DOI:
10.1039/D2SC04750K
(Edge Article)
Chem. Sci., 2022,
13, 13836-13842
Alkynyl Prins carbocyclization cascades for the synthesis of linear-fused heterocyclic ring systems†
Received
25th August 2022
, Accepted 4th November 2022
First published on 10th November 2022
Abstract
We report a Brønsted acid-catalyzed carbocyclization cascade, featuring condensation of an alcohol/sulfonamide with an aldehyde followed by an intramolecular three-component coupling involving an alkyne, an oxocarbenium/iminium ion, and an arene. A formal cycloaddition is embedded in the cationic cascade, which enables the synthesis of a wide range of fused heterotricycles. The diastereoselectivity of the cascade is studied using secondary alcohols/sulfonamides with different carbonyl partners. The described method results in the preparation of synthetically versatile scaffolds with ample opportunity for further derivatization at the resulting tetrasubstituted olefin, or by inclusion of other functionalizable motifs from the starting materials. It is worth noting that this chemistry also facilitates the synthesis of piperidines and 1,4-oxazepanes, as well as the inclusion of indoles and benzofurans, which are privileged motifs for medicinal chemistry. Herein we present the generality of this approach and some chemical transformations that can be achieved with our substrates.
Introduction
The development of methods that enable the synthesis of small molecules is essential for populating high throughput screening libraries used in drug discovery campaigns.1 In particular, the preparation of synthetically versatile molecules that allow for differential functionalization around a polycyclic scaffold will help maximize diversity among derivatives and provide the ability to tune physicochemical properties.2
Alkyne-carbonyl coupling (Prins) reactions most commonly terminate with the capture of a presumed vinyl cation intermediate 2 with a nucleophile (Scheme 1).3
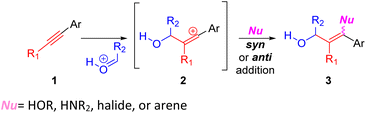 |
| Scheme 1 Alkyne-carbonyl coupling (Prins) reactions. = HOR, HNR2, halide, or arene. | |
Without a means to control stereoselectivity or E/Z geometry, the synthetic utility of these reactions is limited. The most well-developed versions feature intramolecular alkyne–carbonyl couplings, with a water or a halide acting to capture the intermediate cation 2.4 Our lab has recently been engaged in the development of alkynyl Prins coupling methods: Prins terminated by halide,5 aza-Prins terminated by halide,6 and Prins annulation terminated with sulfonamide.7 More rarely, the vinyl cation intermediate can be trapped with an arene nucleophile, terminating the Prins coupling with C–C rather than C-heteroatom bond formation. Intermolecular C–C bond formation (cf.Scheme 1;
= arene) is only successful when the arene reactant is in large excess (as solvent).8
Examples of intramolecular arene capture are limited to those shown in Scheme 2. Stepwise [4 + 2] cycloaddition processes generate functionalized naphthalenes 5 (Scheme 2A),9 and cationic cascades developed by Hinkle et al. generate benzo[f]isochromenes 8 (Scheme 2B).10 These two types of carbocyclizations employ simple (phenyl) arenes as the terminating nucleophile and eliminate H2O (via4 and 7) to generate a naphthalene moiety.
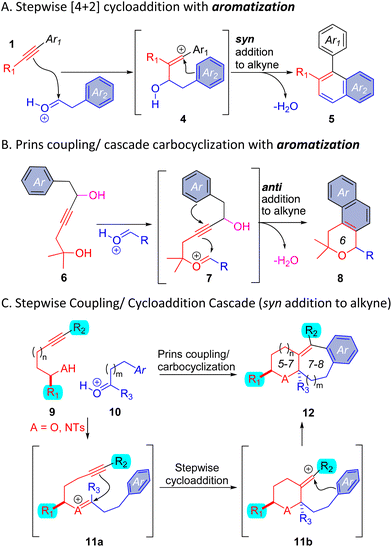 |
| Scheme 2 Cascade Prins carbocyclizations. (A) Stepwise [4 + 2] cycloaddition with aromatization. (B) Prins coupling/cascade carbocyclization with aromatization. (C) Stepwise coupling/cycloaddition cascade (syn addition to alkyne). | |
In this paper, we describe a carbocyclization cascade that generates fused polycyclic adducts 12 (Scheme 2C). Condensation of readily available building blocks 9 and 10 generates an oxocarbenium or iminium ion 11a, aligned with an alkyne and an arene such that a formal cycloaddition occurs diastereoselectively. As indicated in Scheme 2C, the broad scope of the one-step process enables variation of ring size, at R1, R2, R3, and in the arene ring. The scaffolds represent a novel structure class, offering entry into new polycyclic chemical space.
Optimization of the Prins cascade carbocyclization
Optimization experiments for this reaction were done using arenyne alcohol 9a and aldehyde 10a, with an electron-rich nucleophilic tether (Table 1). Substoichiometric amounts of trifluoromethanesulfonic acid (TfOH) are sufficient to promote the cyclization to afford 12a. This, in combination with 5 Å molecular sieves (MS) in a solvent mixture of dichloromethane (DCM) and 1,1,1,3,3,3-hexafluoropropan-2-ol (HFIP) results in optimal yields (see Table 1). HFIP was chosen as cosolvent due to its demonstrated ability to stabilize carbocationic intermediates.11 Omission of the HFIP gives the desired product 12a in 40% yield, as well as unreacted aldehyde, acetal (resulting from condensation of the aldehyde with two molecules of arenyne alcohol; see ESI† for details), and other unidentifiable byproducts (Table 1, entry 1). Lowering the TfOH loading to 10 mol% gives good yields for 12a (entry 2), however, since other substrates required more acid for full consumption of the starting material, we chose 20 mol% TfOH as part of our standard conditions. Reduction of the amount of HFIP by half gives 78% yield of cyclized product 12a (entry 3). Using bis(trifluoromethane)sulfonamide (Tf2NH) instead of TfOH also affords the desired product in good yields (entry 4). Finally, exclusion of molecular sieves leads to sluggish reactions where the starting material is never fully consumed, even after warming to room temperature (entry 5).
Table 1 Optimization of the alkynyl Prins carbocyclization
Substrate scope
Using these optimized conditions, the alkyne and carbonyl scope was explored. Electron-rich, neutral, and deficient arenyne alcohols react cleanly to give fused products 12a–12g in good to excellent yields (Scheme 3). Enyne alcohols work as well to afford products 12h–12j in good yields. An internal alkyne engages to give product 12k in 64% yield, although 40 mol% of TfOH and stirring at room temperature is required for the reaction to go to completion, otherwise the acetal of 10a is isolated (see ESI†). Although electron deficient arenynes engage well in this chemistry, 4-cyanoarenyne (a more basic functional group) is not tolerated. Similarly, tests with a terminal alkyne, bromoalkyne, alkynylsilane, and diyne also result in the formation of complex mixtures (see ESI†).
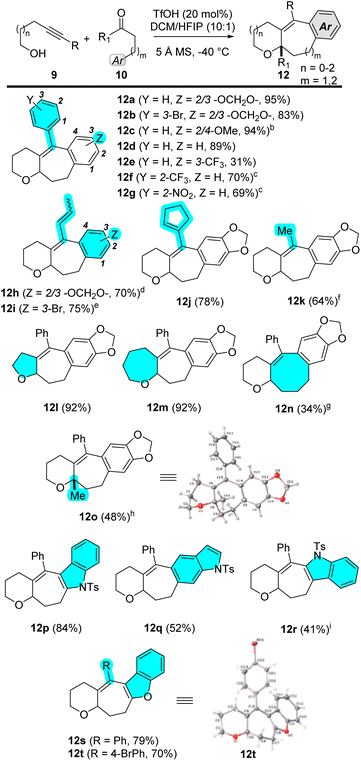 |
| Scheme 3 Substrate scope for alkynyl oxa-Prins carbocyclization. (a) Conditions: to a mixture of 9, 10, and molecular sieves in DCM/HFIP at −40 °C was added TfOH, dropwise. Stir at −40 °C until all starting material is consumed by TLC (b) 1.7 : 1 p/o-trapped (c) warmed to 0 °C 1 hour after adding TfOH, then to room temperature (d) 6.3 : 1 E/Z (e) 7.9 : 1 E/Z (f) 40 mol% TfOH added at −40 °C, then warmed to room temperature after an hour (g) 40 mol% TfOH added at −40 °C, then to 0 °C after an hour (h) 1.5 equiv. of corresponding dimethyl ketal 10 (R1 = Me) used with 1.0 equiv. of alcohol 9a (i) 1 : 1 mixture of conformers. | |
Aldehyde 10 (with Ar = m-methoxyphenyl) reacts well to give cycloaddition product 12c as a 1.7
:
1 mixture of the para/ortho-trapped product in 94% combined yield. Aldehyde 10 (with Ar = Ph) also traps well to give 12d in 89% yield. Even an electron-deficient arene engages to give 12e, albeit in 31% yield. Different tether lengths of the alcohol work without any negative impact on the yield, giving access to five-membered oxacycle 12l in 92% yield, and seven-membered oxacycle 12m in 82% yields. Eight-membered carbocycle formation is also feasible under the reaction conditions to give product 12nvia a formal [6 + 2] pathway, albeit in lower yields. Ketone 10 (R1 = Me) also reacts to afford [5 + 2] adduct 12o, although in only 20% yield. Using the dimethyl ketal of 10 (R1 = Me) instead brings this yield up to 48% (Scheme 3).
Heteroaromatic nucleophiles also react smoothly under these conditions. Tosyl indole aldehydes engage well to afford products 12p, 12q, and 12r in 84, 52, and 41% yields, respectively. Product 12r is obtained as a 1
:
1 mixture of conformers. We hypothesize that these conformers arise from steric interactions between the tosyl protecting group and the phenyl ring on the cycloheptene (Scheme 3). This hypothesis is supported by the fact that, upon detosylation of the indole, a single product is observed (see ESI†). Benzofuran aldehydes give 12s (79% yield) and 12t (70% yield). When N-methyl, or deprotected indoles are subjected to the reaction conditions, the aldehyde undergoes decomposition, and no Prins product is formed (see ESI†).12
The alkynyl Prins reaction of secondary alcohols with aldehydes is known to proceed diastereoselectively.4g In a previous paper from our group, we demonstrated that the oxa-Prins reaction with an enantioenriched alcohol like 13 produces enantioenriched Prins adducts.7Scheme 4 shows the diastereoselectivity of our Prins coupling/cycloaddition approach, using secondary alcohols 13 and aldehydes or ketals 10 (R2 = Me, iPr). As evidenced with cases 14a through 14f, secondary alcohols 13 react well with aldehydes to give five-, six-, and seven-membered oxacycles in excellent yields and good diastereoselectivities. With a homoallylic alcohol, cycloaddition product 14g is generated in excellent yield and 16
:
1 dr. The diastereoselectivity is lower with a homopropargylic alcohol, which gives 14h (3.4
:
1 dr).
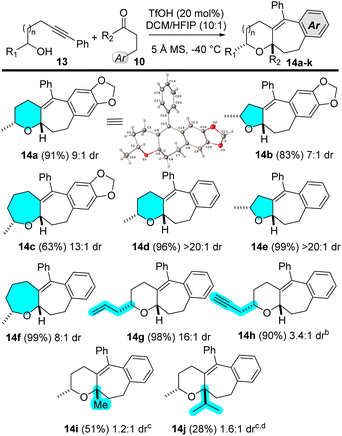 |
| Scheme 4 Diastereoselective Prins carbocyclizations with secondary alcohol reactants. (a) Conditions: to a mixture of 13, 10, and molecular sieves in DCM/HFIP at −40 °C was added TfOH, dropwise. Stir at −40 °C until all starting material is consumed by TLC. (b) Reported dr was observed for 0.2 mmol scale reaction. At 1.0 mmol scale, dr went down to 2.8 : 1. (c) Dimethyl ketal of corresponding ketone used (d) Reaction warmed up to 0 °C after 24 h until all the alcohol was consumed. | |
The Prins carbocyclization of secondary alcohol 13a and dimethyl ketal 10 (R2 = Me) gives Prins product 14i (51% yield), with virtually no diastereoselectivity. Replacing the methyl with a bulkier isopropyl group makes the reaction sluggish, delivering cycloaddition product 14j in 28% yield as a 1.6
:
1 mixture of diastereomers.13
Synthesis of linear-fused N-heterocyclic systems
Furthermore, it was exciting to discover that the reaction conditions translate to the preparation of piperidines from sulfonamides 15 (Scheme 5). The reaction performs well for alpha-primary sulfonamide 15a to give tosyl-protected piperidine 16a in 54% yield. Starting with alpha-secondary sulfonamide 15b leads to the diastereoselective formation of piperidine 16b in 56% yield and >20
:
1 dr. The tosyl protecting group of 16a can be removed using sodium naphthalenide to afford piperidine 17a in 81% yield.
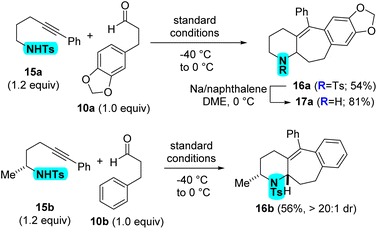 |
| Scheme 5 Carbocyclization for synthesis of fused piperidines. | |
It is also possible to prepare 1,4-oxazepane 19 in 89% yield from alcohol 18, using the standard reaction conditions (Scheme 6). A single crystal x-ray structure confirmed the identity of the cycloadduct.
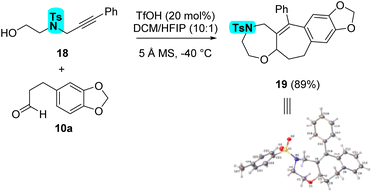 |
| Scheme 6 Prins carbocyclization for synthesis of 1,4-oxazepanes. | |
Finally, we focused on unmasking N-Ts indoles 12p, 12q, and 12r to access the N–H indole motif, which is ubiquitous among bioactive natural products and drug targets.14 In these cases, detosylation occurred smoothly upon treatment with potassium tert-butoxide (3 equiv.) in DMSO, providing access to the target scaffolds 20a–c as shown (Scheme 7).15
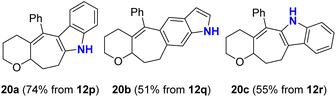 |
| Scheme 7 Deprotection of N-Ts indole substrates. | |
Diversification of polycyclic adducts
The synthetic versatility of products 12 and 14 can be exploited to give access to new chemical space. Scheme 8 shows examples of some chemical transformations that can be done to enhance the complexity of these scaffolds. Hydrogenation of the tetrasubstituted olefin in 12a occurs using palladium on carbon (Pd/C) and gives quantitative yields of 21a as a 1
:
1 mixture of diastereomers that can be separated by column chromatography. Hydrogenation of 14a gives the corresponding hydrogenated product as a 5
:
1 mixture of inseparable diastereomers (see ESI†). Oxidation of 14a with m-CPBA delivers epoxide 22a in 89% yield, with no purification required.
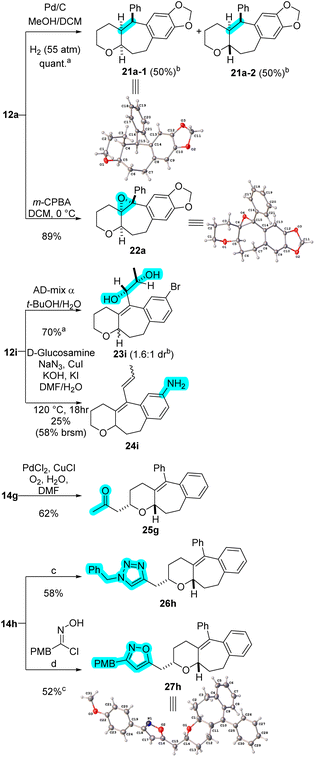 |
| Scheme 8 Diversification of cycloadducts. (a) Combined yield of two diastereomers (b) dr determined based on isolated yields of separable diastereoisomers (c) BnN3, Cu powder, CuSO4·5H2O, sodium ascorbate, H2O/tBuOH, rt. (d) Cu powder, CuSO4·5H2O, NaHCO3, H2O/tBuOH, rt. | |
The olefin in 12i can be oxidized with AD-mix α to give diol 23i as a 1.6
:
1 mixture of diastereomers that can be separated by column chromatography. The aryl bromide is a potential functional handle for subsequent cross-coupling operation. Aniline derivative 24i, for example, was obtained in 25% yield (58% based on recovered starting material) after a copper-mediated amination.16
Subjection of cycloadduct 14g (bearing a pendent alkene) to Wacker oxidation conditions gives ketone 25g in good yields.17 Finally, applying [3 + 2] cycloaddition strategies to cycloadduct 14h (bearing a pendent alkyne) enables efficient introduction of interesting heterocycles such as triazole 26h and isoxazole 27h.18
Conclusions
In summary, alkynyl Prins coupling/carbocyclization sequences have been developed for the diastereoselective preparation of novel polycyclic systems. The linear-fused ring arrays contain a saturated heterocyclic ring, a seven- or eight-membered carbocycle, and an aromatic or heteroaromatic ring. In the presence of substoichiometric amounts of acid, this method couples two simple building blocks (an aldehyde and an alkynyl alcohol) in a cascade sequence, forging three new bonds (one C–O; two C–C) and two new rings in one pot.
The polycyclic small molecules described here represent a new structure class.19 The multiple sites available for easy diversification should enable synthesis of derivatives that extend into new areas of chemical space. Thus, the method can offer access to a diverse library of compounds different from any that have been prepared and tested before. If the molecules exhibit biological activity, the simple synthetic procedure will allow for rapid preparation of analogs for follow-up studies. Ongoing work includes the exploration of an asymmetric carbocyclization, expanding the scope of the aza-Prins variant of the reaction, and exploring other means of trapping the vinyl cation intermediate.
Data availability
All of the related experimental and computational data are provided in the ESI.†
Author contributions
A. J. F. and J. J. H. designed the research study and prepared the manuscript. All the experiments, product characterization, and computational studies were performed by J. J. Hernandez.
Conflicts of interest
There are no conflicts to declare.
Acknowledgements
We thank the NSF (CHE-1900050 and CHE- 2154854) for funding this study. J. J. H acknowledges the NIGMS (1F31GM142259-01A1) for fellowship support. We thank Dr W. W. Brennessel (Dept. of Chemistry, University of Rochester) for running X-ray crystallography analyses and the NSF (CHE-1725028) for funding our X-ray diffractometer. We also thank Kevin Wells, the University of Rochester Mass Spectrometry Resource Laboratory, and are thankful for the NIH instrument grant (S10OD021486). We also thank Aleksa Milosavljevic (Dep. Of Chemistry, University of Rochester) for helpful discussions.
Notes and references
-
(a) S. Dandapani and L. A. Marcaurelle, Grand Challenge Commentary: Accessing new chemical space for 'undruggable' targets, Nat. Chem. Biol., 2010, 6, 861–863 CrossRef CAS PubMed;
(b) M. Follmann, H. Briem, A. Steinmeyer, A. Hillisch, M. H. Schmitt, H. Haning and H. Meier, An approach towards enhancement of a screening library: The Next Generation Library Initiative (NGLI) at Bayer — against all odds?, Drug Discov., 2019, 24, 668–672 CAS;
(c) A. Barker, J. G. Kettle, T. Nowak and J. E. Pease, Expanding medicinal chemistry space, Drug Discov., 2013, 18, 298–304 CAS;
(d) M. Garcia-Castro, S. Zimmermann, M. G. Sankar and K. Kumar, Scaffold Diversity Synthesis and Its Application in Probe and Drug Discovery, Angew. Chem., Int. Ed., 2016, 55, 7586–7605 CrossRef CAS PubMed.
-
(a) P. Ertl, S. Jelfs, J. Mühlbacher, A. Schuffenhauer and P. Selzer, Quest for the Rings. In Silico Exploration of Ring Universe to Identify Novel Bioactive Heteroaromatic Scaffolds, J. Med. Chem., 2006, 49, 4568–4573 CrossRef CAS PubMed;
(b) I. P. Silvestri and P. J. J. Colbon, The Growing Importance of Chirality in 3D Chemical Space Exploration and Modern Drug Discovery Approaches for Hit-ID, ACS Med. Chem. Lett., 2021, 12, 1220–1229 CrossRef CAS PubMed.
-
(a) S. Abdul-Rashed, C. Holt and A. J. Frontier, Alkynyl Prins and Alkynyl Aza-Prins Annulations: Scope and Synthetic Applications, Synth, 2020, 52, 1991–2007 CrossRef CAS;
(b) P. Padmaja, P. N. Reddy and B. V. Subba Reddy, Tandem Prins cyclizations for the construction of oxygen containing heterocycles, Org. Biomol. Chem., 2020, 18, 7514–7532 RSC.
-
(a) J. J. Jaber, K. Mitsui and S. D. Rychnovsky, Stereoselectivity and Regioselectivity in the Segment-Coupling Prins Cyclization, J. Org. Chem., 2001, 66, 4679–4686 CrossRef CAS PubMed;
(b) P. O. Miranda, D. D. Díaz, J. I. Padrón, J. Bermejo and V. S. Martín, Iron (III)-Catalyzed Prins-Type Cyclization Using Homopropargylic Alcohol: A Method for the Synthesis of 2-Alkyl-4-halo-5,6-dihydro-2H-pyrans, Org. Lett., 2003, 5, 1979–1982 CrossRef CAS PubMed;
(c) P. O. Miranda, D. D. Díaz, J. I. Padrón, M. A. Ramírez and V. S. Martín, Fe (III) Halides as Effective Catalysts in Carbon−Carbon Bond Formation: Synthesis of 1,5-Dihalo-1,4-dienes, α,β-Unsaturated Ketones, and Cyclic Ethers, J. Org. Chem., 2005, 70, 57–62 CrossRef CAS PubMed;
(d) P. O. Miranda, M. A. Ramírez, V. S. Martín and J. I. Padrón, Factors Controlling the Alkyne Prins Cyclization: The Stability of Dihydropyranyl Cations, Chem.–Eur. J., 2008, 14, 6260–6268 CrossRef CAS PubMed;
(e) P. O. Miranda, R. M. Carballo, V. S. Martín and J. I. Padrón, A New Catalytic Prins Cyclization Leading to Oxa- and Azacycles, Org. Lett., 2009, 11, 357–360 CrossRef CAS PubMed;
(f) G. Sabitha, K. B. Reddy, M. Bhikshapathi and J. S. Yadav, TMSI mediated Prins-type cyclization of ketones with homoallylic and homopropargylic alcohol: synthesis of 2,2-disubstituted-, spirocyclic-4-iodo-tetrahydropyrans and 5,6-dihydro-2H-pyrans, Tetrahedron Lett., 2006, 47, 2807–2810 CrossRef CAS;
(g) S. N. Chavre, H. Choo, J. H. Cha, A. N. Pae, K. I. Choi and Y. S. Cho, 5-Exocyclic Products, 2,3,5-Trisubstituted Tetrahydrofurans via Prins-Type Cyclization, Org. Lett., 2006, 8, 3617–3619 CrossRef CAS PubMed;
(h) S. N. Chavre, H. Choo, J. K. Lee, A. N. Pae, Y. Kim and Y. S. Cho, 5- and 6-Exocyclic Products, cis-2,3,5-Trisubstituted Tetrahydrofurans, and cis-2,3,6-Trisubstituted Tetrahydropyrans via Prins-Type Cyclization, J. Org. Chem., 2008, 73, 7467–7471 CrossRef CAS PubMed.
-
(a) G. Alachouzos and A. J. Frontier, Diastereoselective Construction of Densely Functionalized 1-Halocyclopentenes Using an Alkynyl Halo- Prins/Halo-Nazarov Cyclization Strategy, Angew. Chem., Int. Ed., 2017, 56, 15030–15034 CrossRef CAS PubMed;
(b) G. Alachouzos and A. J. Frontier, Cationic Cascade for Building Complex Polycyclic Molecules from Simple Precursors: Diastereoselective Installation of Three Contiguous Stereogenic Centers in a One-Pot Process, J. Am. Chem. Soc., 2019, 141, 118–122 CrossRef CAS PubMed;
(c) C. Holt, G. Alachouzos and A. J. Frontier, Leveraging the Halo-Nazarov Cyclization for the Chemodivergent Assembly of Functionalized Haloindenes and Indanones, J. Am. Chem. Soc., 2019, 141, 5461–5469 CrossRef CAS PubMed;
(d) G. Alachouzos, C. Holt and A. J. Frontier, Stereochemical Relay through a Cationic Intermediate: Helical Preorganization Dictates Direction of Conrotation in the halo-Nazarov Cyclization, Org. Lett., 2020, 22, 4010–4015 CrossRef CAS PubMed.
- J. J. Hernandez and A. J. Frontier, Synthesis of Spirocyclic Isoindolones Using an Alkynyl aza-Prins/Oxidative halo-Nazarov Cyclization Sequence, Org. Lett., 2021, 23, 1782–1786 CrossRef CAS PubMed.
- S. Abdul-Rashed, G. Alachouzos, W. W. Brennessel and A. J. Frontier, One-Pot Double-Annulation Strategy for the Synthesis of Unusual Fused Bis-Heterocycles, Org. Lett., 2020, 22, 4350–4354 CrossRef CAS PubMed.
-
(a) U. C. Reddy and A. K. Saikia, One-Pot, Three-Component Synthesis of 4-Aryl-5,6-dihydropyran via Prins-Friedel-Crafts Reaction, Synlett, 2010, 2010, 1027–1032 CrossRef;
(b) T. Kotipalli and D.-R. Hou, Synthesis of Indenes by a BF3·OEt2-Mediated, One-Pot Reaction of Aryl Homopropargyl Alcohols, Aldehydes, and Arenes, Org. Lett., 2018, 20, 4787–4790 CrossRef CAS PubMed.
-
(a) G. S. Viswanathan, M. Wang and C. J. Li, A highly regioselective synthesis of polysubstituted naphthalene derivatives through gallium trichloride catalyzed alkyne–aldehyde coupling, Angew. Chem., 2002, 114, 2242–2245 CrossRef;
(b) Z.-Q. Wang, Y. Liang, Y. Lei, M.-B. Zhou and J.-H. Li, Iron-catalyzed annulations of 2-(2-alkynyl)phenoxy)-1-arylethanones leading to substituted naphthalen-1-ols, Chem. Commun., 2009, 5242–5244 RSC;
(c) S. Ponra, M. R. Vitale, V. Michelet and V. Ratovelomanana-Vidal, HNTf2-Catalyzed Regioselective Preparation of Polysubstituted Naphthalene Derivatives Through Alkyne–Aldehyde Coupling, J. Org. Chem., 2015, 80, 3250–3257 CrossRef CAS PubMed;
(d) V. Gudla, M. Sudheer, C. J. Rao, P. D. Sanasi and V. R. Battula, Triflic acid catalysed regioselective synthesis of substituted naphthalenes by benzannulation of carbonyls with alkynes, Tetrahedron, 2021, 90, 132214 CrossRef CAS;
(e) A. K. Sahu, R. Unnava, B. K. Behera and A. K. Saikia, Synthesis of dibenzocyclohepta[1,2-a]naphthalene derivatives from phenylacetaldehyde and alkynyl benzyl alcohols via sequential electrophilic addition and double Friedel-Crafts reactions, Org. Biomol. Chem., 2021, 19, 2430–2435 RSC.
-
(a) R. J. Hinkle and S. E. Lewis, Atom Economical, One-Pot, Three-Reaction Cascade to Novel Tricyclic 2,4-Dihydro-1H-benzo[f]isochromenes, Org. Lett., 2013, 15, 4070–4073 CrossRef CAS PubMed;
(b) R. J. Hinkle, Y. Chen, C. P. Nofi and S. E. Lewis, Electronic effects on a one-pot aromatization cascade involving alkynyl-Prins cyclization, Friedel–Crafts alkylation and dehydration to tricyclic benzo[f]isochromenes, Org. Biomol. Chem., 2017, 15, 7584–7593 RSC;
(c) R. J. Hinkle, D. J. Speer, B. B. Carnell, B. L. Kanter and R. D. Pike, Mild, Modular, and Convergent Synthesis of Helical Naphtho[2,1-c]chromenes via a Multistep Cyclization/Aromatization Cascade Sequence, J. Org. Chem., 2019, 84, 15633–15641 CrossRef CAS PubMed.
-
(a) V. D. Vuković, E. Richmond, E. Wolf and J. Moran, Catalytic Friedel–Crafts Reactions of Highly Electronically Deactivated Benzylic Alcohols, Angew. Chem., Int. Ed., 2017, 56, 3085–3089 CrossRef PubMed;
(b) F. Noël, V. D. Vuković, J. Yi, E. Richmond, P. Kravljanac and J. Moran, Catalytic Synthesis of Trifluoromethylated Allenes, Indenes, Chromenes, and Olefins from Propargylic Alcohols in HFIP, J. Org. Chem., 2019, 84, 15926–15947 CrossRef;
(c) I. Colomer, A. E. R. Chamberlain, M. B. Haughey and T. J. Donohoe, Hexafluoroisopropanol as a highly versatile solvent, Nat. Rev. Chem., 2017, 1, 1–12 CrossRef;
(d) H. F. Motiwala, A. M. Armaly, J. G. Cacioppo, T. C. Coombs, K. R. K. Koehn, V. M. Norwood and J. Aubé, HFIP in Organic Synthesis, Chem. Rev., 2022, 122, 12544–12747 CrossRef CAS PubMed.
- Subjecting the aldehyde to the reaction conditions (in the absence of alcohol 9) leads to decomposition of the aldehyde.
- Stereochemical assignment in 14i and 14j is tentative, based on aldehyde behavior and x-ray structure for 14a.
-
(a) M. A. Corsello, J. Kim and N. K. Garg, Indole diterpenoid natural products as the inspiration for new synthetic methods and strategies, Chem. Sci., 2017, 8, 5836–5844 RSC;
(b) W. Gul and M. T. Hamann, Indole alkaloid marine natural products: An established source of cancer drug leads with considerable promise for the control of parasitic, neurological and other diseases, Life Sci., 2005, 78, 442–453 CrossRef CAS PubMed;
(c) J. Vaca, F. Salazar, A. Ortiz and E. Sansinenea, Indole alkaloid derivatives as building blocks of natural products from Bacillus thuringiensis and Bacillus velezensis and their antibacterial and antifungal activity study, J. Antibiot., 2020, 73, 798–802 CrossRef CAS PubMed.
- M. D. Heredia, W. D. Guerra, S. M. Barolo, S. J. Fornasier, R. A. Rossi and M. E. Budén, Transition-Metal-Free and Visible-Light-Mediated Desulfonylation and Dehalogenation Reactions: Hantzsch Ester Anion as Electron and Hydrogen Atom Donor, J. Org. Chem., 2020, 85, 13481–13494 CrossRef CAS PubMed.
- R. J. Lundgren, B. D. Peters, P. G. Alsabeh and M. A. P. Stradiotto, N-Ligand for Palladium-Catalyzed Ammonia Arylation: Coupling of Deactivated Aryl Chlorides, Chemoselective Arylations, and Room Temperature Reactions, Angew. Chem., Int. Ed., 2010, 49, 4071–4074 CrossRef CAS PubMed.
- A. Zúñiga, M. Pérez, G. Pazos, G. Gómez and Y. Fall, A Concise, Enantioselective Synthesis of (+)-Decarestrictine L from Tri-O-acetyl-d-glucal, Synthesis, 2010, 2446–2450 Search PubMed.
- F. Himo, T. Lovell, R. Hilgraf, V. V. Rostovtsev, L. Noodleman, K. B. Sharpless and V. V. Fokin, Copper(I)-Catalyzed Synthesis of Azoles. DFT Study Predicts Unprecedented Reactivity and Intermediates, J. Am. Chem. Soc., 2005, 127, 210–216 CrossRef CAS PubMed.
- A PubChem search of the general scaffold reported yields no similar structures at a 95% Tanimoto threshold. At 90% Tanimoto threshold 180 hits are observed, all of which lack the tricyclic core (most of them are not even bicyclic). There is only one report where a similar tricycle is observed as a minor byproduct (Chem. Commun., 2015, 51, 10318–10321.).
|
This journal is © The Royal Society of Chemistry 2022 |
Click here to see how this site uses Cookies. View our privacy policy here.