DOI:
10.1039/D2RA02216H
(Paper)
RSC Adv., 2022,
12, 16589-16598
I2/TBHP mediated domino synthesis of 2-(2,4-dioxo-1,4-dihydroquinazolin-3(2H)-yl)-N-aryl/alkyl benzamides and evaluation of their anticancer and docking studies†
Received
5th April 2022
, Accepted 24th May 2022
First published on 6th June 2022
Abstract
A novel I2/TBHP mediated domino synthesis of 2-(2,4-dioxo-1,4-dihydroquinazolin-3(2H)-yl)-N-phenyl benzamides by reaction of isatins with o-amino N-aryl/alkyl benzamides was described. This was the first application of o-amino N-aryl/alkyl benzamides participating in oxidative rearrangement with isatins for synthesis of desired products. The synthesized compounds contained amide and quinazoline units and their combination resulted in molecular hybridization of two important pharmacophores. In this study, the synthesized compounds 3a–r were screened for cytotoxicity against four cancer cell lines A549, DU145, B16-F10, and HepG2 and also non-cancerous cell line CHO-K1. The compounds 3c, 3l and 3o gave promising results. The in silico molecular docking studies (PDB ID 1N37) also validated the anticancer activity of these compounds showing good binding affinity with target DNA and by acting as DNA intercalators.
Introduction
Molecular hybridization has been a strategy in drug discovery research in recent years.1 It involves blending or linking of two or more pharmacophore moieties to generate a single molecular entity which could exhibit better selectivity, and improved affinity and activity, and overcome the drug resistance due to a mixed mechanism of action. Cancer, a debilitating disease, is a worldwide major human health problem. Though many anticancer agents are available, still there is a call to identify effective and safe drugs for treatment of cancer. Numerous heterocyclic compounds have been researched to discover new anticancer agents.2
Quinazoline pharmacophore is found in various pharmaceuticals including antimicrobials and cytotoxic agents.3–5 Quinazolines exhibit a wide range of biological properties such as anti-hypertensive,6 anti-HIV-1,7 anti-cancer,8 antitumor,9 anti-inflammatory,10 progesterone receptor antagonists11 and antibacterial activity.12 Some small molecules containing quinazolines motif are also known to exhibit potent and specific puromycin-sensitive amino peptidase (PSA) inhibitory activity13 and reversible inhibition of Bruton's tyrosine kinase (BTK).14 Quinazoline scaffold is found also in several natural products.15
Carboxamide scaffolds are of immense interest in medicinal chemistry.16,17 The pharmaceutically important ergot alkaloids such as dehydro ergotamine and ergotamine contain amide linkage. There are many drugs like atorvastatin, valsartan, paracetamol and penicillin available in the market, which contains amide linkage in the nucleus and possesses varied therapeutic activities like antimycobaterial, antimicrobial, antifungal, cytotoxic, antiepileptic and analgesic properties.2,18 The amide bond is planar, resonating and stable with large dipole moment, resembles the protein's peptide linkage.19 Use of peptide-based therapeutics is steadily increasing, for example, cyclosporine A is a peptide which is a widely used powerful immunosuppressive agent.20 Anticancer agents with amide pharmacophore21 and quinazoline pharmacophore are shown in Fig. 1.
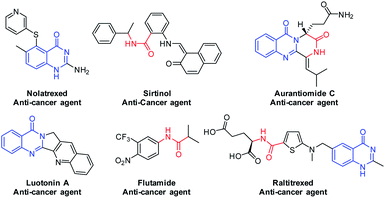 |
| Fig. 1 Cancer drugs with quinazoline, amide and hybrid pharmacophores. | |
Hybrid drugs with multiple mechanism of action are of great interest for cancer treatment, for example, quinazoline-amide hybrid drug raltitrexed1c known for potent anticancer activity.22 In the present work, we aimed at synthesis of 2-(2,4-dioxo-1,4-dihydroquinazolin-3(2H)-yl)-N-aryl/alkyl benzamides, which were new quinazoline-amide hybrid molecules. No literature is available for synthesis of these quinazoline-amide hybrid molecules. Some existing literature was available for synthesis of simple 2,4-quninozolinediones23 but these methods had one or more disadvantages such as metal catalysts, harsh reaction conditions and limited substrate scope.
Under existing literature conditions were not promoted the synthesis of desired molecular hybrids 2-(2,4-dioxo-1,4-dihydroquinazolin-3(2H)-yl)-N-aryl/alkyl benzamides by reaction of isatins with 2-amino-(N-alkly/aryl) benzamides. Therefore, we were much interest to synthesize these quinazoline-amide hybrid molecules, because of their important applications in organic synthesis and medicinal chemistry. Moreover, I2/TBHP mediated oxidative coupling reactions are very important than those transition-metal-mediated oxidative reactions for they are greener and less expensive. It has been widely studied and applied for a variety of oxidation reactions.24
Domino reactions have been one of the mainly constructive and powerful synthetic tools in organic synthesis. The advantage of these domino reactions as a methodology involve two or more bond constructions that takes place under the same reaction conditions in one-pot. In the past several years, authors have developed a variety of multicomponent domino reactions that can afford easy access to functionalized various heterocycles.25
Here, we observed for the first time a new domino reaction of isatins with 2-amino N-aryl/alkyl benzamides in the presence of I2/TBHP under reflux in methanol to give 2-(2,4-dioxo-1,4-dihydroquinazolin-3(2H)-yl)-N-aryl/alkyl benzamides in high yields with broad functional group tolerance and short reaction time as shown in Scheme 1.
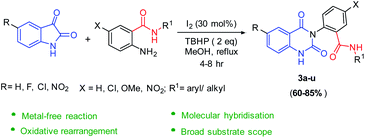 |
| Scheme 1 Synthesis of 2-(2,4-dioxo-1,4-dihydroquinazolin-3(2H)-yl)-N-aryl/alkyl benzamides. | |
To the best of our knowledge, this I2/TBHP reagent system has not been used for preparation of 2-(2,4-dioxo-1,4-dihydroquinazolin-3(2H)-yl)-N-aryl/alkyl benzamides through oxidative rearrangement of isatins and 2-amino-(N-alkly/aryl) benzamides.
Furthermore, all the synthesized compounds were well characterized and assessed for their cytotoxicity by MTT assay against four cancer cell lines such as A549, DU145, B16-F10, HepG2 and non-cancerous cell line CHO-K1. In addition, to understand the structure–activity relationships, evaluate the possible intercalating potency of the synthesized compounds with DNA and validate the biological studies, molecular docking studies were conducted.
Results and discussion
Chemistry
In our preliminary experiments, reaction of isatin 1a with 2-amino-N-phenylbenzamide 2a in presence of t-butyl hydroperoxide (TBHP, 2 equivalents) as oxidant and iodine as a catalyst (10 mol%) under reflux in methanol gave 2-(2,4-dioxo-1,2-dihydroquinazolin-3(4H)-yl)-N-phenylbenzamide 3a in 70% yield (entry 1, Table 1). In this reaction, yield of 3a was found to increase with increasing amount of catalyst, for example, 20% and 30 mol% of iodine gave 3a in 80% and 85% yields respectively (entries 2 and 3, Table 1). In the absence of I2/TBHP, no reaction was observed (entry 4, Table 1). These observations showed that catalyst played important role in the formation of product 3a. We also studied the reaction using 30 mol% of iodine and H2O2 as oxidant to obtain 3a in 65% yield (entry 5, Table 1). We also studied the action using 30 mol% of iodine and TBHP in various solvents such as acetonitrile, ethanol, 2-propanol, 1,2-dichloroethane (DCE), dichloromethane, toluene, 1,4-dioxane and chloroform to obtain 3a in 80%, 78%, 76%, 73%, 72%, 70%, 60% and 50% yields respectively (entries 6–13, Table 1). In the absence of solvent, no reaction was observed (entry 14, Table 1).
Table 1 Optimization of reaction conditions
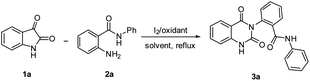
|
Entry |
I2 (mol%) |
Oxidant (2 eq.) |
Solvent |
Time (h) |
% Yielda (3a) |
Isolated yields. NR = no reaction. |
1 |
I2 (10) |
TBHP |
MeOH |
15 |
70 |
2 |
I2 (20) |
TBHP |
MeOH |
15 |
80 |
3 |
I2 (30) |
TBHP |
MeOH |
4 |
85 |
4 |
— |
— |
MeOH |
15 |
NR |
5 |
I2 (30) |
H2O2 |
MeOH |
15 |
65 |
6 |
I2 (30) |
TBHP |
CH3CN |
15 |
80 |
7 |
I2 (30) |
TBHP |
EtOH |
15 |
78 |
8 |
I2 (30) |
TBHP |
2-Propanal |
15 |
76 |
9 |
I2 (30) |
TBHP |
DCE |
15 |
73 |
10 |
I2 (30) |
TBHP |
DCM |
15 |
72 |
11 |
I2 (30) |
TBHP |
Toulene |
15 |
70 |
12 |
I2 (30) |
TBHP |
1,4-Dioxane |
15 |
60 |
13 |
I2 (30) |
TBHP |
CH3Cl |
15 |
50 |
14 |
I2 (30) |
TBHP |
— |
15 |
NR |
After the condition was optimal (entry 3, Table 1), we next turned our interest towards evaluating the generality of this transformation with different 2-amino-(N-alkyl/aryl)benzamides (2a–h), which contain phenyl, methoxy, methyl, fluoro, bromo, phenethyl, n-butyl or ethyl group on the aryl ring and N-alkyl or N-aryl functionality on the amide group. These compounds afforded 2-(2,4-dioxo-1,4-dihydroquinazolin-3(2H)-yl)-N-aryl/alkyl benzamides 3a–h (Table 2) in good to excellent yields (65–85%). Interestingly, having free amide functionality the corresponding quinazolinedione product could undergo further cyclization to obtain 5H-quinazolino [3,2-a]quinazoline-5,12(7H)-dione 6a in 82% yield as shown in Table 2. In this study, substituted 2-amino-(N-alkyl/aryl)benzamides with both electron donating and electron-withdrawing groups on amide group operate well in this transformation to produce the corresponding 2-(2,4-dioxo-1,4-dihydroquinazolin-3(2H)-yl)-N-aryl/alkyl benzamides in good to excellent yields.
Table 2 Scope of 2-aminobenzamides
Isolated yields. All products gave satisfactory 1H NMR, 13C NMR, IR and mass spectral data. |
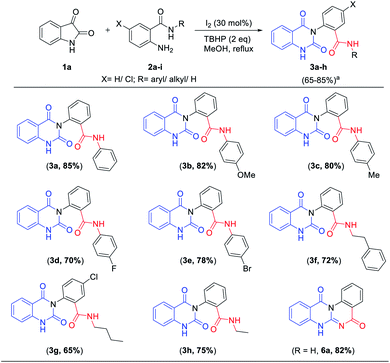 |
To further expand the substrate scope, the reactivity of diversely substituted isatins (1b–d) and 2-amino-(N-alkyl/aryl) benzamides (2a–i) were examined under optimized conditions (Table 3). Reactions of substituted isatins such as 5-fluoro-, 5-chloro-, 5-nitro isatins (2b–d) smoothly reacted with 2-amino (N-aryl/alkyl) benzamides containing 4-methoxy, 4-fluoro, 4-bromo, n-butyl and ethyl gave good to excellent yields (68–78%) and 2-amino N-phenylbenzamide 2i react with 5-chloro isatin 1c to produce the corresponding product 6b in good yield of 79%.
Table 3 Scope of 2-amino (N-aryl/alkyl)benzamides and isatins
Isolated yields. All products gave satisfactory 1H NMR, 13C NMR, IR and mass spectral data. |
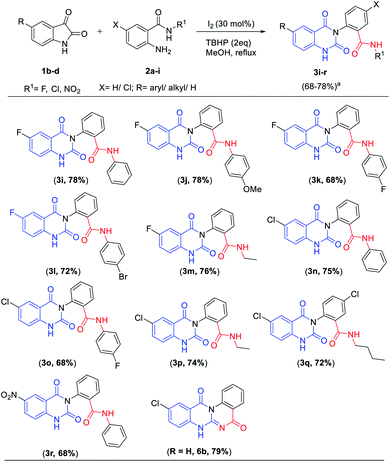 |
To further expand the substrate scope, the reactivity of diversely substituted isatin (1a) and 2-amino-N-phenyl benzamides (2j–l) were examined under optimized conditions. Reactions of 5-OMe/5-chloro/5-nitro substituted 2-amino N-phenyl benzamides smoothly reacted with isatin gave quinazolinediones 3s–u (Table 4) as good to excellent yields (60–81%). In this transformation isatin react with electron withdrawing group (–NO2) contained 2-amino N-phenyl benzamide gave moderate yield (3u, 60%) when compared to electron donating group (–OMe) contained 2-amino N-phenyl benzamides (3s, 81%).
Table 4 Scope of 2-amino N-phenyl benzamides
Isolated yields. |
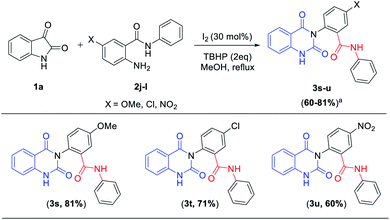 |
The results observed in the control experiments are shown in Scheme 2. In this study, formation of 3a was observed only when reaction was conducted using I2/TBHP under reflux in methanol. This reaction did not proceed when TBHP used alone, and also no reaction was observed when conduct at cooling and ambient temperatures (Scheme 2, eqn (1) and (2)). However, when the reaction was carried out in presence of iodine without oxidant, it gave the intermediate (Z)-2-((2-oxoindolin-3-ylidene)amino)-N-phenyl benzamide 4a (Scheme 2, eqn (3)). In our study, the intermediate 4a was found to react with TBHP and converted into intermediate (Z)-2-((2-oxo-1,2-dihydro-4H-benzo[d][1,3]oxazin-4-ylidene)amino)-N-phenylbenzamide 5a (Scheme 2, eqn (4)). The important observation noted in this study is, the intermediate 5a was converted into product 3a when treated with iodine under reflux in methanol (Scheme 2, eqn (5)). The intermediate 4a also directly converted into 3a when treated with I2/TBHP or I2/H2O2 under reflux in methanol (Scheme 2, eqn (6) and (7)).
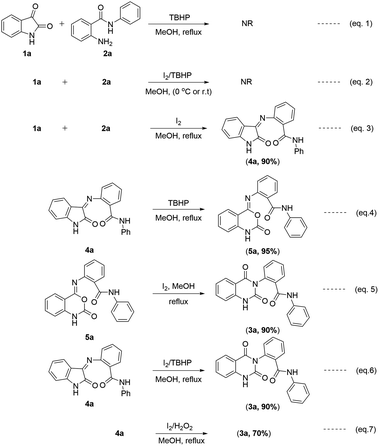 |
| Scheme 2 Study of control experiments. | |
In view of the observations described above, the plausible reaction mechanism for the domino reaction of isatin 1a and 2-amino-N-phenyl benzamide 2a in the presence of I2/TBHP is shown in Scheme 3. Here, the compound 4a is initially produced by the condensation of isatin 1a and 2-amino N-phenyl benzamide 2a under catalysis of iodine. Next 4a transforms into 3a through a domino reaction sequence promoted by I2/TBHP under optimized conditions.
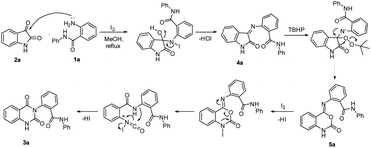 |
| Scheme 3 Plausible mechanism for the formation of 2-(2,4-dioxo-1,2-dihydroquinazolin-3(4H)-yl)-N-phenylbenzamide (3a). | |
Bioactivity studies
(i) Cytotoxicity assay. The synthesized derivatives were screened for their cytotoxicity towards cancer cell lines A549, B16-F10, DU145, and HepG2 and non-cancerous cell line CHO-K1 by MTT assay using doxorubicin as a standard drug. The cytotoxicity results expressed as IC50 values are depicted in Table 5.
Table 5 In vitro cytotoxicity of 2-(2,4-dioxo-1,4-dihydroquinazolin-3(2H)-yl)-N-aryl/alkyl benzamides derivatives against A549, B16-F10, DU145, HepG2 and CHO-K1 cell linesa
Sr. no. |
Compounds |
IC50 values (μM) |
A549 |
B16-F10 |
DU145 |
HEPG2 |
CHO |
A549 – Homo sapiens lung carcinoma (ATCC® CCL-185™); B16-F10 – Mus musculus mouse skin melanoma (ATCC® CRL-6475™); DU145 – Homo sapiens prostrate carcinoma (ATCC® HTB-81™); Hep G2 – Homo sapiens hepatocellular carcinoma (ATCC® HB-8065™); CHO-K1 – Cricetulus griseus chinese hamster ovary cells (ATCC® CCL-61™); 5FU, 5-flourouracil, positive control, doxorubicin as standard drug-for comparison, NA – no activity. |
1 |
3a |
29.40 ± 0.45 |
39.51 ± 0.13 |
14.83 ± 0.13 |
8.73 ± 0.02 |
NA |
2 |
3b |
19.47 ± 0.25 |
24.78 ± 0.70 |
11.56 ± 0.06 |
23.30 ± 0.52 |
NA |
3 |
3c |
15.15 ± 0.70 |
17.90 ± 0.17 |
11.49 ± 0.04 |
5.33 ± 0.12 |
NA |
4 |
3d |
21.02 ± 0.12 |
25.77 ± 0.13 |
12.84 ± 0.01 |
14.19 ± 0.13 |
NA |
5 |
3e |
11.65 ± 0.14 |
14.67 ± 0.05 |
12.05 ± 0.02 |
9.48 ± 0.05 |
69.41 ± 5.17 |
6 |
3f |
16.44 ± 0.53 |
43.95 ± 0.26 |
11.06 ± 0.34 |
14.39 ± 0.02 |
NA |
7 |
3g |
30.41 ± 5.52 |
20.51 ± 0.91 |
18.65 ± 0.08 |
15.30 ± 0.78 |
NA |
8 |
3h |
34.76 ± 4.65 |
27.14 ± 0.68 |
10.44 ± 0.02 |
16.71 ± 1.72 |
94.21 ± 2.44 |
10 |
3i |
19.32 ± ±0.09 |
32.41 ± 0.13 |
11.47 ± 0.19 |
10.09 ± 0.07 |
83.21 ± 1.32 |
11 |
3j |
30.38 ± 1.35 |
34.34 ± 1.55 |
12.33 ± 0.17 |
8.74 ± 0.34 |
NA |
12 |
3k |
17.17 ± 0.41 |
29.99 ± 0.42 |
11.59 ± 0.06 |
28.68 ± 0.92 |
NA |
13 |
3l |
17.42 ± 0.18 |
28.47 ± 0.31 |
12.63 ± 0.08 |
7.77 ± 0.02 |
51.54 ± 2.74 |
14 |
3m |
26.27 ± 1.32 |
41.42 ± 0.81 |
19.39 ± 0.14 |
20.18 ± 0.31 |
88.04 ± 3.56 |
15 |
3n |
14.69 ± 0.18 |
14.83 ± 0.01 |
10.43 ± 0.13 |
12.30 ± 0.26 |
73.88 ± 2.81 |
16 |
3o |
26.32 ± 1.03 |
16.03 ± 0.06 |
5.17 ± 0.07 |
3.02 ± 0.06 |
74.53 ± 9.44 |
17 |
3p |
22.75 ± 0.71 |
31.67 ± 0.14 |
13.02 ± 0.03 |
33.88 ± 0.71 |
NA |
18 |
3q |
28.67 ± 1.38 |
36.46 ± 0.10 |
9.37 ± 0.03 |
8.51 ± 0.20 |
NA |
19 |
3r |
16.70 ± 0.30 |
33.13 ± 0.12 |
15.59 ± 0.07 |
15.95 ± 0.51 |
NA |
19 |
4a |
23.76 ± 0.12 |
22.17 ± 0.56 |
17.37 ± 0.32 |
16.04 ± 0.41 |
NA |
20 |
5FU |
29.64 ± 1.64 |
7.55 ± 0.01 |
16.32 ± 0.02 |
22.97 ± 0.27 |
NA |
Doxorubicin |
0.55 ± 0.16 |
0.7 ± 0.56 |
0.363 ± 0.01 |
0.72 ± 0.012 |
8.8.1 ± 1.04 |
Interestingly all the synthesized compounds exhibited significant cytotoxicity to cancer cell lines as compared to normal cell line. Predominantly, most of the compounds exhibited promising activity against DU145 and HepG2 cell lines. Notably, amongst all the derivatives, compounds 3o, 3c and 3l displayed the most potent activity against DU145 and HepG2. From the structure–activity relationship perspective, it was seen that type of substitutions on quinazoline and amide pharmacophores affects the cytotoxic activity. Different substitutions on quinazoline ring like –Cl, –H, –F and –NO2 showed activity in the order of Cl > H > F > NO2. Whereas, substitutions on amide functionality like aromatic and aliphatic also influenced the cytotoxicity. Here, N-aryl functionality showed high activity as compared to N-alkyl functionality. Type of substitutions on aromatic ring also imparted effect on cytotoxicity which follows the order F > Br > Me > OMe. Compound 3o having chlorine substitution on quinazoline pharmacophore with fluorine on aromatic ring of amide functionality displayed highly potent cytotoxicity. The compounds 3c and 3l are the next potent derivatives amongst the series. Here, 3c has no substitution on quinazoline moiety and its amide functionality is substitution of N-(4-Me)Ph. In 3l, fluorine substitution is present on quinazoline moiety and amide functionality is substituted with N-(4-Br)Ph. These observations suggest that presence of electron-withdrawing group on both the pharmacophores may be a promising factor for the high cytotoxicity.
(ii) Docking studies. Docking studies were implemented to validate the biological studies and to explore the plausible modes of interaction between the potent derivatives 3o, 3c, 3l and DNA [Protein Data Bank (PDB) 1N37].26 All the potent compounds were docked using AutoDock 4.2.6 against the promising anticancer target. For each ligand, the docking score was calculated in terms of kcal mol−1. All the screened compounds, 3c, 3l and 3o were found to interact with DG B:13, DG A:5, DC B:12 and DC A:4. The molecular docking results are tabulated in Table 6.
Table 6 Molecular docking studies on DNA (PBD ID 1N37)
Compounds |
Binding energy (kcal mol−1) |
Inhibition constant (Ki) |
H-bonds |
π–π stacking |
Nucleotides |
Interacting atoms |
Nucleotides |
Interacting atoms |
3c |
−9.13 |
0.20 μM |
DG A:5 |
Quinazoline ketone |
DG B:13, DG A:5, DC B:12, DC A:4 |
C C in amide aromatic ring |
3l |
−8.31 |
0.81 μM |
DG A:5 |
Quinazoline ketone |
DG B: 13, DG A: 5, DC B: 12, DC A: 4 |
C C in amide aromatic ring |
3o |
−8.04 |
1.28 μM |
DG B:13 |
Quinazoline ketone |
DG B: 13, DG A: 5, DC B: 12, DC A: 4 |
C C in quinazoline aromatic ring |
DG A:5 |
Amide ketone |
Doxo-rubicin |
−8.98 |
0.26 μM |
DG B:13, DC A:4 |
Oxygen present in ester |
DG B: 13, DC A: 4, DC B:12, DG A:5 |
C C in amide aromatic ring |
Binding interactions like hydrogen bond and π–π interactions, binding energies and inhibition constants of the molecules are used to explain the affinity of a molecule with DNA and stability of the complex. Fig. 2(A–C) depicts the binding interaction of 3c, 3l, and 3o with DNA. From the figures it is clear that the molecules have been intercalated between the DNA base pairs. Docking studies showed that the compound 3c exhibits strong H-bond interactions with higher binding energy (−9.13 kcal mol−1) as compared to compounds 3l (−8.31 kcal mol−1) and 3o (−8.04 kcal mol−1).
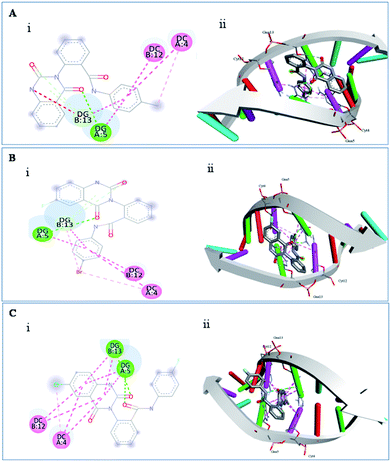 |
| Fig. 2 Molecular docking (i) 2D and (ii) 3D binding interactions of (A) 3c, (B) 3l and (C) 3o with the DNA (PDB 1N37); green lines correspond to hydrogen bonding and pink lines correspond to π–π stacking interactions with DNA. | |
Conclusions
We have developed a new and efficient metal-free oxidative domino reaction for preparation of 2-(2,4-dioxo-1,4-dihydro quinazolin-3(2H)-yl)-N-aryl/alkyl benzamides by reaction of an isatins with 2-amino N-aryl/alkyl benzamides using I2/TBHP under mild conditions. These 2-(2,4-dioxo-1,4-dihydro quinazolin-3(2H)-yl)-N-aryl/alkyl benzamides consisted of quinazolines and amide moieties, which are important pharmacophores in several anticancer agents. In this study, the synthesized compounds also were found to display excellent cytotoxicity towards tested cancer cell lines A549, B16-F10, DU145, and HepG2 and no or lesser cytotoxic effects towards normal CHO-K1 cells. Especially, compounds 3c, 3l and 3o exhibited promising bioactivity at micro molar concentration and these were found to be more potent than 5-flourouracil towards different cancer cell lines. Moreover, docking studies accomplished on these compounds also validated the results of cytotoxicity studies. The present study reveals that the compounds 3c, 3l and 3o have potent therapeutic applications for treatment of different types of cancers.
Experimental section
General information
Melting points of all the compounds were recorded on Veego programmable melting point apparatus and are uncorrected. IR spectra were recorded on a PerkinElmer FT-IR 240-C spectrophotometer using KBr optics. 1H NMR and 13C NMR spectra were recorded on Bruker AV 400 MHz in CDCl3 and DMSO-d6 using TMS as internal standard. High resolution mass spectra (HRMS) [ESI+] were obtained using either a TOF or a double focusing spectrometer, micro mass VG 70-70H or LC/MSD trap SL spectrometer operating at 70 eV using direct inlet system. All the reactions were monitored by thin layer chromatography (TLC) on percolated silica gel 60 F254 (mesh); spots were visualized with UV light. Merck silica gel (60–120 mesh) was used for column chromatography.
Typical procedure for synthesis of 2-(2,4-dioxo-1,2-dihydroquinazolin-3(4H)-yl)-N-phenylbenzamide (3a). Isatin 1a (0.30 g, 2.04 mmol), 2-amino-N-phenylbenzamide 2a (0.43 g, 2.04 mmol), iodine (0.15 g, 0.61 mmol), TBHP (0.56 ml, 70% aq, 4.08 mmol) and MeOH (10 mL) were taken into a round bottomed flask fitted with a condenser. The reaction mixture was stirred under reflux for 6 hours and progress of the reaction was monitored by TLC. Upon the completion of this reaction (monitored by TLC), the mixture was evaporated in vacuum, diluted with ethyl acetate (3 × 10 mL), and washed by hypo solution. The combined organic layer was washed with brine solution, dried over anhydrous Na2SO4 and concentrated under reduced pressure. The crude product was purified by normal column chromatography (silica gel 60–120 mesh, gradient solvent system of hexane–EtOAc) furnished 2-(2,4-dioxo-1,2-dihydroquinazolin-3(4H)-yl)-N-phenylbenzamide 3a (0.62 g, 85%) as a white solid which gave satisfactory characterization data: mp: 274–276 °C.1H NMR (400 MHz, DMSO-d6): δ 11.51 (1H, s), 10.37 (1H, s), 7.93–7.90 (1H, m), 7.82–7.80 (1H, m), 7.71–7.64 (2H, m), 7.60 (3H, d, J = 7.6 Hz), 7.47–7.45 (1H, m), 7.28–7.19 (4H, m), 7.05–7.01 (1H, m); 13C NMR (100 MHz, DMSO-d6): δ 163.69, 162.39, 150.26, 140.25, 140.21, 139.44, 137.52, 136.45, 135.81, 134.64, 133.31, 129.06, 128.12, 124.21, 123.09, 120.47, 115.78, 114.55, 94.96; FT-IR (KBr): ν 3281, 3220, 2951, 1715, 1660, 1439, 1321, 1268, 756 cm−1; ESI-MS: m/z 358 [M + H]+; ESI-HRMS: calcd for C21H16N3O3 [M + H]+ 358.11862; found: 358.11995.
2-(2,4-dioxo-1,2-dihydroquinazolin-3(4H)-yl)-N-phenylbenzamide (3a). White solid; (yield: 85%), mp: 274–276 °C; 1H NMR (400 MHz, DMSO-d6): δ 11.51 (1H, s), 10.37 (1H, s), 7.93–7.90 (1H, m), 7.82–7.80 (1H, m), 7.71–7.64 (2H, m), 7.60 (3H, d, J = 7.6 Hz), 7.47–7.45 (1H, m), 7.28–7.19 (4H, m), 7.05–7.01 (1H, m); 13C NMR (100 MHz, DMSO-d6): δ 163.69, 162.39, 150.26, 140.25, 140.21, 139.44, 137.52, 136.45, 135.81, 134.64, 133.31, 129.06, 128.12, 124.21, 123.09, 120.47, 115.78, 114.55, 94.96; FT-IR (KBr): ν 3281, 3220, 2951, 1715, 1660, 1439, 1321, 1268, 756 cm−1; ESI-MS: m/z 358 [M + H]+; ESI-HRMS: calcd for C21H16N3O3 [M + H]+ 358.11862; found: 358.11995.
2-(2,4-dioxo-1,2-dihydroquinazolin-3(4H)-yl)-N-(4-methoxy phenyl)benzamide (3b). Pale brown solid; (yield: 82%), mp: 255–257 °C; 1H NMR (400 MHz, CDCl3 + DMSO-d6): δ 11.39 (1H, s), 9.99 (1H, s), 7.99 (1H, d, J = 7.9 Hz), 7.81 (1H, d, J = 7.3 Hz), 7.61–7.51 (5H, m), 7.37–7.35 (1H, m), 7.22 (1H, d, J = 8.1 Hz), 7.16 (1H, t, J = 7.5 Hz), 6.78–6.76 (2H, m), 3.75–3.71 (3H, m); 13C NMR (100 MHz, CDCl3 + DMSO-d6): δ 169.65, 167.53, 160.67, 155.49, 144.88, 139.87, 139.82, 139.11, 137.18, 135.71, 135.24, 133.81, 133.27, 132.82, 127.36, 126.63, 120.44, 119.33, 118.54, 60.17; FT-IR (KBr): ν 3291, 3212, 2954, 1722, 1664, 1436, 1326, 1278, 759 cm−1; ESI-MS: m/z 388 [M + H]+; ESI-HRMS: calcd for C22H18N3O4 [M + H]+ 388.12918; found: 388.13114.
2-(2,4-dioxo-1,2-dihydroquinazolin-3(4H)-yl)-N-(p-tolyl)benzamide (3c). White solid; (yield: 80%), mp: 245–247 °C; 1H NMR (400 MHz, CDCl3 + DMSO-d6): δ 11.36 (1H, s), 9.84 (1H, s), 8.01 (1H, d, J = 7.9 Hz), 7.82 (1H, d, J = 7.5 Hz), 7.61–7.54 (3H, m), 7.49 (2H, d, J = 7.7 Hz), 7.36 (1H, d, J = 7.7 Hz), 7.21 (1H, d, J = 8.1 Hz), 7.16 (1H, t, J = 7.5 Hz), 7.03 (2H, d, J = 7.6 Hz), 5.40 (3H, s); 13C NMR (100 MHz, CDCl3 + DMSO-d6): δ 169.80, 167.66, 155.59, 144.80, 141.30, 139.96, 139.84, 138.91, 138.31, 137.96, 135.80, 135.14, 133.88, 133.37, 132.86, 127.41, 125.03, 120.46, 119.28, 25.63; FT-IR (KBr): ν 3305, 3251, 2985, 1716, 1662, 1441, 1327, 1265, 753 cm−1; ESI-MS: m/z 372 [M + H]+; ESI-HRMS: calcd for C22H18N3O3 [M + H]+ 372.13427; found: 372.13600.
2-(2,4-dioxo-1,2-dihydroquinazolin-3(4H)-yl)-N-(4-fluorophenyl) benzamide (3d). White solid; (yield: 70%), mp: 277–279 °C; 1H NMR (400 MHz, CDCl3 + DMSO-d6): δ 11.40 (1H, s), 10.25 (1H, s), 7.99 (1H, d, J = 7.8 Hz), 7.82 (1H, d, J = 6.9 Hz), 7.62–7.55 (5H, m), 7.37 (1H, d, J = 7.5 Hz), 7.22 (1H, d, J = 8.0 Hz), 7.16 (1H, t, J = 7.4 Hz), 6.94 (2H, t, J = 8.4 Hz); 13C NMR (100 MHz, CDCl3 + DMSO-d6): δ 169.90, 167.44, 164.67, 162.27, 155.39, 144.91, 140.40, 139.89, 139.54, 139.28, 135.89, 135.40, 133.82, 133.26, 132.80, 127.40, 126.76, 126.69, 120.46, 119.80, 119.32; FT-IR (KBr): ν 3291, 3226, 2965, 1721, 1663, 1436, 1315, 1260, 751 cm−1;ESI-MS: m/z 376 [M + H]+; ESI-HRMS: calcd for C21H15FN3O3 [M + H]+ 376.10920; found: 376.11086.
N-(4-Bromophenyl)-2-(2,4-dioxo-1,2-dihydroquinazolin-3(4H)-yl)benzamide (3e). Wheatish solid; (yield: 78%), mp: 261–263 °C; 1H NMR (400 MHz, CDCl3 + DMSO-d6): δ 11.43 (1H, s), 10.40 (1H, s), 7.97 (1H, d, J = 7.9 Hz), 7.81 (1H, d, J = 7.6 Hz), 7.65–7.53 (5H, m), 7.39–7.33 (3H, m), 7.22 (1H, d, J = 8.1 Hz), 7.17 (1H, t, J = 11.1 Hz); 13C NMR (100 MHz, CDCl3 + DMSO-d6): δ 170.08, 167.53, 155.47, 144.85, 143.42, 139.91, 139.46, 139.22, 136.23, 136.04, 135.35, 133.86, 133.31, 132.84, 127.45, 126.73, 120.66, 120.49, 119.28; FT-IR (KBr): ν 3289, 3212, 2978, 1720, 1662, 1448, 1329, 1265, 752 cm−1; ESI-MS: m/z 436 [M + H]+; ESI-HRMS: calcd for C21H15BrN3O3 [M + H]+ 436.02913; found: 436.03052.
2-(2,4-Dioxo-1,2-dihydroquinazolin-3(4H)-yl)-N-phenethyl benzamide (3f). White solid; (yield: 72%), mp: 207–209 °C; 1H NMR (400 MHz, CDCl3 + DMSO-d6): δ 11.36 (1H, s), 8.00 (1H, d, J = 7.9 Hz), 7.82 (1H, s), 7.64–7.54 (3H, m), 7.48–7.45 (1H, m), 7.30 (1H, d, J = 7.8 Hz), 7.25–7.22 (3H, m), 7.17–7.15 (4H, m), 3.11 (2H, t, J = 9.8 Hz), 2.74 (2H, t, J = 7.1 Hz); 13C NMR (100 MHz, CDCl3 + DMSO-d6): δ 171.33, 167.51, 155.48, 145.01, 144.36, 139.78, 139.58, 139.11, 135.54, 135.27, 133.60, 133.41, 133.26, 133.20, 132.73, 130.98, 127.30, 120.47, 119.41, 45.82, 40.16; FT-IR (KBr): ν 3285, 3215, 2976, 1718, 1664, 1436, 1326, 1265, 752 cm−1; ESI-MS: m/z 386 [M + H]+; ESI-HRMS: calcd for C23H20N3O3 [M + H]+ 386.14992; found: 386.15168.
N-Butyl-5-chloro-2-(2,4-dioxo-1,2-dihydroquinazolin-3(4H)-yl)benzamide (3g). Wheatish solid; (yield: 65%), mp: 203–205 °C; 1H NMR (400 MHz, CDCl3 + DMSO-d6): δ 11.46 (1H, s), 8.18 (1H, s), 7.96 (1H, d, J = 7.5 Hz), 7.68 (1H, s), 7.61–7.53 (2H, m), 7.31 (1H, d, J = 8.1 Hz), 7.23 (1H, d, J = 8.0 Hz), 7.16 (1H, t, J = 7.4 Hz), 1.39 (1H, t, J = 6.7 Hz), 1.28–1.19 (4H, m), 0.81 (3H, t, J = 7.0 Hz); 13C NMR (100 MHz, CDCl3 + DMSO-d6): δ 169.87, 167.37, 155.28, 144.97, 141.37, 139.92, 138.44, 137.77, 136.93, 135.25, 133.54, 132.71, 127.38, 120.50, 119.28, 43.98, 36.12, 24.68, 18.71; FT-IR (KBr): ν 3279, 3216, 2975, 1718, 1663, 1425, 1310, 1240, 761 cm−1; ESI-MS: m/z 372 [M + H]+; ESI-HRMS: calcd for C19H19N3O3Cl [M + H]+ 372.11095; found: 372.11240.
2-(2,4-Dioxo-1,2-dihydroquinazolin-3(4H)-yl)-N-ethylbenzamide (3h). Pale brown solid; (yield: 75%), mp: 286–288 °C; 1H NMR (400 MHz, CDCl3 + DMSO-d6): δ 11.40 (1H, s), 7.99 (1H, d, J = 7.8 Hz), 7.85 (1H, s), 7.68 (1H, d, J = 7.5 Hz), 7.61–7.54 (2H, m), 7.48 (1H, t, J = 7.5 Hz), 7.30 (1H, d, J = 7.7 Hz), 7.24 (1H, d, J = 8.2 Hz), 7.16 (1H, t, J = 7.6 Hz), 3.18 (2H, q, J = 13.2, 7.1 Hz), 1.04 (3H, t, J = 7.2 Hz); 13C NMR (100 MHz, CDCl3 + DMSO-d6): δ 171.08, 167.44, 155.42, 145.01, 139.82, 139.73, 139.11, 135.42, 135.31, 133.37, 133.26, 132.70, 127.31, 120.43, 119.42, 39.04, 19.53; FT-IR (KBr): ν 3281, 3245, 2925, 1723, 1662, 1435, 1365, 1248, 748 cm−1; ESI-MS: m/z 310 [M + H]+; ESI-HRMS: calcd for C17H16N3O3 [M + H]+ 310.11862; found: 310.11935.
2-(6-Fluoro-2,4-dioxo-1,2-dihydroquinazolin-3(4H)-yl)-N-phenyl benzamide (3i). Wheatish solid; (yield: 78%), mp: 160–162 °C; 1H NMR (400 MHz, CDCl3 + DMSO-d6): δ 11.46 (1H, s), 10.13 (1H, s), 7.81 (1H, d, J = 10.0 Hz), 7.61–7.54 (5H, m), 7.39–7.31 (3H, m), 7.23 (3H, d, J = 5.9 Hz), 7.04–6.99 (1H, m); 13C NMR (100 MHz, CDCl3 + DMSO-d6): δ 169.96, 166.77, 163.92, 161.52, 155.17, 144.09, 141.43, 139.62, 139.03, 135.90, 135.23, 133.94, 133.42, 128.58, 127.97, 127.73, 125.08, 122.60, 120.34, 120.26, 118.04, 117.80; FT-IR (KBr): ν 3279, 3215, 2987, 1721, 1661, 1438, 1325, 1265, 751 cm−1; ESI-MS: m/z 376 [M + H]+; ESI-HRMS: calcd for C21H15FN3O3 [M + H]+ 376.10920; found: 376.11088.
2-(6-Fluoro-2,4-dioxo-1,2-dihydroquinazolin-3(4H)-yl)-N-(4-methoxyphenyl)benzamide (3j). Pale brown solid; (yield: 78%), mp: 160–162 °C; 1H NMR (400 MHz, CDCl3 + DMSO-d6): δ 11.50 (1H, s), 10.05 (1H, s), 7.84 (3H, t, J = 8.8 Hz), 7.66–7.62 (2H, m), 7.57–7.51 (3H, m), 7.39–7.32 (2H, m), 7.28–7.22 (1H, m), 6.79 (2H, J = 8.9 Hz), 3.76 (3H, s); 13C NMR (100 MHz, CDCl3 + DMSO-d6): δ 169.61, 166.80, 163.91, 161.51, 160.71, 155.20, 141.42, 139.70, 138.93, 137.10, 135.79, 135.15, 133.86, 133.40, 127.92, 127.68, 126.69, 122.57, 120.33, 118.55, 118.02, 117.78, 60.17; FT-IR (KBr): ν 3289, 3210, 2995, 1723, 1661, 1510, 1440, 1344, 1247, 752 cm−1; ESI-MS: m/z 406 [M + H]+; ESI-HRMS: calcd for C22H17N3O4F [M + H]+ 406.11976; found: 406.12108.
2-(6-Fluoro-2,4-dioxo-1,2-dihydroquinazolin-3(4H)-yl)-N-(4-fluorophenyl)benzamide (3k). Pale gray solid; (yield: 68%), mp: 262–264 °C; 1H NMR (400 MHz, CDCl3 + DMSO-d6): δ 11.46 (1H, s), 10.20 (1H, s), 7.83 (1H, d, J = 7.3 Hz), 7.65–7.61 (4H, m), 7.55 (1H, t, J = 7.4 Hz), 7.38–732 (2H, m), 7.26–7.22 (1H, m), 6.94 (2H, t, J = 8.6 Hz); 13C NMR (100 MHz, CDCl3 + DMSO-d6): δ 169.88, 166.81, 164.79, 163.94, 162.38, 161.54, 155.20, 141.40, 140.17, 139.47, 138.98, 135.96, 135.19, 133.89, 133.40, 127.94, 127.70, 126.79, 126.72, 122.58, 120.31, 120.00, 119.78, 118.05, 117.81; FT-IR (KBr): ν 3286, 3212, 2968, 1724, 1668, 1435, 1320, 1271, 753 cm−1; ESI-MS: m/z 394 [M + H]+; ESI-HRMS: calcd for C21H14N3O3F2 [M + H]+ 394.09977; found: 394.10152.
N-(4-Bromophenyl)-2-(6-fluoro-2,4-dioxo-1,2-dihydroquinazolin-3(4H)-yl)benzamide (3l). Cream solid; (yield: 72%), mp: 272–274 °C; 1H NMR (400 MHz, CDCl3 + DMSO-d6): δ 11.54 (1H, s), 10.45 (1H, s), 7.82 (1H, d, J = 6.5 Hz), 7.64–7.55 (5H, m), 7.38–7.35 (4H, m), 7.26 (1H, s); 13C NMR (100 MHz, CDCl3 + DMSO-d6): δ 169.98, 166.60, 163.86, 161.46, 155.01, 143.60, 141.54, 139.24, 136.29, 136.11, 135.48, 133.89, 133.41, 128.16, 127.92, 126.83, 122.72, 122.64, 120.54, 120.33, 120.25, 117.93, 117.69; FT-IR (KBr): ν 3282, 3219, 2956, 1721, 1663, 1446, 1329, 1271, 758 cm−1; ESI-MS: m/z 454 [M + H]+; ESI-HRMS: calcd for C21H14N3O3BrF [M + H]+ 454.01971; found: 454.02096.
N-Ethyl-2-(6-fluoro-2,4-dioxo-1,2-dihydroquinazolin-3(4H)-yl)benzamide (3m). Brown solid; (yield: 76%), mp: 225–227 °C; 1H NMR: (400 MHz, CDCl3 + DMSO-d6): δ 11.21 (1H, s), 7.71–7.63 (2H, m), 7.55 (1H, d, J = 7.5 Hz), 7.48 (1H, t, J = 7.3 Hz), 7.29 (2H, t, J = 7.3 Hz), 7.22–7.18 (1H, m), 6.72 (1H, s), 3.28–3.25 (2H, m), 1.02 (3H, t, J = 6.9 Hz); 13C NMR (100 MHz, CDCl3 + DMSO-d6): δ 171.16, 166.80, 163.89, 161.49, 155.23, 141.50, 139.64, 138.77, 135.51, 135.07, 133.42, 127.85, 127.61, 122.59, 122.52, 120.37, 120.29, 117.89, 117.65, 39.13, 19.46; FT-IR (KBr): ν 3275, 3205, 2916, 1716, 1675, 1435, 1358, 1271, 751 cm−1; ESI-MS: m/z328 [M + H]+; ESI-HRMS: calcd forC17H15N3O3F [M + H]+ 328.10147; found: 328.10210.
2-(6-Chloro-2,4-dioxo-1,2-dihydroquinazolin-3(4H)-yl)-N-phenylbenzamide (3n). White solid; (yield: 75%), mp: 165–167 °C; 1H NMR (400 MHz, CDCl3 + DMSO-d6): δ 11.60 (1H, s), 10.28 (1H, s), 7.91 (1H, s), 7.83 (1H, s), 7.63–7.55 (5H, m), 7.40–7.34 (1H, m), 7.24–7.21 (3H, m), 7.01 (2H, d, J = 5.0 Hz); 13C NMR (100 MHz, CDCl3 + DMSO-d6): δ 175.41, 169.93, 166.48, 155.12, 144.14, 143.60, 139.83, 139.55, 139.02, 135.91, 135.25, 133.97, 133.42, 132.29, 131.99, 128.57, 125.08, 122.40, 120.56; FT-IR (KBr): ν 3297, 3249, 3077, 1722, 1666, 1438, 1324, 1273, 754 cm−1; ESI-MS: m/z 392 [M + H]+; ESI-HRMS: calcd for C21H15ClN3O3 [M + H]+ 392.07965; found: 392.08167.
2-(6-Chloro-2,4-dioxo-1,2-dihydroquinazolin-3(4H)-yl)-N-(4-fluorophenyl)benzamide (3o). Wheatish solid; (yield: 68%), mp: 265–267 °C; 1H NMR (400 MHz, CDCl3 + DMSO-d6): δ 11.46 (1H, s), 10.00 (1H, s), 7.96 (1H, d, J = 2.4 Hz), 7.83 (1H, d, J = 7.5 Hz), 7.64–7.59 (3H, m), 7.55 (1H, d, J = 7.4 Hz), 7.51–7.48 (1H, m), 7.36 (1H, d, J = 7.8 Hz), 7.19 (1H, d, J = 8.7 Hz), 6.94 (2H, t, J = 8.8 Hz); 13C NMR (100 MHz, CDCl3 + DMSO-d6): δ 169.85, 166.62, 164.85, 162.44, 155.28, 143.48, 140.07, 139.79, 139.46, 138.86, 136.01, 135.13, 133.92, 133.46, 132.51, 132.12, 126.76, 126.69, 122.30, 120.48, 120.01, 119.79; FT-IR (KBr): ν 3302, 3240, 2922, 1731, 1661, 1503, 1370, 1224, 750 cm−1; ESI-MS: m/z 410 [M + H]+; ESI-HRMS: calcd for C21H14N3O3ClF [M + H]+ 410.07022; found: 410.07150.
2-(6-Chloro-2,4-dioxo-1,2-dihydroquinazolin-3(4H)-yl)-N-ethylbenzamide(3p). Pale brown solid; (yield: 74%), mp: 280–282 °C; 1H NMR (400 MHz, CDCl3 + DMSO-d6): δ 11.37 (1H, s), 8.32 (1H, s), 8.00 (2H, s), 7.78 (1H, d, J = 6.8 Hz), 7.66 (1H, d, J = 7.6 Hz), 7.30 (1H, d, J = 7.6 Hz), 7.20 (1H, d, J = 8.6 Hz), 6.68 (1H, s), 3.27 (2H, q, J = 12.8, 6.0 Hz), 1.05 (3H, t, J = 7.3 Hz); 13C NMR (100 MHz, CDCl3 + DMSO-d6): δ 171.02, 166.45, 155.13, 143.71, 139.74, 139.47, 138.88, 135.50, 135.19, 133.45, 133.38, 132.09, 131.82, 122.42, 120.64, 39.08, 19.52; FT-IR (KBr): ν 3301, 3245, 2941, 1726, 1665, 1462, 1352, 1245, 753 cm−1; ESI-MS: m/z 344 [M + H]+; ESI-HRMS: calcd for C17H15N3O3Cl [M + H]+ 344.07965; found: 344.07831.
N-Butyl-5-chloro-2-(6-chloro-2,4-dioxo-1,2-dihydroquinazolin-3(4H)-yl)benzamide (3q). Pale brown solid; (yield: 72%), mp: 205–207 °C; 1H NMR (400 MHz, CDCl3 + DMSO-d6): δ 11.59 (1H, s), 8.03 (1H, s), 7.93–7.92 (1H, m), 7.70 (1H, s), 7.54–7.52 (2H, m), 7.29–7.26 (1H, m), 7.23 (1H, d, J = 8.7 Hz), 1.41 (2H, t, J = 6.7 Hz), 1.31–1.22 (4H, m), 0.85–0.81 (3H, t, J = 7.3 Hz); 13C NMR (100 MHz, CDCl3 + DMSO-d6): δ 169.92, 166.48, 155.05, 143.58, 141.16, 139.85, 138.78, 137.30, 136.63, 135.37, 133.62, 132.36, 131.90, 122.44, 120.42, 44.08, 36.11, 24.69, 18.65; FT-IR(KBr): ν 3292, 3216, 2997, 1723, 1665, 1436, 1329, 1245, 750 cm−1; ESI-MS: m/z 406 [M + H]+; ESI-HRMS: calcd for C19H18N3O3Cl2 [M + H]+ 406.07197; found: 406.07371.
2-(6-Nitro-2,4-dioxo-1,2-dihydroquinazolin-3(4H)-yl)-N-phenylbenzamide (3r). Pale yellow solid; (yield: 68%), mp: 195–198 °C; 1H NMR (400 MHz, CDCl3 + DMSO-d6): δ 12.13 (1H, s), 10.33 (1H, s), 8.79–8.77 (1H, m), 8.41 (1H, d, J = 8.9 Hz), 7.87 (1H, d, J = 7.3 Hz), 7.68–7.57 (4H, m), 7.42–7.39 (2H, m), 7.23 (2H, t, J = 7.6 Hz), 7.02 (1H, t, J = 7.1 Hz); 13C NMR (100 MHz, CDCl3 + DMSO-d6): δ 169.82, 166.10, 154.94, 149.58, 147.32, 144.06, 139.19, 138.65, 136.11, 135.22, 134.50, 134.10, 133.68, 133.44, 129.36, 128.65, 125.16, 121.84, 119.30; FT-IR (KBr): ν 3285, 3234, 2960, 1718, 1663, 1446, 1322, 1261, 751 cm−1; ESI-MS: m/z 403 [M + H]+; ESI-HRMS: calcd for C21H15N4O5 [M + H]+ 403.10370; found: 403.10511.
2-(2,4-Dioxo-1,4-dihydroquinazolin-3(2H)-yl)-5-methoxy-N-phenylbenzamide (3s). Brown solid; (yield: 81%), mp: 234–236 °C; 1H NMR (400 MHz, CDCl3 + DMSO-d6): δ 11.03 (1H, s), 9.38 (1H, s), 7.71 (2H, d, J = 7.3 Hz), 7.51 (2H, d, J 7.4 Hz), 7.33–7.21 (5H, m), 7.05 (2H, t, J = 7.9 Hz), 6.92 (1H, t, J = 8.5 Hz), 6.86 (1H, d, J = 7.7 Hz), 6.48 (2H, t, J = 8.5 Hz), 3.44 (3H, s); 13C NMR (100 MHz, CDCl3 + DMSO-d6): δ 163.71, 161.63, 154.73, 149.59, 138.91, 133.98, 133.85, 133.10, 131.19, 129.77, 129.25, 127.87, 127.34, 126.89, 121.41, 120.66, 114.49, 113.38, 112.59, 54.22; FT-IR (KBr): ν 3273, 3214, 2936, 1719, 1659, 1439, 1321, 1271, 753 cm−1; ESI-MS: m/z 388 [M + H]+; ESI-HRMS: calcd for C22H18N3O4 [M + H]+ 388.12918; found: 388.12973.
5-Chloro-2-(2,4-dioxo-1,4-dihydroquinazolin-3(2H)-yl)-N-phenylbenzamide (3t). Wheatish solid; (yield: 71%), mp: 245–247 °C; 1H NMR (400 MHz, CDCl3 + DMSO-d6): δ 11.83 (1H, s), 10.54 (1H, s), 8.08 (1H, s), 8.03 (1H, d, J = 6.8 Hz), 7.83–7.77 (5H, m), 7.59 (1H, s), 7.43 (3H, s), 7.22 (1H, d, J = 6.8 Hz); 13C NMR (100 MHz, CDCl3 + DMSO-d6): δ 164.95, 161.58, 150.23, 139.05, 138.56, 134.82, 134.63, 133.93, 130.95, 130.18, 128.99, 128.44, 127.43, 127.10, 123.61, 120.09, 117.37, 115.55; FT-IR (KBr): ν 3280, 3229, 2985, 1716, 1653, 1432, 1312, 1260, 752 cm−1; ESI-MS: m/z 392 [M + H]+; ESI-HRMS: calcd for C21H15ClN3O3 [M + H]+ 392.07965; found: 392.07895.
2-(2,4-Dioxo-1,4-dihydroquinazolin-3(2H)-yl)-5-nitro-N-phenylbenzamide (3u). Pale yellow solid; (yield: 60%), mp: 228–230 °C; 1H NMR (400 MHz, CDCl3 + DMSO-d6): δ 11.69 (1H, s), 9.74 (1H, s), 8.47 (1H, d, J = 2.2 Hz), 8.00 (1H, d, J 6.5 Hz), 7.50 (1H, d, J = 7.5 Hz), 7.28–7.22 (4H, m), 7.02–7.00 (2H, m), 6.86 (2H, t, J = 7.9 Hz), 6.65 (1H, t, J = 7.4 Hz); 13C NMR (100 MHz, CDCl3 + DMSO-d6): δ 164.10, 160.42, 149.33, 143.80, 141.63, 138.14, 133.58, 132.74, 130.36, 129.29, 128.57, 128.38, 127.96, 127.69, 123.89, 122.94, 119.39, 116.01, 113.57; FT-IR (KBr): ν 3275, 3214, 2968, 1721, 1652, 1441, 1345, 1215, 758 cm−1; ESI-MS: m/z 403 [M + H]+; ESI-HRMS: calcd for C21H15N4O5 [M + H]+ 403.10370; found: 403.10461.
5H-Quinazolino[3,2-a]quinazoline-5,12(7H)-dione (6a). Brown solid; (yield: 82%), mp: 236–238 °C; 1H NMR (400 MHz, CDCl3 + DMSO-d6): δ 8.61–8.49 (1H, m), 8.32–8.24 (1H, m), 8.02 (1H, s), 7.81–7.76 (1H, m), 7.73–7.69 (1H, m), 7.55 (1H, d, J = 8.5 Hz), 7.51–7.48 (1H, m), 7.31–7.13 (2H, m); 13C NMR (100 MHz, CDCl3 + DMSO-d6): δ163.39, 161.70, 160.27, 148.65, 144.56, 137.19, 135.03, 134.14, 133.59, 127.38, 126.97, 126.72, 126.10, 123.25, 115.16; FT-IR (KBr): ν 3254, 3011, 1719, 1642, 1451, 1332, 1265, 757 cm−1; ESI-MS: m/z 264 [M + H]+; ESI-HRMS: calcd for C15H10N3O2 [M + H]+ 264.07675; found: 264.07608.
10-Chloro-5H-quinazolino[3,2-a]quinazoline-5,12(7H)-dione (6b). Wheatish solid; (yield: 79%), mp: 182–185 °C; 1H NMR (400 MHz, CDCl3 + DMSO-d6): δ 8.55 (1H, d, J = 3.4 Hz), 8.24 (1H, s), 8.00 (2H, d, J = 3.0 Hz), 7.78–7.75 (1H, m), 7.71–7.68 (1H, m), 7.50–7.47 (2H, m); 13C NMR (100 MHz, CDCl3 + DMSO-d6): δ 167.27, 166.56, 165.15, 153.57, 150.08, 149.46, 147.59, 139.79, 139.02, 134.11, 132.09, 131.61, 130.97, 127.76, 122.20; FT-IR (KBr): ν 3256, 3021, 1722, 1645, 1432, 1341, 1236, 751 cm−1; ESI-MS: m/z 298 [M + H]+; ESI-HRMS: calcd for C15H9N3O2Cl [M + H]+ 298.03125; found: 298.03194.
Typical procedure for synthesis of (Z)-2-((2-oxoindolin-3-ylidene)amino)-N-phenylbenzamide (4a). Isatin 1a (0.30 g, 2.04 mmol), 2-amino-N-phenylbenzamide 2a (0.43 g, 2.04 mmol), iodine (0.15 g, 0.61 mmol) and MeOH (10 mL) were taken into a round bottomed flask fitted with a condenser. The reaction mixture was stirred under reflux for 2 hours and progress of the reaction was monitored by TLC. Upon the completion of this reaction (monitored by TLC), the mixture was evaporated in vacuum, diluted with ethyl acetate (3 × 10 mL), and washed by hypo solution. The combined organic layer was washed with brine solution, dried over anhydrous Na2SO4 and concentrated under reduced pressure. The crude product was purified by normal column chromatography (silica gel 60–120 mesh, gradient solvent system of hexane–EtOAc) furnished (Z)-2-((2-oxoindolin-3-ylidene)amino)-N-phenylbenzamide 4a (0.63 g, 90%)as a pale brown solid which gave satisfactory characterization data.
(Z)-2-((2-Oxoindolin-3-ylidene)amino)-N-phenyl benzamide (4a). Pale brown solid (Yield: 90%), mp: 225–227 °C; 1H NMR (400 MHz, DMSO-d6): δ 11.54 (1H, s), 10.45 (1H, s), 8.12 (1H, d, J = 1.6 Hz), 8.04–8.01 (1H, m Hz), 7.91 (1H, d, J = 7.2 Hz), 7.69 (1H, t, J = 7.0 Hz), 7.58 (2H, d, J = 7.8 Hz), 7.29–7.25 (3H, m), 7.20 (2H, d, J = 7.8 Hz), 7.04 (1H, t, J = 7.4 Hz); 13C NMR (100 MHz, DMSO-d6): δ 163.69, 162.39, 150.26, 140.25, 140.21, 139.44, 137.52, 136.45, 135.81, 134.64, 133.31,129.06, 128.12, 124.21, 123.09, 120.47, 115.78, 114.55, 94.96; FT-IR (KBr): ν 3212, 3150, 2920, 1718, 1656, 1428, 1325, 1259, 752 cm−1; ESI-MS: m/z 342 [M + H]+; ESI-HRMS: calcd for C21H16N3O2 [M + H]+ 342.12370; found: 342.12497.
Typical procedure for synthesis of (Z)-2-((2-oxo-1H-benzo[d][1,3]oxazin-4(2H)-ylidene)amino)-N-phenyl benzamide (5a). (Z)-2-((2-Oxoindolin-3-ylidene)amino)-N-phenyl benzamide 4a (0.5 g, 1.46 mmol) and MeOH (10 mL) were taken into a round bottomed flask fitted with a condenser. Then add 70% TBHP (0.4 ml, 2.93 mmol) to reaction mixture was stirred under reflux for 2 hours and progress of the reaction was monitored by TLC. Upon the completion of this reaction (monitored by TLC), the mixture was evaporated in vacuum, diluted with ethyl acetate (3 × 10 mL). The combined organic layer was washed with brine solution, dried over anhydrous Na2SO4 and concentrated under reduced pressure. The crude product was purified by normal column chromatography (silica gel 60–120 mesh, gradient solvent system of hexane–EtOAc) furnished typical procedure for synthesis of (Z)-2-((2-oxo-1H-benzo[d][1,3]oxazin-4(2H)-ylidene)amino)-N-phenylbenzamide 5a (0.49 g, 95%) as a pale brown solid which gave satisfactory characterization data.
(Z)-2-((2-Oxo-1H-benzo[d][1,3]oxazin-4(2H)-ylidene)amino)-N-phenylbenzamide (5a). Pale brown; (yield: 95%), mp: 246–248 °C; 1H NMR (400 MHz, CDCl3): δ 9.63 (1H, s), 8.34 (1H, s), 8.00 (1H, d, J = 7.8 Hz), 7.69 (1H, d, J = 6.1 Hz), 7.45–7.37 (5H, m), 7.22 (1H, d, J = 7.2 Hz), 7.14 (2H, t, J = 7.6 Hz), 7.08 (1H, d, J = 7.4 Hz), 6.96 (1H, t, J = 7.2 Hz), 6.84 (1H, d, J = 7.0 Hz); 13C NMR (100 MHz, CDCl3 + DMSO-d6): δ 165.05, 163.02, 150.92, 139.85, 138.73, 135.31, 135.01, 133.68, 131.15, 130.17, 129.02, 128.71, 128.62, 128.14, 123.81, 122.65, 119.98, 115.62, 114.41; FT-IR (KBr): ν 3244, 3070, 2940, 1716, 1642, 1451, 1321, 1272, 759 cm−1; ESI-MS: m/z 358 [M + H]+; ESI-HRMS: calcd for C21H16N3O3 [M + H]+ 358.11862; found: 358.11718.
MTT assay
All the synthesized compounds were tested for their cytotoxicity efficacy using MTT assay on both cancer and normal cell lines following the protocol of our earlier published literature.27
For this assay, cancer lines A549 – Homo sapiens lung carcinoma (ATCC® CCL-185™); B16-F10 – Mus musculus mouse skin melanoma (ATCC® CRL 6475™); DU145 – Homo sapiens prostrate carcinoma (ATCC® HTB-81™); Hep G2 – Homo sapiens hepatocellular carcinoma (ATCC® HB-8065™); CHO-K1 – Cricetulus griseus Chinese hamster ovary cells (ATCC® CCL-61™) were used. All the cell lines used in this study were obtained from the ATCC (Bethesda, MD, USA) and grown in DMEM with 10% Fetal Bovine (FBS) along with 100 U mL−1 penicillin, 100 μg mL−1 streptomycin and 2 mM L-glutamine. Initially, each cell line was grown in the 96 well culture plate at 37 °C at 5% CO2 in an incubator and allowed to attach properly. After attaining the growth of cells, each test compound at different concentrations (from 1 to 100 μM) was treated to the cells and incubated for 24 h. After the incubation of the cells, MTT (0.5 mg mL−1) was added and further incubated for 3 h. About 100 μl DMSO was added to each well to dissolve the insoluble formazan crystals and finally, the absorbance reading was taken for each plate using a multi-mode plate reader, all the experiments were carried out in triplicate and 50% inhibitory concentration (IC50 value) of each compound was calculated using Graphpad prism-6. Simultaneously, 5-fluorouracil (5FU) and doxorubicin were used as positive control and standard drug respectively for the comparison of cytotoxicity activity of the test compounds.
Molecular docking
In order to explore plausible interaction models of compounds 3c, 3l and 3o, docking studies were performed with the anti-parallel propeller type telomeric DNA and oncogene c-Myc promoter [Protein Data Bank (PDB) entry 1N37].26 The protein and ligand preparation were performed using Discovery studio 2021.28 Molecular docking was carried out using AutoDock 4.2.6 version.29 The structures of 3c, 3l and 3o were drawn and converted into 3D molecules using Discovery studio 2021. Water molecules were eliminated and the polar hydrogen atoms were added to the PDB system. To check the interactions of the ligands with all G-quadruplex DNA conformations, a docking experiment was done on the active site using AutoDock tools-1.5.7 and the best docked structure was taken using a dG-score (G score) function. The DNA–ligand interactions were visualized and the 3D & 2D figures were generated using the Discovery studio 2021. The protein–ligand interactions as g-score are tabulated in Table 6.
Conflicts of interest
The authors declare that they have no conflict of interest.
Acknowledgements
Authors A. K. S., and V. K. E. are thankful to UGC, New Delhi S. K. C. is thankful to CSIR, New Delhi for financial support in the form of research fellowship. I am thankful to DKIM Division (IICT Communication No. IICT/Pubs./2022/094) for the support.
Notes and references
-
(a) N. Kerru, P. Singh, N. Koorbanally, R. Raj and V. Kumar, Eur. J. Med. Chem., 2017, 142, 179 CrossRef CAS;
(b) A. Ouahrouch, H. Ighachane, M. Taourirte, J. W. Engels, M. H. Sedra and H. B. Lazrek, Arch. Pharm. Chem. Life Sci., 2014, 347, 1 CrossRef PubMed;
(c) P. S. Auti, G. George and A. T. Paul, RSC Adv., 2020, 10, 41353 RSC;
(d) P. S. Singu, U. Chilakamarthi, N. S. Mahadik, B. Keerti, N. Valipenta, S. N. Mokale, N. Nagesh and R. M. Kumbhare, RSC Med. Chem., 2021, 12, 416 RSC.
- N. Kerru, L. Gummidi, S. Maddila, K. K. Gangu and S. B. Jonnalagadda, Molecules, 2020, 25, 1909 CrossRef CAS.
- E. F. Ismail, I. A. I. Ali, W. Fathalla, A. A. Alsheikh and E. S. E. Tamney, Arkivoc, 2017, 104 Search PubMed.
- A. A. El-Barbary, A. A. El-Shehawy and N. I. Abdo, Phosphorus, Sulfur Silicon Relat. Elem., 2014, 183, 400 CrossRef.
- I. M. El-Deeb, S. M. Bayoumi, M. A. El-Sherbeny and A. A.-M. Abdel-Aziz, Eur. J. Med. Chem., 2010, 45, 2516 CrossRef CAS PubMed.
-
(a) S. I. Mirallai, M. J. Manos and P. A. Koutentis, J. Org. Chem., 2013, 78, 9906 CrossRef CAS PubMed;
(b) E. Enciso, J. Sarmiento-Sanchez, H. S. Lopez-Moreno, A. Ochoa-Teran, U. Osuna-Martinez and E. Beltran-Lopez, Mol. Diversity, 2016, 20, 821 CrossRef CAS PubMed;
(c) I. A. Rivero, K. Espinoza and R. Somanathan, Molecules, 2004, 9, 609 CrossRef CAS PubMed.
- D. Kang, H. Zhang, Z. Zhou, B. Huang, L. Naesens, P. Zhan and X. Liu, Bioorg. Med. Chem. Lett., 2016, 26, 5182 CrossRef CAS PubMed.
- A. H. Abdelmonsef and A. M. Mosallam, J. Heterocycl. Chem., 2020, 1, 1–18 Search PubMed.
- A. N. Al-Romaizan, N. S. Ahmed and S. M. Elfeky, Journal of Chemistry, 2019, 1, 1–12 Search PubMed.
- M. Redondo, J. G. Zarruk, P. Ceballos, D. I. Perez, C. Perez, A. Perez-Castillo, M. A. Moro, J. Brea, C. Val, M. I. Cadavid, M. I. Loza, N. E. Campillo, A. Martinez and C. Gil, Eur. J. Med. Chem., 2012, 47, 175 CrossRef CAS PubMed.
- A. Nakagawa, S. Uno, M. Makishima, H. Miyachi and Y. Hashimoto, Bioorg. Med. Chem., 2008, 16, 7046 CrossRef CAS PubMed.
- T. P. Tran, E. L. Ellsworth, M. A. Stier, J. M. Domagala, H. D. H. Showalter, S. J. Gracheck, M. A. Shapiro, T. E. Joannides and R. Singh, Bioorg. Med. Chem. Lett., 2004, 14, 4405 CrossRef CAS.
- H. Kakuta, A. Tanatani, K. Nagasawa and Y. Hashimoto, Chem. Pharm. Bull., 2003, 51, 1273 CrossRef CAS PubMed.
- S. H. Watterson, G. V. D. Lucca, Q. Shi, C. M. Langevine, Q. Liu, D. G. Batt, M. B. Bertrand, H. Gong, J. Dai, S. Yip, P. Li, D. Sun, D.-R. Wu, C. Wang, Y. Zhang, S. C. Traeger, M. A. Pattoli, S. Skala, L. Cheng, M. T. Obermeier, R. Vickery, L. N. Discenza, C. J. Darienzo, Y. Zhang, E. Heimrich, K. M. Gillooly, T. L. Taylor, C. Pulicicchio, K. W. Mcintyre, M. A. Galella, A. J. Tebben, J. K. Muckelbauer, C. Y. Chang, R. Rampulla, A. Mathur, L. Salter-Cid, J. C. Barrish, P. H. Carter, A. Fura, J. R. Burke and J. A. Tino, J. Med. Chem., 2016, 59, 9173 CrossRef CAS PubMed.
-
(a) M. Demeunynck and I. Baussanne, Curr. Med. Chem., 2013, 20, 794 CAS;
(b) M. Asif, Int. J. Med. Biochem., 2014, 395637 Search PubMed.
- N. F. Lazareva and V. F. Sidorkin, Efficient Methods for Preparing Silicon Compounds, 2016, p. 295 Search PubMed.
- P. Rajput and A. Sharma, Journal of Pharmacology and Medicinal Chemistry, 2018, 2, 22 Search PubMed.
-
(a) P. S. Singu, S. Kanugala, S. A. Dhawale, C. G. Kumar and R. M. Kumbhare, ChemistrySelect, 2020, 5, 117 CrossRef CAS;
(b) K. Chavva, S. Pillalamarri, V. Banda, S. Gautham, J. Gaddamedi, P. Yedla, C. G. Kumar and N. Banda, Bioorg. Med. Chem. Lett., 2013, 23, 5893 CrossRef CAS PubMed;
(c) G. J. Dev, Y. Poornachandra, K. R. Reddy, R. N. Kumar, N. Ravikumar, D. K. Swaroop, P. Ranjitreddy, G. S. Kumar, J. B. Nanubolu, C. G. Kumar and B. Narsaiah, Eur. J. Med. Chem., 2017, 130, 223 CrossRef PubMed;
(d) N. R. Kumar, D. K. Swaroop, N. Punna, K. Sirisha, T. Ganapathi, C. G. Kumar and B. Narsaiah, ChemistrySelect, 2018, 3, 7813 CrossRef CAS.
- J. Boonen, A. Bronselaer, J. Nielandt, L. Veryser, G. D. Tre and B. D. Spiegeleer, J. Ethnopharmacol., 2012, 142, 563 CrossRef CAS PubMed.
- A. Harrington and Y. Tal-Gan, Future Med. Chem., 2019, 11, 2759 CrossRef CAS PubMed.
-
(a) E. Spaczynska, A. Mrozek-Wilczkiewicz, K. Malarz, J. Kos, T. Gonec, M. Oravec, R. Gawecki, A. Bak, J. Dohanosova, I. Kapustikova, T. Liptaj, J. Jampilek and R. Musiol, Sci. Rep., 2019, 9, 6387 CrossRef PubMed;
(b) N. S. Thirukovela, S. K. Nukala, N. Sirassu, R. Manchal, P. Gundepaka and S. Paidakula, ChemistrySelect, 2020, 5, 12317 CrossRef CAS;
(c) Z.-H. Zhang, H.-M. Wu, S.-N. Deng, X.-Y. Cai, Y. Yao, M. C. Mwenda, J.-Y. Wang, D. Cai and Y. Chen, Journal of Chemistry, 2018, 1, 1–8 CrossRef.
-
(a) X. Zhou, X. Xie and G. Liu, Mol. Diversity, 2013, 17, 197 CrossRef CAS PubMed;
(b) J. Zhou, M. Ji, H. Yao, R. Cao, H. Zhao, X. Wang, X. Chen and B. Xu, Org. Biomol. Chem., 2018, 1, 1–17 Search PubMed.
-
(a) M. C. Willis, R. H. Snell, A. J. Fletcher and R. L. Woodward, Org. Lett., 2006, 8, 5089 CrossRef CAS PubMed;
(b) C. Larksarp and H. Alper, J. Org. Chem., 2000, 65, 2773 CrossRef CAS PubMed;
(c) X. Wu and Z. Yu, Tetrahedron Lett., 2010, 51, 1500 CrossRef CAS;
(d) H. Chen, P. Li, R. Qin, H. Yan, G. Li and H. Huang, ACS Omega, 2020, 5, 9614 CrossRef CAS PubMed;
(e) G. Shi, X. He, Y. Shang, C. Yang and L. Xiang, Chin. J. Chem., 2017, 1 Search PubMed;
(f) D.-Q. Shi, G.-L. Dou, Z.-Y. Li, S.-N. Ni, X.-Y. Li, X.-S. Wang, H. Wu and S.-J. Ji, Tetrahedron, 2007, 63, 9764 CrossRef CAS.
-
(a) H.-X. Wang, T.-Q. Wei, P. Xu, S.-Y. Wang and S.-J. Ji, J. Org. Chem., 2018, 83, 13491 CrossRef CAS PubMed;
(b) G. C. Senadi, W.-P. Hu, T.-Y. Lu, A. M. Garkhedkar, J. K. Vandavasi and J.-J. Wang, Org. Lett., 2015, 17, 1521 CrossRef CAS PubMed;
(c) Y. Zi, Z.-J. Cai, S.-Y. Wang and S.-J. Ji, Org. Lett., 2014, 16, 3094 CrossRef CAS PubMed.
-
(a) A. Nagaraju, B. J. Ramulu, G. Shukla, A. Srivastav, G. K. Verma, K. Raghuvanshi and M. S. Singh, Green Chem., 2015, 17, 950–958 RSC;
(b) Y.-W. Sun and L.-Z. Wang, New J. Chem., 2018, 42, 20032–20040 RSC;
(c) S. Muthusaravanan, B. D. Bala and S. Perumal, Tetrahedron Lett., 2013, 54, 5302–5306 CrossRef CAS;
(d) S. Su, J. Li, M. Sun, H. Zhao, Y. Chen and J. Li, Chem. Commun., 2018, 54, 9611–9614 RSC;
(e) B. Jiang, G. Zhang, N. Ma, F. Shi, S.-J. Tu, P. Kaur and G. Li, Org. Biomol. Chem., 2011, 9, 3834–3838 RSC.
- M. S. Searle, A. J. Maynard and H. E. L. Williams, Org. Biomol. Chem., 2003, 1, 60 RSC.
- M. Balakrishna, M. S. L. Karuna, R. B. N. Prasad, E. V. Krisha, S. Misra, C. G. Kumar and S. S. Kaki, SN Appl. Sci., 2020, 2, 1229 CrossRef CAS.
- BIOVIA Discovery studio visualizer, San Diego, Dassault Systemes, 2021 Search PubMed.
- G. M. Morris, D. S. Goodsell, M. E. Pique, W. L. Lindstrom, R. Huey, S. Forli, W. E. Hart, S. Halliday, R. Belew and A. J. Olson, Automated Docking, 2014 Search PubMed.
|
This journal is © The Royal Society of Chemistry 2022 |
Click here to see how this site uses Cookies. View our privacy policy here.