DOI:
10.1039/D2RA03906K
(Paper)
RSC Adv., 2022,
12, 23898-23911
Removal of hexavalent chromium ions using micellar modified adsorbent: isothermal and kinetic investigations
Received
24th June 2022
, Accepted 15th August 2022
First published on 24th August 2022
Abstract
Hexavalent chromium is a very poisonous oxyanion and has had a negative impact on human health. This study assessed the viability of removing chromium(VI) using micellar modified adsorbents. In this study, chromium(VI) was removed from locally accessible wheat bran using separate applications of anionic sodium dodecyl sulfate (SDS) and cationic cetyltrimethylammonium bromide (CTAB) surfactants. The initial chromium content (5–12 ppm), pH (2–12), adsorbent dose (1–6 g/100 mL), agitation time (15–240 min), agitation speed (50–300 rpm), and temperature (15–50 °C) were all varied in the adsorption investigation. Pseudo first-order and second-order kinetic models were utilized to analyze the kinetic investigation. To determine thermodynamic parameters, the van't Hoff relationship was used. The maximum result for chromium(VI) uptake was obtained as 87.7%, 83.5% and 98.9% for WB, SDS-mWB, and CTAB-mWB, respectively, at an agitation time of 240 min (i.e., 4 h), temperature (i.e., 25–30 °C), agitation speed (150 rpm). However, both WB and CTAB-mWB derives metal ion removal at lower pH levels (2–4), whereas SDS-mWB requires a pH between 4 and 6 for maximum percentage removal of Cr(VI). The equilibrium data of WB and SDS-mWB were modeled by the Langmuir adsorption isotherm, while the data of CTAB-mWB fitted well in the Freundlich isotherm model. The kinetic analysis of WB, SDS-mWB, and CTAB-mWB revealed that the pseudo-second-order kinetic model provides a thorough explanation for each of these adsorbents. It was found that CTAB-mWB can preferably be used for the removal of chromium(VI) due to its high affinity with adsorbate molecules and adsorption capacity.
1. Introduction
Due to the limited amount of naturally occurring water resources, water pollution is a more difficult and significant problem than any other type of environmental issue. Due to the unavailability of the latest technologies for waste-water treatment, most of the under-developing and poor nations are at high risk. Numerous different forms of contaminants are poisoning the water supplies, harming aquatic life and threatening the availability of recreational water.1 Heavy metals are highly dangerous, non-biodegradable and environment persistent pollutants as compared to other pollutants. These metals pollute water resources from both anthropogenic and natural sources. Nowadays, researchers are focusing on health risks brought by heavy metal contamination.2,3
There are different sources of contaminants that pollute the water, such as municipal waste, mining, present-day farming practices, marine discard, radioactive disposal, oil spillage, underground stockpiling spillages, and industries. Out of all the above-mentioned resources the major one is industrial waste. Industrial waste may be in the form of solid, liquid, or gas, which directly discharges into the environment. Many industries (i.e. tanning, oil, pharmaceuticals, textile, sugar mill, soap or detergent units, paint and pigments, electroplating units, and fertilizers) discharge numerous toxic chemicals directly into the water, which cause severe effects on flora and fauna.4–7
Small amounts of heavy metals cause some organic processes, while in bulk these become highly toxic. As these are non-biodegradable and pile up at different trophic levels via the food chain and harm human health. These metals multiply the health risk when entered into the human body or accumulate in living tissues. Few of these metals cause only physical distress while others may cause hazardous diseases or may harm the vital body system. Therefore, it is essential to minimize the release of these metals into the environment.8
Chromium has much importance as compared to all other heavy metals, and both of its oxidation forms (Cr(III) and Cr(VI)) are used chiefly in electroplating, leather tanning, metallurgy, paint and pigments, wood preservatives, dyeing, textile, and steel manufacturing. Out of both its oxidation forms, hexavalent form (i.e. Cr(VI)) is one of the unwanted heavy metals as it badly affects human health via accumulating in the food chain and causing different diseases.9–11 Salts of chromium are mostly utilized for tanning purposes, trivalent chromium sulfate ([Cr(H2O)6]2(SO4)3) is one of the most effective and efficient tanning agents.12,13 It was reported that the samples of groundwater from a few districts of Pakistan demonstrated chromium contents in the range from <0.001 to 9.8 mg L−1, the samples of well water collected from Kasur (local area), Punjab province showed maximum results i.e. 2.12 mg L−1,14 though the samples of surface water collected from Bara River (Nowshera), KPK province showed the results in 0.16–0.29 mg L−1 range.15 These findings reflect that industrial wastewater may affect the quality of water. With a few exemptions,16,17 the majority of findings show that the chromium contents in soil are present within the tolerable range of 100–150 mg kg−1, and the average amount of the chromium in world soil has been built up to 60 mg kg−1.9
Diverse techniques, including photocatalysis, membrane separation, biological oxidation, ozonation, adsorption, ion-exchange, oxidation, reverse osmosis, ultra-filtration, electro-dialysis, and electrochemical approaches, have been used to remove colours and trace metals from wastewater.18–22 All of the above-mentioned methods are not very useful because these are best for the isolation of high contents of trace metals from wastewater while not much effective for trace amounts of metals. Some other disadvantages of these methods are partial removal of metals, dependence on pH change, costly hardware and checking framework prerequisites, high energy or reagent necessities, and production of poisonous waste materials that need disposal.23–27 Furthermore, these methods might be ineffectual or incredibly costly when metal contents in wastewater approach the range of 1–100 ppm.28
The utilization of biomass for the removal of metal is viewed as a suitable option in contrast to conventional methods. Biosorption of trace metals is described as the utilization of biomass for the extraction of trace metals from wastewater via metabolically mediated or physicochemical adsorption of metal.29 The very important characteristics of biosorption are low cost and highly efficient for removal of trace metals even at very low concentrations.30 The most commonly used adsorbent is activated carbon. Unconventional adsorbents have been used for the removal of dyes from wastewater, including clay, calcium alginate hydrogel beads,31 guar gum-based hydrogels,32 wheat bran,33,34 walnut shell,35 flyy ash, microalga Spirulina platensis,36 rice straw,37 Cucumis sativus peel,38 crop residues,39 corncob, palm ash,40 barley husk,41 Salix babylonica leaves powder,42 nanomaterials,43,44 and polymer particles.45 Agricultural wastes and by-products, which are inexpensive, environmentally benign, and resource sustainable, are commonly employed for wastewater treatment.46 Table 1 summarizes the findings of certain studies adsorption reported in the literature employed for the removal of chromium VI metal ion.
Table 1 Summary of various adsorbents used for chromium(VI) removal
Adsorbent |
Time [h] |
pH |
T [°C] |
Cr(VI) [mg L−1] |
Dose [g L−1] |
Adsorption capacity [mg g−1] |
Ref. |
Low cost adsorbent |
Rice straw |
1.5 |
2–6 |
27 |
100 |
10 |
3.2 |
48 |
Saw dust |
5.0 |
1–10 |
30 |
20–1000 |
2–24 |
16 |
48 |
Charcoal |
5.0 |
1–10 |
30 |
20–1000 |
2–24 |
17 |
48 |
Kaolinite |
4.0 |
4.6 |
25 |
4.6 |
2000 |
6.1 |
49 |
Red clay |
5.0 |
4–6 |
— |
0.46 |
40 |
1.3 |
50 |
Rice straw |
1.5 |
2–6 |
27 |
100 |
10 |
3.2 |
48 |
Microorganism |
Sargassum dentifolium |
1.0 |
7 |
50 |
100 |
1500 |
41.2 |
51 |
Exiguobacterium sp. ZM-2 |
2.0 |
2.5 |
25 |
100 |
— |
29.8 |
52 |
Saccharomyces cerevisiae and Rhizobium |
3 |
2 |
50 |
— |
— |
24–31 |
53 |
Aspergillus niger |
— |
2 |
40 |
200 |
2 |
97.1 |
54 |
Carbon NM's |
Low cost activated carbon |
6 |
3.5 |
25 |
— |
1 |
74.63 |
55 |
Graphene oxide |
2 |
3.0 |
25 |
— |
— |
49 |
56 |
MWCNT's |
0.5 |
2.0 |
25 |
— |
2–6 |
23–32 |
57 |
Ag2O/saw dust nanocomposites |
2–10 |
3 |
25 |
25–100 |
— |
60.24 |
58 |
Surfactant modified zeolites |
HDTMA-modified zeolites erionite |
7 |
2 |
25 |
200 |
0.1 g/50 mL |
0.103 |
59 |
HDTMA-modified zeolite cowlestie |
7 |
7 |
25 |
200 |
0.1 g/50 mL |
0.763 |
60 |
HDTMA-modified clinoptilolite |
7 |
2–3 |
15 |
200–600 |
20 mg/10 mL |
0.124 |
61 |
ODA-modified clinoptilolite |
— |
7 |
— |
500 |
20 mg/10 mL |
54 |
61 |
Surfactants are basically like short-chain fatty acids and have both hydrophilic and hydrophobic characteristics. They have both polar and nonpolar poles which show an affinity for polar and nonpolar molecules, respectively. The molecules of surfactant form a monolayer and decrease the surface tension of the medium at the interface. In some cases, they behave like a molecule that is capable of forming micelles. They are also known as surface-active agents, amphiphiles, tensides, or paraffin chain salts. Detergents are also surfactants and are used for cleaning purposes. In the aqueous condition surfactant forms two ends, one of them is known as the head (i.e., hydrophilic) and the other is known as the tail (i.e., hydrophobic). They are sorted into different classes e.g., cationic (i.e., trimethyl dodecyl ammonium chloride, lauryl amine hydrochloride, cetyltrimethylammonium bromide), anionic (i.e., sodium stearate, sodium dodecylbenzene sulfonate, sodium dodecyl sulfate), non-ionic (i.e. polyoxyethylene alcohol, polysorbate 80, alkylphenol ethoxylate, propylene oxide-modified polydimethylsiloxane) and zwitterionic (i.e. coco amido-2-hydroxypropyl sulfobetaine, dodecyl betaine, lauramidopropyl betaine). CTAB and SDS are the most frequently used cationic and anionic surfactants, respectively.47
The objective of this study is to evaluate the removal efficiency of metal ions using a low-cost adsorbent i.e., wheat bran and its modified forms. Surface modification of wheat bran was done using cationic and anionic surfactants. Surfactants being amphiphilic structures and owing to their surface properties are expected to enhance the surface area of the used adsorbent. In this work, a comparison will be made between the adsorbent and its modified forms in terms of the percentage extraction of metal ions following batch adsorption studies. Several parameters like the effect of temperature, pH, adsorbate amount, and contact time were considered. From data obtained, isotherms, kinetic models, and thermodynamics were also evaluated.
2. Materials and methods
2.1 Preparation of adsorbents
The wheat (Triticum aestivum) bran is an agricultural waste obtained from flour milling in Abdul Hakim (Village), Punjab, Pakistan). The collected adsorbent was firstly washed with double-distilled water, dried in a calibrated oven at a temperature of 60 °C for 12 h and then cooled in the air-tight desiccator for about 20–30 min. Dried WB was ground to a particle size of 80–100 mesh.62
2.1.1 Preparation of modified wheat bran. The obtained unmodified WB was separately treated with solutions of surfactants (SDS and CTAB) using surfactant concentration 1 mmol L−1. The mixture was stirred at a magnetic plate for 24 h at 30 °C with a ratio of WB (1 g): surfactant solution (50 mL). The sample was filtered after the 24 hours were up and washed three times with double-distilled water to get rid of the unreacted surfactant components. The resulting residue was dried at 60 °C for 12 hours. Glass vials that were airtight used to keep the dry powder. The produced adsorbent materials' functional groups were examined using an FTIR Spectrophotometer (Bruker-Model Alpha).
2.2 Sorption studies
Three adsorbents, wheat bran, CTAB modified wheat bran, and SDS modified wheat bran, were used in batch sorption experiments to assess the percentage removal of chromium VI in aqueous solution. For the sorption experiments, a dissolution tester (Galvano Scientific, BETA-8L) and magnetic hot plate (Cacao 78-1, China) were employed. A combination of factors, including adsorbate concentration (2.5–12.5 ppm), adsorbent dose (1–6 g), pH effect (2–12), agitation time (15–240 min), and agitation speed (50–300 rpm), were studied in the sorption studies. Each combination was sampled after the predetermined amount of time and filtered using whatmann filter paper. Adsorbent dosage (5 g/100 mL), temperature (30 ± 2 °C), pH (4), contact period (1 h), and speed (150 rpm) were all used in the adsorption isotherm studies, while the concentration of the chromium solution was changed from 2.5–12.5 ppm.
Adsorption isotherm study for the adsorption of chromium(VI) metal ions was done by varying the adsorbate concentration from 2.5 to 12.5 ppm, while other parameters including adsorbent dosage (5 g/100 mL), temperature (30 ± 2 °C), pH (4), contact time (1 h), and speed (150 rpm) were kept constant. Kinetic tests at various time intervals i.e., 15–240 min were conducted keeping all other set of conditions the same. By using a UV-VIS spectrophotometer (Shimadzu, UV1800) set at 350 nm, the residual concentration of chromium(VI) in the samples was determined. The total % elimination of metal ions was determined using eqn (1).
|
 | (1) |
where
C0 (mg L
−1) is the initial chromium(
VI) concentration and
Ct (mg L
−1) is the equilibrium chromium concentration.
3. Results and discussion
3.1. Properties of the adsorbents
The characterization of all adsorbents including WB, SDS-mWB, and CTAB-mWB was done by FTIR. Three different infrared spectra have been obtained that help to identify the attached functional groups. The infrared spectra of WB, SDS-mWB, and CTAB-mWB are shown in Fig. 1(a)–(c) respectively. WB biosorbent showed the number of peaks in the infrared region (i.e., 500 to 4000 cm−1). The peak showed on 1018.29 cm−1 may be due to the presence of the (C–O–C) functional group. The peak at 1247.16 cm−1 represents the –COOH stretching of organic compounds (i.e., carboxylic acid). The strong CH2 asymmetric distortion appears at 1412.40 cm−1 while asymmetric C
C stretching occurs at 1542.58 cm−1. The peaks at 1647.30–1733.50 cm−1 are stretching of C
O stretching. The C–H stretching appears at 2853.38 and 2923.54 cm−1, while the stretching of the O–H group occurs at 3267.13 cm−1 (ref. 63) (see Fig. 1(a)).
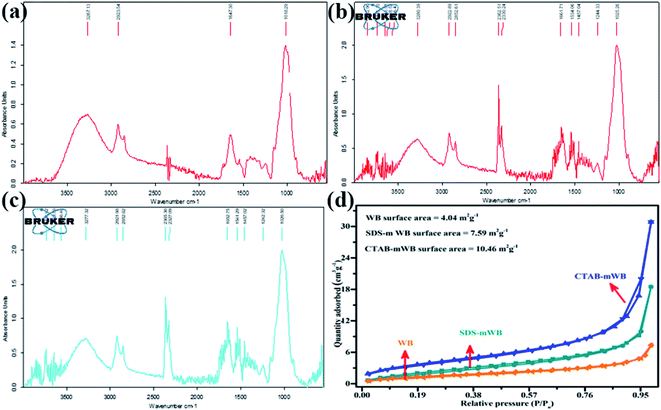 |
| Fig. 1 FTIR spectra for modified and unmodified wheat bran (a) WB, (b) SDS-mWB, (c) CTAB-mWB and (d) the BET surface area isotherms are calculated using the adsorption–desorption of N2 using WB, SDS-mWB and CTAB-mWB. | |
But in the case of SDS-mWB the more intense peak showed at 1025.26 cm−1 which represents the OSO3− symmetric stretching. While the peaks at 1244.33, 1457.04, 1534.06, 1661.71, 2852.61–2922.89 cm−1 showed –COOH (stretching), –CH2 (asymmetric distortion), C
C (stretching), C
O (stretching), and C–H (stretching) respectively.64 The increase in the intensities of peaks is due to high concentration involving functional groups, and the change in wavenumber causes the conversion of the hydrophilic nature of adsorbent to hydrophobic. This is because the water molecules attached to the surface of surfactant are replaced by hydrated cations.44 While the peak of O–H stretching (i.e., at 3280.39 cm−1) for adsorbent SDS-mWB showed the same intensity as that of WB (see Fig. 1(b)). It can be concluded that these sulfate groups (i.e., OSO3−) of SDS-mWB will affect the adsorption process. While the CTAB-mWB showed the more intense but identical peaks as that was obtained for WB (see Fig. 1(c)). Similar to the SDS-mWB, an increase in wavenumber was observed representing its hydrophobic nature.44 The calculated BET surface areas for WB, SDS-mWB and CTAB-mWB were, respectively, 4.04, 7.59 and 10.46 m2 g−1 (Fig. 1(d)). The surface areas of SDS-mWB and CTAB-mWB are higher than that of WB.
3.2. Batch adsorption study
3.2.1. Effect of adsorbate amount. Variable starting chromium levels were used to investigate the influence of adsorbate concentration on the adsorption of chromium(VI) ions from aqueous solution (2.5, 5.0, 7.5, 10.0 and 12.5 ppm). Fig. 2 depicts the influence of adsorbate concentration on chromium removal by WB, SDS-mWB, and CTAB-mWB. The individual results of WB, SDS-mWB and CTAB-mWB showed that the adsorption of chromium ions is continuously going on decreasing with the increase in adsorbate amount, a similar effect was reported by Nameni.65 In case of a lower concentration of adsorbate, more active sites will be available and the possibility of contact of HCrO4− and Cr2O72− ions will be maximum. But on increasing the adsorbate amount the availability of contact surface will be decreased, and there are also the chances of repulsion by already attached metal ions.65,66. On comparing the results of all three samples the order of chromium adsorption is CTAB-mWB > WB > SDS-mWB (76.4% > 52.4% > 23.3%) at initial concentration 2.5 ppm. As we know the CTAB-mWB is cationic therefore it shows a better result as compared to WB and SDS-mWB. And SDS-mWB (anionic-modified adsorbent) showed the adsorption efficiency even less than WB, this may be due to repulsion between already attached anions at contact surface and introducing chromium(VI) metal ions.67
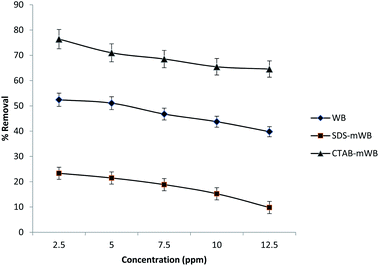 |
| Fig. 2 Effect of adsorbate amount on the sorption of chromium ions (pH = 4; adsorbent dose = 5 g/100 mL; agitation speed = 150 rpm; temp = 30 ± 2 °C; contact time = 1 h). | |
3.2.2. Effect of adsorbent dosage. The impact of adsorbent dosage on the removal of Cr(VI) metal ions from aqueous solution has been determined under ideal conditions with a constant pH of 3 and various adsorbent doses (1.0, 2.0, 3.0, 4.0, 5.0, and 6.0 g/100 mL). The impact of adsorbent dose on the removal of chromium using WB, SDS-mWB, and CTAB-mWB is shown in Fig. 3. Individual WB and CTAB-mWB data revealed that the clearance percent steadily rose as the adsorbent dosage was increased. This is due to the presence of more active sites that increase with the adsorbent dosage and the probability of contact of HCrO4− and Cr2O72−2 ions on adsorbent particles increases consequently the removal efficiency of chromium ions increases.65,68 However, as the adsorbent dosage is increased, the removal effectiveness of SDS-mWB decreases. Because of the negative charges (SO4−) that are present on the surface of the adsorbent, SDS is known to be an anionic surfactant. The concentration of surfactant SDS will be increased with the increase of adsorbent dose, therefore the negative charges on the adsorbent surface will hinder the attachment of HCrO4− andCr2O72− ions thus the adsorption efficiency will be decreased.67 The maximum removal for Cr(VI) on WB, SDS-mWB, and CTAB-mWB adsorbents was found to be 77.6%, 24.5%, and 95.4%, respectively (see Fig. 3). If we compare the results of WB, SDS-mWB, and CTAB-mWB then it can be seen that CTAB-mWB is a highly efficient adsorbent. As we know the CTAB is a cationic surfactant and produces a positive charge on the surface of the adsorbent therefore the tendency of the adsorbent to attract the anions (HCrO4− andCr2O72−2) will be increased.69
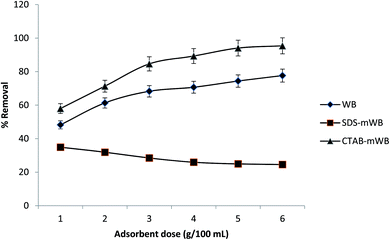 |
| Fig. 3 Effect of adsorbent dose on the sorption of chromium ions (pH = 3; initial Cr conc. = 5 ppm; agitation speed = 150 rpm; temp = 30 ± 2 °C; contact time = 2 h). | |
3.2.3. Effect of agitation time. For the investigation of the contact time effect on the adsorption of Cr(VI), the adsorbent samples (including WB, SDS-mWB, and CTAB-mWB) were taken into the separate flasks with an adsorbent dosage of 5 g in 100 mL of 5 ppm chromium solution at fix pH 3.0, temperature 30 ± 2 °C, and speed 150 rpm. The effect of agitation time was noted at a series of intervals (15, 30, 45, 60, 120, 180, and 240 min) by measuring the absorbance value of filtered samples. The variation in percentage removal of Cr(VI) metal ions with the time of contact has been shown in Fig. 4. All three adsorbents WB, SDS-mWB, and CTAB-mWB showed maximum percentage removal of Cr(VI) at a time of 240 min, which was equal to 79.0%, 27.0%, and 98.7%, respectively. Fig. 4 illustrated that the removal efficiency of chromium for all the samples (WB, SDS-mWB, and CTAB-mWB) initially increased rapidly within 60 min (i.e., 1 h) and after this, no significant changes occur. The equilibrium will be established after 60 min, similar results were reported by Nameni.65
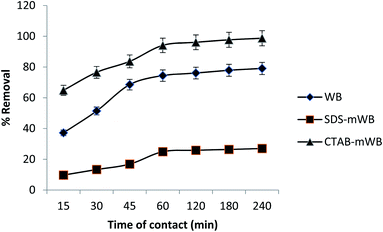 |
| Fig. 4 Effect of contact time on the sorption of chromium ions (pH = 3; adsorbent dose = 5 g/100 mL; initial Cr conc. = 5 ppm; agitation speed = 150 rpm; temp = 30 ± 2 °C). | |
3.2.4. pH effect. The impact of pH on the adsorption process is more critical than the effects of other parameters. For the investigation of the pH effect on the adsorption of Cr(VI), the adsorbent samples (i.e., WB, SDS-mWB, and CTAB-mWB) were taken into the separate flasks with an adsorbent dosage of 5 g in 100 mL of 5 ppm chromium solution at fix conditions temperature 30 ± 2 °C, and speed 150 rpm. By adjusting the pH (2, 4, 6, 8, 10, and 12) and evaluating the absorbance of filtered samples, the impact of pH was investigated. The pH was maintained using a 0.1 M HCl and 0.1 M NaOH solution. The experimental data of this effect is given in Fig. 5 and 6. The obtained results of WB and CTAB-mWB showed that the adsorption efficiency of chromium metal ions is inversely proportional to the pH of chromium solution. At lower pH = 2 both WB and CTAB-mWB showed maximum percentage removal for chromium metal ions equal to 87.6% and 98.8%, respectively. But with the increase in the pH the adsorption efficiency of both (i.e., WB and CTAB-mWB) decreased drastically. The decline in adsorption efficiency may be caused by a weakening of the electrostatic contacts between the oppositely charged adsorbent and adsorbate.70 As the removal of Cr(VI) varies to pH, and at lower pH HCrO4− ion appears as the leading specie over H2CrO4, CrO42− and Cr2O72− ions. At lower pH, the hydroxyl ions (OH−) that are present at the adsorbent surface are easily replaced by the HCrO4− ions (see eqn (3)).65,71
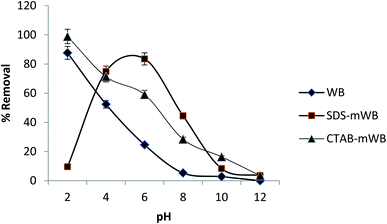 |
| Fig. 5 Effect of pH on the sorption of chromium ions (adsorbent dose = 5 g/100 mL; initial Cr conc. = 5 ppm; agitation speed = 150 rpm; temp = 30 ± 2 °C; contact time = 1 h). | |
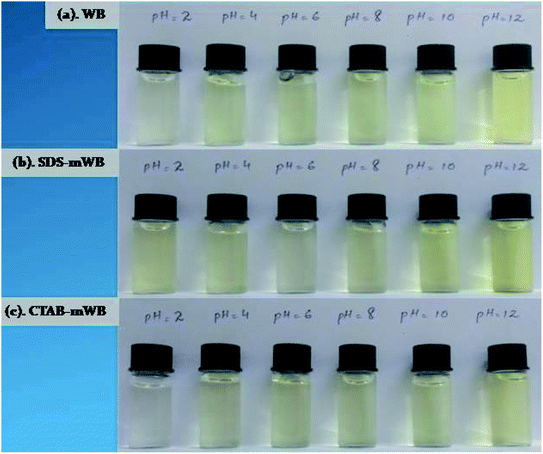 |
| Fig. 6 Effect of pH on adsorbents, (a) WB, (b) SDS-mWB (c) CTAB-mWB. | |
The SDS-mWB showed maximum percentage removal for chromium metal ions (74.87–83.5%) at pH = 4–6. Because at acidic pH the Cr2O72− ions will be converted into the Cr3+ ions (see eqn (4)) which will be strongly attracted toward the anionic surface of SDS-mWB. The adsorption efficiency of SDS-mWB is decreased before pH 4, due to the excess of a proton (H+) as compared to chromium metal ion (Cr3+). At pH 4–6 (less acidic), Cr3+ ions become dominant over protons (H+) and attracted toward the anionic surface of SDS-mWB, therefore maximum removal will occur.72 But at pH greater than the 6 the surface of SDS-mWB becomes more anionic which will strongly hinder the HCrO4−, CrO42− and Cr2O72− ions. Therefore, the SDS-mWB will show minimum results for chromium removal.
|
(Adsorbent)OH + HCrO4− + H+(adsorbent)HCrO4 + H2O
| (2) |
|
Cr2O72− + 6e− + 14H+ ⇔ 2Cr3+ + 7H2O
| (3) |
3.2.5. Effect of agitation speed. To estimate the impact of agitation speed on the extraction of Cr(VI) ions, the adsorbent samples (including WB, SDS-mWB, and CTAB-mWB) were taken into the separate flasks with fix adsorbent dosage of 5 g in 100 mL of 5 ppm chromium solution under constant temperature 30 ± 2 °C and pH 3.0 for the contact time 60 min (i.e. 1 h). The study of the speed effect on adsorption of Cr(VI) was carried out at different speeds (50, 100, 150, 200, 250 and 300 rpm). Fig. 7 shows the gradual increase in adsorption rate with the increase in agitation speed, but optimal results were obtained at 150 rpm. The overall results showed that the adsorbents WB, SDS-mWB, and CTAB-mWB give the maximum adsorption results of 74.5%, 24.9% and 93.9%, respectively. Above 150 rpm, a negligible change in results was observed and the equilibrium was attained. The decrease in thickness of the boundary layer surrounding the adsorbent particles causes the removal percentage to increase with agitation speed.73
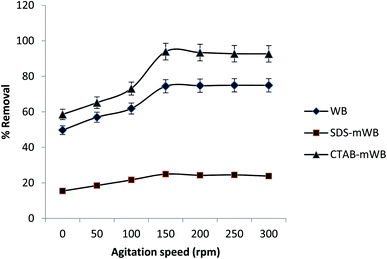 |
| Fig. 7 Effect of agitation speed on the sorption of chromium ions (adsorbent dose = 5 g/100 mL; initial Cr conc. = 5 ppm; pH = 3.0; temp = 30 ± 2 °C; contact time = 1 h). | |
3.2.6. Effect of temperature. The impact of temperature on the adsorption of aqueous Cr(VI) metal ions by adsorbents (i.e., WB, SDS-mWB, and CTAB-mWB) was carried out at varying the temperature from 15 to 50 °C with a constant adsorbent dosage of 5 g in 100 mL of 5 ppm chromium solution, speed 150 rpm, pH 3.0 for contact time 60 min (1 h). The experimental data obtained for all adsorbents show that a significant increase in removal capacities will occur with the increase in the temperature up to 30 °C (see Fig. 8). After 30 °C, there is no significant increase in the percentage removal of all adsorbents was observed and above this temperature, the equilibrium was established. At 50 °C temperature WB, SDS-WB, and CTAB-WB showed maximum percentage removal equal to 66.2%, 21.0%, and 99.4%, respectively. The swelling action within the internal structure of the adsorbent may cause this increase in adsorption with temperature, allowing the big molecules of chromium to penetrate further. Increased temperature may result in more adsorbate–adsorbent interaction when chromium metal ions are removed.74
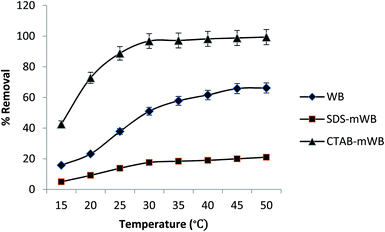 |
| Fig. 8 Effect of temperature on the sorption of chromium ions (adsorbent dose = 5 g/100 mL; initial Cr conc. = 5 ppm; pH = 3.0; agitation speed = 150 rpm; contact time = 1 h). | |
3.3. Isothermal analysis
The isothermal analysis of adsorbents (i.e. WB, SDS-mWB, and CTAB-mWB) was carried out by the estimation of the characterization and removal capacity of adsorbents by taking the help of a series of various isothermal analyses done by researchers.44,65,67 The equilibrium data was applied to both Langmuir (eqn (5)) and Freundlich equation (eqn (6)). The Langmuir model assumes that the adsorption of chromium ions will take place on a uniform monolayer of –OH− ions present on the surface of unmodified wheat bran (WB) and there will be no interaction between adsorbed ions (Fig. 9). Similar behavior was shown in the case of SDS-mWB, but in this case, the monolayer of sulfate group (–SO3−) of anionic surfactant will be responsible for the adsorption of chromium metal ions (Cr(III)) as discussed in the effect of pH on adsorption. The mathematical expression for the Langmuir isotherm is given below;65 |
1/qe = 1/bqmax1/Ce + 1/qmax
| (4) |
where qmax is the maximum quantity of adsorbate that may be absorbed per unit dry weight of sorbent, b is the empirical constant that characterizes the affinity of sorbent to sorbate, adsorbate's equilibrium concentration is known as Ce, and its equilibrium quantity of adsorbed material is known as qe.
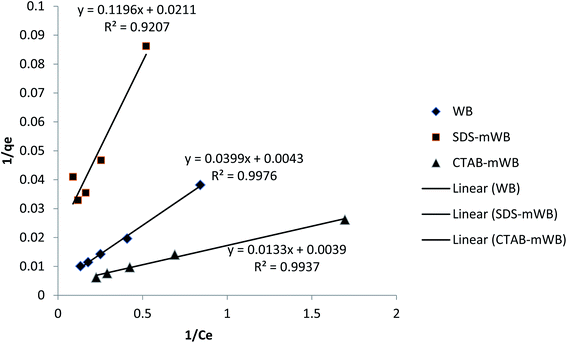 |
| Fig. 9 Test of nature of adsorption by Langmuir adsorption isotherm. | |
The mathematical expression for the Freundlich isotherm is given below.
|
log qe = log Kf + 1/nlog Ce
| (5) |
where
qe is the adsorbed chromium quantity (experimental value),
Ce is residual chromium concentration,
Kf is the Freundlich constant, related to sorption capacity of adsorbent, and 1/
n is the Freundlich isotherm constant related to adsorption intensity. The values of
Kf and
n were obtained by plotting the graph between log
qe versus log
Ce (
Fig. 10).
Kf value was calculated from the intercept, while the value of
n was estimated from the slope.
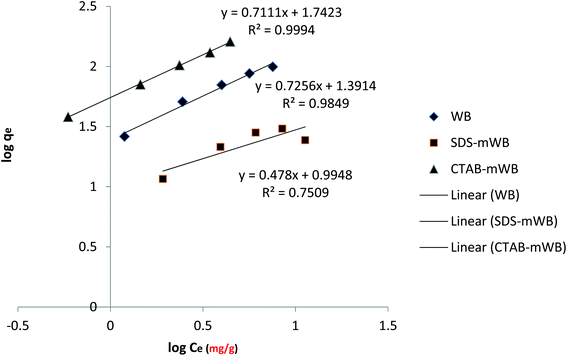 |
| Fig. 10 Test of nature of adsorption by Freundlich adsorption isotherm. | |
The plot of the Freundlich model showed that the cationic modified wheat bran (CTAB-mWB) will follow the Freundlich isotherm with a suitable correlation factor R2 = 0.999 and the value of n is 1.41 (Fig. 10). The Freundlich model assumes that the adsorption energy of chromate ions (HCrO4−) on the CTAB-mWB adsorbent will decrease exponentially with increasing surface saturation and the surface of the adsorbent is heterogeneous.75,76
3.4. Adsorption kinetic
To verify the experimental data and identify the regulated mechanisms of the adsorption process, such as mass transfer and chemical reaction, first- and second-order kinetic models are utilized. All of the adsorbents (including WB, SDS-mWB, and CTAB-mWB) were tested at various intervals (15, 30, 45, 60, 120, 180, and 240 minutes) with the adsorbent dosage of 5 g in 100 mL of 5 ppm chromium solution of pH 3.0, temperature 30 ± 2 °C, and speed 150 rpm held constant. The obtained experimental data is then applied to the pseudo-first equation (Lagergren's equation)65 and second-order equation77 for the study of adsorption kinetic.
Pseudo first order equation (Lagergren's equation)
|
log(qe − qt) = log qe − (k1/2.303)t
| (6) |
Pseudo second order equation
|
t/qt = 1/k2qe2 + t/qe
| (7) |
where
qt denotes metal uptake per unit weight of adsorbent (mg g
−1) at time
t,
qe denotes metal uptake per unit weight of adsorbent (mg g
−1) at equilibrium, and
k1 (min
−1) and
k2 (g mg
−1 min
−1) denote the rate constants of the pseudo-first-order (
Fig. 11) and pseudo-second-order kinetic equations, respectively.
65 These curves' slopes and intercepts were utilised to calculate
k1 and
k2, as well as the equilibrium capacity (
qe). According to the findings, the adsorption process follows a pseudo second-order model. As illustrated in
Fig. 12, the plot of t/
qt vs. 1/
qe produces a straight line.
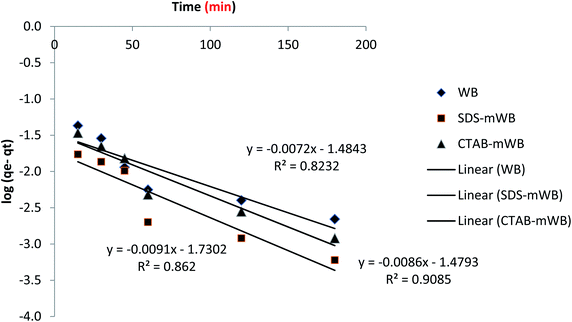 |
| Fig. 11 Graph of pseudo-first order. | |
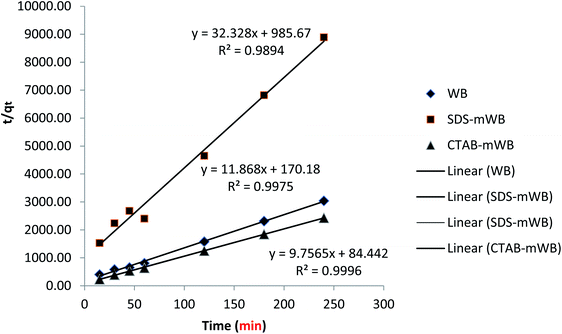 |
| Fig. 12 Graph of pseudo second order. | |
The results of pseudo first and second order kinetics were obtained after the adsorption kinetic of all adsorbents including WB, SDS-mWB and CTAB-mWB. The equilibrium data of WB, SDS-mWB and CTAB-mWB showed that the R2 values of WB, SDS-mWB and CTAB-mWB for first order kinetics and second order kinetic were 0.823, 0.862, 0.908 and 0.997, 0.989, 0.999, respectively. The value of R2 should be closed to 1. The kinetic study showed that all the samples will follow the pseudo second order kinetic as their R2 values are very close to 1.
3.5. Thermodynamic study
Thermodynamic study for the adsorption of aqueous Cr(VI) metal ions on adsorbents (i.e., WB, SDS-mWB, and CTAB-mWB) was conducted by varying the temperature from 15 to 50 °C at constant adsorbent dosage 5 g in 100 mL of 5 ppm chromium solution, speed 150 rpm, pH 3.0 for contact time 60 min (1 h).
The fact that ΔG0 is negative denotes that adsorption is spontaneous.78 The value of the adsorption capacity will be greater at a higher temperature since G0 increases as the temperature rises. The positive value of ΔH0 denotes that adsorption is endothermic, and the positive value of ΔS0 denotes that the adsorbents have a favorable affinity for the chromium solution.65 By comparing the values of ΔS0 for all adsorbents (i.e., WB, SDS-mWB, and CTAB-mWB), it can be concluded that the order of adsorbent–adsorbate affinity is CTAB-mWB > WB > SDS-mWB (345.5 > 78.21 > 14.10) (Fig. 13).
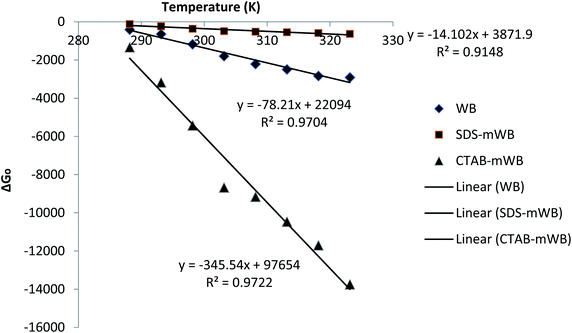 |
| Fig. 13 Thermodynamic study for adsorption of chromium(VI) by adsorbent (adsorbent dose = 5 g/100 mL; initial Cr conc. = 5 ppm; pH = 3.0; agitation speed = 150 rpm; contact time = 1 h). | |
3.6. Adsorption mechanisms
Normally adsorbate–adsorbent interaction is caused by various factors including ionic exchange, electrostatic interaction, and hydrogen bonding. It was observed (Fig. 14) that the unmodified wheat bran (i.e., WB) shows the maximum adsorption capacities at optimum adsorption conditions (i.e. adsorbent dosage 5 g, adsorbate concentration 5 ppm, pH 2, speed 150 rpm, temperature 30 ± 2 °C, contact time 60 min). A homogeneous monolayer of hydroxyl groups is present on the surface of wheat bran. At a more acidic pH (i.e., 2), the –OH− ions will be replaced with HCrO4− (which are more dominant in acidic conditions) by this the chromium adsorption efficiency will be increased. The adsorption of chromium by WB will follow the Langmuir isotherm. The adsorption reaction between adsorbent and adsorbate will be spontaneous and endothermic. The kinetic study showed that the adsorption reaction will be pseudo-second order. The excess number of the proton (H+) will attract the hydroxyl groups of WB and at the same time HCrO4− ions will repel the –OH− by electrostatic forces of attraction and repulsion, respectively (see eqn (3)).
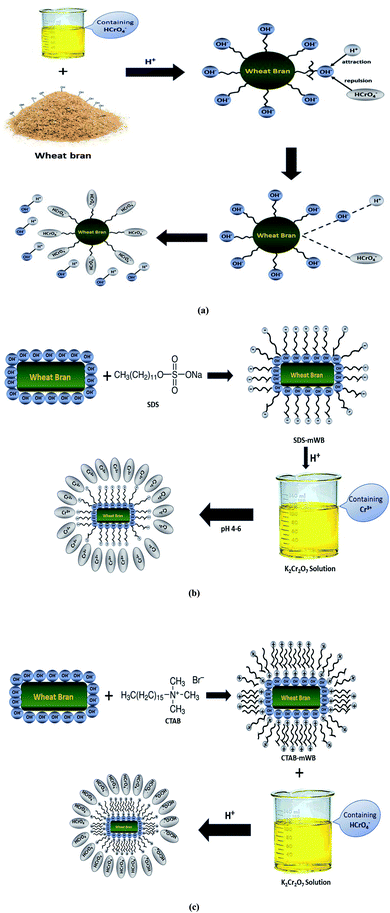 |
| Fig. 14 Adsorption mechanism for the removal of Cr(VI) by adsorbent (a) WB, (b) SDS-mWB and (c) CTAB-mWB. | |
The adsorption study of SDS modified wheat bran SDS-mWB (anionic adsorbent) showed that the maximum removal of chromium will occur at optimum adsorption conditions (i.e., adsorbent dosage 5 g, adsorbate concentration 5 ppm, pH 4–6, speed 150 rpm, temperature 30 ± 2 °C, contact time 60 min). As sulphate groups (–SO4−) of SDS form a homogenous monolayer on WB and follow the Langmuir isotherm model. At suitable acidic pH (i.e., 4–6), the sulphate ions (–SO4−) will attract the Cr+3 ions (which are more dominant at pH 4–6) by strong forces of attraction (see eqn (4)). The kinetic study of SDS-mWB showed that the adsorption reaction will follow the pseudo-second order. In the case of SDS-mWB adsorption reaction between adsorbent and adsorbate will be spontaneous and endothermic.
The adsorption study of CTAB modified wheat bran CTAB-mWB (cationic adsorbent) showed that the maximum removal of chromium will occur at optimum adsorption conditions (i.e., adsorbent dosage 5 g, adsorbate concentration 5 ppm, pH 2, speed 150 rpm, temperature 30 ± 2 °C, contact time 60 min). As trimethylamine groups (–N+(CH3)3) of CTAB from a heterogenous monolayer on WB and follow the Freundlich isotherm model. At a more acidic pH (<3), the trimethylamine ions (–N+(CH3)3) will attract the HCrO4− ions (which are more dominant at pH < 3) by strong forces of attraction (see eqn (3)). The adsorption reaction between adsorbent and adsorbate will be spontaneous and endothermic. The kinetic study of CTAB-mWB showed that the adsorption reaction will follow the pseudo-second order.
4. Conclusion
In a batch system, WB, SDS-mWB, and CTAB-mWB were used to adsorb chromium metal from an aqueous solution. The initial chromium content, pH, agitation time, adsorbent dose, temperature and agitation speed were all varied to better understand the adsorption mechanism. The removal efficiency of WB and CTAB-mWB declined with increasing chromium concentration and increased with increasing adsorbent dosage, according to the results of the experiments. With increasing starting chromium concentration and adsorbent dosage, SDS-mWB removal effectiveness dropped. The optimum pH for chromium removal by WB and CTAB-mWB is 2, while for SDS-mWB the suitable pH is between 4 and 6. Agitation time of 240 min The adsorption value of chromium was also related to the agitation speed and temperature. At agitation speed (i.e., 150 rpm) and temperature (i.e. 25–30 °C), the adsorption results for all adsorbents reach a maximum value and attain equilibrium. The maximum result for chromium uptake was obtained as 87.7%, 83.5% and 98.8% for WB, SDS-mWB, and CTAB-mWB, respectively. The equilibrium data of WB and SDS-mWB was modeled by the Langmuir adsorption isotherm, while the data of CTAB-mWB was fitted well in the Freundlich isotherm model. The kinetic study of WB, SDS-mWB, and CTAB-mWB showed that all of these adsorbents are excellently justified by the pseudo-second-order kinetic model. And the thermodynamic study of all adsorbents confirmed the spontaneous chemical adsorption between adsorbate and adsorbent. It was found that CTAB-mWB can preferably be used for the removal of chromium due to its high affinity with adsorbate molecules and adsorption capacity.
Author contributions
Sadaf Sarfraz: conception, performed metal extraction experiments, visualization of data, writing reviewing, and editing. Safdar Ameer: material synthesis, visualization of data, writing reviewing, and editing. Mohsin Javed: conception, design of study, writing-original draft preparation and critical revision, supervision. Shahid Iqbal: design of study, performed major experimental works, writing-original draft preparation. Samar O. Aljazzar: methodology, reviewed original manuscript, and critical revision. Manzar Zahra: conducted metal extraction experiments, acquisition of data, writing-original draft preparation. Shahid Amin: analysis and/or interpretation of data, performed FTIR analysis. Khizar Hussain Shah: drafting the revised manuscript, performed adsorption analysis and critical revision. Mohammed A. S. Abourehab: conception, design of the study, acquisition of data, interpret the data. Eslam B. Elkaeed: visualization of data, reviewed the original manuscript and critical revision. Nasser S. Awwad: drafting the revised manuscript, acquisition of data, and critical revision. Hala A. Ibrahium: analysis and/or interpretation of data, performed FTIR analysis.
Conflicts of interest
The authors declare no conflict of interest.
Acknowledgements
The authors would like to express their gratitude to King Khalid University, Saudi Arabia, for providing administrative and technical support. This research was funded by Princess Nourah bint Abdulrahman University Researchers Supporting Project number (PNURSP2022R134), Princess Nourah bint Abdulrahman University, Riyadh, Saudi Arabia. “The authors would like to thank the Deanship of Scientific Research at Umm Al-Qura University for supporting this work by Grant Code: (22UQU4290565DSR60). Email: maabourehab@uqu.edu.sa.
References
- L. Rai, S. Singh and S. Pradhan, Biotechnological potential of naturally occurring and laboratory-grown Microcystis in biosorption of Ni 2+ and Cd 2+, Curr. Sci., 1998, 461–464 CAS.
- G. M. Gadd and X. Pan, Biomineralization, bioremediation and biorecovery of toxic metals and radionuclides, Taylor & Francis, 2016 Search PubMed.
- J. Wang and C. Chen, Chitosan-based biosorbents: modification and application for biosorption of heavy metals and radionuclides, Bioresour. Technol., 2014, 160, 129–141 CrossRef CAS PubMed.
- T. Akar and S. Tunali, Biosorption performance of Botrytis cinerea fungal by-products for removal of Cd (II) and Cu (II) ions from aqueous solutions, Miner. Eng., 2005, 18, 1099–1109 CrossRef CAS.
- M. Gavrilescu, Removal of heavy metals from the environment by biosorption, Eng. Life Sci., 2004, 4, 219–232 CrossRef CAS.
- M. Iqbal and R. Edyvean, Biosorption of lead, copper and zinc ions on loofa sponge immobilized biomass of Phanerochaete chrysosporium, Miner. Eng., 2004, 17, 217–223 CrossRef CAS.
- S. Rajendra, Pollution in Abu drainage-a preliminary report, Adv. Plant Sci., 2000, 13, 43–45 Search PubMed.
- C. M. Lee and H. E. Allen, The ecological risk assessment of copper differs from that of hydrophobic organic chemicals, Human and Ecological Risk Assessment, An International Journal, 1998, 4, 605–617 CAS.
- A. A. Meharg, Trace Elements in Soils and Plants, ed. A. Kabata-Pendias, CRC Press/Taylor & Francis Group, Boca Raton, FL, USA, 2010, p. 548, US $159.95. ISBN 9781420093681, Experimental Agriculture, 47 (2011) 739–739 Search PubMed.
- S.-J. Park and W.-Y. Jung, Removal of chromium by activated carbon fibers plated with copper metal, Carbon Lett., 2001, 2, 15–21 Search PubMed.
- C. Raji and T. Anirudha, Chromium (VI) adsorption by sawdust carbon: Kinetics and equilibrium, ( 1997) Search PubMed.
- A. D. Covington, Modern tanning chemistry, Chem. Soc. Rev., 1997, 26, 111–126 RSC.
- J. A. Wilson, The chemistry of leather manufacture, Chemical Catalog Company, Incorporated, 1923 Search PubMed.
- S. R. Tariq, M. H. Shah, N. Shaheen, M. Jaffar and A. Khalique, Statistical source identification of metals in groundwater exposed to industrial contamination, Environ. Monit. Assess., 2008, 138, 159–165 CrossRef CAS PubMed.
- W. Nazif, S. Perveen and S. A. Shah, Evaluation of irrigation water for heavy metals of Akbarpura area, J. Agric. Biol. Sci., 2006, 1, 51–54 Search PubMed.
- R. N. Malik, S. Z. Husain and I. Nazir, Heavy metal contamination and accumulation in soil and wild plant species from industrial area of Islamabad, Pakistan, Pak. J. Bot., 2010, 42, 291–301 CAS.
- S. Muhammad, M. T. Shah and S. Khan, Heavy metal concentrations in soil and wild plants growing around Pb–Zn sulfide terrain in the Kohistan region, northern Pakistan, Microchem. J., 2011, 99, 67–75 CrossRef CAS.
- N. Ahalya, T. Ramachandra and R. Kanamadi, Biosorption of heavy metals, Res. J. Chem. Environ., 2003, 7, 71–79 CAS.
- V. Gupta, Application of low-cost adsorbents for dye removal–a review, J. Environ. Manage., 2009, 90, 2313–2342 CrossRef CAS PubMed.
- T.-Y. Kim, S.-K. Park, S.-Y. Cho, H.-B. Kim, Y. Kang, S.-D. Kim and S.-J. Kim, Adsorption of heavy metals by brewery biomass, Korean J. Chem. Eng., 2005, 22, 91–98 CrossRef CAS.
- S. K. Mehta and J. P. Gaur, Removal of Ni and Cu from single and binary metalsolutions by free and immobilized Chlorella vulgaris, European Journal of Protistology, 2001, 37, 261–271 CrossRef.
- E. Sandau, P. Sandau and O. Pulz, Heavy metal sorption by microalgae, Acta Biotechnol., 1996, 16, 227–235 CrossRef CAS.
- Z. Aksu, F. Gönen and Z. Demircan, Biosorption of chromium (VI) ions by Mowital® B30H resin immobilized activated sludge in a packed bed: comparison with granular activated carbon, Process Biochem., 2002, 38, 175–186 CrossRef CAS.
- M. Alimohamadi, G. Abolhamd and A. Keshtkar, Pb (II) and Cu (II) biosorption on Rhizopus arrhizus modeling mono-and multi-component systems, Miner. Eng., 2005, 18, 1325–1330 CrossRef CAS.
- J. Chiou, K.-S. Wang, C.-H. Chen and Y.-T. Lin, Lightweight aggregate made from sewage sludge and incinerated ash, Waste Management, 2006, 26, 1453–1461 CrossRef PubMed.
- D. Gavrilescu, Energy from biomass in pulp and paper mills, Environ. Eng. Manage. J., 2008, 7, 537–546 CrossRef CAS.
- G. Yan and T. Viraraghavan, Heavy metal removal in a biosorption column by immobilized M. rouxii biomass, Bioresour. Technol., 2001, 78, 243–249 CrossRef CAS PubMed.
- S. Mehta and J. Gaur, Use of algae for removing heavy metal ions from wastewater: progress and prospects, Crit. Rev. Biotechnol., 2005, 25, 113–152 CrossRef CAS PubMed.
- E. Fourest and J.-C. Roux, Heavy metal biosorption by fungal mycelial by-products: mechanisms and influence of pH, Appl. Microbiol. Biotechnol., 1992, 37, 399–403 CrossRef CAS.
- B. Yu, Y. Zhang, A. Shukla, S. S. Shukla and K. L. Dorris, The removal of heavy metals from aqueous solutions by sawdust adsorption—removal of lead and comparison of its adsorption with copper, J. Hazard. Mater., 2001, 84, 83–94 CrossRef CAS PubMed.
- X. Vecino, R. Devesa-Rey, J. Cruz and A. Moldes, Study of the physical properties of calcium alginate hydrogel beads containing vineyard pruning waste for dye removal, Carbohydr. Polym., 2015, 115, 129–138 CrossRef CAS PubMed.
- N. Thombare, U. Jha, S. Mishra and M. Siddiqui, Borax cross-linked guar gum hydrogels as potential adsorbents for water purification, Carbohydr. Polym., 2017, 168, 274–281 CrossRef CAS PubMed.
- S. Ong, C. Lee and Z. Zainal, Removal of basic and reactive dyes using ethylenediamine modified rice hull, Bioresour. Technol., 2007, 98, 2792–2799 CrossRef CAS PubMed.
- Q.-Q. Zhong, Q.-Y. Yue, Q. Li, X. Xu and B.-Y. Gao, Preparation, characterization of modified wheat residue and its utilization for the anionic dye removal, Desalination, 2011, 267, 193–200 CrossRef CAS.
- R. Tang, C. Dai, C. Li, W. Liu, S. Gao and C. Wang, Removal of methylene blue from aqueous solution using agricultural residue walnut shell: equilibrium, kinetic, and thermodynamic studies, J. Chem., 2017, 2017, 8404965 Search PubMed.
- F. Deniz and R. A. Kepekci, Equilibrium and kinetic studies of azo dye molecules biosorption on phycocyanin-extracted residual biomass of microalga Spirulina platensis, Desalin. Water Treat., 2016, 57, 12257–12263 CrossRef CAS.
- S. Chowdhury, S. Chakraborty and P. Das, Adsorption of crystal violet from aqueous solution by citric acid modified rice straw: equilibrium, kinetics, and thermodynamics, Sep. Sci. Technol., 2013, 48, 1339–1348 CrossRef CAS.
- L. Y. Lee, S. Gan, M. S. Y. Tan, S. S. Lim, X. J. Lee and Y. F. Lam, Effective removal of Acid Blue 113 dye using overripe Cucumis sativus peel as an eco-friendly biosorbent from agricultural residue, J. Cleaner Prod., 2016, 113, 194–203 CrossRef CAS.
- R.-k. Xu, S.-c. Xiao, J.-h. Yuan and A.-z. Zhao, Adsorption of methyl violet from aqueous solutions by the biochars derived from crop residues, Bioresour. Technol., 2011, 102, 10293–10298 CrossRef CAS PubMed.
- A. Ahmad, B. Hameed and N. Aziz, Adsorption of direct dye on palm ash: Kinetic and equilibrium modeling, J. Hazard. Mater., 2007, 141, 70–76 CrossRef CAS PubMed.
- T. Robinson, B. Chandran and P. Nigam, Removal of dyes from an artificial textile dye effluent by two agricultural waste residues, corncob and barley husk, Environ. Int., 2002, 28, 29–33 CrossRef CAS PubMed.
- A. Khodabandehloo, A. Rahbar-Kelishami and H. Shayesteh, Methylene blue removal using Salix babylonica (Weeping willow) leaves powder as a low-cost biosorbent in batch mode: kinetic, equilibrium, and thermodynamic studies, J. Mol. Liq., 2017, 244, 540–548 CrossRef CAS.
- M. Jafari, M. R. Rahimi, M. Ghaedi, H. Javadian and A. Asfaram, Fixed-bed column performances of azure-II and auramine-O adsorption by Pinus eldarica
stalks activated carbon and its composite with zno nanoparticles: Optimization by response surface methodology based on central composite design, J. Colloid Interface Sci., 2017, 507, 172–189 CrossRef CAS PubMed.
- P. Zhang, I. Lo, D. O'Connor, S. Pehkonen, H. Cheng and D. Hou, High efficiency removal of methylene blue using SDS surface-modified ZnFe2O4 nanoparticles, J. Colloid Interface Sci., 2017, 508, 39–48 CrossRef CAS PubMed.
- M. S. de Luna, R. Castaldo, R. Altobelli, L. Gioiella, G. Filippone, G. Gentile and V. Ambrogi, Chitosan hydrogels embedding hyper-crosslinked polymer particles as reusable broad-spectrum adsorbents for dye removal, Carbohydr. Polym., 2017, 177, 347–354 CrossRef PubMed.
- G. M. Gadd, Biosorption: critical review of scientific rationale, environmental importance and significance for pollution treatment, J. Chem. Technol. Biotechnol., 2009, 84, 13–28 CrossRef CAS.
- N. Alwadani and P. Fatehi, Synthetic and lignin-based surfactants: Challenges and opportunities, Carbon Resour. Convers., 2018, 1, 126–138 CrossRef CAS.
- M. A. Islam, M. J. Angove and D. W. Morton, Recent innovative research on chromium (VI) adsorption mechanism, Environ. Nanotechnol., Monit. Manage., 2019, 12, 100267 Search PubMed.
- K. G. Bhattacharyya and S. Sen Gupta, Adsorption of chromium (VI) from water by clays, Ind. Eng. Chem. Res., 2006, 45, 7232–7240 CrossRef CAS.
- A. Gładysz-Płaska, M. Majdan, S. Pikus and D. Sternik, Simultaneous adsorption of chromium (VI) and phenol on natural red clay modified by HDTMA, Chem. Eng. J., 2012, 179, 140–150 CrossRef.
- S. Husien, A. Labena, E. El-Belely, H. M. Mahmoud and A. S. Hamouda, Adsorption studies of hexavalent chromium [Cr (VI)] on micro-scale biomass of Sargassum dentifolium, Seaweed, J. Environ. Chem. Eng., 2019, 7, 103444 CrossRef CAS.
- M. Z. Alam and S. Ahmad, Chromium removal through biosorption and bioaccumulation by bacteria from tannery effluents contaminated soil, Clean: Soil, Air, Water, 2011, 39, 226–237 CAS.
- T. Sathvika, A. Soni, K. Sharma, M. Praneeth, M. Mudaliyar, V. Rajesh and N. Rajesh, Potential application of Saccharomyces cerevisiae and Rhizobium immobilized in multi walled carbon nanotubes to adsorb hexavalent chromium, Sci. Rep., 2018, 8, 1–13 CAS.
- B. Ren, Q. Zhang, X. Zhang, L. Zhao and H. Li, Biosorption of Cr (VI) from aqueous solution using dormant spores of Aspergillus niger, RSC Adv., 2018, 8, 38157–38165 RSC.
- H. Arslanoğlu, S. Kaya and F. Tümen, Cr (VI) adsorption on low-cost activated carbon developed from grape marc-vinasse mixture, Part. Sci. Technol., 2020, 38, 768–781 CrossRef.
- M. Shaban, M. R. Abukhadra, M. Rabia, Y. A. Elkader, A. El-Halim and R. Mai, Investigation the adsorption properties of graphene oxide and polyaniline nano/micro structures for efficient removal of toxic Cr (VI) contaminants from aqueous solutions; kinetic and equilibrium studies, Rendiconti Lincei, Scienze Fisiche e Naturali, 2018, 29, 141–154 Search PubMed.
- F. J. Alguacil and F. A. Lopez, Removal of Cr (VI) from waters by multi-walled carbon nanotubes: Optimization and kinetic investigations, Water and Wastewater Treatment, IntechOpen, London, UK, 2019 Search PubMed.
- F. Ostovar, R. Ansari and H. Moafi, Preparation and application of silver oxide/sawdust nanocomposite for Chromium (VI) ion removal from aqueous solutions using column system, Global NEST J., 2017, 19, 412–422 CAS.
- V. Swarnkar, N. Agrawal and R. Tomar, Sorption of chromate by HDTMA-exchanged zeolites, J. Chem. Pharm. Res., 2011, 3, 520–529 CAS.
- V. Swarnkar, N. Agrawal and R. Tomar, Sorption of chromate and arsenate by surfactant modified erionite (E-SMZ), J. Dispersion Sci. Technol., 2012, 33, 919–927 CrossRef CAS.
- C. Rosales-Landeros, C. E. Barrera-Díaz, B. Bilyeu, V. V. Guerrero and F. Ure, A review on Cr (VI) adsorption using inorganic materials, ( 2013) Search PubMed.
- W.-X. Zhang, L. Lai, P. Mei, Y. Li, Y.-H. Li and Y. Liu, Enhanced removal efficiency of acid red 18 from aqueous solution using wheat bran modified by multiple quaternary ammonium salts, Chem. Phys. Lett., 2018, 710, 193–201 CrossRef CAS.
- K. Kaya, E. Pehlivan, C. Schmidt and M. Bahadir, Use of modified wheat bran for the removal of chromium (VI) from aqueous solutions, Food Chem., 2014, 158, 112–117 CrossRef CAS PubMed.
- L. Deng, H. Zeng, Z. Shi, W. Zhang and J. Luo, Sodium dodecyl sulfate intercalated and acrylamide anchored layered double hydroxides: A multifunctional adsorbent for highly efficient removal of Congo red, J. Colloid Interface Sci., 2018, 521, 172–182 CrossRef CAS PubMed.
- M. Nameni, M. A. Moghadam and M. Arami, Adsorption of hexavalent chromium from aqueous solutions by wheat bran, Int. J. Environ. Sci. Technol., 2008, 5, 161–168 CrossRef CAS.
- D. Sidiras, D. Politi, F. Batzias and N. Boukos, Efficient removal of hexavalent chromium from aqueous solutions using autohydrolyzed Scots Pine (Pinus Sylvestris) sawdust as adsorbent, Int. J. Environ. Sci. Technol., 2013, 10, 1337–1348 CrossRef CAS.
- A. A. AbdulRazak and S. Rohani, Sodium dodecyl sulfate-modified Fe2O3/molecular sieves for removal of rhodamine B dyes, Adv. Mater. Sci. Eng., 2018, 2018, 3849867 Search PubMed.
- K. Morshedzadeh, H. Soheilizadeh, S. Zangoie and M. Aliabadi, Removal of chromium from aqueous solutions by lignocellulosic solid wastes, 1st Environment conference, Tehran University, Department of Environment Engineering, 2007 Search PubMed.
- N. Saman, N. A. A. Kamal, J. W. P. Lye and H. Mat, Synthesis and characterization of CTAB-silica nanocapsules and its adsorption behavior towards Pd (II) ions in aqueous solution, Adv. Powder Technol., 2020, 31, 3205–3214 CrossRef CAS.
- S. S. Baral, S. N. Das and P. Rath, Hexavalent chromium removal from aqueous solution by adsorption on treated sawdust, Biochem. Eng. J., 2006, 31, 216–222 CrossRef CAS.
- M. Nadeem, M. Shabbir, M. Abdullah, S. Shah and G. McKay, Sorption of cadmium from aqueous solution by surfactant-modified carbon adsorbents, Chem. Eng. J., 2009, 148, 365–370 CrossRef CAS.
- N. Daneshvar, D. Salari and S. Aber, Chromium adsorption and Cr (VI) reduction to trivalent chromium in aqueous solutions by soya cake, J. Hazard. Mater., 2002, 94, 49–61 CrossRef CAS PubMed.
- E. Demirbaş, M. Kobya, S. Öncel and S. Şencan, Removal of Ni (II) from aqueous solution by adsorption onto hazelnut shell activated carbon: equilibrium studies, Bioresour. Technol., 2002, 84, 291–293 CrossRef.
- G. McKay, Adsorption of dyestuffs from aqueous solutions with activated carbon I: Equilibrium and batch contact-time studies, J. Chem. Technol. Biotechnol., 1982, 32, 759–772 CrossRef CAS.
- E. Bulut, M. Özacar and İ. A. Şengil, Adsorption of malachite green onto bentonite: equilibrium and kinetic studies and process design, Microporous Mesoporous Mater., 2008, 115, 234–246 CrossRef CAS.
- A. Q. Selim, E. A. Mohamed, M. Mobarak, A. M. Zayed, M. K. Seliem and S. Komarneni, Cr (VI) uptake by a composite of processed diatomite with MCM-41: Isotherm, kinetic and thermodynamic studies, Microporous Mesoporous Mater., 2018, 260, 84–92 CrossRef CAS.
- R. Zhang, Y. Zhou, X. Gu and J. Lu, Competitive adsorption of methylene blue and Cu2+ onto citric acid modified pine sawdust, Clean: Soil, Air, Water, 2015, 43, 96–103 CrossRef CAS.
- T. A. Khan, I. Ali, V. V. Singh and S. Sharma, Utilization of fly ash as low-cost adsorbent for the removal of methylene blue, malachite green and rhodamine B dyes from textile wastewater, Journal of environmental protection science, 2009, 3, 11–22 Search PubMed.
|
This journal is © The Royal Society of Chemistry 2022 |