DOI:
10.1039/D2SC04229K
(Edge Article)
Chem. Sci., 2022,
13, 13893-13897
InI3-catalyzed polyene cyclization of allenes and its application in the total synthesis of seven abietane-type diterpenoids†
Received
29th July 2022
, Accepted 11th October 2022
First published on 2nd November 2022
Abstract
A novel polyene cyclization using the allene group as the initiator has been successfully developed. This methodology provides an efficient strategy for the construction of an abietane-type tricyclic skeleton with a functionalizable C2–C3 double bond and features a wide substrate scope and excellent stereoselectivities. Potential utility of this approach has been well demonstrated by the collective total synthesis of seven abietane-type diterpenoids. Specifically, (±)-2,3-dihydroxyferruginol and (±)-2,3-dihydroxy-15,16-dinor-ent-pimar-8,11,13-triene were synthesized for the first time.
Introduction
Polyene cyclization, as one of the most powerful synthetic strategies, has been widely applied to the synthesis of complex polycyclic natural products and bioactive molecules.1 Since the elegant strategy was first successfully introduced for synthesizing diterpenes, many synthetic chemists have strived to explore more convenient and general synthetic approaches toward biologically active polycyclic terpenes2 or the key 6/6/6 tricyclic skeleton.3 During the process, many functional groups have been used as initiators to trigger this kind of cyclization procedure, such as alkene4 or alkyne,5 epoxide,6 allylic alcohol,7 propargylic ester8 and carbonyl-related groups.9 Nevertheless, it is still highly desirable to develop new activation patterns that can spontaneously introduce functional groups on the resulting polycyclic products to facilitate subsequent synthetic manipulations, in particular, the synthesis of corresponding bioactive molecules (Fig. 1).
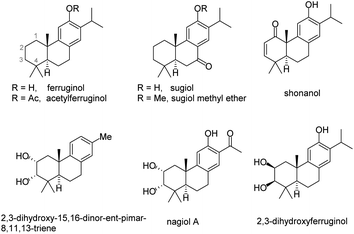 |
| Fig. 1 Selected abietane-type diterpenoids. | |
Based on the pioneering work of polyene cyclization and in connection with our research interests in the development of efficient synthetic methodologies toward the synthesis of bioactive molecules, an Au(I)-catalyzed polyene cyclization strategy using propargylic ester as the initiator was developed by our group recently. In this procedure, an enol ester moiety at the C2–C3 site of the key 6/6/6 tricyclic skeleton can be readily installed and used as a functional handle for subsequent synthetic transformations (Scheme 1a), which has been shown through the divergent synthesis of five abietane-type diterpenoids encouraging us to carry out further investigation in this field.8 Over the past decades, the allene group, as a typical versatile synthon, has attracted broad attention from chemists.10 In particular, it has been applied to a series of cyclization modes to construct various ring systems with an alkene moiety.11 Inspired by these results, we envisioned that it should be viable to develop a new polyene cyclization mode using the allene functional group as the initiator to afford a 6/6/6 tricyclic cyclization product with a double bond at the C2–C3 position (Scheme 1b). Since the double bond can be readily transformed to structural moieties like vicinal diol or enone, realization of such a methodology should lead to a new synthetic strategy toward the collective total synthesis of some biological abietane-type diterpenoids with substituents at both C2 and C3-positions, such as (±)-shonanol, (±)-2,3-dihydroxy-15,16-dinor-ent-pimar-8,11,13-triene, and (±)-2,3-dihydroxyferruginol (Fig. 1). Herein, we present an InI3-catalyzed polyene cyclization of allenes and its application to the total synthesis of seven abietane-type diterpenoids.
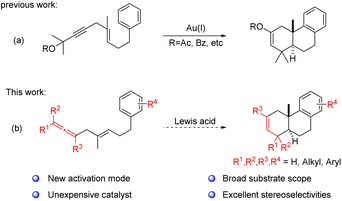 |
| Scheme 1 Background information and the synthetic strategy. | |
Results and discussion
Initially, we attempted a model reaction of 1a with various Lewis acids (see the ESI†). Fortunately, the desired product 2a was obtained in 10% yield with In(OTf)3 (Table 1, entry 1), whereas other strong Lewis acids like Sn(OTf)2 and Zn(OTf)2 did not give product 2a (Table 1, entries 2–3). Therefore, indium catalysts with different anions such as InCl3, InBr3, InI3 were further screened. Fortunately, the use of InI3 led to the best yield of 63% (Table 1, entry 6). Subsequently, several solvents were also tested, and CH2Cl2 was still the best choice after many trials, while other solvents were not efficient enough (Table 1, entries 7–12). Furthermore, examining different temperatures ranging from −60 °C to 0 °C revealed the best yield of 71% at −40 °C (Table 1, entries 13–16). Finally, the reaction was conducted in CH2Cl2 at −40 °C (Table 1, entry 15).
Table 1 Reaction condition optimizationa
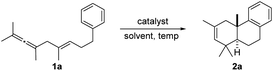
|
Entry
|
Cat. |
Equiv. |
Sol. |
T (°C) |
t/h |
Yield (%)b |
Unless specified, all reactions were carried out with 1a (0.1 mmol, 1.0 equiv.) and solvent (2 mL) in reaction tubes.
Isolated yield of product 2a.
NR = no Reaction.
|
1 |
In(OTf)3 |
0.2 |
DCM |
r.t. |
18 |
10 |
2 |
Sn(OTf)2 |
0.2 |
DCM |
0 |
1 |
NRc |
3 |
Zn(OTf)2 |
0.2 |
DCM |
r.t. |
120 |
NRc |
4 |
InCl3 |
0.2 |
DCM |
r.t. |
1 |
40 |
5 |
InBr3 |
0.2 |
DCM |
r.t. |
1 |
40 |
6 |
InI3 |
0.2 |
DCM |
−20 |
4 |
63 |
7 |
InI3 |
0.2 |
CH3OH |
r.t. |
120 |
NRc |
8 |
InI3 |
0.2 |
DMF |
r.t. |
120 |
NRc |
9 |
InI3 |
0.2 |
DCE |
−20 |
3 |
45 |
10 |
InI3 |
0.2 |
Toluene |
−20 |
3 |
20 |
11 |
InI3 |
0.2 |
CH3CN |
r.t. |
120 |
NRc |
12 |
InI3 |
0.2 |
THF |
r.t. |
120 |
NRc |
13 |
InI3 |
0.2 |
DCM |
r.t. |
0.5 |
45 |
14 |
InI3 |
0.2 |
DCM |
0 |
1.5 |
54 |
15
|
InI
3
|
0.2
|
DCM
|
−40
|
6
|
71
|
16 |
InI3 |
0.2 |
DCM |
−60 |
27 |
63 |
With the optimal reaction conditions in hand, the substrate scope of this reaction was then investigated. As shown in Scheme 2, switching the gem-dimethyl moiety to cyclopentane and cyclohexane rings was feasible, giving the desired products 2b and 2c in 45% and 41% yields, respectively. The slightly decreased yields might be attributed to the steric hindrance of these moieties. Notably, a mono phenyl substituted substrate 1d could deliver 2d smoothly in 50% yield with excellent diastereoselectivity, and the relative configuration of 2d was further determined by X-ray crystal diffraction. Next, changing the R3 group of substrates from methyl to o-methylphenyl and p-methylphenyl was successful to give corresponding products 2e and 2f in moderate yields of 40% and 50% at 0 °C and room temperature, respectively. Subsequently, it was observed that bromo substituted substrate 1g was amenable to this reaction, albeit giving 2g with only 34% yield. Subsequently, substrates with electro-donating groups such as methyl, methoxy, tert-butyl and phenyl groups as R4 at different positions on the phenyl ring were also investigated. All these substrates could produce the expected products in moderate to good yields (2h–2n). Generally, electron-donating substituents were beneficial for better yields of corresponding products (2gvs.2h–2n). Consistent with the above observation, electron-donating substituents like TBS ether on the phenyl ring and the use of the naphthalene ring could also lead to the desired products 2o and 2p in good yields of 81% and 70%, respectively. It is worth mentioning that substrate 1r with two electron-donating substituents gave the best yield of 95%, whereas 1q bearing both electron-rich and electron-deficient substituents produced 2q in only 44% yield. Moreover, the electronic effect was also shown by comparison between 2r and 2t (40% vs. 83%). To further demonstrate the potential practicality of this reaction, a 2 mmol scale reaction of 1s was also conducted, and an excellent yield of 91% yield was obtained. Additionally, substrate 1u with a (Z)-1,4-enallene moiety was also tested under the standard conditions, and an inseparable mixture of 2a and 2u was obtained in 50% total yield and 1
:
1 diasteromeric ratio (trans/cis).12,4h,5e
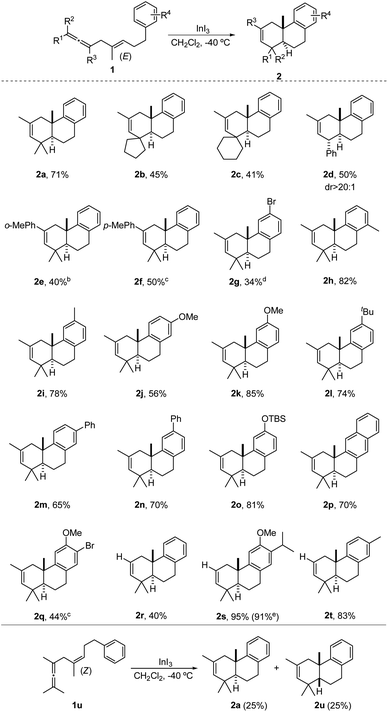 |
| Scheme 2 Substrate scope investigation of the reactiona. aUnless specified, all reactions were carried out with 1 (0.1 mmol, 1.0 equiv.), InI3 (20 mol%) and CH2Cl2 (2 mL) in reaction tubes at −40 °C for 4 h. bReaction temperature was 0 °C. cReaction temperature was 25 °C. dReaction temperature was −20 °C. eYield obtained in a 2 mmol scale reaction. | |
To show the synthetic utility of the reaction, a collective total synthesis of seven abietane-type diterpenes, i.e., (±)-2,3-dihydroxyferruginol,13 (±)-shonanol,14 (±)-2,3-dihydroxy-15,16-dinor-ent-pimar-8,11,13-triene,15 (±)-ferruginol,16 (±)-sugiol methyl ether,17 (±)-sugiol,18 and (±)-acetylferruginol,19 was then conducted from compounds 2s and 2t. As shown in Scheme 3, demethylation of 2s delivered 3 in 85% yield in the presence of NaSEt, and (±)-2,3-dihydroxyferruginol was facilely obtained through dihydroxylation of 3 in 50% yield along with its epimer 7. The relative configuration of (±)-2,3-dihydroxyferruginol was confirmed by X-ray diffraction (see the ESI†). In another successful example, selective oxidization of 2s with SeO2 and further oxidization could give ketone 4 in 60% yield using DMP as the oxidant. Subsequently, the synthesis of (±)-shonanol was readily finished after simple demethylation, and the relative configuration was also confirmed by X-ray diffraction (see the ESI†). Other syntheses of abietane-type diterpenoids, such as (±)-ferruginol, (±)-sugiol methyl ether, (±)-sugiol, and (±)-acetylferruginol are also exhibited in Scheme 3 starting from a common advanced intermediate 5, which was easily prepared through hydrogenation of 2s in 81% yield. Next, (±)-ferruginol was obtained by demethylation of 5 in 90% yield in the presence of BBr3. Acylation of (±)-ferruginol gave (±)-acetylferruginol in 95% yield. Alternatively, compound 5 could be oxidized with CrO3 to give (±)-sugiol methyl ether in 83% yield, and demethylation of (±)-sugiol methyl ether with NaSEt afforded (±)-sugiol. Additionally, another natural product (±)-2,3-dihydroxy-15,16-dinor-ent-pimar-8,11,13-triene and its epimer 6 were also rapidly prepared in 71% total yield through a dihydroxylation of compound 2t. These synthetic applications well exhibit the utility of our newly developed polyene cyclization mode, in particular the usefulness of the retained C2–C3 double bond for further functionalization.
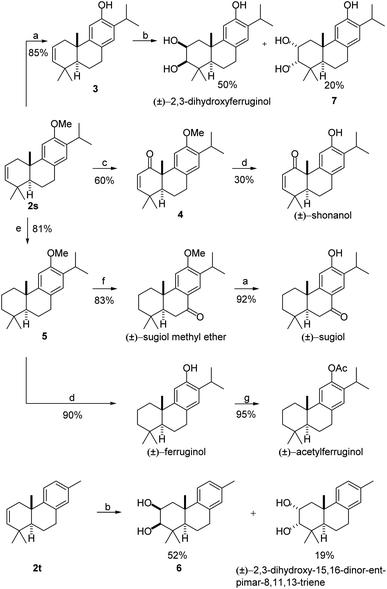 |
| Scheme 3 Synthetic applications. (a) NaSEt, DMF, 140 °C; (b) OsO4, NMO, citric acid, t-BuOH/acetone/H2O = 1 : 1 : 1; (c) SeO2, 1,4-dioxane, 100 °C, then DMP, DCM, r.t.; (d) BBr3, DCM, −20 °C; (e) H2, Pd/C, CH3OH, r.t.; (f) CrO3, AcOH, r.t.; (g) Ac2O, Et3N, DMAP, DCM, r.t. | |
Conclusions
In conclusion, a new mode of polyene cyclization initiated by the allene moiety has been successfully realized under the catalysis of InI3, and the retained C2–C3 double bond provides the very crucial reaction site for further transformations, in particular, towards the synthesis of corresponding abietane diterpenes, such as (±)-2,3-dihydroxyferruginol, (±)-shonanol and (±)-2,3-dihydroxy-15,16-dinor-ent-pimar-8,11,13-triene. In addition, other diterpenes like (±)-ferruginol, (±)-sugiol methyl ether, (±)-sugiol, and (±)-acetylferruginol have also been collectively synthesized after simple operations. Currently, biological activity tests of these abietane diterpenes and their analogues along with the synthesis of other bioactive polycyclic terpenes using the same strategy are ongoing in the same laboratory.
Data availability
Experimental data has been uploaded as part of the ESI.†
Author contributions
SHW and DYZ directed this project. CYH, TLZ, WHD, ZHZ and JDW performed the experiments. XMZ, XTX, DYZ and SHW prepared the draft and SHW revised the manuscript.
Conflicts of interest
There are no conflicts to declare.
Acknowledgements
This project was supported by the National Natural Science Foundation of China (21772071), the Science and Technology Program of Gansu Province (20JR10RA608), the Department of Education of Guangdong Province (No. 2019KZDXM035, 2021KCXTD044, and 2021KTSCX135), the Fundamental Research Funds for the Central Universities (lzujbky-2022-ct03, lzujbky-2022-sp09), the Collaborative Innovation Center for Northwestern Chinese Medicine of Lanzhou University and the Drug Research Project of Gansu Province (No.2022GSMPA0010).
References
-
(a) J. Justicia, L. Alvarez de Cienfuegos, A. G. Campana, D. Miguel, V. Jakoby, A. Gansauer and J. M. Cuerva, Chem. Soc. Rev., 2011, 40, 3525–3537 RSC;
(b)
S. A. Snyder and A. M. Levinson, in Comprehensive Organic Synthesis, ed. P. Knochel, Elsevier, Amsterdam, 2nd edn, 2014, pp. 268–292, DOI:10.1016/B978-0-08-097742-3.00309-8;
(c) C. N. Ungarean, E. H. Southgate and D. Sarlah, Org. Biomol. Chem., 2016, 14, 5454–5467 RSC;
(d) A. Barrett, T.-K. Ma and T. Mies, Synthesis, 2019, 51, 67–82 CrossRef CAS;
(e) A. D'Hollander, L. Peilleron, T. Grayfer and K. Cariou, Synthesis, 2019, 51, 1753–1769 CrossRef;
(f) B. Maji, Adv. Synth. Catal., 2019, 361, 3453–3489 CrossRef CAS;
(g) J. M. Feilner, F. L. Haut and T. Magauer, Chem.—Eur. J., 2021, 27, 7017–7021 CrossRef CAS PubMed;
(h) X.-S. Peng, H. N. C. Wong, Z. Zhong, M.-Y. Lyu and H.-R. Ma, Synlett, 2021, 32, 1796–1815 CrossRef;
(i) G. Alachouzos and A. J. Frontier, Isr. J. Chem., 2021, 61, 469–485 CrossRef CAS.
-
(a) M. A. Gonzalez, Eur. J. Med. Chem., 2014, 87, 834–842 CrossRef CAS PubMed;
(b) M. A. Gonzalez, Nat. Prod. Rep., 2015, 32, 684–704 RSC;
(c) M. A. González, Tetrahedron, 2015, 71, 1883–1908 CrossRef.
-
(a) A. M. Gonzalez, Curr. Bioact. Compd., 2007, 3, 1–36 CrossRef;
(b) R. J. Peters, Nat. Prod. Rep., 2010, 27, 1521–1530 RSC;
(c) A. P. Wardana, N. S. Aminah, M. Rosyda, M. I. Abdjan, A. N. Kristanti, K. N. W. Tun, M. I. Choudhary and Y. Takaya, Heliyon, 2021, 7, e07777 CrossRef CAS PubMed.
-
(a) K. Ishihara, H. Ishibashi and H. Yamamoto, J. Am. Chem. Soc., 2001, 123, 1505–1506 CrossRef CAS;
(b) K. Ishihara, H. Ishibashi and H. Yamamoto, J. Am. Chem. Soc., 2002, 124, 3647–3655 CrossRef CAS PubMed;
(c) H. Ishibashi, K. Ishihara and H. Yamamoto, J. Am. Chem. Soc., 2004, 126, 11122–11123 CrossRef CAS PubMed;
(d) A. Sakakura, A. Ukai and K. Ishihara, Nature, 2007, 445, 900–903 CrossRef CAS PubMed;
(e) Y.-J. Zhao, S.-S. Chng and T.-P. Loh, J. Am. Chem. Soc., 2007, 129, 492–493 CrossRef CAS PubMed;
(f) Y.-J. Zhao and T.-P. Loh, J. Am. Chem. Soc., 2008, 130, 10024–10029 CrossRef CAS PubMed;
(g) S. A. Snyder and D. S. Treitler, Angew. Chem., Int. Ed., 2009, 48, 7899–7903 CrossRef CAS PubMed;
(h) S. A. Snyder, D. S. Treitler and A. P. Brucks, J. Am. Chem. Soc., 2010, 132, 14303–14314 CrossRef CAS PubMed;
(i) K. Surendra and E. J. Corey, J. Am. Chem. Soc., 2012, 134, 11992–11994 CrossRef CAS PubMed;
(j) Y. Sawamura, H. Nakatsuji, A. Sakakura and K. Ishihara, Chem. Sci., 2013, 4, 4181–4186 RSC;
(k) R. C. Samanta and H. Yamamoto, J. Am. Chem. Soc., 2017, 139, 1460–1463 CrossRef CAS PubMed;
(l) D. Vrubliauskas and C. D. Vanderwal, Angew. Chem., Int. Ed., 2020, 59, 6115–6121 CrossRef CAS PubMed.
-
(a) H. Imagawa, T. Iyenaga and M. Nishizawa, Org. Lett., 2005, 7, 451–453 CrossRef CAS PubMed;
(b) W.-W. Qiu, K. Surendra, L. Yin and E. J. Corey, Org. Lett., 2011, 13, 5893–5895 CrossRef CAS PubMed;
(c) K. Surendra, W. Qiu and E. J. Corey, J. Am. Chem. Soc., 2011, 133, 9724–9726 CrossRef CAS PubMed;
(d) K. Surendra and E. J. Corey, J. Am. Chem. Soc., 2014, 136, 10918–10920 CrossRef CAS PubMed;
(e) J. Yao, C.-L. Li, X. Fan, Z. Wang, Z.-X. Yu and J. Zhao, CCS Chem., 2021, 3, 3133–3143 Search PubMed.
-
(a) S. P. Morcillo, D. Miguel, S. Resa, A. Martin-Lasanta, A. Millan, D. Choquesillo-Lazarte, J. M. Garcia-Ruiz, A. J. Mota, J. Justicia and J. M. Cuerva, J. Am. Chem. Soc., 2014, 136, 6943–6951 CrossRef CAS PubMed;
(b) G. Rajendar and E. J. Corey, J. Am. Chem. Soc., 2015, 137, 5837–5844 CrossRef CAS PubMed;
(c) Y. Tian, X. Xu, L. Zhang and J. Qu, Org. Lett., 2016, 18, 268–271 CrossRef CAS PubMed.
-
(a) M. A. Schafroth, D. Sarlah, S. Krautwald and E. M. Carreira, J. Am. Chem. Soc., 2012, 134, 20276–20278 CrossRef CAS PubMed;
(b) F. Z. Zhang, Y. Tian, G. X. Li and J. Qu, J. Org. Chem., 2015, 80, 1107–1115 CrossRef CAS PubMed.
- T.-L. Zheng, S.-Z. Liu, C.-Y. Huo, J. Li, B.-W. Wang, D.-P. Jin, F. Cheng, X.-M. Chen, X.-M. Zhang, X.-T. Xu and S.-H. Wang, CCS Chem., 2020, 2, 2795–2802 Search PubMed.
-
(a) M. Uyanik, K. Ishihara and H. Yamamoto, Org. Lett., 2006, 8, 5649–5652 CrossRef CAS PubMed;
(b) S. Rendler and D. W. C. MacMillan, J. Am. Chem. Soc., 2010, 132, 5027–5029 CrossRef CAS PubMed;
(c) Y.-J. Zhao, B. Li, L.-J. S. Tan, Z.-L. Shen and T.-P. Loh, J. Am. Chem. Soc., 2010, 132, 10242–10244 CrossRef CAS PubMed;
(d) B. Li, Y. C. Lai, Y. Zhao, Y. H. Wong, Z. L. Shen and T. P. Loh, Angew. Chem., Int. Ed., 2012, 51, 10619–10623 CrossRef CAS PubMed;
(e) L. Fan, C. Han, X. Li, J. Yao, Z. Wang, C. Yao, W. Chen, T. Wang and J. Zhao, Angew. Chem., Int. Ed., 2018, 57, 2115–2119 CrossRef CAS PubMed.
-
(a) S. Ma, Chem. Rev., 2005, 105, 2829–2871 CrossRef PubMed;
(b) S. Ma, Acc. Chem. Res., 2009, 42, 1679–1688 CrossRef CAS PubMed;
(c) T. Caneque, F. M. Truscott, R. Rodriguez, G. Maestri and M. Malacria, Chem. Soc. Rev., 2014, 43, 2916–2926 RSC;
(d) J. Ye and S. Ma, Acc. Chem. Res., 2014, 47, 989–1000 CrossRef CAS PubMed;
(e) X. Huang and S. Ma, Acc. Chem. Res., 2019, 52, 1301–1312 CrossRef CAS PubMed.
-
(a) S. Ma, Acc. Chem. Res., 2003, 36, 701–712 CrossRef CAS PubMed;
(b) B. Alcaide, P. Almendros and T. Martinez del Campo, Angew. Chem., Int. Ed., 2007, 46, 6684–6687 CrossRef CAS PubMed;
(c) F. Zhao and T. P. Loh, Angew. Chem., Int. Ed., 2009, 48, 7232–7235 CrossRef PubMed;
(d) W. Li, W. Yuan, M. Shi, E. Hernandez and G. Li, Org. Lett., 2010, 12, 64–67 CrossRef CAS PubMed;
(e) B. L. Lu and M. Shi, Angew. Chem., Int. Ed., 2011, 50, 12027–12031 CrossRef CAS PubMed;
(f) B. Bolte and F. Gagosz, J. Am. Chem. Soc., 2011, 133, 7696–7699 CrossRef CAS PubMed;
(g) S. Yu and S. Ma, Angew. Chem., Int. Ed., 2012, 51, 3074–3112 CrossRef CAS PubMed;
(h) B. Alcaide, P. Almendros, S. Cembellin, T. Martinez del Campo and I. Fernandez, Chem. Commun., 2013, 49, 1282–1284 RSC;
(i) J. Ye and S. Ma, Org. Chem. Front., 2014, 1, 1210–1224 RSC;
(j) D. Y. Li, Y. Wei, I. Marek, X. Y. Tang and M. Shi, Chem. Sci., 2015, 6, 5519–5525 RSC;
(k) C. Ji, Q. Xu and M. Shi, Adv. Synth. Catal., 2017, 359, 974–983 CrossRef CAS;
(l) L.-P. Zhao, P.-J. Li, L. Wang and Y. Tang, Angew. Chem., Int. Ed., 2022, e202207360 CAS.
-
(a) G. Stork and A. W. Burgstahler, J. Am. Chem. Soc., 1955, 77, 5068–5077 CrossRef CAS;
(b) K. Ishihara, S. Nakamura and H. Yamamoto, J. Am. Chem. Soc., 1999, 121, 4906–4907 CrossRef CAS;
(c) S. Nakamura, K. Ishihara and H. Yamamoto, J. Am. Chem. Soc., 2000, 122, 8131–8140 CrossRef CAS;
(d) P. Alonso, P. Pardo, R. Fontaneda, F. J. Fananas and F. Rodriguez, Chem.—Eur. J., 2017, 23, 13158–13163 CrossRef CAS PubMed;
(e) S. J. Plamondon, J. M. Warnica, D. Kaldre and J. L. Gleason, Angew. Chem., Int. Ed., 2020, 59, 253–258 CrossRef CAS PubMed;
(f) S. J. Plamondon and J. L. Gleason, Org. Lett., 2022, 24, 2305–2309 CrossRef CAS PubMed.
-
(a) R. E. Cox, S. Yamamoto, A. Otto and B. R. T. Simoneit, Biochem. Syst. Ecol., 2007, 35, 342–362 CrossRef CAS;
(b) H. Zhao, H. Li, G. Huang and Y. Chen, Nat. Prod. Rep., 2017, 31, 844–848 CrossRef CAS PubMed.
-
(a) Y.-T. Lin and K.-T. Liu, J. Chin. Chem. Soc., 1965, 12, 51–60 CrossRef CAS;
(b) T. Matsumoto, S. Imai and S. Yuki, Bull. Chem. Soc. Jpn., 1981, 54, 1448–1455 CrossRef CAS;
(c) J.-M. Fang, S.-T. Jan and Y.-S. Cheng, Phytochemistry, 1987, 26, 853–854 CrossRef CAS.
- H. Li, J. J. Zhao, J. L. Chen, L. P. Zhu, D. M. Wang, L. Jiang, D. P. Yang and Z. M. Zhao, Phytochemistry, 2015, 117, 400–409 CrossRef CAS PubMed.
-
(a) W. P. Campbell and D. Todd, J. Am. Chem. Soc., 1942, 64, 928–935 CrossRef CAS;
(b) C.-C. Wen, Y.-H. Kuo, J.-T. Jan, P.-H. Liang, S.-Y. Wang, H.-G. Liu, C.-K. Lee, S.-T. Chang, C.-J. Kuo, S.-S. Lee, C.-C. Hou, P.-W. Hsiao, S.-C. Chien, L.-F. Shyur and N.-S. Yang, J. Med. Chem., 2007, 50, 4087–4095 CrossRef CAS PubMed;
(c) Z. Tao, K. A. Robb, K. Zhao and S. E. Denmark, J. Am. Chem. Soc., 2018, 140, 3569–3573 CrossRef CAS PubMed;
(d) A. R. Jassbi, H. Hadavand Mirzaei, O. Firuzi, S. Pirhadi, M. Asadollahi, J. N. Chandran and B. Schneider, Nat. Prod. Rep., 2022, 36, 3183–3188 CrossRef CAS PubMed.
-
(a) P. Sengupta, S. N. Choudhuri and H. N. Khastgir, Tetrahedron, 1960, 10, 45–54 CrossRef CAS;
(b) Z. Yang, Y. Kitano, K. Chiba, N. Shibata, H. Kurokawa, Y. Doi, Y. Arakawa and M. Tada, Bioorg. Med. Chem., 2001, 9, 347–356 CrossRef CAS PubMed.
-
(a) E. Wenkert, J. de Paiva Campello, J. D. McChesney and D. J. Watts, Phytochemistry, 1974, 13, 2545–2549 CrossRef CAS;
(b) I. Cordova, L. G. Leon, F. Leon, L. San Andres, J. G. Luis and J. M. Padron, Eur. J. Med. Chem., 2006, 41, 1327–1332 CrossRef CAS PubMed.
-
(a) H. Akita and T. Oishi, Chem. Pharm. Bull., 1981, 29, 1567–1579 CrossRef CAS;
(b) C. Thommen, C. K. Jana, M. Neuburger and K. Gademann, Org. Lett., 2013, 15, 1390–1393 CrossRef CAS PubMed.
|
This journal is © The Royal Society of Chemistry 2022 |