DOI:
10.1039/D2SC01834A
(Edge Article)
Chem. Sci., 2022,
13, 8686-8692
Pd(II)-catalyzed meta-C–H bromination and chlorination of aniline and benzoic acid derivatives†
Received
31st March 2022
, Accepted 4th July 2022
First published on 5th July 2022
Abstract
The classic electrophilic bromination leads to ortho- and para-bromination of anilines due to their electron-rich properties. Herein we report the development of an unprecedented Pd-catalyzed meta-C–H bromination of aniline derivatives using commercially available N-bromophthalimide (NBP), which overcomes the competing ortho/para-selectivity of electrophilic bromination of anilines. The addition of acid additives is crucial for the success of this reaction. A broad range of substrates with various substitution patterns can be tolerated in this reaction. Moreover, benzoic acid derivatives bearing complex substitution patterns are also viable with this mild bromination reaction, and meta-C–H chlorination is also feasible under similar reaction conditions. The ease of the directing group removal and subsequent diverse transformations of the brominated products demonstrate the application potential of this method and promise new opportunities for drug discovery.
Introduction
Aromatic bromides represent a class of highly important synthetic precursors in organic synthesis,1 especially in their use for powerful cross-coupling reactions,2 which has led to the pursuit of numerous methods for their synthesis.1a–c Moreover, many brominated aromatic derivatives, especially those of anilines, are often found as target molecules in pharmaceuticals (Scheme 1a), as well as in functional molecules and natural products.1a,d Classic methods for synthesis of brominated arenes, such as the Friedel–Crafts-type electrophilic bromination, often suffer from poor regioselectivity, harsh reaction conditions, long reaction time, tedious reaction procedures, or limited substrate scope.1a,3 Moreover, although the fundamental reactivity pattern of anilines can provide predictable selectivity according to the electron-density distribution in the substrate and steric properties of the substituents in simple cases, it is difficult to access isomers that cannot be anticipated based on their fundamental reactivity. In addition, when there is more than one substituent with varied electronic or steric properties, the competition between these substituents in such complex systems may lead to a mixture of products that are difficult to isolate. Consequently, direct C–H bromination of anilines that overcomes the inherent site-selectivity, which is also applicable for anilines with multiple substituents, is extremely useful for producing valuable complex substituted aniline derivatives in a straightforward and unconventional manner.
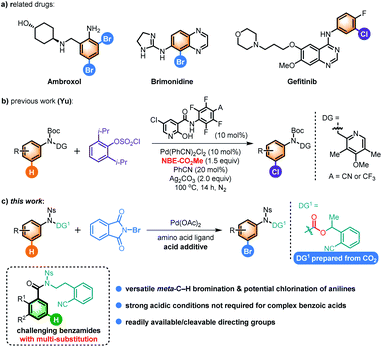 |
| Scheme 1 Direct meta-C–H halogenation of anilines and benzoic acids. | |
Recently, transition metal-catalyzed C–H activation has emerged as one of the most promising methods for the synthesis of ortho- and para-C–H halogenated anilines.1b,c,4,5 Notably, very limited, though elegant, protocols for producing meta-C–H halogenated arenes have also been developed, mainly through the formation of cycloruthenated complex intermediates by Greaney,6a Huang,6b Ackermann,6c,f and others,6 as well as by using a pyridyl-based meta-directing group (DG) with a Pd-catalyst by Yu7a,b and Dai7c groups. Recently, we have also developed meta-C–H iodination of hydrocinnamic acid via a formal metathesis reaction.8a However, the meta-C–H halogenation of anilines is still a formidable challenge since the conventional highly reactive sites are ortho/para positions. Although there have been several significant strategies disclosed for meta-C–H functionalization of arenes9–28 and a few of them have been applied for aniline derivatives by the groups of Gaunt,10 Yu,11 Ackermann,12 Phipps,13a Nakao,14 and others15 as well as our group,16 to date, only a single29 report on meta-C–H halogenation (i.e. chlorination) of aniline derivatives has been disclosed by the Yu group using a substituted pyridine as the DG for the initial ortho-C–H activation and modified norbornene as the mediator for subsequent meta-C–H chlorination (Scheme 1b).11b However, some substrates such as the simple aniline without a substituent did not work. Moreover, it is often observed in cross-coupling reactions that bromoarenes are more reactive than their chloroarene counterparts. Notably, the bromine atom generally forms stronger halogen bonding than chlorine, which is beneficial for drug discovery.1d Therefore, the development of a practical and complementary meta-C–H bromination reaction of anilines would be highly desirable.30
Herein, we disclose the first Pd(II)-catalyzed meta-C–H bromination of aniline derivatives using a readily cleavable nitrile-based DG, overcoming the competing ortho/para-selectivity of anilines (Scheme 1c). Moreover, this method can be modified to be compatible with benzoic acid derivatives18f,20b,28 bearing complex substitution patterns, whose bromination via conventional methods generally requires harsh reaction conditions such as the addition of strong acid or high reaction temperature. In addition, meta-C–H chlorination was also found to be feasible. Finally, diverse transformations of the brominated anilines demonstrate the versatility of our methods and promise new opportunities for drug discovery.1d,31
Results and discussion
Our investigation was initiated by treating carbamate 1a, which could be synthesized in one step from aniline, CO2 and 2-(1-bromoethyl)benzonitrile under basic conditions developed in our previous study on meta-C–H activation of anilines assisted by a nitrile-based DG,16 with various brominating agents using palladium acetate as the catalyst and N-protected amino acid (N-Ac–Gly–OH) as the ligands in HFIP at 90 °C under argon. Selected optimization results are summarized in Table 1. To our delight, the reactions with commercially available N-bromosuccinimide (NBS, Br-1) or N-bromophthalimide (NBP, Br-2) led to meta-C–H brominated aniline 2a in 23% and 28% combined yield of mono- and di-brominated anilines, respectively, with a trace of the para-C–H brominated aniline side product (entries 1 and 2), whereas 1,3-dibromo-5,5-dimethyl-imidazolidine-2,4-dione (Br-3) was ineffective and led to the formation of para-C–H brominated aniline (entry 3). To further improve the yield, acid additives such as acetic acid (HOAc), CF3COOH (TFA) and pivalic acid (PivOH) were utilized and could improve the results evidently (entries 4–6). We were then pleased to find that addition of half an equivalent of pentafluorobenzoic acid (A-1) increased the yield of 2a notably (entry 7). Tetrafluorobenzoic acid A-2 was also used, and the same result was obtained (entry 8). Much to our delight, significant improvements in the yield were observed when the loading of A-1 was increased gradually to five equivalents (entries 9–12). These low-cost electron-deficient polyfluorobenzoic acid additives might act as the activator of brominating reagents,1a while the costly silver carbonate activator was employed in the previous meta-C–H chlorination of aniline derivatives.11b It should be mentioned that polyfluorobenzoic acid might also act as a ligand to increase the electrophilicity and thus the reactivity of the Pd(II) catalyst.
Table 1 Optimization of reaction conditionsa

|
Entry |
Ligand |
Br+
|
Additive (equiv.) |
Yield [%] (mono/di) |
para-Br side product |
Reaction conditions: 1a (0.1 mmol), Pd(OAc)2 (10 mol%), ligand (60 mol%), Br+ (03 mmol), additive, HFIP (1 mL), 90 °C, 24 h, Ar. Yield was determined by 1H NMR with CH2Br2 as the internal standard.
Without Pd(OAc)2.
Isolated yield.
Br-2 (0.4 mmol), 100 °C, 48 h.
Br-2 (0.2 mmol).
12 h. N.D.: not detected.
|
1 |
N-Ac–Gly–OH |
Br-1
|
— |
23% (11/1) |
3% |
2 |
N-Ac–Gly–OH |
Br-2
|
— |
28% (8.3/1) |
3% |
3 |
N-Ac–Gly–OH |
Br-3
|
— |
— |
8% |
4 |
N-Ac–Gly–OH |
Br-2
|
HOAc (0.5) |
36% (6.2/1) |
4% |
5 |
N-Ac–Gly–OH |
Br-2
|
TFA (0.5) |
46% (2.8/1) |
3% |
6 |
N-Ac–Gly–OH |
Br-2
|
PivOH (0.5) |
40% (7.0/1) |
4% |
7 |
N-Ac–Gly–OH |
Br-2
|
A-1 (0.5) |
50% (3.1/1) |
2% |
8 |
N-Ac–Gly–OH |
Br-2
|
A-2 (0.5) |
50% (3.1/1) |
2% |
9 |
N-Ac–Gly–OH |
Br-2
|
A-1 (1) |
66% (3.7/1) |
2% |
10 |
N-Ac–Gly–OH |
Br-2
|
A-1 (1.5) |
70% (4.0/1) |
2% |
11 |
N-Ac–Gly–OH |
Br-2
|
A-1 (2) |
75% (3.4/1) |
3% |
12 |
N-Ac–Gly–OH |
Br-2
|
A-1 (5) |
89% (3.2/1) |
4% |
13 |
N-Ac–Gly–OH |
Br-2
|
A-1 (5) |
N.Db |
24% |
14 |
N-Ac–β-Ala–OH |
Br-2
|
A-1 (5) |
93% (2.2/1) |
4% |
15 |
N-TFA–Gly–OH |
Br-2
|
A-1 (5) |
94% (2.8/1) |
4% |
16 |
N-TFA–β-Ala–OH |
Br-2
|
A-1 (5) |
92% (2.4/1) |
4% |
17
|
N-Tf–β-Ala–OH
|
Br-2
|
A-1
(5)
|
93% (2.2/1)
|
3%
|
18
|
N-Tf–β-Ala–OH
|
Br-2
|
A-2
(5)
|
94% (1.8/1)
|
3% |
19 |
N-Tf–β-Ala–OH |
Br-2
|
A-2 (5) |
90% (1/1.1)c,d |
2% |
20 |
N-Tf–β-Ala–OH |
Br-2
|
A-1 (5) |
86% (4.1/1)c,e |
2% |
21 |
N-Tf–β-Ala–OH |
Br-2
|
A-1 (5) |
89% (4.6/1)c,f |
2% |
|
Notably, without the addition of Pd(OAc)2, not any meta-bromination products could be detected, whereas the para-bromination side product generated from the Friedel–Crafts-type electrophilic bromination was detected as the major product (entry 13). Moreover, only slight improvements in the desired products' overall yield were achieved by switching the N-protected amino acid ligands (entries 14–17). It should be mentioned that the use of 60% ligand is important for effective binding to the Pd center possibly due to the presence of excess of the acid additive. The optimal results that led to the highest catalytic turnover of the Pd catalyst were obtained using the N-Tf–β-Ala–OH ligand with A-2 as the additive (entry 18). In addition, the proportion of the di-brominated product could be improved by adding 4 equivalents of Br-2 and running the reaction for 48 hours at 100 °C (entry 19). Of note, the mono/di ratio could be increased greatly by either decreasing the loading of Br-2 (entry 20) or shortening the reaction time (entry 21), though the overall yields were slightly lower (see the ESI† for more reaction conditions).
With the optimized reaction conditions in hand, we examined the scope of this meta-selective C–H bromination of anilines with a variety of substrates (Table 2). Substrates with methyl and fluoro groups at the ortho-position were well tolerated (2b and 2c). The reactions also proceeded smoothly with various electron-donating and electron-withdrawing substituents at the meta-position (2d–2g). para-Substituted aniline carbamates bearing fluoro, chloro or bromo groups were also viable to deliver the desired bromination products in moderate to good yields (2h–2j). Notably, the method was also applicable for several di-substituted anilines, providing a straightforward and unconventional method to access highly substituted aniline derivatives (2k–2q). Importantly, the direct generation of several di- and tri-halogenated anilines could be very interesting for drug discovery such as by providing more halogen bonding possibility (2c, 2e, 2f, and 2h–2q).1d It should also be mentioned that the site-selectivity of the reaction was excellent, and other halogenation isomers were in trace amounts. Moreover, no C–H brominated products could be delivered for substituted anilines (1b–1q) under the optimized conditions without the Pd catalyst, except that substrate 1n gave 8% of the para-brominated product.
Table 2 The scope of meta-C–H bromination of aniline derivativesa
Reaction conditions: 1 (0.1 mmol), Pd(OAc)2 (0.01 mmol), N-Tf–β-Ala–OH (0.06 mmol), Br-2 (0.3 mmol), A-2 (0.5 mmol), HFIP (1 mL), 24 h, 90 °C, Ar. Isolated yields.
A-1 (0.5 mmol) instead of A-2.
N-TFA–β-Ala–OH (0.06 mmol) instead of N-Tf–β-Ala–OH.
HOAc (0.5 mmol) instead of A-2.
N-TFA–Gly–OH (0.06 mmol) instead of N-Tf–β-Ala–OH.
60 °C.
48 h.
100 °C.
Yield in the square brackets is that of the para-brominated product from the reaction without using Pd(OAc)2.
|
|
Following the successful bromination of aniline scaffolds, we moved on to investigate this method with benzoic acid derivatives attached with a nitrile-based DG that has been developed in our previous report on meta-C–H olefination of benzoic acids (Table 3).20b It should be noted that classic methods for bromination of benzoic acids often require harsh reaction conditions such as the addition of strong acid or high reaction temperature. After extensive modification of the reaction conditions (see ESI Table S1†), it was found that most of these benzoic acids afforded the best yields of the desired products with A-1 as the optimal acid additive and N-Ac–Gly–OH as the ligand. Notably, no/trace product was obtained without using Pd(OAc)2 for model substrate 3a and most of the substrates, indicating that a Friedel–Crafts type bromination could hardly occur under the mild conditions. It was observed that substrates bearing both methyl and halogen groups at the ortho- and para-positions were well tolerated (4b–4h). para-Halogenated benzoic acids were also viable to deliver the desired bromination products in good yields (4i–4k). Notably, this method was also suitable for meta-C–H brominating several challenging di-substituted benzoic acids (4l–4t), which may be useful for drug discovery. In these reactions, valuable products that may require several steps to synthesize by conventional methods were afforded in a straightforward and operationally simple manner. It should also be mentioned that the site-selectivity of this bromination was excellent, and only 9% of 4bmono and 55% of 4o could be produced without Pd(OAc)2, probably due to a higher tendency for 3b and 3o to undergo a Friedel–Crafts type reaction.
Table 3 The scope of meta-C–H bromination of benzoic acid derivativesa
Reaction conditions: 3 (0.1 mmol), Pd(OAc)2 (0.01 mmol), N-Ac–Gly–OH (0.06 mmol), Br-2 (0.3 mmol), A-1 (0.05 mmol), HFIP (1 mL), 24 h, 60 °C, Ar. Isolated yields. No/trace product without Pd(OAc)2, except 4b and 4o.
The yields in the square brackets are the 1H NMR yields of reactions without using Pd(OAc)2.
Chloranil (0.1 mmol) was added instead of A-1.
16 h.
Without adding A-1.
48 h.
N-Formyl–Gly–OH (0.06 mmol) instead of N-Ac–Gly–OH.
|
|
Since meta-chlorinated arenes are often found as important core structures in numerous drug molecules, we continued to explore the generality of chlorination of aniline and benzoic acid derivatives based on the optimized conditions for the above bromination reactions by using 1,3-dichloro-5,5-dimethylhydantoin (DCH) as the chlorinating reagent (Table 4), which led to limited scope of substrates with the current reaction conditions. The model aniline substrate afforded good overall yield of desired products (5a), and the mono-chlorinated product could be used as the substrate, for example, 2e in Table 2, demonstrating the possibility of sequential meta-chlorination and bromination. The ortho-substituted anilines gave the meta-chlorinated products in moderate yields (5b, 5c). Notably, di-substituted anilines were also viable in this reaction (5k and 5l). We also selected benzoic acids as the testing compounds, and the scope is slightly better. Besides the simple substrate (6a), both mono (6b) and several di-substituted substrates (6l–6q) were viable substrates, giving moderate to good yields of desired products. It should be noted that no desired chlorinated product could be obtained for these substrates without Pd(OAc)2. In addition, only low yield of iodinated products could be obtained with our aniline substrates using N-iodosuccinimide (NIS).
Table 4 The meta-C–H chlorination of aniline and benzoic acid derivativesa
Reaction conditions A: 1 (0.1 mmol), Pd(OAc)2 (0.01 mmol), N-TFA–β-Ala–OH (0.06 mmol), DCH (0.3 mmol), A-2 (0.5 mmol), HFIP (1 mL), 48 h, 110 °C, Ar. Isolated yields. Reaction conditions B: 3 (0.1 mmol), Pd(OAc)2 (0.01 mmol), N-Ac–Gly–OH (0.06 mmol), DCH (0.3 mmol), A-1 (0.1 mmol), HFIP (1 mL), 48 h, 90 °C, Ar. Isolated yields.
HOAc (0.5 mmol) instead of A-2.
N-TFA–Gly–OH (0.06 mmol) instead of N-TFA–β-Ala–OH.
N-Tf–β-Ala–OH (0.06 mmol) instead of N-TFA–β-Ala–OH. DCH = 1,3-dichloro-5,5-dimethylhydantoin.
|
|
To demonstrate the utility of our meta-C–H bromination method, a gram-scale reaction was conducted, and the desired product 2l was obtained in 90% yield (Scheme 2a). The carbamate DG and nosyl protecting group could be removed easily under mild conditions in good yields of 7b and 8b, respectively (Scheme 2b). The broad synthetic utility of the meta-C–H bromination reaction was then demonstrated by carrying out arylation, amination, cyanation, and borylation of 7b and 8b, respectively, using known established reaction conditions (Scheme 2c).
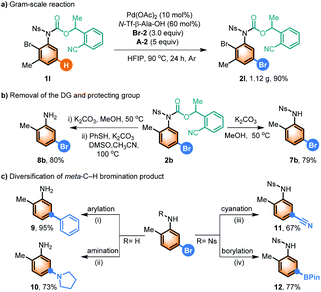 |
| Scheme 2 Synthetic elaborations. Reaction conditions: (i) 8b (0.2 mmol), Pd(OAc)2 (2 mol%), phenylboronic acid (1.5 equiv.), (i-Pr)2NH (2.0 equiv.), H2O (0.5 mL), 100 °C, 4 h, Ar. (ii) 8b (1.5 mmol), CuI (10 mol%), L-proline (20 mol%), K2CO3 (2.0 equiv.), tetrahydropyrrole (1.5 equiv.), DMSO (1 mL), 90 °C, 29 h, Ar. (iii) 7b (0.1 mmol), Pd(PPh3)4 (10 mol%), Zn(CN)2 (2.0 equiv.), DMF (1 mL), 150 °C, 24 h, Ar. (iv) 7b (0.1 mmol), B2Pin2 (1.2 equiv.), KOAc (3.0 equiv.), THF (6 mL), PdCl2(dppf)–DCM (10 mol%), 80 °C, 24 h, Ar. | |
On the basis of the above results and previous studies,9h,j,m a plausible catalytic cycle is proposed in Scheme 3 for meta-C–H bromination of aniline derivative 1a. Intermediate B is generated by coordination of substrate 1a with Pd(II) species A, bringing the Pd(II) metal center close to the meta-C–H bond of the aromatic ring of aniline. Then chelation-assisted insertion of Pd(II) into the meta-C–H bond leads to complex C. Oxidative addition of C with brominating reagent Br-2, which might be activated by polyfluorobenzoic acid such as A-1, delivers a Pd(IV) intermediate D. It should be noted that polyfluorobenzoic acid might also act as a ligand to increase the electrophilicity of the Pd(II) catalyst. Finally, reductive elimination of D affords meta-brominated product 2a and phthalimide after ligand exchange, liberating Pd(II) species A to re-enter the catalytic cycle.
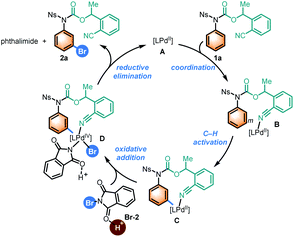 |
| Scheme 3 Proposed catalytic cycle of meta-C–H bromination of aniline derivatives. | |
Conclusions
In summary, we have developed the first Pd(II)-catalyzed meta-C–H bromination of aniline derivatives with commercially available N-bromophthalimide (NBP, Br-2) using the electron-deficient polyfluorobenzoic acid as the crucial additive. Moreover, a range of substituted benzoic acid derivatives are also viable, and meta-C–H chlorination is also promising under similar reaction conditions. Notably, many multiple-halogenated aniline and benzoic acid derivatives could be afforded by our methods, which is possibly interesting for medicinal chemistry. Finally, the DG can be removed under mild conditions, and the brominated products could be readily elaborated to various synthetically useful derivatives. We believe these results will find synthetic application for building up higher levels of structural complexity via halogenating arenes at unusual challenging positions.
Data availability
Experimental procedures, characterisation data, and NMR spectra for new compounds can be found in the ESI.†
Author contributions
H. Wang performed the experiments and developed the method. L. Fu and C. Zhou prepared some of the substrates and repeated some of the reactions. G. Li directed the project and wrote the manuscript with feedback from other authors.
Conflicts of interest
There are no conflicts to declare.
Acknowledgements
We are grateful for the financial support from NSFC (Grant No. 22022111 and 22071248) and the Natural Science Foundation of Fujian Province (2020J02008).
Notes and references
-
(a) I. Saikia, A. J. Borah and P. Phukan, Chem. Rev., 2016, 116, 6837 CrossRef CAS PubMed;
(b) D. A. Petrone, J. Ye and M. Lautens, Chem. Rev., 2016, 116, 8003 CrossRef CAS PubMed;
(c) L. Voskressensky, N. Golantsov and A. Maharramov, Synthesis, 2016, 48, 615 CrossRef CAS;
(d) R. Wilcken, M. O. Zimmermann, A. Lange, A. C. Joerger and F. M. Boeckler, J. Med. Chem., 2013, 56, 1363 CrossRef CAS PubMed.
-
A. de Meijere and F. Diederich, Metal-Catalyzed Cross-Coupling Reactions, Wiley-VCH Verlag GmbH & Co. KGaA, Weinheim, Germany, 2004 Search PubMed.
-
(a)
R. Taylor, Electrophilic Aromatic Substitution, Wiley, Chichester, 1990 Search PubMed;
(b) V. Snieckus, Chem. Rev., 1990, 90, 879 CrossRef CAS.
-
(a) X. Chen, K. M. Engle, D.-H. Wang and J.-Q. Yu, Angew. Chem., Int. Ed., 2009, 48, 5094 CrossRef CAS PubMed;
(b) T. W. Lyons and M. S. Sanford, Chem. Rev., 2010, 110, 1147 CrossRef CAS PubMed;
(c) L. McMurray, F. O'Hara and M. J. Gaunt, Chem. Soc. Rev., 2011, 40, 1885 RSC;
(d) C. S. Yeung and V. M. Dong, Chem. Rev., 2011, 111, 1215 CrossRef CAS PubMed;
(e) N. Kuhl, M. N. Hopkinson, J. Wencel-Delord and F. Glorius, Angew. Chem., Int. Ed., 2012, 51, 10236 CrossRef CAS PubMed;
(f) J. Wencel-Delord and F. Glorius, Nat. Chem., 2013, 5, 369 CrossRef CAS PubMed;
(g) B.-J. Li and Z.-J. Shi, Chem. Soc. Rev., 2012, 41, 5588 RSC;
(h) G. Song, F. Wang and X. Li, Chem. Soc. Rev., 2012, 41, 3651 RSC;
(i) S. A. Girard, T. Knauber and C.-J. Li, Angew. Chem., Int. Ed., 2014, 53, 74 CrossRef CAS PubMed;
(j) O. Daugulis, J. Roane and L. D. Tran, Acc. Chem. Res., 2015, 48, 1053 CrossRef CAS PubMed;
(k) G. He, B. Wang, W. A. Nack and G. Chen, Acc. Chem. Res., 2016, 49, 635 CrossRef CAS PubMed;
(l) Z. Chen, B. Wang, J. Zhang, W. Yu, Z. Liu and Y. Zhang, Org. Chem. Front., 2015, 2, 1107 RSC;
(m) G. Liao and B.-F. Shi, Acta Chim. Sin., 2015, 73, 1283 CrossRef CAS;
(n) A. Dey, S. Maity and D. Maiti, Chem. Commun., 2016, 52, 12398 RSC;
(o) M. Pichette Drapeau and L. J. Gooßen, Chem.–Eur. J., 2016, 22, 18654 CrossRef CAS PubMed;
(p) Y. Park, Y. Kim and S. Chang, Chem. Rev., 2017, 117, 9247 CrossRef CAS PubMed;
(q) R. Shang, L. Ilies and E. Nakamura, Chem. Rev., 2017, 117, 9086 CrossRef CAS PubMed;
(r) P. Wedi and M. van Gemmeren, Angew. Chem., Int. Ed., 2018, 57, 13016 CrossRef CAS PubMed;
(s) P. Gandeepan, T. Müller, D. Zell, G. Cera, S. Warratz and L. Ackermann, Chem. Rev., 2019, 119, 2192 CrossRef CAS PubMed;
(t) Q. Zhang and B.-F. Shi, Chin. J. Chem., 2019, 37, 647 CrossRef CAS;
(u) Y. Hu and C. Wang, Acta Phys.-Chim. Sin., 2019, 35, 913 CAS;
(v) S. Rej, Y. Ano and N. Chatani, Chem. Rev., 2020, 120, 1788 CrossRef CAS PubMed;
(w) A. Trowbridge, S. M. Walton and M. J. Gaunt, Chem. Rev., 2020, 120, 2613 CrossRef CAS PubMed;
(x) Y. Qiu, C. Zhu, M. Stangier, J. Struwe and L. Ackermann, CCS Chem., 2020, 2, 1529 Search PubMed;
(y) H. Tong and G. Chen, Chin. J. Org. Chem., 2021, 41, 4103 CrossRef;
(z) U. Dutta, S. Maiti, T. Bhattacharya and D. Maiti, Science, 2021, 372, 658 CrossRef PubMed.
-
(a) X. Wan, Z. Ma, B. Li, K. Zhang, S. Cao, S. Zhang and Z. Shi, J. Am. Chem. Soc., 2006, 128, 7416 CrossRef CAS PubMed;
(b) C.-L. Ciana, R. J. Phipps, J. R. Brandt, F.-M. Meyer and M. J. Gaunt, Angew. Chem., Int. Ed., 2011, 50, 458 CrossRef CAS PubMed;
(c) K. Sun, Y. Li, T. Xiong, J. Zhang and Q. Zhang, J. Am. Chem. Soc., 2011, 133, 1694 CrossRef CAS PubMed;
(d) N. Schroder, J. Wencel-Delord and F. Glorius, J. Am. Chem. Soc., 2012, 134, 8298 CrossRef PubMed;
(e) Q. Li, S.-Y. Zhang, G. He, Z. Ai, W. A. Nack and G. Chen, Org. Lett., 2014, 16, 1764 CrossRef CAS PubMed;
(f) B. B. Zhan, Y. H. Liu, F. Hu and B. F. Shi, Chem. Commun., 2016, 52, 4934 RSC;
(g) J. A. Leitch, C. L. McMullin, A. J. Paterson, M. F. Mahon, Y. Bhonoah and C. G. Frost, Angew. Chem., Int. Ed., 2017, 56, 15131 CrossRef CAS PubMed;
(h) H. Kajita and A. Togni, ChemistrySelect, 2017, 2, 1117 CrossRef CAS;
(i) M. T. Mihai, B. D. Williams and R. J. Phipps, J. Am. Chem. Soc., 2019, 141, 15477 CrossRef CAS PubMed;
(j) J. R. M. Bastidas, T. J. Oleskey, S. L. Miller, M. R. Smith and R. E. Maleczka, J. Am. Chem. Soc., 2019, 141, 15483 CrossRef PubMed.
-
(a) C. J. Teskey, A. Y. Lui and M. F. Greaney, Angew. Chem., Int. Ed., 2015, 54, 11677 CrossRef CAS PubMed;
(b) Q. Yu, L. Hu, Y. Wang, S. Zheng and J. Huang, Angew. Chem., Int. Ed., 2015, 54, 15284 CrossRef CAS PubMed;
(c) S. Warratz, D. J. Burns, C. Zhu, K. Korvorapun, T. Rogge, J. Scholz, C. Jooss, D. Gelman and L. Ackermann, Angew. Chem., Int. Ed., 2017, 56, 1557 CrossRef CAS PubMed;
(d) G. M. Reddy, N. S. Rao and H. Maheswaran, Org. Chem. Front., 2018, 5, 1118 RSC;
(e) Z. Fan, H. Lu, Z. Cheng and A. Zhang, Chem. Commun., 2018, 54, 6008 RSC;
(f) Y. Wang, H. Simon, X. Chen, Z. Lin, S. Chen and L. Ackermann, Angew. Chem., Int. Ed., 2022, e202201595, DOI:10.1002/anie.202201595.
-
(a) L. Chu, M. Shang, K. Tanaka, Q. Chen, N. Pissarnitski, E. Streckfuss and J.-Q. Yu, ACS Cent. Sci., 2015, 1, 394 CrossRef CAS PubMed;
(b) Z. Jin, L. Chu, Y.-Q. Chen and J.-Q. Yu, Org. Lett., 2018, 20, 425 CrossRef CAS PubMed;
(c) M. Liu, L.-J. Li, J. Zhang, H. Xu and H.-X. Dai, Chin. Chem. Lett., 2020, 31, 1301 CrossRef CAS.
-
(a) S. Li, C. Zhang, L. Fu, H. Wang, L. Cai, X. Chen, X. Wang and G. Li, CCS Chem., 2022, 4, 1889 CrossRef;
(b) T. Zhang, S. Li, C. Zhou, X. Wang, M. Zhang, Z. Gao and G. Li, Chin. J. Org. Chem., 2021, 41, 3511 CrossRef.
-
(a) J. Li, S. De Sarkar and L. Ackermann, Top. Organomet. Chem., 2015, 55, 217 CrossRef;
(b) J. A. Leitch and C. G. Frost, Chem. Soc. Rev., 2017, 46, 7145 RSC;
(c) M. Font, J. M. Quibell, G. J. P. Perry and I. Larrosa, Chem. Commun., 2017, 53, 5584 RSC;
(d) Y. Gao and G. Li, Aldrichimica Acta, 2017, 50, 61 CAS;
(e) A. Biafora and L. J. Gooßen, Synlett, 2017, 28, 1885 CrossRef CAS;
(f) M. T. Mihai, G. R. Genov and R. J. Phipps, Chem. Soc. Rev., 2018, 47, 149 RSC;
(g) C. Haldar, M. E. Hoque, R. Bisht and B. Chattopadhyay, Tetrahedron Lett., 2018, 59, 1269 CrossRef CAS;
(h) A. Dey, S. K. Sinha, T. K. Achar and D. Maiti, Angew. Chem., Int. Ed., 2019, 58, 10820 CrossRef CAS PubMed;
(i) J. Wang and G. Dong, Chem. Rev., 2019, 119, 7478 CrossRef CAS PubMed;
(j) G. Meng, N. Y. S. Lam, E. L. Lucas, T. G. Saint-Denis, P. Verma, N. Chekshin and J.-Q. Yu, J. Am. Chem. Soc., 2020, 142, 10571 CrossRef CAS PubMed;
(k) G. Liao, Y.-J. Wu and B.-F. Shi, Acta Chim. Sin., 2020, 78, 289 CrossRef CAS;
(l) L. Ackermann, K. Korvorapun, R. C. Samanta and T. Rogge, Synthesis, 2021, 53, 2911 CrossRef;
(m) S. K. Sinha, S. Guin, S. Maiti, J. P. Biswas, S. Porey and D. Maiti, Chem. Rev., 2022, 122, 5682 CrossRef CAS PubMed.
- R. J. Phipps and M. J. Gaunt, Science, 2009, 323, 1593 CrossRef CAS PubMed.
-
(a) R.-Y. Tang, G. Li and J.-Q. Yu, Nature, 2014, 507, 215 CrossRef CAS PubMed;
(b) H. Shi, P. Wang, S. Suzuki, M. E. Farmer and J.-Q. Yu, J. Am. Chem. Soc., 2016, 138, 14876 CrossRef CAS PubMed;
(c) P. Wang, M. E. Farmer, X. Huo, P. Jain, P. X. Shen, M. Ishoey, J. E. Bradner, S. R. Wisniewski, M. D. Eastgate and J.-Q. Yu, J. Am. Chem. Soc., 2016, 138, 9269 CrossRef CAS PubMed;
(d) P. Wang, G. C. Li, P. Jain, M. E. Farmer, J. He, P. X. Shen and J.-Q. Yu, J. Am. Chem. Soc., 2016, 138, 14092 CrossRef CAS PubMed.
- J. Li, S. Warratz, D. Zell, S. De Sarkar, E. E. Ishikawa and L. Ackermann, J. Am. Chem. Soc., 2015, 137, 13894 CrossRef CAS PubMed.
-
(a) H. J. Davis, M. T. Mihai and R. J. Phipps, J. Am. Chem. Soc., 2016, 138, 12759 CrossRef CAS PubMed;
(b) H. J. Davis, G. R. Genov and R. J. Phipps, Angew. Chem., Int. Ed., 2017, 56, 13351 CrossRef CAS PubMed;
(c) G. R. Genov, J. L. Douthwaite, A. S. K. Lahdenperä, D. C. Gibson and R. J. Phipps, Science, 2020, 367, 1246 CrossRef CAS PubMed.
- S. Okumura, T. Komine, E. Shigeki, K. Semba and Y. Nakao, Angew. Chem., Int. Ed., 2018, 57, 929 CrossRef CAS PubMed.
-
(a) L. Wang, Z. Han and R. Fan, Adv. Synth. Catal., 2010, 352, 3230 CrossRef CAS;
(b) S. Vásquez-Céspedes, M. Holtkamp, U. Karst and F. Glorius, Synlett, 2017, 28, 2759 CrossRef;
(c) G. Li, X. Ma, C. Jia, Q. Han, Y. Wang, J. Wang, L. Yu and S. Yang, Chem. Commun., 2017, 53, 1261 RSC;
(d) R. Bisht, M. E. Hoque and B. Chattopadhyay, Angew. Chem., Int. Ed., 2018, 57, 15762 CrossRef CAS PubMed;
(e) B. Wang, Y. Zhou, N. Xu, X. Xu, X. Xu and Z. Jin, Org. Lett., 2019, 21, 1885 CrossRef CAS PubMed.
- L. Yang, L. Fu and G. Li, Adv. Synth. Catal., 2017, 359, 2235 CrossRef CAS.
-
(a) L. Ackermann, P. Novák, R. Vicente and N. Hofmann, Angew. Chem., Int. Ed., 2009, 48, 6045 CrossRef CAS PubMed;
(b) O. Saidi, J. Marafie, A. E. W. Ledger, P. M. Liu, M. F. Mahon, G. Kociok-Köhn, M. K. Whittlesey and C. G. Frost, J. Am. Chem. Soc., 2011, 133, 19298 CrossRef CAS PubMed;
(c) N. Hofmann and L. Ackermann, J. Am. Chem. Soc., 2013, 135, 5877 CrossRef CAS PubMed;
(d) Z. Fan, J. Ni and A. Zhang, J. Am. Chem. Soc., 2016, 138, 8470 CrossRef CAS PubMed;
(e) G. Li, D. Li, J. Zhang, D.-Q. Shi and Y. Zhao, ACS Catal., 2017, 7, 4138 CrossRef CAS;
(f) G. Li, P. Gao, X. Lv, C. Qu, Q. Yan, Y. Wang, S. Yang and J. Wang, Org. Lett., 2017, 19, 2682 CrossRef CAS PubMed;
(g) J. A. Leitch, C. L. McMullin, M. F. Mahon, Y. Bhonoah and C. G. Frost, ACS Catal., 2017, 7, 2616 CrossRef CAS;
(h) B. Li, S.-L. Fang, D.-Y. Huang and B.-F. Shi, Org. Lett., 2017, 19, 3950 CrossRef CAS PubMed;
(i) X. G. Wang, Y. Li, H. C. Liu, B. S. Zhang, X. Y. Gou, Q. Wang, J. W. Ma and Y. M. Liang, J. Am. Chem. Soc., 2019, 141, 13914 CrossRef CAS PubMed;
(j) P. Gandeepan, J. Koeller, K. Korvorapun, J. Mohr and L. Ackermann, Angew. Chem., Int. Ed., 2019, 58, 9820 CrossRef CAS PubMed;
(k) A. Sagadevan and M. F. Greaney, Angew. Chem., Int. Ed., 2019, 58, 9826 CrossRef CAS PubMed;
(l) K. Korvorapun, M. Moselage, J. Struwe, T. Rogge, A. M. Messinis and L. Ackermann, Angew. Chem., Int. Ed., 2020, 59, 18795 CrossRef CAS PubMed.
-
(a) D. Leow, G. Li, T.-S. Mei and J.-Q. Yu, Nature, 2012, 486, 518 CrossRef CAS PubMed;
(b) S. Lee, H. Lee and K. L. Tan, J. Am. Chem. Soc., 2013, 135, 18778 CrossRef CAS PubMed;
(c) L. Wan, N. Dastbaravardeh, G. Li and J.-Q. Yu, J. Am. Chem. Soc., 2013, 135, 18056 CrossRef CAS PubMed;
(d) Y.-F. Yang, G.-J. Cheng, P. Liu, D. Leow, T.-Y. Sun, P. Chen, X. Zhang, J.-Q. Yu, Y.-D. Wu and K.-N. Houk, J. Am. Chem. Soc., 2014, 136, 344 CrossRef CAS PubMed;
(e) J. Xu, J. Chen, F. Gao, S. Xie, X. Xu, Z. Jin and J.-Q. Yu, J. Am. Chem. Soc., 2019, 141, 1903 CrossRef CAS PubMed;
(f) L. Fang, T. G. Saint-Denis, B. L. H. Taylor, S. Ahlquist, K. Hong, S. Liu, L. Han, K. N. Houk and J.-Q. Yu, J. Am. Chem. Soc., 2017, 139, 10702 CrossRef CAS PubMed;
(g) Z. Fan, K. L. Bay, X. Chen, Z. Zhuang, H. S. Park, K. S. Yeung, K. N. Houk and J.-Q. Yu, Angew. Chem., Int. Ed., 2020, 59, 4770 CrossRef CAS PubMed;
(h) N. Y. S. Lam, Z. Fan, K. Wu, H. S. Park, S. Y. Shim, D. A. Strassfeld and J.-Q. Yu, J. Am. Chem. Soc., 2022, 144, 2793 CrossRef CAS PubMed.
-
(a) M. Bera, A. Maji, S. K. Sahoo and D. Maiti, Angew. Chem., Int. Ed., 2015, 54, 8515 CrossRef CAS PubMed;
(b) S. Bag, R. Jayarajan, R. Mondal and D. Maiti, Angew. Chem., Int. Ed., 2017, 56, 3182 CrossRef CAS PubMed;
(c) R. Jayarajan, J. Das, S. Bag, R. Chowdhury and D. Maiti, Angew. Chem., Int. Ed., 2018, 57, 7659 CrossRef CAS PubMed;
(d) R.-J. Mi, Y. Z. Sun, J.-Y. Wang, J. Sun, Z. Xu and M.-D. Zhou, Org. Lett., 2018, 20, 5126 CrossRef CAS PubMed;
(e) S. Fang, X. Wang, F. Yin, P. Cai, H. Yang and L. Kong, Org. Lett., 2019, 21, 1841 CrossRef CAS PubMed;
(f) S. Porey, X. Zhang, S. Bhowmick, V. K. Singh, S. Guin, R. S. Paton and D. Maiti, J. Am. Chem. Soc., 2020, 142, 3762 CrossRef CAS PubMed;
(g) S. Bag, S. K, A. Mondal, R. Jayarajan, U. Dutta, S. Porey, R. B. Sunoj and D. Maiti, J. Am. Chem. Soc., 2020, 142, 12453 CrossRef CAS PubMed;
(h) S. Bag, S. Jana, S. Pradhan, S. Bhowmick, N. Goswami, S. K. Sinha and D. Maiti, Nat. Commun., 2021, 12, 1393 CrossRef CAS PubMed;
(i) A. Saha, S. Guin, W. Ali, T. Bhattacharya, S. Sasmal, N. Goswami, G. Prakash, S. K. Sinha, H. B. Chandrashekar, S. Panda, S. S. Anjana and D. Maiti, J. Am. Chem. Soc., 2022, 144, 1929 CrossRef CAS PubMed.
-
(a) S. Li, H. Wang, Y. Weng and G. Li, Angew. Chem., Int. Ed., 2019, 58, 18502 CrossRef CAS PubMed;
(b) S. Li, L. Cai, H. Ji, L. Yang and G. Li, Nat. Commun., 2016, 7, 10443 CrossRef PubMed;
(c) S. Li, H. Ji, L. Cai and G. Li, Chem. Sci., 2015, 6, 5595 RSC.
-
(a) L. Zhang, C. Zhao, Y. Liu, J. Xu, X. Xu and Z. Jin, Angew. Chem., Int. Ed., 2017, 56, 12245 CrossRef CAS PubMed;
(b) S. Xie, S. Li, W. Ma, X. Xu and Z. Jin, Chem. Commun., 2019, 55, 12408 RSC;
(c) G. Li, Y. Yan, P. Zhang, X. Xu and Z. Jin, ACS Catal., 2021, 11, 10460 CrossRef CAS.
-
(a) H.-J. Xu, Y.-S. Kang, H. Shi, P. Zhang, Y.-K. Chen, B. Zhang, Z.-Q. Liu, J. Zhao, W.-Y. Sun, J.-Q. Yu and Y. Lu, J. Am. Chem. Soc., 2019, 141, 76 CrossRef CAS PubMed;
(b) H.-J. Xu, Y. Lu, M. E. Farmer, H.-W. Wang, D. Zhao, Y.-S. Kang, W.-Y. Sun and J.-Q. Yu, J. Am. Chem. Soc., 2017, 139, 2200 CrossRef CAS PubMed.
-
(a) J. Cornella, M. Righi and I. Larrosa, Angew. Chem., Int. Ed., 2011, 50, 9429 CrossRef CAS PubMed;
(b) J. Luo, S. Preciado and I. Larrosa, J. Am. Chem. Soc., 2014, 136, 4109 CrossRef CAS PubMed;
(c) D. Lee and S. Chang, Chem.–Eur. J., 2015, 21, 5364 CrossRef CAS PubMed;
(d) X.-Y. Shi, K.-Y. Liu, J. Fan, X.-F. Dong, J.-F. Wei and C.-J. Li, Chem.–Eur. J., 2015, 21, 1900 CrossRef CAS PubMed;
(e) Y. Zhang, H. Zhao, M. Zhang and W. Su, Angew. Chem., Int. Ed., 2015, 54, 3817 CrossRef CAS PubMed;
(f) X. Qin, D. Sun, Q. You, Y. Cheng, J. Lan and J. You, Org. Lett., 2015, 17, 1762 CrossRef CAS PubMed;
(g) L. Huang, A. Biafora, G. Zhang, V. Bragoni and L. J. Gooßen, Angew. Chem., Int. Ed., 2016, 55, 6933 CrossRef CAS PubMed;
(h) A. R. A. Spencer, R. Korde, M. Font and I. Larrosa, Chem. Sci., 2020, 11, 4204 RSC.
-
(a) X.-C. Wang, W. Gong, L.-Z. Fang, R.-Y. Zhu, S. Li, K. M. Engle and J.-Q. Yu, Nature, 2015, 519, 334 CrossRef CAS PubMed;
(b) Z. Dong, J. Wang and G. Dong, J. Am. Chem. Soc., 2015, 137, 5887 CrossRef CAS PubMed;
(c) H. Shi, A. N. Herron, Y. Shao, Q. Shao and J.-Q. Yu, Nature, 2018, 558, 581 CrossRef CAS PubMed;
(d) P.-X. Ling, K. Chen and B.-F. Shi, Chem. Commun., 2017, 53, 2166 RSC;
(e) M. Zhang, A. Luo, Y. Shi, R. Su, Y. Yang and J. You, ACS Catal., 2019, 9, 11802 CrossRef CAS.
- Y. Kuninobu, H. Ida, M. Nishi and M. Kanai, Nat. Chem., 2015, 7, 712 CrossRef CAS PubMed.
- R. Bisht and B. Chattopadhyay, J. Am. Chem. Soc., 2016, 138, 84 CrossRef CAS PubMed.
-
(a) Y. Yang, R. Li, Y. Zhao, D. Zhao and Z. Shi, J. Am. Chem. Soc., 2016, 138, 8734 CrossRef CAS PubMed;
(b) P. Jiang, F. Li, Y. Xu, Q. Liu, J. Wang, H. Ding, R. Yu and Q. Wang, Org. Lett., 2015, 17, 5918 CrossRef CAS PubMed;
(c) M. Zhang, A. Luo, Y. Shi, R. Su, Y. Yang and J. You, ACS Catal., 2019, 11802 CrossRef CAS.
- L. Yang, N. Uemura and Y. Nakao, J. Am. Chem. Soc., 2019, 141, 7972 CrossRef CAS PubMed.
- During the review of this work, our group also submitted the work on meta-C–H iodination of aniline derivatives: N. Wang, Z. Chi, X. Wang, Z. Gao, S. Li and G. Li, Org. Lett., 2022, 24, 3657 CrossRef CAS PubMed.
-
(a) R. A. Rodriguez, C.-M. Pan, Y. Yabe, Y. Kawamata, M. D. Eastgate and P. S. Baran, J. Am. Chem. Soc., 2014, 136, 6908 CrossRef CAS PubMed;
(b) S. C. Fosu, C. M. Hambira, A. D. Chen, J. R. Fuchs and D. A. Nagib, Chem, 2019, 5, 417 CrossRef CAS PubMed;
(c) S. Song, X. Li, J. Wei, W. Wang, Y. Zhang, L. Ai, Y. Zhu, X. Shi, X. Zhang and N. Jiao, Nat. Catal., 2019, 3, 107 CrossRef.
-
(a) M. Simonetti, D. M. Cannas, X. Just-Baringo, I. J. Vitorica-Yrezabal and I. Larrosa, Nat. Chem., 2018, 10, 724 CrossRef CAS PubMed;
(b) T. K. Allred, F. Manoni and P. G. Harran, Chem. Rev., 2017, 117, 11994 CrossRef CAS PubMed.
|
This journal is © The Royal Society of Chemistry 2022 |
Click here to see how this site uses Cookies. View our privacy policy here.