DOI:
10.1039/D2RA04270C
(Paper)
RSC Adv., 2022,
12, 25118-25122
Insight into systematic formation of hexafluorosilicate during crystallization via self-assembly in a glass vessel†
Received
11th July 2022
, Accepted 29th August 2022
First published on 5th September 2022
Abstract
Formation of the unexpected hexafluorosilicate (SiF62−) anion during crystallization via self-assembly in glassware is scrutinized. Self-assembly of M(BF4)2 (M2+ = Cu2+ and Zn2+) with tridentate N-donors (L) in a mixture solvent including methanol in a glass vessel gives rise to an SiF62−-encapsulated Cu3L4 double-decker cage and a Zn2L4 cage, respectively. Induced reaction of CuX2 (X− = PF6− and SbF6−) instead of Cu(BF4)2, with the tridentate ligands, produces the same species. The formation time of SiF62− is in the order of anions BF4− < PF6− < SbF6− under the given reaction conditions. The SiF62− anion, acting as a cage template or cage-to-cage bridge, seems to be formed from the reaction of polyatomic anions containing fluoride with the SiO2 of the surface of the glass vessel.
Introduction
Anion-related chemistry is a rapidly burgeoning field owing to the timeliness and importance including environmental pollution, industrial chemicals, biological processes, ionic liquids, lithium batteries, templates, ion-pairing, and health.1–12 Some anions play a significant role in the construction and behaviors of desirable molecular architectures based on negative charge, size, shape, solvents, and pH.13–18 In particular, F-containing polyatomic anions such as BF4−, PF6−, and SbF6− have been extensively employed for various purposes such as important chemical phenomena, cage-templates, coordination, and the Hofmeister series;19–26 however, they are known to be not thermodynamically stable depending on conditions27,28 relative to the F-free polyatomic anions NO3−, ClO4−, CH3CO2−, and SO42−. For instance, the PF6− anion could be converted to PFn(OH)6−n− during the complexation of Pd(II)-L.29 Furthermore, BF4− is known to be changed to the SiF62− anion via subsequent reaction of dissociated F− with the Si–O moieties of a glass vessel or stoneware.30–35 Hexafluorosilicate (SiF62−) is a stable octahedral anion with six Si–F bond distances of 1.71 Å,36 and its solubility is significantly dependent on cations.37 Furthermore, the dianion species have seen the following diverse uses: leather and wood preservation agents, fluoridation agents for drinking water, two-solution fluoride mouth rinse, commercial laundry, enamels/enamel frits for china and porcelain, opalescent glass, metallurgy of aluminum and beryllium, glue, ore flotation, insecticides and rodenticides, fluorinating agent in organic synthesis, and water fluoridation.38–47
In this context, systematic research on the unusual formation process and recognition of SiF62− is very much in demand. Thus, herein, formation of hexafluorosilicate via conversion of PF6− and SbF6− beside BF4− during self-assembly or recrystallization in a glass vessel is scrutinized. This paper reports the unique SiF62−-encapsulated cages constructed via self-assembly of M2+ (M = Cu2+ and Zn2+) with tridentate N-donor ligands in a glass vessel. To our knowledge, this article presents clear systematic research on the formation of hexafluorosilicate by reactions of glassware with F-containing polyatomic anions.
Experimental
Materials and measurements
All of the chemicals, including copper(II) tetrafluoroborate (Cu(BF4)2), copper(II) nitrate (Cu(NO3)2), copper(II) chloride (CuCl2), silver(I) hexafluorophosphate (AgPF6), silver(I) hexafluoroantimonate (AgSbF6), and zinc(II) tetrafluoroborate (Zn(BF4)2), were purchased from Aldrich and used without further purification. (1S,1′S,1′′S,2R,2′R,2′′R)-(Benzenetricarbonyltris(azanediyl))tris(2,3-dihydro-1H-indene-2,1-diyl)triisonicotinate (L1) and 1,3,5-tris(isonicotinoyloxy-methyl)benzene (L2) were synthesized according to the literature,48,49 respectively. Glass vessels (Hubena Co, Seongnam, Korea) were employed in all of the self-assembly reactions. The 1H and 19F NMR spectra were recorded on a Varian Mercury Plus operating at 400 MHz. The infrared (IR) spectra were obtained on a Nicolet 380 FT-IR spectrophotometer with samples prepared as KBr pellets. Elemental microanalyses (C, H, N) were performed on solid samples at the Pusan Center, KBSI, using a Vario-EL III. Thermal analyses were carried out under a dinitrogen atmosphere at a scan rate of 10°C min−1 using a Labsys TGA-DSC 1600.
[(SiF6)2@Cu3L14](SiF6)·16CH3OH
Method 1. A methanol solution (2.0 mL) of copper(II) tetrafluoroborate (4.7 mg, 0.02 mmol) was carefully layered onto a dichloromethane solution (2.0 mL) of L1 (18.4 mg, 0.02 mmol). After 2 weeks, blue crystals suitable for single crystal X-ray structure determination were obtained in an 83% yield.
Method 2. A methanol solution (1.0 mL) of silver(I) hexafluorophosphate (10.1 mg, 0.04 mmol) was added to copper(II) chloride (2.7 mg, 0.02 mmol) dispersed in methanol at room temperature. The reaction mixture was stirred for 30 min, after which, the precipitated silver chloride was filtered off. The methanol solution of Cu(PF6)2 was carefully layered onto a dichloromethane solution (2.0 mL) of L1 (18.4 mg, 0.02 mmol). After 4 weeks, blue crystals suitable for single crystal X-ray structure determination were obtained in a 78% yield.
Method 3. A methanol solution (1.0 mL) of silver(I) hexafluoroantimonate (13.7 mg, 0.04 mmol) was added to a suspension of copper(II) chloride (2.7 mg, 0.02 mmol) dispersed in methanol at room temperature. The reaction mixture was stirred for 30 min, after which, the precipitated silver chloride was filtered off. The methanol solution of Cu(SbF6)2 was carefully layered onto a dichloromethane solution (2.0 mL) of L1 (18.4 mg, 0.02 mmol). After 6 weeks, blue crystals suitable for single crystal X-ray structure determination were obtained in a 66% yield.
Method 4. A methanol solution (2.0 mL) of copper(II) nitrate (2.8 mg, 0.015 mmol), a dichloromethane solution (4.0 mL) of L1 (18.4 mg, 0.02 mmol), and an aqueous solution (0.1 mL) of (NH4)2SiF6 (3.6 mg, 0.02 mmol) were left at room temperature, and after 1 day, blue crystals suitable for single crystal X-ray structure determination were obtained in a 62% yield. m. p. 280 °C (dec). Anal. Calcd for: C, 58.55; H, 4.65; N, 7.19%. Found: C, 58.40; H, 4.52; N, 7.23%. IR (KBr pellet, cm−1): 3294(br), 1733(s), 1661(s), 1524(s), 1425(m), 1329(m), 1286(s), 1179(w), 1127(s), 1063(m), 1032(m), 859(w), 756(s), 705(s), 472(w).
[(SiF6)@Zn2L24](SiF6)·2C4H8O·4CH2Cl2
A mixture of tetrahydrofuran with a methanol solution (3.0 mL/1.0 mL) of zinc(II) tetrafluoroborate (9.6 mg, 0.04 mmol) and a dichloromethane solution (3.0 mL) of L2 (14.5 mg, 0.04 mmol) were left at room temperature, and after 2 weeks, blue crystals suitable for single crystal X-ray structure determination were obtained in a 67% yield. m. p. 260 °C (dec). Anal. Calcd for: C, 50.88; H, 3.84; N, 5.93%. Found: C, 50.79; H, 3.81; N, 5.97%. IR (KBr pellet, cm−1): 3425(br), 1729(s), 1616(w), 1564(w) 1409(m), 1373(w) 1326(m), 1280(s), 1224(w), 1122(s), 1062(m), 1024(w), 862(m), 759(s), 705(s), 468(w).
Single crystal X-ray structure determination
The X-ray diffraction data for [(SiF6)2@Cu3L14](SiF6)·16CH3OH were measured at 100 K with synchrotron radiation (λ = 0.76000 Å) on a Rayonix MX225HS detector at 2D SMC with a silicon (111) double-crystal monochromator (DCM) at the Pohang Accelerator Laboratory (PAL), Korea. The PAL BL2D-SMDC program50 was used for data collection (detector distance: 66 mm, omega scan: Δω = 3°, exposure time: 1 s per frame), and HKL3000sm (ver. 703r)51 was used for cell refinement, reduction, and absorption correction. X-ray data on [(SiF6)@Zn2L24](SiF6)·2C4H8O·4CH2Cl2 were collected on a Bruker SMART automatic diffractometer with graphite-monochromated Mo Kα radiation (λ = 0.71073 Å). Thirty-six (36) frames of 2D diffraction images were collected and processed to obtain the cell parameters and orientation matrix. The data were corrected for Lorentz and polarization effects. The absorption effects were corrected using the multi-scan method (SADABS).52 The structures were resolved using the direct method (SHELXS) and refined by full-matrix least squares techniques (SHELXL 2018/3).53 The non-hydrogen atoms were refined anisotropically, and the hydrogen atoms were placed in calculated positions and refined only for the isotropic thermal factors. The crystal parameters and procedural information corresponding to the data collection and structure refinement are listed in Table 1.
Table 1 Selected bond lengths (Å) and angles (°) for [(SiF6)2@Cu3L14](SiF6)·16CH3OH, and [(SiF6)@Zn2L24](SiF6)·2C4H8O·4CH2Cl2
[(SiF6)2@Cu3L14](SiF6)·16CH3OH |
[(SiF6)@Zn2L24](SiF6)·2C4H8O·4CH2Cl2 |
x − 1, y + 1, z, x + 1, y − 1, z. x + 1, y, z − 1. −x + 1/2, −y + 1/2, z |
Cu(1)–N(6) |
2.007(4) |
Zn(1)–N(1) |
2.115(4) |
Cu(1)–N(3) |
2.019(3) |
Zn(1)–F(4) |
2.122(5) |
Cu(1)–F(5) |
2.317(2) |
Zn(1)–F(3) |
2.361(5) |
N(6)a–Cu(1)–N(6) |
87.5(2) |
N(1)–Zn(1)–N(1)a |
89.952(6) |
N(6)a–Cu(1)–N(3)a |
176.4(3) |
N(1)a–Zn(1)–N(1)b |
176.7(2) |
N(6)–Cu(1)–N(3)a |
91.2(2) |
N(1)a–Zn(1)–N(1)c |
89.953(6) |
N(6)–Cu(1)–N(3) |
176.4(2) |
N(1)–Zn(1)–F(4) |
91.6(1) |
N(3)a–Cu(1)–N(3) |
90.3(3) |
N(1)–Zn(1)–F(3) |
88.4(1) |
|
|
F(4)–Zn(1)–F(3) |
180.0 |
Results and discussion
Synthesis aspects
Self-assembly of Cu(BF4)2 with L1 in a mixture of methanol and dichloromethane produced crystals consisting of [(SiF6)2@Cu3L14](SiF6)·16CH3OH suitable for single crystal X-ray structure determination after 2 weeks, as shown in Scheme 1. Moreover, the reactions with Cu(PF6)2 and Cu(SbF6)2 instead of Cu(BF4)2 gave rise to the same product after significant durations, specifically 4 and 6 weeks, respectively. The reaction time may be owed to the stability of the BF4− < PF6− < SbF6− anions under the given reaction conditions. As expected, self-assembly of Cu(BF4)2 with L1 in the presence of (NH4)2SiF6 produced blue crystals within 1 day. In order to investigate the metal effects, self-assembly of Zn(BF4)2 instead of Cu(BF4)2 with L2 was performed, which generated crystals of [(SiF6)@Zn2L24](SiF6)·2C4H8O·4CH2Cl2 after 2 weeks, indicating that the central metal cation is not a significant factor in anion transformation in a glass vessel. For all of the reactions, the SiF62− anion acted either as a template by which to form the cage products or as a cage-to-cage bridge.
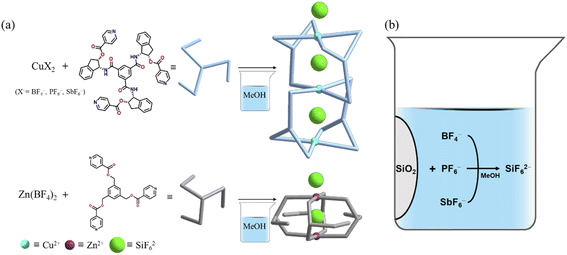 |
| Scheme 1 Construction of cages (a) via SiF62− formation (b) during self-assembly in a glass vessel. | |
Both crystalline products are stable under anaerobic condition at room temperature. The crystals are insoluble in common organic solvents such as acetone, chloroform, toluene, benzene and tetrahydrofuran, but are partially dissociated in dimethyl sulfoxide and N,N-dimethylformamide. Their compositions and structures were confirmed by elemental analyses, IR-spectral analysis (Fig. S1 and S2†), thermal analysis (Fig. S3†), 1H NMR (Fig. S4, as dissoociated in Me2SO-d6†), and single-crystal X-ray crystallography. The characteristic IR bands of SiF62− were easily assigned. The thermogravimetric analysis (TGA) and differential scanning calorimetry (DSC) showed [(SiF6)2@Cu3L14](SiF6)·16CH3OH and [(SiF6)@Zn2L24](SiF6)·2C4H8O·4CH2Cl2 to be stable up to 277 and 257 °C, respectively. Evaporation of the solvate molecules of the two kinds of crystals occurred in the 10–60 and 20–135 °C temperature ranges, respectively (Fig. S3†).
Crystal structures
The crystal structure of [(SiF6)2@Cu3L14](SiF6)·16CH3OH is depicted in Fig. 1, and its bond lengths and angles are listed in Table 1. The geometry of each copper(II) ion is an octahedral N4F2 coordination arrangement with four N donors from four L1s and F donors from two SiF62− anions (Cu–N = 2.007(4) − 2.019(3) Å; Cu–F(encapsulated) = 2.317(2), 2.300(3) Å; Cu–F(bridged) = 2.357(3) Å) in axial positions, resulting in the formation of the Cu3L4 double-decker occupied by two SiF62− anions. Concomitantly, for the nestled SiF62−, the equatorial Si–F bond lengths (1.628(7) − 1.684(7) Å) are comparable to the axial Si–F lengths (1.666(3) and 1.693(3) Å). Thus, the ligand is coordinated to two Cu(II) ions in a bidentate and a monodentate fashion, respectively. The intra-cage Cu⋯Cu distance is 7.968(2) Å. Its packing structure is in the linear double-decker⋯SiF62−⋯double-decker⋯SiF62− mode (Fig. S5†). The crystal structure of [(SiF6)@Zn2L24](SiF6)·2C4H8O·4CH2Cl2 is a typical Zn2L4 cage, as depicted in Fig. 2, and the coordination geometry of the Zn(II) ion is an octahedral arrangement with two SiF62− groups in the trans position (Zn–F = 2.095(5) − 2.273(5) Å; F–Zn–F′ = 180.0(0)°) and four pyridine N donors of four L2s on the basal plane. Each L connects two zinc(II) ions in a bidentate mode with a free pyridyl donor. Concomitantly, for the nestled SiF62−, the equatorial Si–F bond lengths (1.637(4) Å) are slightly shorter than the axial Si–F lengths (1.733(5) – 1.746(6) Å). The intra-cage Zn⋯Zn distance is 8.113(2) Å. Its packing structure is in the linear cage⋯SiF62−⋯ cage⋯SiF62− mode (Fig. S5†). The solvate molecules of [(SiF6)2@Cu3L14](SiF6)·16CH3OH were squeezed. The volumes of solvate molecules in [(SiF6)2@Cu3L14](SiF6)·16CH3OH and [(SiF6)@Zn2L24](SiF6)·2C4H8O·4CH2Cl2 were 56.2% (10
282 Å3/18
304 Å3) and 37.8% (2093.4 Å3/5539.5 Å3), respectively, on the basis of a PLATON/SOLV calculation.54
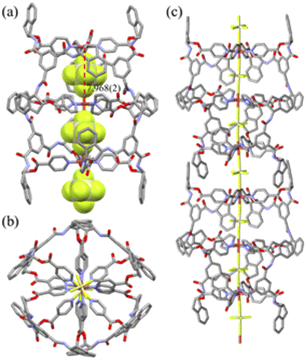 |
| Fig. 1 Side view (a), top view (b), and packing structure (c) of double-decker, [(SiF6)2@Cu3L14](SiF6)·16CH3OH. The nestled and outside bridged SiF62− anions were clearly depicted in light green color. | |
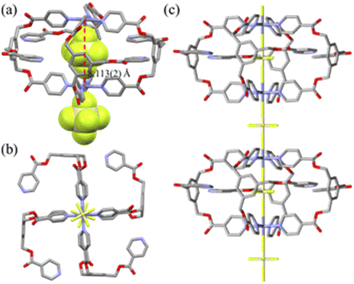 |
| Fig. 2 Partial crystal structures of [Ag(s,r-L)](PF6)·3C4H8O2·0.5H2O (a) and [Ag(r,s-L)](PF6)·3C4H8O2·0.5H2O (b). The solvate molecules were omitted for clarity. | |
Discussion
Formation of SiF62− during self-assembly of more than 2 weeks' duration requires some explanation, because the anion was not added to the self-assembly solution in a glass vessel. It should be noted that the SiF62− anion replaces the starting BF4− anion in the self-assembly reaction and that it is formed after extracting Si4+ from the glass vessel in methanol solvent—a rare but well-known phenomenon.55 The generation of SiF62− by slow release of Si4+ from the glass vessel in the methanol solution was clearly confirmed, and the resultant anions were separated as counteranions. The SiF62− anions appear to play significant roles as both encapsulator and bridge in the construction of the cage skeleton. The stable anion, by contrast, forms as a consequence of the hydrolysis of the BF4− anion and subsequent reaction of F− with the Si–O moieties of the glass vessel56,57 in which a methanol solution of M(BF4)2 is kept for self-assembly or crystallization. The formation of SiF62− seems to be promoted by the presence of a small quantity of H2O in addition to a mixture solvent including methanol. Formation of SiF62− from BF4− and PF6− in a glass vessel in water has been observed by other groups,30–32,56 but that of SiF62− from SbF6− heretofore has not been recorded. The present self-assembly of MX2 (M2+ = Cu2+, Zn2+; X− = BF4−, PF6−, SbF6−) with each tridentate ligand in each glass vessel systematically transformed BF4−, PF6− or SbF6− into the SiF62− anion according to the following eqn (1): |
SiO2 + 6HF → SiF62− + 2H3O+
| (1) |
Of course, formation of SiF62− from CF3SO3− (C–F compound) could not be carried out in a similar reaction performed in a polypropylene vessel instead of a glass one; that is, the reaction did not yield crystals containing the SiF62− anion.
The presence of SiF62− in Me2SO-d6 was confirmed by 19F NMR spectra (Fig. S6†). The SiF62− occurred as a singlet at δ = −134 ppm. Spectra taken of the sample preparation one month later indicated that almost all of the original BF4− anions had been converted to SiF62− anions. As regards the formation of SiF62− from PF6− and SbF6− anions, PF6− (−70.8 ppm) and SbF6− chemical shifts (−119.9 ppm) disappeared after 4 and 6 weeks, respectively, and appeared at the position of the SiF62− anion. As for construction of the coordination architecture, insight into the stability and geometry of a specific anion as a template is useful to the tuning of the shape and topology of coordination species. In the present study, the encapsulated and bridged SiF62− anions helped to stabilize the crystal structure of the cages. Certainly, transformation of polyatomic anions and their role is a topic worthy of further investigation.
Conclusions
In summary, transformation of polyatomic anions BF4−, PF6−, and SbF6− into SiF62− during self-assembly or recrystallization in a glass vessel was systematically confirmed. This shows that the surface of regular laboratory glassware should be given serious consideration for long-duration reactions or self-assembly with F− species. Further studies on other means of anion-bridging or -encapsulation of cage units will contribute to the development of task-specific polyatomic anions for recognition as well as environmental molecular materials such as adsorbents and sensing materials.
Conflicts of interest
There are no conflicts to declare.
Acknowledgements
This work was supported by National Research Foundation of Korea (NRF) grants funded by the Korean Government [MEST] (2021R1A2C2005105, 2016R1A5A1009405 (OSJ)), and 2021R1I1A3059982 (YAL)). The X-ray crystallography at the PLS-II 2D-SMC beamline was supported in part by MSIP and POSTECH.
Notes and references
- J. L. Anthony, J. L. Anderson, E. J. Maginn and J. F. Brennecke, J. Phys. Chem. B, 2005, 109, 6366–6374 CrossRef CAS PubMed.
- Z. Wang, X. Li, W. Guo and Y. Fu, Adv. Funct. Mater., 2021, 31, 2009875 CrossRef CAS.
- J. P. Carpenter, C. T. McTernan, T. K. Ronson and J. R. Nitschke, J. Am. Chem. Soc., 2019, 141, 11409–11413 CrossRef CAS PubMed.
- P. A. Gale, R. Perez-Tomas and R. Quesada, Acc. Chem. Res., 2013, 46, 2801–2813 CrossRef CAS PubMed.
- D. H. Shin, M. Kim, Y. Kim, I. Jun, J. Jung, J. H. Nam, M. H. Cheng and M. G. Lee, Pflugers Arch. - Eur. J. Physiol., 2020, 472, 1003–1018 CrossRef CAS PubMed.
- Y. Zhao, Y. Li, S. Yuan, J. Zhu, S. Houtmeyers, J. Li, R. Dewil, C. Gao and B. Van der Bruggen, J. Mater. Chem. A, 2019, 7, 6348–6356 RSC.
- Y. Zhao, Y. Cotelle, L. Liu, J. López-Andarias, A.-B. Bornhof, M. Akamatsu, N. Sakai and S. Matile, Acc. Chem. Res., 2018, 51, 2255–2263 CrossRef CAS PubMed.
- Y. Bando, Y. Haketa, T. Sakurai, W. Matsuda, S. Seki, H. Takaya and H. Maeda, Chem. Eur. J., 2016, 22, 7843–7850 CrossRef CAS PubMed.
- S. Patnaik, D. P. Sahoo and K. Parida, Carbon, 2021, 172, 682–711 CrossRef CAS.
- Y. Kim, P. Kang, Y. Jeon, H. M. Cho and M.-G. Choi, Bull. Korean Chem. Soc., 2021, 42, 240–244 CrossRef CAS.
- D. H. Sohn, T. Ohn, E. Han, A. B. Atar, S. J. Cho and J. Kang, Bull. Korean Chem. Soc., 2021, 42, 193–199 CrossRef CAS.
- J. Lee, Y. Hong, S. Yeon, D. Moon and T.-S. You, Bull. Korean Chem. Soc., 2021, 42, 563–566 CrossRef CAS.
- Z. Xu, X. Chen, R. Chen, X. Li and H. Zhu, Npj Comput. Mater., 2020, 6, 1–8 CrossRef.
- D. Liu, J. Wang, Y. Wang and Y. Zhu, Catal. Sci. Technol., 2018, 8, 3278–3285 RSC.
- J.-J. Liu, S.-B. Xia, Y.-L. Duan, T. Liu, F.-X. Cheng and C.-K. Sun, Polymers, 2018, 10, 165 CrossRef PubMed.
- G.-B. Li, Z. Zhang, L.-S. Liao, R.-K. Pan and S.-G. Liu, Spectrochim. Acta, Part A, 2021, 254, 119588 CrossRef CAS PubMed.
- A. Caballero, F. Zapata and P. D. Beer, Coord. Chem. Rev., 2013, 257, 2434–2455 CrossRef CAS.
- J. E. Wong, H. Zastrow, W. Jaeger and R. von Klitzing, Langmuir, 2009, 25, 14061–14070 CrossRef CAS PubMed.
- X. He, K. Zhang, Y. Liu, F. Wu, P. Yu and L. Mao, Angew. chem., 2018, 130, 4680–4683 CrossRef.
- G. Sarada, A. Kim, D. Kim and O.-S. Jung, Dalton Trans., 2020, 49, 6183–6190 RSC.
- J. Lee, S. Lim, D. Kim, O.-S. Jung and Y.-A. Lee, Dalton Trans., 2020, 49, 15002–15008 RSC.
- S.-i. Noro and T. Nakamura, NPG Asia Mater., 2017, 9, e433 CrossRef.
- M. Takeuchi, N. Matubayasi, Y. Kameda, B. Minofar, S.-i. Ishiguro and Y. Umebayashi, J. Phys. Chem. B, 2012, 116, 6476–6487 CrossRef CAS PubMed.
- A. Pramanik, D. R. Powell, B. M. Wong and M. A. Hossain, Inorg. Chem., 2012, 51, 4274–4284 CrossRef CAS PubMed.
- D. Kim, G. Gwak, J. Han, D. Kim and O.-S. Jung, Dalton Trans., 2022, 51, 5810–5817 RSC.
- J. Jaganathan, M. Sivapragasam and C. Wilfred, J. Chem. Eng. Process Technol., 2016, 7, 1–6 Search PubMed.
- R. P. Swatloski, J. D. Holbrey and R. D. Rogers, Green Chem., 2003, 5, 361–363 RSC.
- C. A. Reed, Chem. N. Z., 2011, 75, 174–179 CAS.
- T. H. Noh, H. Lee, Y.-A. Lee and O.-S. Jung, Inorg. Chim. Acta, 2013, 405, 9–14 CrossRef CAS.
- M.-Y. Sun, X.-Z. Wang, Z.-Y. Chen, X.-P. Zhou and D. Li, Inorg. Chem., 2019, 58, 12501–12505 CrossRef CAS PubMed.
- M. Mizuhata, Y. Saito, M. Takee and S. Deki, J. Ceram. Soc. Jpn., 2009, 117, 335–339 CrossRef CAS.
- D. L. Reger, A. E. Pascui and M. D. Smith, Eur. J. Inorg. Chem., 2012, 2012, 4593–4604 CrossRef CAS.
- A. Galstyan, W. Z. Shen, E. Freisinger, H. Alkam, W. Hiller, P. J. Sanz Miguel, M. Schürmann and B. Lippert, Chem. Eur. J., 2011, 17, 10771–10780 CrossRef CAS PubMed.
- W. Chen, J. Chu, I. Mutikainen, J. Reedijk, U. Turpeinen and Y.-F. Song, CrystEngComm, 2011, 13, 7299–7304 RSC.
- M. M. Nielsen and C. M. Pedersen, Chem. Sci., 2022 Search PubMed.
- E. Wiberg, A. F. Holleman and N. Wiberg, Inorganic chemistry, Academic press, 2001 Search PubMed.
- J. Frayret, A. Castetbon, G. Trouve and M. Potin-Gautier, Chem. Phys. Lett., 2006, 427, 356–364 CrossRef CAS.
- G. O. Bianchetti, C. L. Devlin and K. R. Seddon, RSC Adv., 2015, 5, 65365–65384 RSC.
- E. Urbansky and M. Schock, Int. J. Environ. Sci., 2000, 57, 597–637 CAS.
- H. Amouri, C. Desmarets and J. Moussa, Chem. Rev., 2012, 112, 2015–2041 CrossRef CAS PubMed.
- M. Shindo and T. Iwata, Synlett, 2022, 33, 531–545 CrossRef CAS.
- C. M. Thompson, Dianion chemistry in organic synthesis, CRC press, 1994 Search PubMed.
- T. W. Hudnall, C.-W. Chiu and F. P. Gabbai, Acc. Chem. Res., 2009, 42, 388–397 CrossRef CAS PubMed.
- G. Derkson, P. Poon and A. Richardson, J. Dent. Res., 1982, 61, 660–664 CrossRef CAS PubMed.
- L. C. Chow, S. Takagi, C. M. Carey and B. Sieck, J. Dent. Res., 2000, 79, 991–995 CrossRef CAS PubMed.
- R. P. Sharma, R. Sharma, R. Bala, U. Rychlewska, B. Warzajtis and V. Ferretti, J. Mol. Struct., 2005, 753, 182–189 CrossRef CAS.
- J. Lusher and F. Sebba, J. Appl. Chem., 1966, 16, 129–132 CrossRef CAS.
- D. Kim, K.-D. Seo, Y.-B. Shim, K. Lee, S. H. Lee, Y.-A. Lee and O.-S. Jung, Dalton Trans., 2022, 51, 6046–6052 RSC.
- D. Choi, H. Lee, J. J. Lee and O.-S. Jung, Cryst. Growth Des., 2017, 17, 6677–6683 CrossRef CAS.
- J. W. Shin, K. Eom and D. Moon, J. Synchrotron Radiat., 2016, 23, 369–373 CrossRef PubMed.
- Z. Otwinowski and W. Minor, Methods in Enzymology, ed. C. W. Carter Jr. and R. M. Sweet, Academic Press, New York, 1997, 276, p. 307 Search PubMed.
- G. M. S. A. D. A. B. S. Sheldrick, A program for Empirical Absorption Correction of Area Detector Data, University of Göttingen, Germany, 1996 Search PubMed.
- G. M. Sheldrick, Acta Crystallogr., Sect. C: Struct. Chem., 2015, 71, 3–8 Search PubMed.
- A. Spek, Acta Crystallogr., Sect. D: Biol. Crystallogr., 2009, 65, 148–155 CrossRef CAS PubMed.
- B. O. Mysen and D. Virgo, Chem. Geol., 1986, 57, 303–331 CrossRef CAS.
- J. Y. Wu, M. S. Zhong and M. H. Chiang, Chem. Eur. J., 2017, 23, 15957–15965 CrossRef CAS PubMed.
- J. Jia, A. J. Blake, N. R. Champness, P. Hubberstey, C. Wilson and M. Schroder, Inorg. Chem., 2008, 47, 8652–8664 CrossRef CAS PubMed.
|
This journal is © The Royal Society of Chemistry 2022 |
Click here to see how this site uses Cookies. View our privacy policy here.