DOI:
10.1039/D2RA06271B
(Paper)
RSC Adv., 2022,
12, 34325-34334
Synthesis and characterization of thiophene-derived palladium(II) complex immobilized on FSM-16 and its application in the novel synthesis of 7-(aryl)-7,12-dihydro-6H-indeno[1,2,4]triazolo[1,5-a]pyrimidine-6-one derivatives†
Received
5th October 2022
, Accepted 22nd November 2022
First published on 29th November 2022
Abstract
The present study aims at synthesizing a palladium complex with a thiophene-carboimine ligand, supported on FSM-16 as a mesoporous silica support. Firstly, the prepared FSM-16 was modified using 3-aminopropyl group. The imine bond was subsequently formed by condensation of FSM-16-propyl amine with thiophene-2-carbaldehyde. Finally, the imine/thiophene-FSM-16 reacted with PdCl2 to form PdCl2-imine/thiophene-FSM-16. The structural and physicochemical properties of the prepared nanocomposite were characterized using FT-IR, TEM, XRD, FE-SEM, EDS, BET, and TGA analyses. PdCl2-imine/thiophene-FSM-16 exhibited efficient catalytic activity in the synthesis of indeno-1,2,4-triazolo[1,5-a]pyrimidine derivatives via a new three-component reaction between indan-1,3-dione, aromatic aldehydes and 3-amino-1H-1,2,4-triazole in water as the green solvent. Significantly, the heterogeneous catalyst can be easily separated from the reaction mixture and reused in another reaction.
1. Introduction
In recent years, heterogeneous catalysts have attracted much attention due to simple separation, good stability, high performance, recyclability, and reusability.1–3 The heterogeneous catalysts with mesoporous structures have received intensive research due to their fascinating properties. Mesoporous silica materials with unique properties which possess high pore volume and surface area have also been regarded as significant compounds.4–7 They have pore sizes in the range of 2 to 50 nm.8,9 Mesoporous silica materials, such as FSM-16 and MCM-41, can be applied as solid substrates for adsorbents, chromatography, catalysts, and electrodes and also in drug delivery fields.10–17 Also, some important works reported on the synthesis of mesoporous Pd based nanocatalyst are Fe3O4@SiO2@IL-PMO/Pd, Fe3O4@MePMO-IL/Pd, HMS–CPTMS–Cy–Pd, MCM(Pd)-41.18–21 In this regard, FSM-16 has attracted great attention, as compared with other porous materials. Accordingly, a folded-sheet mechanism is applied, which results in forming a hexagonal array of channels.22,23 Because of the large surface area, large pore size, and high-density surface silanol sites, various functional groups can be grafted or incorporated onto the surface of FSM-16.24,25 Some transition metal complexes are prepared on the functionalized FSM-16 support and successfully used in organic reactions.26–28 Moreover, when the metal source is added to the kanemite preparation stages, various metals are directly substituted in the silica framework of FSM-16.29 FSM-16 shows thermal stability, which can be regarded as the most significant parameter in membrane application. Some recently studied FSM-16 catalysis systems are Fe3O4@FSM-16-SO3H, FSM-16/AEPC-SO3H, FSM-16-SO3H, and FSM-16-Met.30–33 Furthermore, FSM-16 materials have various uses in photo-metathesis reactions. Consequently, the development of synthetic procedures that yield novel heterogeneous FSM-16-based catalysts is still a great challenge for researchers.
Nowadays, the most critical challenge would be the design of effective chemical reaction sequences, providing great structural complexity with minimum synthetic steps.34,35 Multi-component reactions (MCRs) can be regarded as transformations in which, to form the product, we need more than two starting materials. Moreover, it is worth mentioning that such reactions will nearly incorporate the atoms of the starting materials. Multi-component one-step reactions play a significant role in synthesizing heterocyclic compounds. This is why the design of reactions based on these methods has received much attention for researchers in recent years.36–38 Heterocycles are among the most famous and remarkable compounds of organic chemistry and are also the basis of many drugs, chemicals, veterinarians, and agriculture.39–41 Many natural drugs such as atropine, codeine, reserpine, papaverine, and morphine are heterocycles.42 Also, new and efficient methods for synthesizing new heterocycles are still in high demand. The N-fused heterocyclic compounds, such as agrochemicals, colors, plastics and pharmaceuticals, are used in daily life. Amongst the best-selling therapeutic drugs, approximately one-third contain fused heterocyclic structures.43–46 Many powerful pharmaceutical products, biologically active compounds, and natural products contain bicyclic heterocycles fused with a pyrimidine.47–52 Among this plethora of combinations, the triazolo pyrimidines scaffolding was considered dramatically in natural compounds. Also, different triazolo pyrimidine derivatives have anti-inflammatory, antiviral, and anti-cancer properties. Triazolo pyrimidines have captured the attention of medicinal chemists owing to their wide variety of applications in medicinal chemistry and biochemistry.53–56
In recent years, multi-component reactions containing 1,3-indanedione have been successfully developed to synthesize spiro heterocycles, spirocycles, and fused heterocycles.57–60 The fused heterocycles are containing the 1,3-indanedione structure from important medicinal and biological frameworks. These compounds have attracted the attention of pharmacologists and chemists due to their broad scope of biological activities. Furthermore, they are widely used in materials, such as semiconductors and nonlinear optical properties of organic photovoltaic systems.61–63
As a follow-up to our research program for the synthesis of new recyclable heterogeneous nanocatalysts,64–71 our attention was focused on FSM-16, which serves as a support for the immobilization of palladium complexes (Scheme 1). The FSM-16-based catalyst, also known as FSM-16@Imine-Thiophen/Pd, was used in the multi-component reactions of indan-1,3-dione, aromatic aldehydes and 3-amino-1H-1,2,4-triazole to produce indeno-1,2,4-triazolo[1,5-a]pyrimidine derivatives.
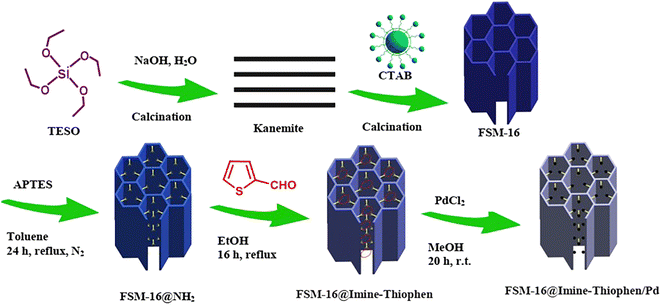 |
| Scheme 1 Synthesis of FSM-16@Imine-Thiophen/Pd nanocatalyst 1. | |
2. Experimental
2.1. Materials
The reagents, chemicals and solvents including tetraethyl ortho silicate (TEOS), cetyltrimethyl ammonium bromide (CTAB), sodium hydroxide (NaOH), 3-aminopropyltriethoxysilane (APTES), thiophene-2-carbaldehyde, PdCl2, 3-amino-1H-1,2,4-triazole, indan-1,3-dione, benzaldehyde, 4-nitrobenzaldehyde, 4-chlorobenzaldehyde, 2-methylbenzaldehyde, 3-ethoxy-4-hydroxyphenylbenzaldehyde, 4-bromobenzaldehyde, indole-3-carboxaldehyde, 4-(benzyloxy)benzaldehyde, ethanol, toluene, methanol were purchased from Fluka, Merck, and Aldrich-Sigma and applied without further purification.
2.2. Instrumental measurements
The FT-IR spectra were carried out using FT-IR JASCO-Model 680 spectroscopy area of 400–4000 cm−1. The NMR spectra analyses were done using Bruker 400 MHz Ultrashield spectrometer device at 400 MHz (1H NMR) and 100 MHz (13C NMR) in DMSO-d6 as solvent. The energy-dispersive X-ray (EDX) analysis was obtained by applying the TESCAN vega model. The nanoparticles morphology was measured using FE-SEM-TESCAN MIRA3, and X-ray analysis was done using the following diffractometer: Rigaku Ultima IV. TEM images were taken using Philips EM208S, the Netherlands, TEM apparatus at 100 kV. Brunauer–Emmett–Teller (BET) determines the technique used to analyze the surface area. Thermogravimetric (TGA) analysis was done using a Perkin-Elmer-6000.
2.3. Catalytic tests
2.3.1. Preparation of FSM-16@NH2. FSM-16 was synthesized based on the literature method.25 Then, the prepared FSM-16 (0.5 g) was dispersed in dry toluene (15 mL) for 15 min, and then 3-aminopropyltriethoxysilane (APTES) (3 mL) was added dropwise, and the reaction mixture was stirred at 90 °C for 24 h under N2 atmosphere. The resulting solid was filtered, washed with dry toluene and dried.
2.3.2. Synthesis of FSM-16@Imine-Thiophen. In the following, the FSM-16-NH2 (0.5 g) was dispersed in ethanol (20 mL) by sonication for 20 min, and thiophene-2-carbaldehyde (1 mL) was added to the mixture. The mixture reaction was stirred for 16 h at 80 °C under an N2 atmosphere. The solid product (FSM-16@Imine-Thiophen) was filtered, washed several times with ethanol and dried.
2.3.3. Preparation of FSM-16@Imine-Thiophen/Pd (nanocatalyst 1). FSM-16@Imine-Thiophen (0.5 g) was dispersed in MeOH (30 mL) for 30 min, and then PdCl2 (0.17 g) was added, and stirred at room temperature for 20 h. In the end, the resultant solid was separated, washed with MeOH, and dried.
2.3.4. General procedure for the synthesis of indeno[1,2,4]triazolo[1,5-a]pyrimidine 5. A mixture of indan-1,3-dione (1 mmol), aldehyde (1 mmol), 3-amino-1H-1,2,4-triazole (1 mmol) and nanocatalyst 1 (0.007 g) was refluxed in H2O (3 mL). TLC was applied to monitor the progress of the reaction. Subsequently, ethanol (10 mL) was added, and the catalyst was separated by filtration. Finally, the pure product was obtained by recrystallization from EtOH.
2.3.5. Spectral data.
2.3.5.1 7-Phenyl-7,12-dihydro-6H-indeno[1,2,4]triazolo[1,5-a]pyrimidine (5a). FT-IR (KBr) (
max, cm−1): 3163, 3062, 2958, 2888, 1708, 1623, 1591, 1530, 1460, 1330, 1309. 1H NMR (400 MHz, DMSO-d6): δ = 11.3 (s, 1H), 8.68 (s, 1H), 8.16 (d, 1H, J = 7.6 Hz), 7.83 (t, 2H, J = 7.2 Hz), 7.09 (d, 2H, J = 7.6 Hz), 6.91 (t, 2H, J = 8.8 Hz), 6.80 (t, 2H, J = 7.2 Hz), 5.89 (s, 1H). 13C NMR (100 MHz, DMSO-d6): δ = 188.76, 167.13, 156.01, 150.14, 144.71, 142.78, 141.20, 139.16, 135.46, 133.40, 130.10, 125.67, 118.84, 109.96, 105.86, 35.16.
2.3.5.2 7-(4-Bromophenyl)-7,12-dihydro-6H-indeno [1,2,4]triazolo[1,5-a]pyrimidine (5b). FT-IR (KBr) (
max, cm−1): 3188, 3107, 3085, 2911, 2855, 1716, 1623, 1590, 1528, 844
670, 523. 1H NMR (400 MHz, DMSO-d6): δ = 12.10 (s, 1H), 8.08 (d, 1H, J = 7.6 Hz), 7.63 (t, 1H, J = 6.4 Hz), 7.58 (t, 1H, J = 7.4 Hz), 7.50 (d, 2H, J = 8 Hz), 7.36 (d, 2H, J = 8 Hz), 7.15 (d, 2H, J = 6.8 Hz), 7.03 (s, 1H), 5.69 (s, 1H). 13C NMR (100 MHz, DMSO-d6): δ = 190.52, 165.81, 153.95, 139.86, 136.27, 135.19, 134.67, 132.26, 131.97, 130.73, 127.62, 123.92, 123.07, 122.79, 117.32, 33.76.
2.3.5.3 7-(4-Nitrophenyl)-7,12-dihydro-6H-indeno[1,2,4]triazolo[1,5-a]pyrimidine (5d). FT-IR (KBr) (
max, cm−1): 3136, 3062, 2923, 2851, 1731, 1618, 1589, 1423, 1326, 1309. 1H NMR (400 MHz, DMSO-d6): δ = 11.15 (s, 1H), 8.17 (d, 2H, J = 8 Hz), 8.12 d (d, 2H, J = 8.4 Hz), 8.07 (d, 2H, J = 7.6 Hz), 7.83–7.93 (m, 2H), 7.71 (t, 1H, J = 7.2 Hz), 5.92 (s, 1H). 13C NMR (100 MHz, DMSO-d6): δ = 188.59, 166.95, 157.40, 152.36, 146.58, 142.29, 137.41, 137.08, 133.24, 132.90, 129.53, 125.12, 117.33, 111.15, 104.50, 36.01.
2.3.5.4 7-(1H-Indol-3-yl)-7,12-dihydro-6H-indeno[1,2,4]triazolo[1,5-a]pyrimidine (5e). FT-IR (KBr) (
max, cm−1): 3178, 3459, 3147, 2960, 2844, 1708, 1612, 1588, 1401. 1H NMR (400 MHz, DMSO-d6): δ = 11.32 (s, 1H), 11.11 (s, 1H), 7.49 (d, 1H, J = 8.8 Hz), 7.38 (t, 2H, J = 8.4 Hz), 7.31 (s, 1H), 7.13 (d, 1H, J = 6.8 Hz), 7.01 (t, 2H, J = 6.4 Hz), 6.60 (d, 2H, J = 7.2 Hz), 6.49 (s, 1H), 5.12 (s, 1H). 13C NMR (100 MHz, DMSO-d6): δ = 190.17, 162.31, 154.17, 151.40, 140.99, 137.12, 129.92, 129.58, 128.60, 128.36, 127.90, 125.59, 122.58, 118.24, 116.64, 113.44, 111.06, 34.65.
2.3.5.5 7-(3-Ethoxy-4-hydroxyphenyl)-7,12-dihydro-6H-indeno[1,2,4]triazolo[1,5-a]pyrimidine (5h). FT-IR (KBr) (
max, cm−1): 3336, 3274, 3119, 2941, 2876, 1725, 1622, 1549, 1438. 1H NMR (400 MHz, DMSO-d6): δ = 12.17 (s, 1H), 11.11 (s, 1H) 7.80 (d, 1H, J = 8 Hz), 7.51 (t, 1H, J = 7.6 Hz), 7.33 (t, 2H, J = 7.2 Hz), 7.15 (s, 1H), 6.83 (s, 1H), 6.75 (d, 2H, J = 8 Hz), 6.64 (d, 1H, J = 8 Hz), 5.05 (s, 1H), 3.58 (q, 4H, J = 7.6 Hz), 1.86 (t, 3H, J = 7.6 Hz). 13C NMR (100 MHz, DMSO-d6): δ = 188.90, 168.35, 161.59, 160.27, 159.53, 155.62, 153.59, 139.27, 135.53, 135.27, 128.38, 117.17, 114.32, 108.83, 107.09, 103.83, 101.35, 65.37, 35.31, 26.13.
3. Results and discussion
3.1. Synthesis and characterization of the catalyst
Herein, the synthesis and characterization of the novel FSM-16-supported nanocatalyst 1 is reported, following the protocol shown in Scheme 1. To prepare FSM-16@Imine-Thiophen/Pd, FSM-16 was primarily synthesized and functionalized with APTES to obtain FSM-16-NH2 nanostructure. Afterward, FSM-16-NH2 reacted with thiophene-2-carbaldehyde to synthesize FSM-16@Imine-Thiophen. Subsequently, regarding the metal coordination complex, PdCl2 was used, affording FSM-16@Imine-Thiophen/Pd nanocatalyst. The synthesized nanocatalyst was investigated using XRD, FT-IR, TGA, FE-SEM, EDS, TEM, and BET techniques.
The TEM, FE-SEM, and XRD analyses of FSM-16@Imine-Thiophen/Pd nanocatalyst were performed to get insight into the structural features of the material. As Fig. 1 illustrates, XRD diffraction patterns of FSM-16 and FSM-16@Imine-Thiophen/Pd catalyst are shown in a range of 2θ = 10–80°. As shown in Fig. 1a, XRD patterns of FSM-16 exhibit four peaks indicative of ordered hexagonal mesostructures at 2θ = 23.4°, 37.1°, 48.5°, and 61.5° assigned to the (100), (110), (200) and (210) planes.72–76 Accordingly, three new peaks can be observed at 2θ = 39.5°, 45.4°, and 68.5°, corresponding to (111), (200), and (220) planes, respectively, which signify the presence of palladium complex on the functionalized FSM-16.75,76 Furthermore, as indicated in Fig. 1b, the intensity of the principal peaks are decreased as compared to bare FSM-16, which is due to the immobilization of organic groups and Pd complex on FSM-16 pore walls.
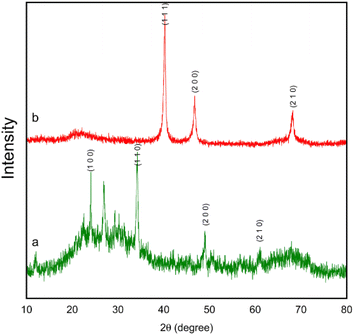 |
| Fig. 1 XRD patterns of FSM-16 (a) and FSM-16@Imine-Thiophen/Pd nanocatalyst (b). | |
The FT-IR spectra of FSM-16, FSM-16-NH2, FSM-16@Imine-Thiophen, and FSM-16@Imine-Thiophen/Pd are illustrated in Fig. 2. Fig. 2a indicates a stretching vibration of the –OH group at about 3417 cm−1. The bands at 474 cm−1, 806 cm−1, and 1106 cm−1 signify the symmetric and asymmetric vibrations of the siloxane group (Si–O–Si). Regarding the spectra of FSM-16-NH2 (Fig. 2b), the appearance of the new peaks at 2927 cm−1 and 3235 cm−1 belong to CH2 and NH2, respectively, which confirms the presence of APTES on FSM-16. Thus, the disappeared of the 3235 cm−1 peak relevant to the NH2 bonds affirms the formation of FSM-16@Imine-Thiophen. Furthermore, as Fig. 2c shows, the band at 1635 cm−1 is related to the C
N bond. According to Fig. 2d, the shifted imine band to a lower frequency in the FSM-16@Imine-Thiophen/Pd nanocatalyst (from 1635 cm−1 to 1627 cm−1), which confirms the formation of palladium complex on the surface of FSM-16@Imine-Thiophen.11,31–33
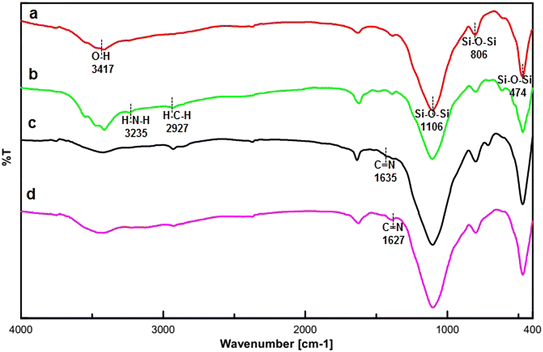 |
| Fig. 2 The FT-IR spectrum of FSM-16 (a), FSM-16@NH2 (b), FSM-16@Imine-Thiophen (c) and FSM-16@Imine-Thiophen/Pd (d). | |
According to Fig. 3, the EDX spectrum of FSM-16@Imine-Thiophen/Pd contains all expected elemental cases, including C, O, N, S, Si, Pd, and Cl elements.
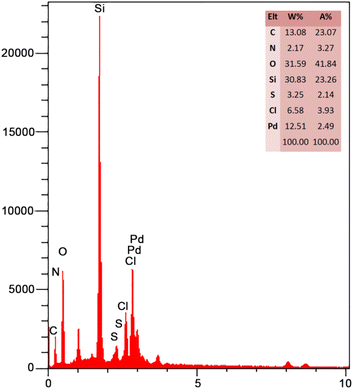 |
| Fig. 3 EDX spectra of FSM-16@Imine-Thiophen/Pd nanocatalyst. | |
The characterization of morphology and size of FSM-16 and FSM-16@Imine-Thiophen/Pd nanoparticles was performed using field effect scanning electron microscopy (FE-SEM) (Fig. 4). Based on FE-SEM images, the surface morphology of the catalyst shows spherical particles, with a regular size of less than 100 nm, possessing a highly ordered pore structure. Furthermore, the compounds FSM-16 and FSM-16@Imine-Thiophen/Pd have similar structures, and there are no significant differences.
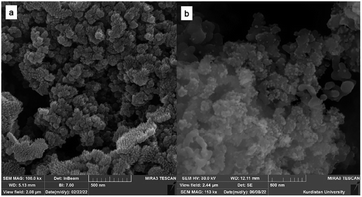 |
| Fig. 4 The FE-SEM images of FSM-16 (a) and FSM-16@Imine-Thiophen/Pd (b). | |
Herein, TEM images of FSM-16@Imine-Thiophen/Pd nanocatalyst, which are shown in Fig. 5, indicate the hexagonal channels with uniform pore size. In these images, the presence Pd nanoparticles with black color on the gray layer FSM-16@Imine-Thiophen have appeared. The histogram of the particle size distribution of the prepared nanocatalyst showed the dimensions of the distribution in the range of 7 to 39 nm and with a mean size of 23 nm.
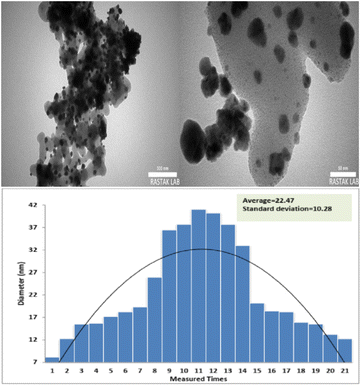 |
| Fig. 5 TEM image of FSM-16@Imine-Thiophen/Pd. | |
Fig. 6 indicates the nitrogen adsorption–desorption isotherms of FSM-16@Imine-Thiophen/Pd nanocatalyst. According to the IUPAC classification, the resulting catalyst is a type VI isotherm, which suggests the presence of mesoporous structures. Based on the BET analysis, the total pore volume, surface area, and mean pore diameter of the catalyst were obtained at 136 m2 g−1, 0.02 cm3 g−1, and 7.75 nm, respectively.
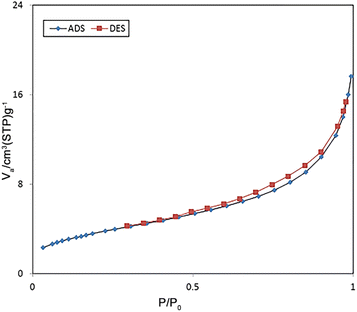 |
| Fig. 6 Nitrogen adsorption–desorption isotherms of FSM-16@Imine-Thiophen/Pd nanocatalyst. | |
Fig. 7 illustrates the TGA analysis of FSM-16@Imine-Thiophen/Pd, which was done to examine the thermal stability of the FSM-16@Imine-Thiophen/Pd nanocatalyst. Regarding the TGA curve, the first weight loss occurs at a temperature below 200 °C of about 2% due to the removal of hydroxyl groups and the physically and chemically adsorbed solvent on the surface of FSM-16@Imine-Thiophen/Pd. Moreover, a weight loss between 200 and 480 °C of about 9% is relevant to the thermal decomposition of organic groups and complexes on the FSM-16 surface.
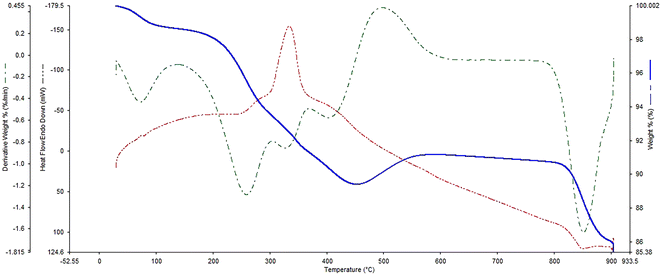 |
| Fig. 7 TGA analysis of the FSM-16@Imine-Thiophen/Pd nanocatalyst. | |
3.2. Synthesis of indeno[1,2,4]triazolo[1,5-a]pyrimidine
After the successful synthesis and characterization of the FSM-16@Imine-Thiophen/Pd, its catalytic activity was investigated in the synthesis of indeno[1,2,4]triazolo[1,5-a]pyrimidine derivatives 5 via the reaction between indan-1,3-dione 2, aromatic aldehydes 3 and 3-amino-1H-1,2,4-triazole 4 (Scheme 2).
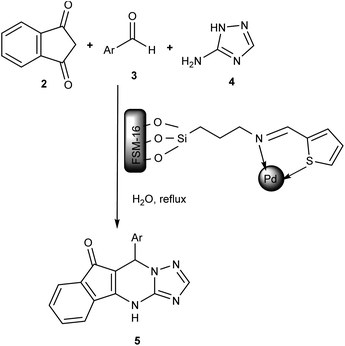 |
| Scheme 2 The synthesis of indeno[1,2,4]triazolo[1,5-a]pyrimidine derivatives in the presence of nanocatalyst 1. | |
Primarily, to optimize the reaction conditions, a reaction between benzaldehyde (1 mmol), indan-1,3-dione (1 mmol), and 3-amino-1H-1,2,4-triazole (1 mmol) was selected as the model reaction. Afterward, different parameters were studied, including various amounts of catalyst, a broad range of temperatures, and various solvents. Initially, the effect of the catalyst amount was examined, and accordingly, the best result was received in the attendance of 0.007 g of the FSM-16@Imine-Thiophen/Pd catalyst. The effect of the various solvents in the model reaction was investigated in the presence of solvents with different polarities and also under solvent-free conditions. The best reaction efficiency in H2O with higher polarity, as a green solvent, environmentally friendly, cheap and, available was obtained. However, due to the hydrophobic effect of organic compounds, the nature of the solvent can actually affect the reaction rate and order of a chemical reaction. Finally, the effect of temperature was also studied. Table 1 presents the summary of the details and results obtained from this study, in which the use of FSM-16@Imine-Thiophen/Pd (0.007 g) as the catalyst in H2O under reflux conditions will be the best condition. To investigate the generality of this protocol, the reaction of different aryl aldehydes, containing both electron-donating and electron-withdrawing groups, was employed in the reaction (Table 2). The reaction proceeded smoothly to afford the desired products 5 in good to excellent yields.
Table 1 The reaction condition optimization in the synthesis of 5aa
Entry |
Catalyst 1 (g) |
Solvent |
Temp. (°C) |
Yieldb (%) |
Reaction conditions: benzaldehyde (1 mmol), 3-amino-1H-1,2,4-triazole (1 mmol), indan-1,3-dione (1 mmol), time: 80 min. Isolated yield. |
1 |
— |
— |
25 |
— |
2 |
— |
— |
80 |
5 |
3 |
— |
— |
90 |
8 |
4 |
— |
— |
100 |
12 |
5 |
0.003 |
— |
100 |
40 |
6 |
0.005 |
— |
100 |
65 |
7 |
0.007 |
— |
100 |
95 |
8 |
0.008 |
— |
100 |
95 |
9 |
0.007 |
EtOH |
Reflux |
75 |
10 |
0.007 |
EtOH : H2O |
Reflux |
80 |
11 |
0.007 |
MeOH |
Reflux |
70 |
12 |
0.007 |
H2O |
Reflux |
96 |
13 |
0.007 |
DMF |
100 |
60 |
14 |
0.007 |
DMSO |
100 |
70 |
15 |
0.007 |
Toluene |
100 |
50 |
16 |
0.007 |
H2O |
50 |
75 |
17 |
0.007 |
H2O |
70 |
80 |
18 |
0.007 |
H2O |
90 |
85 |
Table 2 Synthesis of compound 5 using FSM-16@Imine-Thiophen/Pda
Entry |
Aldehyde |
Product 5 |
Mp (°C) |
Yieldb (%) |
Reaction conditions: aldehyde (1 mmol), 3-amino-1H-1,2,4-triazole (1 mmol), indan-1,3-dione (1 mmol) and FSM-16@Imine-Thiophen/Pd (0.007 g), H2O (5 mL), reflux conditions. Isolated yields. |
5a |
C6H5CHO |
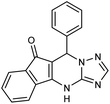 |
313–314 |
96 |
5b |
4-Br–C6H4CHO |
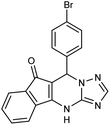 |
330–331 |
94 |
5b |
4-Cl–C6H4CHO |
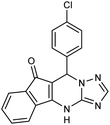 |
291–293 |
96 |
5d |
4-NO2C6H4CHO |
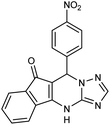 |
320–321 |
90 |
5e |
Indole-3-carboxaldehyde |
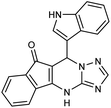 |
323–325 |
82 |
5f |
4-PhCH2O–C6H4CHO |
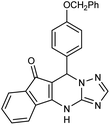 |
341–343 |
80 |
5g |
2-CH3–C6H4CHO |
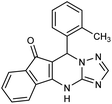 |
288–290 |
87 |
5h |
3-OEt–4-OH–C6H3CHO |
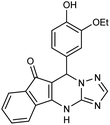 |
345–347 |
80 |
The proposed mechanism for the preparation of indeno[1,2,4]triazolo[1,5-a]pyrimidine derivatives (5a–h) is shown in Scheme 3. Initially, benzene ring of indan-1,3-dione interacts with ring of thiophene via π-stacking and hydrogen atom located between two carbonyl groups shifts to N-atom with formation cation–anion pair which after enol transformation give intermediate (Ia). Next, aldehyde is activated by the Lewis acid site of the catalyst (intermediate Ib). Afterward, the activated carbonyl group of aldehyde (intermediate Ib) is attacked by active methylene of indan-1,3-dione (intermediate Ia) to produce intermediate I (Knoevenagel condensation). Next, 3-amino-1H-1,2,4-triazole attacks intermediate I to give II (Michael addition). Finally, the obtained intermediate III, using intramolecular cyclization and then tautomerization, provided the corresponding product 5 after losing H2O.
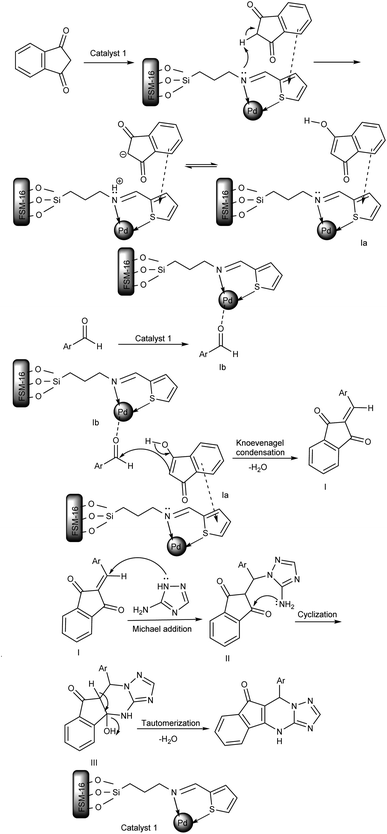 |
| Scheme 3 The suggested mechanism for synthesizing indeno[1,2,4]triazolo[1,5-a]pyrimidine derivatives using FSM-16@Imine-Thiophen/Pd. | |
3.3. Efficiency of catalyst
To investigate whether FSM-16@Imine-Thiophen/Pd operates in a homogeneous or heterogeneous manner, a filtration test has been done in the model reaction under optimized reaction conditions. After nearly 50% of the reaction progress, we separated the FSM-16@Imine-Thiophen/Pd catalyst, using filtration from the reaction mixture. Afterward, the mixture residue continued under optimal conditions, but no substantial increase in product conversion was observed. This experiment confirms that the nanocatalyst is completely heterogeneous, and no leaching of Pd occurs during the reaction process. To investigate the recyclability and reusability of FSM-16@Imine-Thiophen/Pd catalyst, the reaction of benzaldehyde, 3-amino-1H-1,2,4-triazole and indan-1,3-dione was performed under optimum reaction conditions. Once the reaction was complete, ethanol was added, and the catalyst was filtered and washed with ethanol. Next, the recovered catalyst was dried and used for five consecutive cycles without significant reduction in catalytic activity (Fig. 8).
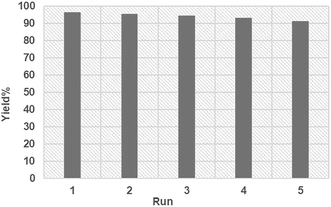 |
| Fig. 8 Reusability of FSM-16@Imine-Thiophen/Pd in the synthesis of 5a. | |
The XRD diffraction patterns (Fig. 9) confirmed the position and relative intensity of the catalyst peaks after recycling and clearly showed their structural stability. Also, the FT-IR analysis was conducted to prove the stability of the catalyst structure following recycling. The results of this spectrum (Fig. 10) showed the high strength of the recycled catalyst.
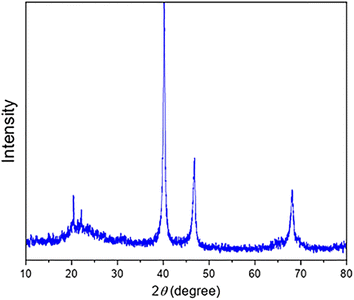 |
| Fig. 9 XRD pattern of the recycled FSM-16@Imine-Thiophen/Pd after the fifth reaction cycle. | |
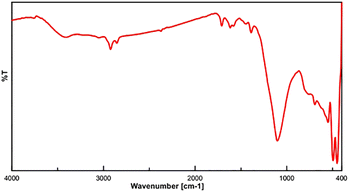 |
| Fig. 10 FT-IR spectrum of the recycled FSM-16@Imine-Thiophen/Pd nanocatalyst 1 (after five times). | |
4. Conclusions
In this article, a novel nanocatalyst based on FSM (FSM-16@Imine-Thiophen/Pd) was prepared and characterized. The XRD analysis, TEM and FE-SEM images of FSM-16@Imine-Thiophen/Pd demonstrated a highly uniform mesostructure. Furthermore, the FT-IR and TGA analyses confirmed the excellent stability of the immobilized palladium(II) complex in the material network. FSM-16@Imine-Thiophen/Pd was successfully used as the catalyst to synthesize novel indeno[1,2,4]triazolo[1,5-a]pyrimidine derivatives. The nanocatalyst can be recovered from the reaction mixture using filtration and reused five times. Some specific advantages of this study are as follows: high yield of the products, using water as the reaction media, mild reaction conditions, short reaction times and high recyclability and stability of the catalyst.
Conflicts of interest
There are no conflicts to declare.
Acknowledgements
The authors gratefully acknowledge the partial support of this work by Yasouj University, Iran.
Notes and references
- K. Sun, H. Shan, G. Lu, C. Cai and M. Beller, Angew. Chem., Int. Ed., 2021, 60, 25188 CrossRef CAS PubMed.
- Y. X. Chen, S. Zhang, Y. J. Xue, L. P. Mo and Z. H. Zhang, Appl. Organomet. Chem., 2022, 36, 6480 Search PubMed.
- Y. Zhang, Y. Lin, T. Duan and L. Song, Mater. Today, 2021, 48, 115 CrossRef CAS.
- G. Martínez-Edo, A. Balmori, I. Pontón, A. M. del Rio and D. Sánchez-García, Catalysts, 2018, 8, 617 CrossRef.
- D. Lu, S. Xu, W. Qiu, Y. Sun, X. Liu, J. Yang and J. Ma, J. Cleaner Prod., 2020, 264, 121644 CrossRef CAS.
- J. A. S. Costa, R. A. de Jesus, D. O. Santos, J. F. Mano, L. P. C. Romão and C. M. Paranhos, Microporous Mesoporous Mater., 2020, 291, 109698 CrossRef CAS.
- C. T. Kresge, J. C. Vartuli, W. J. Roth and M. E. Leonowicz, Stud. Surf. Sci. Catal., 2004, 148, 53 CrossRef CAS.
- Y. Feng, N. Panwar, D. J. H. Tng, S. C. Tjin, K. Wang and K. T. Yong, Coord. Chem. Rev., 2016, 319, 86 CrossRef CAS.
- E. A. Karakhanov, A. P. Glotov, A. G. Nikiforova, A. V. Vutolkina, A. O. Ivanov, S. V. Kardashev, A. L. Maksimov and S. V. Lysenko, Fuel Process. Technol., 2016, 153, 50 CrossRef CAS.
- N. Benyoub, A. Benhamou and A. Debab, J. Adv. Mater. Res., 2022, 1170, 155 Search PubMed.
- M. Hajjami, L. Shiri and A. Jahanbakhshi, Appl. Organomet. Chem., 2015, 29, 668 CrossRef CAS.
- H. Alamgholiloo, S. Nazari, E. Asgari, A. Sheikhmohammadi, B. Hashemzadeh, N. Ghasemian, M. Bigdeloo and A. Ehsani, J. Mol. Liq., 2022, 364, 119990 CrossRef CAS.
- D. Jiraroj, O. Jirarattanapochai, W. Anutrasakda, J. S. M. Samec and D. N. Tungasmita, Appl. Catal., 2021, 291, 120050 CrossRef CAS.
- S. Inagaki, A. Koiwai, N. Suzuki, Y. Fukushima and K. Kuroda, Bull. Chem. Soc. Jpn., 1996, 69, 1449 CrossRef CAS.
- Z. Shariatinia and N. Pourzadi, J. Mol. Struct., 2021, 1242, 130754 CrossRef CAS.
- S. A. Alekseev, V. N. Zaitsev and J. Fraissard, Chem. Mater., 2006, 18, 1987 CrossRef.
- T. Borrego, M. Andrade, M. L. Pinto, A. R. Silva, A. P. Carvalho, J. Rocha, C. Freire and J. Pires, J. Colloid Interface Sci., 2010, 344, 603 CrossRef CAS PubMed.
- M. Neysi and D. Elhamifar, Sci. Rep., 2022, 12, 1 CrossRef PubMed.
- M. Shaker and D. Elhamifar, Mater. Today Chem., 2020, 18, 100377 CrossRef CAS.
- R. Nejat, A. R. Mahjoub, Z. Hekmatian and T. Azadbakht, RSC Adv., 2015, 5, 16029 RSC.
- F. Gholamian and M. Hajjami, Polyhedron, 2019, 170, 649 CrossRef CAS.
- M. Zimowska, A. Michalik-Zym, J. Kryſciak-Czerwenka, R. Dula, R. P. Socha, K. Pamin, M. Bazarnik, K. Bahranowski, Z. Olejniczak, L. Lityſska-Dobrzyſska and E. M. Serwicka, Mater. Res. Bull., 2016, 83, 623 CrossRef CAS.
- T. Selvam, M. Köstner, G. Mabande, W. Schwieger, N. Pfänder and R. Schlögl, J. Porous Mater., 2007, 14, 263 CrossRef CAS.
- A. Hosseini and H. Faghihian, Ind. Eng. Chem. Res., 2019, 76, 122 CrossRef CAS.
- A. Matsumoto, T. Sasaki, N. Nishimiya and K. Tsutsumi, Colloids Surf., A, 2002, 203, 185 CrossRef CAS.
- Y. Liu, T. Hanaoka, K. Murata, K. Okabe, I. Takahara and K. Sakanishi, React. Kinet. Catal. Lett., 2007, 92, 147 CrossRef CAS.
- M. Zimowska, A. Michalik-Zym, J. Kryſciak-Czerwenka, R. Dula, R. P. Socha, K. Pamin, M. Bazarnik, K. Bahranowski, Z. Olejniczak, L. Lityſska-Dobrzyſska and E. M. Serwicka, Mater. Res. Bull., 2016, 83, 623 CrossRef CAS.
- R. Rafieenezhad, A. Izadbakhsh and A. M. Sanati, J. Porous Mater., 2021, 28, 1749 CrossRef CAS.
- O. Jakdetchai, N. Takayama and T. Nakajima, Kinet. Catal., 2005, 46, 56 CrossRef CAS.
- M. Samari, S. Zinadini, A. A. Zinatizadeh, M. Jafarzadeh and F. Gholami, J. Environ. Chem. Eng., 2021, 9, 105386 CrossRef CAS.
- S. Hashemi-Uderji, M. Abdollahi-Alibeik and R. Ranjbar-Karimi, J. Porous Mater., 2019, 26, 467 CrossRef CAS.
- S. Hashemi-Uderji and M. Abdollahi-Alibeik, J. Iran. Chem. Soc., 2018, 15, 1709 CrossRef CAS.
- F. Gholamian, M. Hajjami and A. M. Sanati, Silicon, 2019, 12, 2121 CrossRef.
- H. Yazdani, S. E. Hooshmand and M. H. Stenzel, ACS Sustainable Chem. Eng., 2022, 10, 4359 CrossRef CAS.
- M. T. Nazeri, T. Nasiriani, H. Farhid, S. Javanbakht, F. Bahri, M. Shadi and A. Shaabani, ACS Sustainable Chem. Eng., 2022, 10, 8115 CrossRef CAS.
- M. A. Ghasemzadeh, B. Mirhosseini-Eshkevari, M. Tavakoli and F. Zamani, Green Chem., 2020, 22, 7265 RSC.
- R. Semwal, C. Ravi, S. Saxena and S. Adimurthy, J. Org. Chem., 2019, 84, 14151 CrossRef CAS PubMed.
- E. Martínez de Marigorta, J. M. de Los Santos, A. M. Ochoa de Retana, J. Vicario and F. Palacios, Beilstein J. Org. Chem., 2019, 15, 1065 CrossRef PubMed.
- B. Borah and L. R. Chowhan, RSC Adv., 2022, 12, 14022 RSC.
- M. Mashhadinezhad, F. Shirini, M. Mamaghani and M. Rassa, Polycyclic Aromat. Compd., 2018, 40, 1417 CrossRef.
- A. Shaabani, A. Rahmati, A. H. Rezayan, M. Darvishi, Z. Badri and A. Sarvari, Mol. Inf., 2007, 26, 973 CAS.
- T. P. Selvam, C. R. James, P. V. Dniandev and S. K. Valzita, Res. Pharm., 2015, 2, 1 Search PubMed.
- N. Kerru, L. Gummidi, S. Maddila and S. B. Jonnalagadda, Curr. Org. Chem., 2021, 25, 4 CAS.
- D. Ye, H. Lu, Y. He, Z. Zheng, J. Wu and H. Wei, Nat. Commun., 2022, 13, 1 Search PubMed.
- P. Z. Dong, B. Qiu, X. D. An and J. Xiao, Green Chem., 2022, 24, 3772 RSC.
- M. A. Castro, A. M. Gamito, V. Tangarife-Castaño, V. Roa-Linares, J. M. Miguel del Corral, A. C. Mesa-Arango, L. Betancur-Galvis, A. M. Francesch and A. S. Feliciano, RSC Adv., 2015, 5, 1244 RSC.
- S. Gazzotti, G. Rainoldi and A. Silvani, Drug Discovery, 2019, 14, 639 CAS.
- T. Budragchaa, B. Westermann and L. A. Wessjohann, Bioorg. Med. Chem., 2019, 27, 3237 CrossRef CAS PubMed.
- E. Ruijter and R. Orru, Drug Discovery Today: Technol., 2018, 29, 1 CrossRef PubMed.
- S. Nadar and T. Khan, Chem. Biol. Drug Des., 2021, 1 Search PubMed.
- X. Jiang, B. Pan, X. Qian, H. Liang, Y. Zhang, B. Chen, X. He, H. Shan Chan, A. S. C. Chan and L. Qiu, Adv. Synth. Catal., 2021, 363, 1 CrossRef.
- S. Wang, X. H. Yuan, S. Q. Wang, W. Zhao, X. B. Chen and B. Yu, Eur. J. Med. Chem., 2021, 214, 113218 CrossRef CAS PubMed.
- M. A. Ameen, E. K. Ahmed, H. I. Mahmoud and M. Ramadan, J. Heterocycl. Chem., 2019, 56, 1831 CrossRef CAS.
- R. D. Simone, M. G. Chini, I. Bruno, R. Riccio, D. Mueller, O. Werz and G. Bifulco, J. Med. Chem., 2011, 54, 1565 CrossRef PubMed.
- Y. Peng, H. Tao, Y. Gao, Y. Yang and Z. Chen, Curr. Top. Med. Chem., 2021, 21, 404 CrossRef CAS PubMed.
- A. Bistrović Popov, R. Vianelo, P. Grbčic, M. Sedić, S. Kraljević Pavelić, K. Pavelić and S. Raić-Malić, Molecules, 2021, 26, 3334 CrossRef PubMed.
- S. Das, New J. Chem., 2020, 44, 17148 RSC.
- P. C. Settipalli, Y. P. Reddy, V. B. Gudise and S. Anwar, ChemistrySelect, 2021, 6, 47 CrossRef CAS.
- J. Safari, M. Tavakoli and M. A. Ghasemzadeh, J. Organomet. Chem., 2019, 880, 75 CrossRef CAS.
- M. Bayat, F. Sadat Hosseini and B. Notash, Tetrahedron, 2017, 73, 1196 CrossRef CAS.
- S. M. Yang, Y. L. Tsai, G. M. Reddy, L. Möhlmann and W. Lin, J. Org. Chem., 2017, 82, 9182 CrossRef CAS PubMed.
- P. N. Sudhan, M. Ghashang and S. S. Mansoor, J. Basic Appl., 2016, 5, 340 Search PubMed.
- J. Cao, R. G. Shi, J. Sun, D. Liu, R. Liu, X. N. Xia, Y. Wang and C. G. Yan, J. Org. Chem., 2019, 85, 2168 CrossRef PubMed.
- J. E. Gholtash and M. Farahi, RSC Adv., 2018, 8, 40962 RSC.
- S. Akrami, B. Karami and M. Farahi, RSC Adv., 2017, 7, 34315 RSC.
- R. Keshavarz and M. Farahi, RSC Adv., 2022, 12, 3584 RSC.
- K. Fattahi, M. Farahi, B. Karami and R. Keshavarz, Bulg. Chem. Commun., 2021, 53, 174 Search PubMed.
- B. Karami, M. Farahi, S. Akrami and D. Elhamifar, New J. Chem., 2018, 42, 12811 RSC.
- M. Farahi, B. Karami, R. Keshavarz and F. Khosravian, RSC Adv., 2017, 7, 46644 RSC.
- H. Mohamadi-Tanuraghaj and M. Farahi, New J. Chem., 2019, 43, 4823 RSC.
- I. Sedighimehr, B. Karami and M. Farahi, Chem. Nat. Compd., 2022, 58, 249 CrossRef CAS.
- J. Jung, J. A. Kim, J. K. Suh, J. M. Lee and S. K. Ryu, Water Res., 2001, 35, 937 CrossRef CAS PubMed.
- H. Araki, A. Fukuoka, Y. Sakamoto, S. Inagaki, N. Sugimoto, Y. Fukushima and M. Ichikawa, J. Mol. Catal. A: Chem., 2003, 199, 95 CrossRef CAS.
- H. Araki, A. Fukuoka, Y. Sakamoto, S. Inagaki, N. Sugimoto, Y. Fukushima and M. Ichikawa, J. Mol. Catal. A: Chem., 2003, 199, 95 CrossRef CAS.
- M. Yang, Z. Wang, W. Wang and C. Liu, Nanoscale Res. Lett., 2014, 9, 1 CrossRef CAS PubMed.
- Y. Fatahi, A. Ghaempanah, L. Ma'mani, M. Mahdavi and S. Bahadorikhalili, J. Organomet. Chem., 2021, 936, 121711 CrossRef CAS.
|
This journal is © The Royal Society of Chemistry 2022 |