DOI:
10.1039/D2RA02481K
(Paper)
RSC Adv., 2022,
12, 21181-21190
Determination of furfurals in baby food samples after extraction by a novel functionalized magnetic porous carbon†
Received
18th April 2022
, Accepted 18th July 2022
First published on 2nd August 2022
Abstract
Herein, a novel polypyrrole-polyaniline functionalized magnetic porous carbon (MPC@PPy-PANI) composite material was fabricated and utilized for the separation/extraction of furfurals from baby food and dry milk samples. In this way, magnetite@silica nanoparticles were first synthesized, and then a magnetic metal–organic framework (MMIL-101(Fe)) was prepared. After that, the MMIL-101(Fe) was pyrolyzed in a neutral atmosphere to obtain MPC. Ultimately, the MPC was functionalized with a co-polymer of aniline–pyrrole via oxidation polymerization. The synthesis of MPC@PPy-PANI was confirmed with FT-IR spectroscopy, SEM, TEM, VSM, and XRD techniques. Furfural and hydroxymethyl furfural were selected as the model analytes, which were separated/quantified on an HPLC-UV instrument. The LODs, LOQs, and linear dynamic ranges (LDRs) were in the range of 0.3–0.7 μg kg−1, 1.0–2.5 μg kg−1, and 1.0–600 μg kg−1, respectively. Repeatability of the method was studied as an RSD parameter, and was located in the range of 5.5–6.8% (within-day, n = 5) and 8.2–9.4% (between-day, n = 3 days). The applicability of the proposed method was established by analyzing several baby food and dry milk samples. The relative recovery (RR%) and repeatability were located in the range of 86–111% and 3.3–10.1%, respectively, showing excellent accuracy and precision of the method.
1. Introduction
Furfural (F) and hydroxymethyl furfural (HMF) form during the heat treatment of foods. F and HMF are the decomposition products of pentose and hexose, respectively.1,2 The main reactions in the formation of these pollutants are sugar decomposition, caramelization, and the Maillard reaction. Actually, F and HMF are intermediate products of the Millard reaction that can undergo further decarboxylation, oxidation, dehydration, and reduction reactions to produce melanoidins as the final Maillard products.3,4 Furfurals are mutagenic and exhibit DNA strand-breaking activity.5 Although these compounds are found in fresh and untreated foods, their concentration can be increased during heat processing, and they can be utilized as a valuable tool to evaluate the heat damage to foods.6 Baby formulas contain vitamin A, iron, and lactose compounds, which increase the probability of the Maillard reaction occurring in them.7 According to the European Union legislation, the HMF concentration of honey after processing/blending should be <40 mg kg−1. Moreover, the HMF concentration in honey originating from countries/regions with a tropical ambient temperature should be <80 mg kg−1.8 The furfurals are evaluated as nutritional deterioration and heat damage indicators in honey, fruit juices, and milk-based infant formula, which are utilized in preparing of infants and baby food.3
Diverse sample preparation/analytical techniques including spectroscopy,9 solid-phase extraction-gas chromatography/mass spectrometry (SPE-GC/MS),10 SPE-LC-MS,11 and SPE-LC-MS/MS12 were utilized for the furfurals determination in food samples. A sample pretreatment step is essential before the analysis step owing to the matrix effect, and the low concentration of these compounds in foods. Accordingly, developing an easy, quick, precise, and reliable preconcentration/extraction method before the furfurals determination is vital for analytical chemists.
Magnetic-SPE (MSPE), a new form of SPE, which employs magnetic nanoparticles/composites, is extremely utilized in sample pretreatment.13–16 In this regard, nano/microadsorbents with superparamagnetic properties are utilized which can be magnetically separated from the extraction media and so omit the need for the time-consuming filtration/centrifugation step.17,18 A problem in the case of magnetic adsorbents usage is their high tendency for aggregation, which suppresses their performance during the extraction process. Moreover, the bare magnetic nanoparticles (MNPs) have not the suitable moieties. These problems can be solved by employing a spacer, and functionalization.20 In this way, MNPs were coated with various materials, including carbon-based, silica, metal–organic framework (MOFs), etc. A MOF is a class of meso/nanoporous materials with immense framework structures, composed of a metal/or metals as the centers and an organic compound as the linker. MOF-derived magnetic porous carbons (MPCs) are a class of unique materials obtained from magnetic MOFs as precursors.19 The final represents the superparamagnetic features of MNPs along with the unique characteristics of MPCs.20
Herein, a novel PPy-PANI functionalized MPC material was fabricated and utilized for the separation/extraction of furfurals from baby food and dry milk samples. In this way, magnetite@silica NPs were synthesized firstly, and then a magnetic metal–organic framework layer (MMIL-101(Fe)) was prepared. After that, the MMIL-101(Fe) was pyrolyzed in a neutral atmosphere to obtain MPC. Ultimately, the MPC was functionalized with a co-polymer of aniline-pyrrole via the oxidation polymerization. This is the first report on the fabrication and utilization of MPC@PPy-PANI for furfural extraction/determination in baby food and dry milk samples.
2. Experimental
2.1. Materials and solutions
F and HMF (analytical-grade), acetonitrile and methanol (HPLC-grade), ethyl acetate, ethanol (EtOH), isopropanol, acetone, FeCl3·6H2O, FeCl2·6H2O, terephthalic acid (H2BDC), K3[Fe(CN)6], aniline, ammonium persulfate, pyrrole, NaOH, NaCl, (Zn(OAc)2), N,N-dimethylformamide (DMF), acetic acid, and NH4OH solution (25% w/v) were purchased from Merck (Darmstadt, Germany) or Sigma-Aldrich (Germany). A Milli-Q system (Millipore, MA, USA) was employed to prepare deionized water (DI). The real samples (dried milk/baby food) were obtained from the local drug stores (Tehran, Iran) and maintained in a refrigerator (4 °C) before analysis.
Stock solutions of F and HMF (1000 mg L−1) were prepared in HPLC-grade methanol. The mixed working solutions were prepared by dilution of the proper volumes of the stock solution in DI water. To prepare the Carrez solution, 10.6 g K3[Fe(CN)6] was dissolved in 100 mL DI water. Carrez solution II was obtained by mixing 21.9 g Zn(OAc)2 and 3 mL HOAc in a 100 mL volumetric flask and adjusting the volume by DI water.
2.2. Instrumentation
The chromatographic assay was conducted on a Wellchrom Knauer HPLC system (Berlin, Germany). This instrument is composed of a smart line pump1000, a multiple solvent delivery unit, a 6-port/3-channel injection valve (Knauer, Germany), a K-5020 vacuum degasser, and a smart-line 2500 UV-vis detector. Separation of the analytes were carried out using a C18 Knauer HPLC column (Knauer, Germany, 250 × 4.6 mm I.D., 5 μm). The mobile phase was composed of DI water, and acetonitrile (95
:
5 v/v) which was filtered via a Millipore 0.22 μm membrane filter before use. A flow rate of 1.0 mL min−1 was used for the separation. Detection of the target analytes was performed at 285 nm and the injection volume was 100 μL.
XRD characterization was conducted using a Philips-PW 12C diffractometer instrument (Amsterdam, The Netherlands, Cu Kα as the radiation source). FT-IR characterization was performed on a Bruker IFS-66 spectrophotometer (Karlsruhe, Germany). TEM assay was carried out on a Zeiss-EM10C-100 kV instrument (Carl Zeiss, Germany). Magnetic features (at room temperature and 1 Tesla) were studied utilizing a vibrating sample magnetometer (VSM) (Kashan Kavir; Iran). SEM assay was performed on a KYKY-3200 instrument (Beijing, China).
2.3. Synthesis of the functionalized MPC
MNPs@SiO2 were fabricated using the coprecipitation method according to a previous report.21,22 MIL-101(Fe) was synthesized as follows: in brief, 0.5 g MNPs along with 3.4 g FeCl3·6H2O was dissolved in 50 mL DMF via stirring for 45 min (mixture A). After that, H2BDC 1.0 g was added to 50 mL DMF (mixture B). Thereafter, the two mixtures were transferred into a Teflon-line autoclave and heated for 24 h at 110 °C.23 Ultimately, the magnetic composite (MMIL-101(Fe)) was magnetically gathered from the solvent and washed with 20 mL DI water (3 times) and 20 mL EtOH (3 times) to discard the unreacted chemicals and finally dried at room temperature. MIL-101(Fe) was fabricated in the absence of MNPs.
To obtain MPC, the MMIL-101(Fe) was pyrolyzed at 600 °C in a neutral N2 atmosphere for 2 h. Then, a polymer layer of the type polypyrrole-polyaniline was coated on the surface of MPC via oxidative chemical polymerization. In brief, 1.0 g MPC was suspended in 250 mL DI water and then 6.0 g FeCl3·6H2O was added and stirred for 10 min. After that, a mixture of 0.8 mL pyrrole and 0.4 mL aniline was dropwise added to the reaction mixture and stirred for 5 min. The reaction was continued for 6 h at room temperature. Finally, to discontinue the polymerization, 10 mL acetone was added to the mixture. The MPC@PPy-PANI material was magnetically isolated and washed with DI water and acetone several times to discard the unreacted chemicals and then dried at 50 °C for 12 h.24 The structure of the MPC@PPy-PANI was characterized with various methods such as FT-IR, SEM, TEM, VSM, and XRD.
2.4. Real sample preparation
Briefly, 10.0 g of each sample was added to 10 mL DI water in a conical tube and then spiked with the target analytes. After that, the obtained mixture was stirred for 5 min to gain a homogeneous mixture. Thereafter, 4.0 g of the homogeneous sample was transferred into another conical tube and 0.02 mol L−1 NaOH solution (9.0 mL) was added for hydrolyzing the sample. Next, the pH of solution was fixed at 3.0 and a 4 mL mixture of carrez I and II solutions (1
:
1) was added to the sample for the protein precipitation25 and it was shaken for 2 min. Finally, the mixture was centrifuged for 5 min at 4000 rpm, the supernatant was separated and filtered by a Millipore 0.45 μm cellulose acetate membrane filter.26 In another way, the spiked procedure was carried out directly on the sample, followed by a rest period of 1 h to achieve a better interaction of the analytes (standard solution) with the food matrix. The results revealed no significant difference between the two spiking methods, thereby the spiking process was performed in the solution due to its simplicity and time-saving.
2.5. MSPE procedure
At first, 42 mg of MPC@PPy-PANI was dispersed into 47.5 mL 0.1 μg mL−1 analyte solution/real samples containing 15% w/v NaCl. After that, the pH of sample was fixed at 5.3, and the mixture was stirred for 15 min. Afterwards, the MPC@PPy-PANI was magnetically separated from the extraction medium for less than 2 min. Next, about 45 mL of the supernatant solution was discarded and then the remaining solution was transferred into a 6 mL conical vial. The MPC@PPy-PANI material was magnetically separated again and then washed with 130 μL MeOH for desorption of target analytes under a fierce vortex (3.0 min at 3000 rpm). At the end, the MPC@PPy-PANI was gathered magnetically and the collected eluent was subjected to the HPLC system.
3. Results
3.1. Characterization study
The FT-IR spectra of MMIL-101(Fe), and MPC@PPy-PANI material were recorded to characterize the functional groups of these composites (Fig. 1). In the case of MMIL-101(Fe) (Fig. 1a), the absorption peaks of Fe–O (584 cm−1), Si–O–Si (1045 cm−1), C–O–C (1385 and 1621 cm−1), C
O (1716 cm−1), and O–H (3386 cm−1) are observable and affirm the synthesis of magnetic MIL-101(Fe). For MPC@PPy-PANI (Fig. 1b), the bands at 584 cm−1 (Fe–O), 1295–1442 cm−1 (C–N), 1567 cm−1 (C
N, C
C), and 3402 cm−1 (N–H) are related to a composite of MPC, and PPy-PANI.
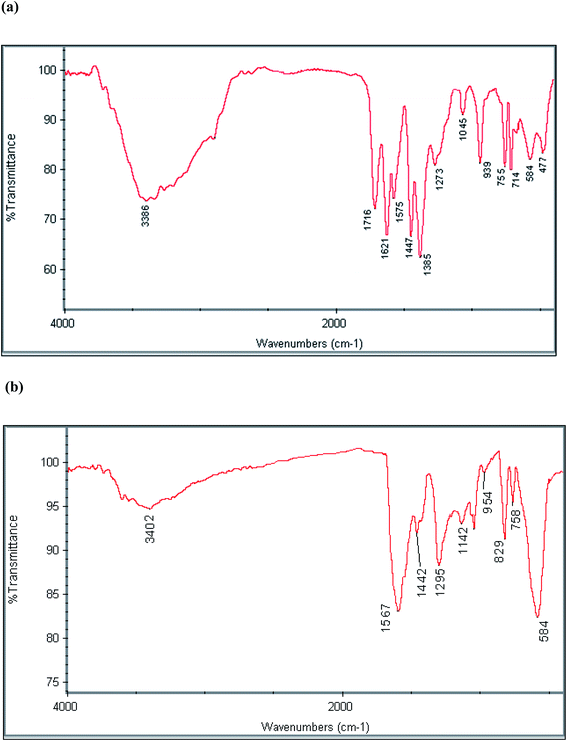 |
| Fig. 1 FT-IR spectra of (a) MMIL-101(Fe) and (b) MPC@PPy-PANI materials. | |
The SEM and TEM methods were utilized to study the morphology/dimension of MPC@PPy-PANI material. SEM micrograph of MPC@PPy-PANI (Fig. 2a) exhibits a 3D porous structure, with a spherical morphology (diameter, ∼45 nm). The TEM micrograph (Fig. 2b) represents that MPC@PPy-PANI with a highly porous structure, and shows no noticeable aggregation with an average particle size of ∼30 nm. The difference between the particle sizes of the two techniques can be attributed to the higher resolution of the TEM method.27
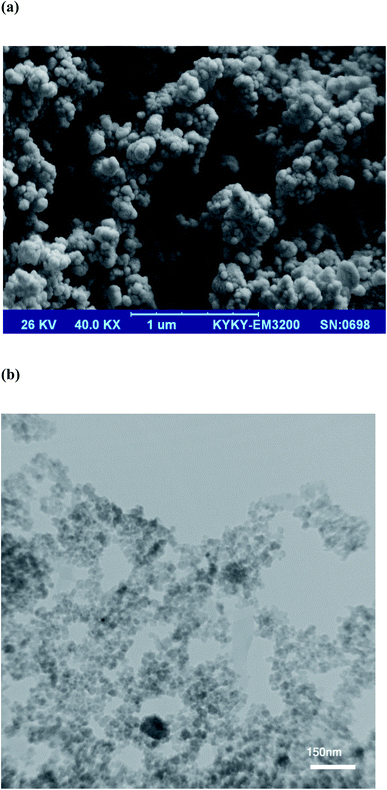 |
| Fig. 2 (a) SEM and (b) TEM micrograph of MPC@PPy-PANI nanocomposite. | |
The structure of MMIL-101 and MPC@PPy-PANI materials was investigated by the XRD technique (Fig. 3). In the case of MMIL-101(Fe), the XRD pattern exhibits diffraction peaks at 2θ = 9, 16.5 and 18.6, which are characteristics of MIL-101(Fe). Moreover, the peaks related to Fe3O4 are observable at 30.2, 35.6, 43.4, 57.2, 63.0, and 74.5. In the case of MPC@PPy-PANI, the presence of diffraction peaks at 30.1, 35.6, 43.3, 57.3, 62.8, and 74.2 is attributed to Fe3O4 crystal and affirms the presence of these particles.
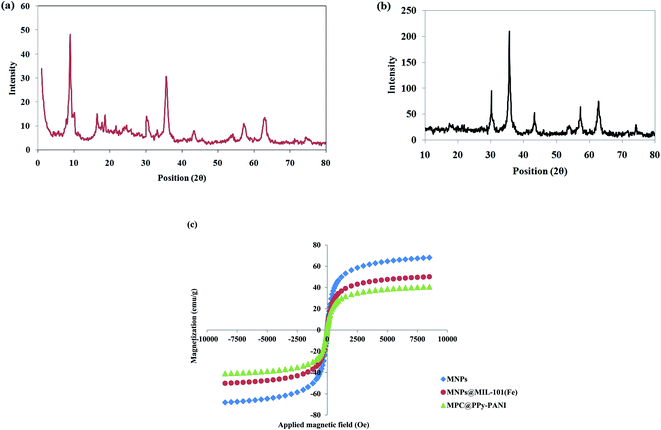 |
| Fig. 3 XRD patterns of (a) MMIL-101(Fe) and (b) MPC@PPy-PANI materials, (c) VSM curves of MNPs, MMIL-101(Fe), and MPC@PPy-PANI. | |
Finally, the magnetic features of magnetite, MMIL-101(Fe), and MPC@PPy-PANI were established using the VSM technique (Fig. 3c). The results reveal no magnetic hysteresis loops in the VSM curves that exhibit superparamagnetic properties of the fabricated materials. The saturation magnetization (SM) of magnetite, MMIL-101(Fe), and MPC@PPy-PANI are 68, 50, and 40 emu g−1, respectively. These SM values are sufficient for the MSPE process. The decrease in magnetization of MMIL-101(Fe), and MPC@PPy-PANI is due to the formation of the carbon skeleton, a polymer layer, and MOF, which acts as a shield.22,26
3.2. Optimization
3.2.1. Adsorbent selection. In this way, the effect of MNPs, MIL-101(Fe), MMIL-101(Fe), MPC, and MPC@PPy-PANI materials on the extraction efficiency of target furfurals were studied (Fig. 4a). The highest extraction efficiency was obtained by employing MPC@PPy-PANI, which can be related to the presence of the co-polymer, and the MPC. The target analytes are high polar compounds (F, log P = 0.41; HMF, log P = −0.39), and so functionalization of MPC with PPy-PANI can enhance their extraction efficiency via π–π stacking, π–cation interaction, and H-bonding formation.26,28–30 Accordingly, MPC@PPy-PANI was selected as the best adsorbent due to its excellent performance and superparamagnetic properties.26
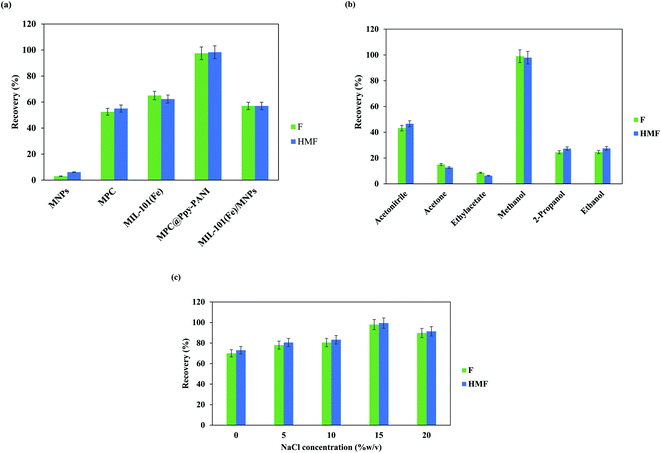 |
| Fig. 4 Effect of (a) nanoadsorbent type, conditions: sample pH, 5.5; nanoadsorbent amount, 40 mg; eluent volume, 150 μL; eluent type, methanol; adsorption time, 20 min; desorption time, 2 min; 10% w/v NaCl. Effects of (b) eluent type, all conditions are similar to (a); except nanoadsorbent type, (c) effect of NaCl concentration on the extraction efficiency, all conditions are similar to (b) except eluent type which is methanol. | |
3.2.2. Effect of eluent and salt addition. Eluent has a very significant role in the MSPE and affects extraction performance. In this regard, a proper eluent should have strong solving power for the target analytes, and also it should be compatible with the detection instrument. In this way, the effects of various eluents such as MeOH, ACN, EtOH, acetone, isopropanol, and ethyl acetate were evaluated. As illustrated in Fig. 4b, MeOH exhibits the highest extraction efficiency and the ability for desorption of the furfurals and so it was selected for the subsequent experiments.The effect of salt addition to the sample solution on the extraction efficiency of the furfurals was explored by varying the NaCl concentration in the domain of 0–20% w/v (Fig. 4c). The salt concentration is a variable that can change the diffusion rate, and solubility of the furfurals in the sample solution. As exhibited in Fig. 4c, the extraction efficiency of the target analytes is improved up to 15% w/v NaCl concentration and then a slight decrease is observable. The enhancement of extraction performance can be attributed to the salting-out effect. The decrease of analytes extractability for NaCl concentration >15% w/v can be corresponded to the solution viscosity increase.30 Moreover, the salt ions occupy the active adsorbent sites and compete with analytes in the adsorption process.30
3.2.3. Design of experiments (DOE). Herein, the effect of various parameters such as the pH of the sample, sorption time, eluent volume, and nanoadsorbent dose on the extraction efficiency was established using a central composite design (CCD) approach. For this purpose, Design-Expert 7.0.0 as a suitable software was employed. In this regard, 30 assays were designed by selecting six replicates in the center point and considering four parameters. The number of the trials is based on the N = 2f + 2f + C0 equation (N is the experiments number; f represents the parameters number; and C0 is the center points number).31–35 Table 1 illustrates the studied parameters and their levels. Accordingly, the geometric mean was used as a suitable response to establish the effect of each parameter and the interactions.
Table 1 The studied parameters and their levels in CCD
|
Level |
Star points (α = 2) |
Lower |
Central |
Upper |
−α |
+α |
A: pH of sample |
2.0 |
3.75 |
5.5 |
1.13 |
6.37 |
B: Sample volume (mL) |
25.0 |
37.5 |
50.0 |
18.8 |
56.2 |
C: Nanosorbent amount (mg) |
25.0 |
37.5 |
50.0 |
18.8 |
56.2 |
D: Adsorption time (min) |
7.5 |
11.25 |
15.0 |
5.6 |
16.8 |
E: Eluent volume (μL) |
100 |
150 |
200 |
75 |
225 |
Based on the analysis of variance (ANOVA) results, the experimental data are in good accordance with the quadratic polynomial model (Table S1, ESI†). Moreover, the ANOVA illustrated that the pH of the sample affects the extraction performance more than other parameters. The sample volume and adsorption time exhibit the second and third significant effects, and nanoadsorbent dose has the least impact on the extraction of target furfurals. The model's p-value was <0.0001 (F-value, 70.23) affirming that the suggested model is significant and implying that there is only a 0.01% chance that it could occur due to noise. Besides, a lack of fit the p-value of 0.7821 (0.05<) implies the absence of pure error.31–35
Finally, the highest extraction performance (desirability value of 1.00) was achieved by employing a pH value of 5.3, a nanoadsorbent dose of 42 mg, an adsorption time of 15 min, and an eluent volume of 130 μL (Fig. 5). The pH of the sample solution affects the predominant species of the analyte as well as the surface charge of the adsorbent. At pH less than 5.3, a repulsive force can occur between the protonated analytes and the positive sites of MPC@PPy-PANI, which reduces the extraction efficiency. Beyond pH 5.3, competition between the analytes species and coexisting ions can decrease the extraction efficiency.30 The MPC@PPy-PANI has a high surface area and short analyte diffusion route due to its nanostructure nature. Hence, the high extraction performance and fast extraction kinetic are expectable compared to the micro-sized adsorbents. Moreover, MeOH volume exhibited a positive effect on the extractability of furfurals up to 130 μL and then a decrease was observed due to the dilution effect.26
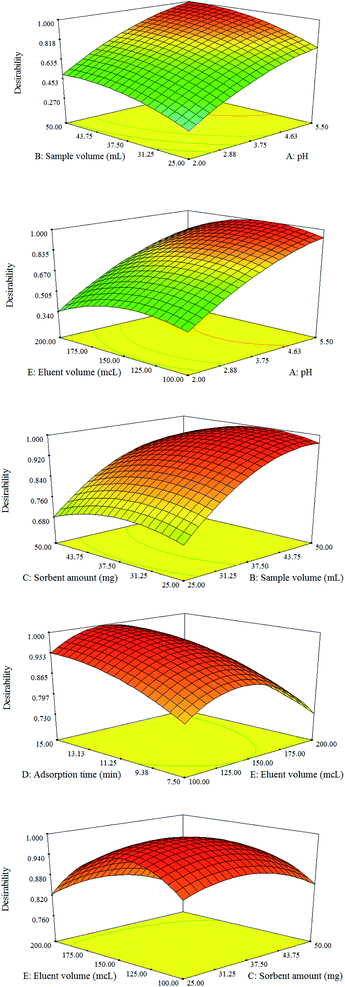 |
| Fig. 5 3D response surface plots of the DOE. | |
3.3. Selectivity assay
Owing to the presence of aromatic rings and NH moieties, the prepared sorbent can be used for extraction of various types of compounds. For example the wide π structure of the polymer can interact with most of organic compounds containing an aromatic structure (benzene rings, heterocyclic aromatic rings and etc.) such as furfurals, PAHs, phthalate esters, organophosphorus, etc. In this regards, the nanosorbent has good tendency toward the furfurals as the model compounds. Under the optimized condition, the fabricated nanosorbent was tested for the extraction of some aromatic compounds such as phenols and aromatic amines but it doesn't show any significant responses.
3.4. Analytical performance
The validation of the new method was performed according to ICH and FDA guidelines. The analytical features of the new method, such as LOD (S/N = 3), LOQ (S/N = 10), enrichment factor (EF), linearity, and repeatability, were established under the optimum conditions (Table 2). The calibration curves were established in a dry milk sample with no contamination as a representative matrix. As it is clear the dry milk sample's matrix is complex so the matrix effect is inevitable, therefore the calibration curve was prepared in the real matrix and the standard solutions for construction of calibration plots were not employed. The results exhibited no matrix effect when the real matrix was utilized. Accordingly, the calibration plots were evaluated in the range of 0.5–600 μg kg−1 (0.5, 1.0, 2.5, 5.0, 10, 25, 50, 100, 200, 300, 400, 500, 600) for furfural, and 1.0–750 μg kg−1 (1.0, 2.5, 5.0, 10, 25, 50, 100, 200, 300, 400, 500, 600, 700) for HMF. Three replicates were performed for each level and all injections were repeated twice. To determine the LODs, the sample was spiked with the concentration levels lower than 1.0 μg Kg−1 for each analyte and then the extraction process was performed. The LODs, LOQs, and linear dynamic ranges (LDRs) were in the range of 0.3–0.7 μg kg−1, 1.0–2.5 μg kg−1, and 1.0–600 μg kg−1, respectively. Repeatability of the method was studied as an RSD parameter, is located in the range of 5.5–6.8% (within-day, n = 5) and 8.2–9.4% (between-day, n = 3 days). The extraction recovery (ER%) and EF were obtained using the following equations:30
where EF is the enrichment factor and Va and Vi are the eluent and sample volume, respectively. EF is computed based on the slope of the calibration plot after extraction (Slope2) to this value before performing the extraction process (Slope1).
Table 2 Analytical characteristics of the proposed method
Analyte |
LOD |
LOQ |
Linear range |
R2 |
EFa |
ERb (%) |
RSD (%)c (within day) |
RSDc (%) (between day) |
1.0 |
40 |
400 |
1.0 |
40 |
400 |
Enhancement factor. Extraction recovery. Relative standard deviations (n = 5 samples for within day and n = 3 days for between day). All concentrations are based on μg kg−1. |
F |
0.3 |
1.0 |
1.0–500 |
0.9982 |
193 |
52.8 |
9.5 |
6.8 |
4.0 |
11.2 |
9.4 |
5.9 |
HMF |
0.7 |
2.5 |
2.5–600 |
0.9964 |
165 |
45.1 |
8.3 |
5.5 |
4.4 |
11.5 |
8.2 |
6.4 |
To explore the applicability of the proposed method, several baby food and dry milk samples were analyzed. The analytes concentration was computed using the calibration plots utilizing the add-found method. The relative recovery (RR%), and repeatability were located in the range of 86–111% and 3.3–10.1%, respectively, showing the good accuracy and precision of the method (Table 3). The obtained values reveal that the proposed method is compatible and applicable in the case of complex-matrix samples.
Table 3 The result of real samples analysisa
Sample |
Analyte |
Real value |
Added |
Found ± SDb |
RRc (%) |
All concentrations are based on μg kg−1. Standard deviation. Relative recovery. |
Dry milk 1 |
F |
8.5 |
10.0 |
18.2 ± 1.0 |
97 |
HMF |
35.4 |
35.0 |
69.1 ± 4.2 |
96 |
Dry milk 2 |
F |
NDb |
10.0 |
9.5 ± 0.7 |
95 |
HMF |
ND |
10.0 |
10.1 ± 0.6 |
101 |
Dry milk 3 |
F |
11.5 |
10.0 |
20.1 ± 2.0 |
86 |
HMF |
178 |
200 |
363 ± 28 |
92 |
Dry milk 4 |
F |
ND |
10.0 |
8.9 ± 0.4 |
89 |
HMF |
18.9 |
20.0 |
36.1 ± 2.8 |
86 |
Baby food 1 |
F |
0.21 |
0.20 |
0.42 ± 0.02 |
105 |
HMF |
1.51 |
1.00 |
2.42 ± 0.08 |
91 |
Baby food 2 |
F |
1.92 |
1.00 |
2.80 ± 0.19 |
88 |
HMF |
1.24 |
1.00 |
2.19 ± 0.10 |
95 |
Baby food 3 |
F |
0.44 |
0.40 |
0.79 ± 0.04 |
87 |
HMF |
1.18 |
1.00 |
2.20 ± 0.15 |
102 |
Baby food 4 |
F |
0.59 |
0.50 |
1.09 ± 0.08 |
100 |
HMF |
7.81 |
1.00 |
8.92 ± 0.64 |
111 |
Baby food 5 |
F |
1.03 |
1.00 |
1.90 ± 0.10 |
87 |
HMF |
2.56 |
2.50 |
5.03 ± 0.44 |
99 |
Baby food 6 |
F |
5.96 |
5.00 |
11.24 ± 0.9 |
106 |
HMF |
0.82 |
1.00 |
1.75 ± 0.09 |
93 |
Baby food 7 |
F |
23.61 |
25.00 |
47.32 ± 3.20 |
95 |
HMF |
2.44 |
2.50 |
5.06 ± 0.51 |
105 |
Baby food 8 |
F |
1.35 |
1.50 |
2.68 ± 0.15 |
89 |
HMF |
3.23 |
3.00 |
6.15 ± 0.45 |
97 |
3.5. Stability and reusability study
The stability of the new nano-material in water and methanol was tested. It was found that the new nanoadsorbent is stable after 48 h remaining in this solvent. The reusability of the MPC@PPy-PANI was explored by conducting repeating the extraction process on 42 mg of this nano-material and measuring the extraction efficiencies of the target furfurals. To activate and prevent the possible carryover, the MPC@PPy-PANI was washed with 4 × 1 mL methanol and then DI water. The results exhibited that the MPC@PPy-PANI can be reused up to 6 cycles without losing the significance extraction performance (90%<).
3.6. Comparison
The analytical features of the proposed method compared to some reported methods are summarized in Table 4. As illustrated in Table 4, the new MSPE-HPLC method exhibits wide LDRs, low LOD and LOQ values, good repeatability, and high EFs compared to the former works. Moreover, our proposed method is easy, quick and consumes a very low organic solvent volume (130 μL).
Table 4 Comparison of the proposed method with the former studies
Analyte |
Method |
DLRa |
R2 |
LODa |
LOQa |
RSD (%) |
Ref. |
All concentrations are based on μg kg−1. Dispersive liquid–liquid microextraction. Mass spectrometry. |
F |
DLLMEb-HPLC-UV |
1.0–200 |
<0.99 |
0.7 |
2.4 |
3.9 |
1 |
HMF |
|
1.8 |
5.9 |
4.9 |
F |
DLLME-HPLC-UV |
0.2–200 |
0.9902 |
1.3 |
4.4 |
4.7 |
3 |
HMF |
0.9915 |
2.1 |
6.7 |
5.1 |
HMF |
SPE-LC-MSc |
50–2000 |
<0.99 |
5.0 |
— |
≥5.1 |
11 |
HMF |
HPLC-UV |
10–200000 |
— |
30.0 |
— |
<2.7 |
36 |
F |
HPLC-UV |
140–3000 |
0.9999 |
3.5 |
11.6 |
— |
37 |
HMF |
80–10000 |
|
8.0 |
27.0 |
|
F |
MSPE-HPLC-UV |
3.0–400 |
0.9971 |
1.0 |
3.0 |
5.2 |
This study |
HMF |
7.0–500 |
0.9945 |
3.0 |
7.0 |
6.4 |
4. Concluding remarks
Herein, a novel PPy-PANI functionalized MPC material was fabricated and utilized for the separation/extraction of furfurals from baby food and dry milk samples. In this way, magnetite@silica NPs were synthesized firstly, and then a magnetic metal–organic framework (MMIL-101(Fe)) was prepared. After that, the MMIL-101(Fe) was pyrolyzed in a neutral atmosphere to obtain MPC. Ultimately, the MPC was functionalized with a co-polymer of aniline-pyrrole via the oxidation polymerization. This is the first report on the fabrication and utilization of MPC@PPy-PANI for furfural extraction/determination in baby food and dry milk samples. The target analytes are high polar compounds (F, log P = 0.41; HMF, log P = −0.39), and so functionalization of MPC with PPy-PANI can enhance their extraction efficiency via π–π stacking, π–cation interaction, and H-bonding formation. Besides, the superparamagnetic properties of the MPC@PPy-PANI lead to a simple and quick method (extraction time <17 min). The relative recovery (RR%), and repeatability for the real samples analysis were located in the range of 86–111% and 3.3–10.1%, respectively showing the good accuracy and precision of the method. The proposed method can potentially be used in the analysis of the real samples with complex a matrix and pre-concentration of other food contaminants such as heavy metals.
Abbreviations
ANOVA | analysis of variance |
CCD | central composite design |
DMF | N,N-dimethylformamide |
EF | enrichment factor |
F | furfural |
HMF | hydroxymethyl furfural |
HPLC-UV | high-performance liquid chromatography-ultraviolet detection |
LOD | limit of detection |
LOQ | limit of quantification |
LDR | linear dynamic range |
MPC | magnetic porous carbon |
MSPE | magnetic solid phase extraction; |
MNPs | magnetite nanoparticles |
MOF | metal–organic framework |
PPy-PANI | polypyrrole-polyaniline |
RR | relative recovery |
RSD | relative standard deviation |
SEM | scanning electron microscopy |
TEM | transmission electron microscopy |
VSM | vibrating sample magnetometry |
XRD | X-ray diffraction |
Ethical statement
This article does not contain any studies with animals performed by any of the authors.
Conflicts of interest
The authors declare that they have no conflict of interest.
Acknowledgements
The authors gratefully acknowledge the Islamic Azad University, Tehran North Branch, for providing facilities.
References
- M. Madani-Tonekaboni, M. Kamankesh and A. Mohammadi, Determination of furfural and hydroxymethyl furfural from baby formula using dispersive liquid–liquid microextraction coupled with high performance liquid chromatography and method optimization by response surface methodology, J. Food Compos. Anal., 2015, 40, 1–7 CrossRef CAS.
- E. Ferrer, A. Alegría, R. Farré, P. Abellán and F. Romero, High-performance liquid chromatographic determination of furfural compounds in infant formulas during full shelf-life, Food Chem., 2005, 89(4), 639–645 CrossRef CAS.
- H. Habibi, A. Mohammadi and M. Kamankesh, Application and optimization of dispersive liquid-liquid microextraction coupled with high-performance liquid chromatography for sensitive determination of furfural and hydroxymethyl furfural in jarred and canned baby-foods, Nutr. Food Sci. Res., 2017, 4(1), 25–32 CrossRef CAS.
- M. J. Antal Jr, W. S. Mok and G. N. Richards, Mechanism of formation of 5-(hydroxymethyl)-2-furaldehyde from D-fructose and sucrose, Carbohydr. Res., 1990, 199(1), 91–109 CrossRef CAS.
- H. Omura, N. Jahan, K. Shinohara, and H. Murakami, in The Maillard Reaction in Foods and Nutrition, Eds., Waller, G. R. and Feather, M. S., ACS Symp. Ser. 215, Am. Chem. Soc., Washington, DC, 1983, p. 537 Search PubMed.
- B. Fallico, E. Arena and M. Zappala, Roasting of hazelnuts. Role of oil in colour development and hydroxymethylfurfural formation, Food Chem., 2003, 81(4), 569–573 CrossRef CAS.
- E. Ferrer, A. Alegrıa, R. Farre, P. Abellan and F. Romero, High-performance liquid chromatographic determination of furfural compounds in infant formulas: Changes during heat treatment and storage, J. Chromatogr. A, 2002, 947(1), 85–95 CrossRef CAS PubMed.
- Directive 2001/110/EC of 20 December 2001, Official Journal of the European Communities, 2001, 47–52.
- R. A. Motiyenko, E. A. Alekseev and S. F. Dyubko, Microwave spectroscopy of furfural in vibrationally excited states, J. Mol. Spectrosc., 2007, 244(1), 9–12 CrossRef CAS.
- E. Teixidó, F. Santos, L. Puignou and M. T. Galceran, Analysis of 5-hydroxymethylfurfural in foods by gas chromatography–mass spectrometry, J. Chromatogr. A, 2006, 1135(1), 85–90 CrossRef PubMed.
- V. Gökmen and H. Z. Şenyuva, Improved method for the determination of hydroxymethylfurfural in baby foods using liquid chromatography− mass spectrometry, J. Agric. Food Chem., 2006, 54(8), 2845–2849 CrossRef PubMed.
- E. Teixido, E. Moyano, F. J. Santos and M. T. Galceran, Liquid chromatography multi-stage mass spectrometry for the analysis of 5-hydroxymethylfurfural in foods, J. Chromatogr. A, 2008, 1185(1), 102–108 CrossRef CAS PubMed.
- S. Rezabeyk and M. Manoochehri, Selective extraction and determination of beryllium in real samples using amino-5, 8-dihydroxy-1, 4-naphthoquinone functionalized magnetic MIL-53 as a novel nanoadsorbent, RSC Adv., 2020, 10(60), 36897–36905 RSC.
- A. A. Asgharinezhad, N. Jalilian, H. Ebrahimzadeh and Z. Panjali, A simple and fast method based on new magnetic ion imprinted polymer nanoparticles for the selective extraction of Ni (II) ions in different food samples, RSC Adv., 2015, 5(56), 45510–45519 RSC.
- M. Landarani, A. A. Asgharinezhad and H. Ebrahimzadeh, A magnetic ion-imprinted polymer composed of silica-coated magnetic nanoparticles and polymerized 4-vinyl pyridine and 2, 6-diaminopyridine for selective extraction and determination of lead ions, New J. Chem., 2020, 44(18), 7561–7568 RSC.
- M. Mehraban, M. Manoochehri and F. A. Taromi, Trace amount determination of Cd (II), Pb (II) and Ni (II) ions in agricultural and seafood samples after magnetic solid phase extraction by MIL-101 (Cr)/phenylthiosemicarbazide-functionalized magnetite nanoparticle composite, New J. Chem., 2018, 42(21), 17636–17643 RSC.
- A. Afkhami, S. Aghajani, T. Madrakian and H. Bagheri, Simultaneous preconcentration and determination of trace quantities of inorganic arsenic species in water using Ni0.5Zn0.5Fe2O4 magnetic nanoparticles, Chem. Pap., 2020, 17, 1–7 Search PubMed.
- M. Zeeb and H. Farahani, Graphene oxide/Fe3O4@polythionine nanocomposite as an efficient sorbent for magnetic solid-phase extraction followed by high-performance liquid chromatography for the determination of duloxetine in human plasma, Chem. Pap., 2018, 72, 15–27 CrossRef CAS.
- W. Xia, J. Zhu, W. Guo, L. An, D. Xia and R. Zou, Well-defined carbon polyhedrons prepared from nano metal–organic frameworks for oxygen reduction, J. Mater. Chem. A, 2014, 2(30), 11606–11613 RSC.
- F. Maya, C. P. Cabello, R. M. Frizzarin, J. M. Estela, G. T. Palomino and V. Cerdà, Magnetic solid-phase extraction using metal-organic frameworks (MOFs) and their derived carbons, TrAC, Trends Anal. Chem., 2017, 90, 142–152 CrossRef CAS.
- A. A. Asgharinezhad, H. Ebrahimzadeh, M. Rezvani, N. Shekari and M. Loni, A novel 4-(2-pyridylazo) resorcinol functionalised magnetic nanosorbent for selective extraction of Cu (II) and Pb (II) ions from food and water samples, Food Addit. Contam., Part A, 2014, 31, 1196–1204 CAS.
- M. B. Sabeghi, H. R. Ghasempour, M. K. Koohi and N. Karimi, Synthesis and application of a novel functionalized magnetic MIL-101 (Cr) nanocomposite for determination of aflatoxins in pistachio samples, Res. Chem. Intermed., 2020, 46(9), 4099–4111 CrossRef CAS.
- M. Babazadeh, R. Hosseinzadeh-Khanmiri, J. Abolhasani, E. Ghorbani-Kalhor and A. Hassanpour, Solid phase extraction of heavy metal ions from agricultural samples with the aid of a novel functionalized magnetic metal–organic framework, RSC Adv., 2015, 5(26), 19884–19892 RSC.
- S. Yang, D. Liu, F. Liao, T. Guo, Z. Wu and T. Zhang, Synthesis, characterization, morphology control of poly (p-phenylenediamine)-Fe3O4 magnetic micro-composite and their application for the removal of Cr2O72- from water, Synth. Met., 2012, 162(24), 2329–2336 CrossRef CAS.
- V. Ghasemzadeh-Mohammadi, A. Mohammadi, M. Hashemi, R. Khaksar and P. Haratian, Microwave-assisted extraction and dispersive liquid–liquid microextraction followed by gas chromatography–mass spectrometry for isolation and determination of polycyclic aromatic hydrocarbons in smoked fish, J. Chromatogr. A, 2012, 1237, 30–36 CrossRef CAS PubMed.
- S. D. Davari, M. Rabbani, A. A. Basti and M. K. Koohi, Synthesis and characterization of a novel magnetic porous carbon coated with poly (p-phenylenediamine) and its application for furfural preconcentration and determination in baby food and dry milk powder samples, RSC Adv., 2021, 11(37), 22983–22992 RSC.
- A. A. Asgharinezhad, N. Mollazadeh, H. Ebrahimzadeh, F. Mirbabaei and N. Shekari, Magnetic nanoparticles based dispersive micro-solid-phase extraction as a novel technique for coextraction of acidic and basic drugs from biological fluids and waste water, J. Chromatogr. A, 2014, 1338, 1–8 CrossRef CAS PubMed.
- A. A. Asgharinezhad, S. Karami, H. Ebrahimzadeh, N. Shekari and N. Jalilian, Polypyrrole/magnetic nanoparticles composite as an efficient sorbent for dispersive micro-solid-phase extraction of antidepressant drugs from biological fluids, Int. J. Pharm., 2015, 494(1), 102–112 CrossRef CAS PubMed.
- H. Bagheri, R. Daliri and A. Roostaie, A novel magnetic poly(aniline-naphthyl-amine)-based nanocomposite for micro solid phase extraction of rhodamine B, Anal. Chim. Acta, 2013, 794, 38–46 CrossRef CAS PubMed.
- N. Jalilian, H. Ebrahimzadeh and A. A. Asgharinezhad, Dispersive micro-solid phase extraction of aromatic amines based on an efficient sorbent made from poly(1,8-diaminonaphtalen) and magnetic multiwalled carbon nanotubes composite, J. Chromatogr. A, 2017, 1499, 38–47 CrossRef CAS PubMed.
- H. Ebrahimzadeh, A. A. Asgharinezhad, L. Adlnasab and N. Shekari, Optimization of ion-pair based hollow fiber liquid phase microextraction combined with HPLC-UV for the determination of methimazole in biological samples and animal feed, J. Sep. Sci., 2012, 35, 2040–2047 CrossRef CAS PubMed.
- A. Ostovan, M. Ghaedi, M. Arabi, Q. Yang, J. Li and L. Chen, Hydrophilic multitemplate molecularly imprinted biopolymers based on a green synthesis strategy for determination of B-family vitamins, ACS Appl. Mater. Interfaces, 2018, 10, 4140–4150 CrossRef CAS PubMed.
- A. R. Bagheri, M. Arabi, M. Ghaedi, A. Ostovan, X. Wang, J. Li and L. Chen, Dummy molecularly imprinted polymers based on a green synthesis strategy for magnetic solid-phase
extraction of acrylamide in food samples, Talanta, 2019, 195, 390–400 CrossRef CAS PubMed.
- A. A. Asgharinezhad and H. Ebrahimzadeh, Magnetic porous carbon nanocomposite derived from cobalt based-metal-organic framework for extraction and determination of homo and hetero-polycyclic aromatic hydrocarbons, Talanta, 2021, 233, 122526 CrossRef CAS PubMed.
- A. A. Asgharinezhad and H. Ebrahimzadeh, A novel polymer coated magnetic porous carbon nanocomposite derived from a metal-organic framework for multi-target environmental pollutants preconcentration, J. Chromatogr. A, 2020, 1634, 461664 CrossRef CAS PubMed.
- L. V. I. B. K. Kalábová and V. Večerek, Hydroxymethylfurfural contents in foodstuffs determined by HPLC method, J. Food Nutr. Res., 2006, 45(1), 34–38 Search PubMed.
- M. Mesias-Garcia, E. Guerra-Hernandez and B. Garcia-Villanova, Determination of furan precursors and some thermal damage markers in baby foods: ascorbic acid, dehydroascorbic acid, hydroxymethylfurfural and furfural, J. Agric. Food Chem., 2010, 58(10), 6027–6032 CrossRef CAS PubMed.
|
This journal is © The Royal Society of Chemistry 2022 |