biomass in soils, Soil Biol. Biochem., 1978, 10(3), 215–221 CrossRef CAS.
-
OECD, OECD Guideline for the Testing of Chemicals: Test No. 307: Aerobic and Anaerobic Transformation in Soil. Organisation for Economic Cooperation and Development (OECD), 2002 Search PubMed.
-
U.S. EPA, Fate, Transport and Transformation Test Guidelines: OPPTS 835.4100 Aerobic Soil Metabolism, OPPTS 835.4200 Anaerobic Soil Metabolism, United States Environmental Protection Agency, 7101, 2008, available from: URL: https://nepis.epa.gov/Exe/ZyNET.exe/P100J6WF.TXT?ZyActionD=ZyDocument&Client=EPA&Index=2006+Thru+2010&Docs=&Query=&Time=&EndTime=&SearchMethod=1&TocRestrict=n&Toc=&TocEntry=&QField=&QFieldYear=&QFieldMonth=&QFieldDay=&IntQFieldOp=0&ExtQFieldOp=0&XmlQuery=&File=D%3A%5Czyfiles%5CIndex%20Data%5C06thru10%5CTxt%5C00000035%5CP100J6WF.txt&User=ANONYMOUS&Password=anonymous&SortMethod=h%7C-&MaximumDocuments=1&FuzzyDegree=0&ImageQuality=r75g8/r75g8/x150y150g16/i425&Display=hpfr&DefSeekPage=x&SearchBack=ZyActionL&Back=ZyActionS&BackDesc=Results%20page&MaximumPages=1&ZyEntry=1&SeekPage=x&ZyPURL Search PubMed.
- S. A. Holm, Simple Sequentially Rejective Multiple Test Procedure, Scand. J. Stat., 1979, 6(2), 65–70 Search PubMed . available from: URL: http://www.jstor.org/stable/4615733.
-
FOCUS, Guidance Document on Estimating Persistence and Degradation Kinetics from Environmental Fate Studies on Pesticides in EU Registration: Report of the FOCUS Work Group on Degradation Kinetics, EC Document Reference Sanco/10058/2005 version, p. 434; 2006, available from: URL: https://esdac.jrc.ec.europa.eu/public_path/projects_data/focus/dk/docs/finalreportFOCDegKinetics.pdf Search PubMed.
-
Computer Assisted Kinetic Evaluation (CAKE). Version 3.4, Abingdon, Oxfordshire, UK, Syngenta, 2020 Search PubMed.
-
T. Katagi, Behavior of Pesticides in Water-Sediment Systems, in Reviews of Environmental Contamination and Toxicology: Continuation of Residue Reviews, ed. Ware GW, Albert LA, Voogt P de, Gerba CP, Hutzinger O, Knaak JBet al.New York, NY, Springer New York, 2006, pp. 133–251 Search PubMed.
-
Modern Crop Protection Compounds, ed. Jeschke P, Witschel M, Krämer W and Schirmer U, Wiley-VCH, Weinheim, Germany, 3rd edn, 2019 Search PubMed.
-
R. C. Fischer, Monograph, Iodosulfuron-methyl-sodium: Draft Assessment Report, Rapporteur Member State, Germany, 2000 Search PubMed.
- M. Baćmaga, A. Borowik, J. Kucharski, M. Tomkiel and J. Wyszkowska, Microbial and enzymatic activity of soil contaminated with a mixture of diflufenican + mesosulfuron-methyl + iodosulfuron-methyl-sodium, Environ. Sci. Pollut. Res., 2015, 22(1), 643–656 CrossRef PubMed.
- V. Silva, H. G. J. Mol, P. Zomer, M. Tienstra, C. J. Ritsema and V. Geissen, Pesticide residues in European agricultural soils - A hidden reality unfolded, Sci. Total Environ., 2019, 1532–1545 CrossRef CAS PubMed.
- M. Hvězdová, P. Kosubová, M. Košíková, K. E. Scherr, Z. Šimek and L. Brodský,
et al. Currently and recently used pesticides in Central European arable soils, Sci. Total Environ., 2018, 613–614, 361–370 CrossRef PubMed.
- N. Begum, C. Qin, M. A. Ahanger, S. Raza, M. I. Khan and M. Ashraf,
et al. Role of Arbuscular Mycorrhizal Fungi in Plant Growth Regulation: Implications in Abiotic Stress Tolerance, Front. Plant Sci., 2019, 10, 1068 CrossRef PubMed.
- M. Cycoń, Z. Piotrowska-Seget, A. Kaczyńska and J. Kozdrój, Microbiological characteristics of a sandy loam soil exposed to tebuconazole and lambda-cyhalothrin under laboratory conditions, Ecotoxicology, 2006, 15(8), 639–646 CrossRef PubMed.
- A. Monkiedje and M. Spiteller, Effects of the phenylamide fungicides, mefenoxam and metalaxyl, on the microbiological properties of a sandy loam and a sandy clay soil, Biol. Fertil. Soils, 2002, 35(6), 393–398 CrossRef CAS.
- H. R. Gerber, J. P. E. Anderson, B. Bügel-Mogensen, D. Castle, K. H. Domsch and H.-P. Malkomes,
et al. 1989 Revision of recommended laboratory tests for assessing side-effects of pesticides on the soil microflora, Toxicol. Environ. Chem., 1991, 30(3–4), 249–261 CrossRef.
- B. Muñoz-Leoz, E. Ruiz-Romera, I. Antigüedad and C. Garbisu, Tebuconazole application decreases soil microbial biomass and activity, Soil Biol. Biochem., 2011, 43(10), 2176–2183 CrossRef.
- H. Zhang, J. Song, Z. Zhang, Q. Zhang, S. Chen and J. Mei,
et al. Exposure to fungicide difenoconazole reduces the soil bacterial community diversity and the co-occurrence network complexity, J. Hazard. Mater., 2021, 405, 124208 CrossRef CAS PubMed.
- Y. Huang, L. Xiao, F. Li, M. Xiao, D. Lin and X. Long,
et al. Microbial Degradation of Pesticide Residues and an Emphasis on the Degradation of Cypermethrin and 3-phenoxy Benzoic Acid: A Review, Molecules, 2018, 23(9), 2313 CrossRef PubMed.
- J. Aislabie and G. Lloyd-Jones, A review of bacterial-degradation of pesticides, Soil Res., 1995, 33(6), 925 CrossRef CAS.
- M. B. Vázquez and M. V. Bianchinotti, Isolation of metsulfuron methyl degrading fungi from agricultural soils in Argentina, Phyton, 2013, 82(1), 113–118 Search PubMed.
- Y. H. He, D. S. Shen, C. R. Fang and Y. M. Zhu, Rapid biodegradation of metsulfuron-methyl by a soil fungus in pure cultures and soil, World J. Microbiol. Biotechnol., 2006, 22(10), 1095–1104 CrossRef CAS.
- R. L. Zimdahl, P. Catizone and C. Butcher Ann, Degradation of Pendimethalin in Soil, Weed Sci., 1984, 32(3), 408–412 CrossRef CAS , available from: URL: http://www.jstor.org/stable/4043955.
- R. L. Zimdahl, B. K. Cranmer and W. W. Stroup, Use of Empirical Equations to Describe Dissipation of Metribuzin and Pendimethalin, Weed Sci., 1994, 42(2), 241–248 CrossRef CAS . available from: URL: http://www.jstor.org/stable/4045401.
-
U. Gisi, Soil Ecology, 2nd edn, Stuttgart, New York, G. Thieme, 1997, (Flexibles Taschenbuch) Search PubMed.
- K. A. Lewis, J. Tzilivakis, D. J. Warner and A. Green, An international database for pesticide risk assessments and management, Hum. Ecol. Risk Assess., 2016, 22(4), 1050–1064 CrossRef CAS.
- M. Brigante, C. Emmelin, L. Previtera, R. Baudot and J. M. Chovelon, Abiotic Degradation of Iodosulfuron-methyl-ester in Aqueous Solution, J. Agric. Food Chem., 2005, 53(13), 5347–5352 CrossRef CAS PubMed.
- M. M. Gul and K. S. Ahmad, Assessment of methyl 2-({[(4,6-dimethoxypyrimidin-2-yl)carbamoyl] sulfamoyl}methyl)benzoate through biotic and abiotic degradation modes, Open Chem., 2020, 18(1), 314–324 CAS.
- S. I. L. Gomes, A. Ammendola, S. Casini and M. J. B. Amorim, Toxicity of fungicides to terrestrial non-target fauna - Formulated products versus active ingredients (azoxystrobin, cyproconazole, prothioconazole, tebuconazole) - A case study with Enchytraeus crypticus (Oligochaeta), Sci. Total Environ., 2021, 754, 142098 CrossRef CAS PubMed.
- S. Prudnikova, N. Streltsova and T. Volova, The effect of the pesticide delivery method on the microbial community of field soil, Environ. Sci. Pollut. Res., 2021, 28(7), 8681–8697 CrossRef CAS PubMed.
- R. B. Schäfer, M. Liess, R. Altenburger, J. Filser, H. Hollert and M. Roß-Nickoll,
et al. Future pesticide risk assessment: narrowing the gap between intention and reality, Environ. Sci. Eur., 2019, 31(1) DOI:10.1186/s12302-019-0203-3.
-
A. Feckler, Chemical Stressors Influence Aquatic Ecosystem Processes: Fungicide Effects on Decomposer Communities and Primary Consumers, Doctoral thesis, Swedish University of Agricultural Sciences, Uppsala, Sweden 2017 Search PubMed.
Footnote |
† Electronic supplementary information (ESI) available. See DOI: 10.1039/d1va00021g |
|
This journal is © The Royal Society of Chemistry 2022 |