DOI:
10.1039/D2RA01631A
(Paper)
RSC Adv., 2022,
12, 15694-15704
Simple simultaneous determination of moxifloxacin and metronidazole in complex biological matrices†
Received
13th March 2022
, Accepted 17th May 2022
First published on 23rd May 2022
Abstract
A simple, sensitive and rapid RP-HPLC method is presented, for the first time, for the simultaneous determination of moxifloxacin hydrochloride and metronidazole in different biological fluids including saliva and plasma without any matrix interference. The separation was performed using ACN and phosphate buffer (30
:
70% v/v) as the mobile phase on a Zorbax Eclipse Plus-C18 column attached to a guard column. The method was validated according to the FDA guidelines for bioanalytical method validation and was successfully applied for simultaneous determination of the studied drugs in saliva and plasma samples. The good precision and selectivity of the developed method allow it to be used for routine therapeutic drug monitoring of such drugs and it presents a simple and sensitive analytical tool for performing versatile pharmacokinetics and bioavailability studies. A DAD detector is valuable to determine each drug at its maximum wavelength to ensure high sensitivity. Determination of such a combination in saliva introduces a quick and non-invasive alternative to blood analysis.
1. Introduction
Moxifloxacin hydrochloride (MX) is a synthetic fluroquinolone antibiotic with bactericidal effects (Fig. 1a). It binds to DNA gyrase (a bacterial enzyme) and inhibits DNA replication. It is active against Gram-positive bacteria such as Micrococcus luteus and Staphylococcus aureus, in addition to aerobic Gram-negative bacteria such as Actinobacter lwoffli and H. influenza. It is available as tablets, ophthalmic solutions and infusion bags for treatment of skin infections and respiratory infections such as pneumonia and bronchitis.1
 |
| Fig. 1 Chemical structure of (a) moxifloxacin hydrochloride and (b) metronidazole. | |
Metronidazole (MT) is a nitroimidazole drug as shown in Fig. 1b. It inhibits the synthesis of nucleic acid as a result of nitroso radicals' formation. It is active against anaerobes and effective for treatment of amebiasis, giardiasis, trichomoniasis and sexually transmitted disease.2
MX has a reduced activity against anaerobic pathogens. Therefore, a combination of MX with an antimicrobial agent active against anaerobes, such as MT, is of extreme importance for the treatment of mixed aerobic/anaerobic infections. The combination therapy of MX and MT is effective for patients having intra-abdominal abscesses.3
The therapeutic effect of the drug is related to its concentration thus monitoring the drug concentration in biological fluids is very essential for therapeutic dose monitoring and pharmacokinetic studies. The method proposed in this work is intended for the determination of the investigated drugs in both saliva and plasma. Analysis of saliva has recently received considerable attention over the last decade for drug testing. It can provide a quick and non-invasive alternative to blood analysis and also can be an easier alternative to urine due to the lower complexity of its matrix.4 This facilitates sample preparation and permits the development of non-invasive simple and sensitive analytical methods for the accurate therapeutic drug monitoring of drugs that are well distributed in saliva.
It is well-reported that MX and MT are absorbed rapidly with high bioavailability without being affected by food or infection.5,6 MX shows a good distribution between saliva and lung. Its maximum concentration (Cmax) in saliva is approximately double its Cmax in plasma which imparts MX its potential activity against oropharynx pathogens. After single dose of MX, Cmax was 3.2 μg mL−1.5 On the other hand, Cmax for MX in human saliva is approximately double its Cmax in plasma. While for MT, the Cmax in human saliva is approximately equal to its Cmax in plasma.7 After co-administration of single 500 mg and 250 mg MT doses, the Cmax is 11.5 μg mL−1 and 6.2 μg mL−1, respectively.8 Moreover, MX is well absorbed in rat plasma reaching maximum concentration at 0.75 ± 0.14 h with Cmax value of 4.53 ± 0.79 μg mL−1 after administration of 40 mg kg−1 of MX.9
Thorough literature review showed that this combination has been determined by very few analytical methods such as, spectrophotometry with chemometric analysis10 and simultaneous equations method.11 However, to the best of our knowledge, there are still no reports on the pharmacokinetics parameters of the binary combination of MT and MX. For this reason, the purpose of this work is to develop, for the first time, a simple, fast and sensitive RP-HPLC method with dual diode array and fluorimetric detection for the simultaneous determination of MX and MT in presence of salicylic acid (SAL) as internal standard (IS) in complex biological fluids that can be applied for the fast therapeutic drug monitoring of such combination.
2. Experimental
2.1. Instrumentation
HPLC Agilent 1200 series formed of quaternary pump, auto-injector, vacuum degasser; Diode Array Detector G1315 C/D, multiple wavelength detector G1365 C/D and FLD detectors is used. The used software was agilent ChemStation (Agilent Technologies, Santa Clara, USA). The separation was performed using agilent Zorbax Eclipse plus C18 analytical column with the following dimensions 4.6 × 250 mm, 5 Micron. Zorbax Eclipse Plus C8 guard column was used to protect the analytical one (4.6 × 12.5 mm, 5 Micron particle size). pH meter JENWAY (model 3505) was used for adjustment of pH of the solutions.
2.2. Materials and reagents
Reference moxifloxacin hydrochloride (99.58%), metronidazole (99.21%) and salicylic acid (99.08%) were purchased from Orchidia Pharmaceutical Industries, Al-Obour City, Egypt and Amriya Pharmaceuticals, Alexandria, Egypt, respectively. HPLC grade acetonitrile was obtained from Macron Fine Chemicals, USA while, sodium dihydrogen phosphate monohydrate, ortho-phosphoric acid 85% and sodium hydroxide were obtained from El-Nasr Chemical Industry Company, Egypt. Ethyl ether anhydrous was obtained from TEDIA Company.
2.3. Chromatographic conditions
The separation was achieved depending on isocratic system at flow rate 1 mL min−1 using acetonitrile (ACN) and phosphate buffer pH 3 (sodium dihydrogen phosphate monohydrate adjusted with ortho-phosphoric acid) in ratio 30
:
70% v/v as mobile phase modified by 0.1% triethylamine. This mobile phase was filtered using 0.45 μm membrane filter. The analysis was performed with 10 μL injection volume at room temperature 25 °C. DAD detector is available to determine each drug at its maximum wavelength to ensure high sensitivity. Thus, determination of MX was performed using dual wavelength system where MX and MT were determined at 300 nm and at 320 nm, respectively. Also, DAD provided peak purity plots to ensure drug purity and absence of any interference. It was observed that MX and SAL (IS) produced response at FLD that was set at λex = 300 nm and λem = 460 nm.
2.4. Preparation of solutions and construction of calibration graphs
2.4.1. Preparation of standard solutions. Stock solutions (1000 μg mL−1) of MX, MT and SAL (IS) were prepared in HPLC grade ACN. Working solutions (500 μg mL−1) were separately prepared using mobile phase as solvent. For preparation of calibrators and quality control samples, 20 μg mL−1 working solution of MX, MT and SAL (IS) were prepared by dilution of the stock solution in ethyl ether.
2.4.2. Analysis of synthetic mixtures. Dilution of working solutions were performed in mobile phase at different ratios to obtain synthetic mixtures with different concentrations within the linearity ranges. These mixtures were valuable to assess reproducibility and repeatability of the proposed method.
2.4.3. Analysis of biological fluids.
2.4.3.1. Analysis of saliva samples. In centrifuge tubes, samples were prepared by spiking 500 μL of human saliva with different concentrations of MX, MT and 10 μg mL−1 SAL. Then 2 mL ethyl ether was added to saliva. All tubes were vortexed for 5 min then centrifuged 10 min at 2500 rpm. Using Pasteur pipettes, the upper layer of the organic solvent was transferred in small tubes that were subjected to evaporation by vacuum till dryness. Reconstitution of the formed residues was made with 500 μL of mobile phase directly before injection. Quality control samples and standard solutions were prepared and analyzed on each day. Peak area of investigated drugs to peak area of SAL (IS) was plotted against concentration of MX and MT to obtain calibration graph of each drug.Low, medium and high-quality control samples (LQC, MQC and HQC) were prepared as 2, 10 and 20 μg mL−1, respectively for both MX and MT by diode array detector and 2, 6 and 20 μg mL−1, respectively, for MX by using FLD detector.
2.4.3.2. Analysis of plasma samples. Spiking of 200 μL human and rat plasma of each drug was performed in the presence of IS to prepare different concentrations, followed by addition of 2 mL ethyl ether to extract the investigated drugs. Then the procedure mentioned above under “Analysis of spiked saliva” was followed to construct the calibration graph.LQC, MQC and HQC were prepared as 4, 10 and 20 μg mL−1, respectively, for MX in rat plasma and 2, 10 and 20 μg mL−1, respectively, in human plasma. Also, the prepared concentrations of MT were 2, 10 and 20 μg mL−1 for LQC, MQC and HQC, respectively, in rat plasma and 1, 5 and 10 μg mL−1 for LQC, MQC and HQC, respectively, in human plasma. For MX at FLD, the concentrations for LQC, MQC and HQC were 4, 6 and 10 μg mL−1 and 2, 6 and 20 μg mL−1 in rat and human plasma, respectively.
All animal experiments were conducted according to a protocol approved by Institutional Animal Care and Use Committee (IACUC), Alexandria University and in compliance with the Guide for Care and Use of Laboratory Animals of the Institute for Laboratory Animal Research of the National Academy of Sciences, USA. It was performed on twelve male Wistar rats weighing 290–340 g. The rats were kept at ambient temperature and humidity-controlled room, in a 12 hour light/dark cycle. The rats received oral dosage combining both MT (7.5 mg kg−1) and MX (9.2 mg kg−1) prepared in saline. Then they were anesthetized and blood samples were collected via retro-orbital bleeding in polypropylene tubes with anti-coagulant EDTA solution at specified time (1 h). Preparation of plasma was performed after centrifugation for 10 min at 2500 rpm then it was kept at −20 °C to be thawed directly before preparation. SAL (10 μg mL−1) was added to 200 μL plasma. The samples were prepared and analyzed as the same procedure mentioned above.
2.5. Stability studies
Freeze and thaw stability for MX and MT was performed by applying three cycles of freeze and thaw. LQC and HQC samples were subjected to freezing (−20 °C) for 1 h then thawed at room temperature (n = 3). This cycle was repeated three times. The samples were measured after the third one. All obtained results were compared with the results of freshly prepared samples. While in short-term stability, LQC and HQC samples were left for 8 h at ambient temperature (25 °C). For post preparative stability, the prepared sample of LQC and HQC were stored for one day at 4 °C before analysis. The samples equivalent to LQC and HQC were prepared and stored for ten days at −20 °C for study of long-term stability. Finally, the stock solutions stability was determined at 25 °C at different time intervals. Moreover, the stocks were measured after freezing within the whole study period.
3. Results and discussion
3.1. Method development and optimization
In order to obtain the optimum chromatographic conditions for the sensitive and accurate analysis of the studied combination, we studied the factors that affect the separation of the investigated drugs with significant effect on system suitability parameters, such as, packing material, pH of phosphate buffer, organic modifier ratio and detection wavelengths.
3.1.1. Stationary phase. Differernt columns with different packing materials and dimensions were tested. For example; Zorbax Eclipse plus C18 analytical column (4.6 × 250 mm), Zorbax SB C8 analytical column (4.6 × 250 mm) and Zorbax Eclipse plus C18 analytical column (4.6 × 150 mm). Zorbax Eclipse plus C18 (4.6 × 250 mm, 5 micron) produced the best separation with short analysis time. It provided the most symmetric peaks in addition to good resolution between peaks. These columns were tested using mobile phase of phosphate buffer and acetonitrile in variable ratios and pH values from 3 to 6.
3.1.2. Modifier ratio. Different percentages of acetonitrile (ACN) from 10% to 30% were examined. Increasing ACN% led to significant changes in retention time. It was obvious that ACN of 30% obtained the best peak shape with good resolution between the eluted peaks (Fig. S1a, ESI†). It produced accepted separation at short retention time.
3.1.3. Buffer pH. Different pH values (pH 3–6) of phosphate buffer were tested. It was obvious that high pH values produced distortion in peak shapes with significant changes in retention time accompanied with minor changes in the pH values higher than pH 4 with longer analysis time as shown in Fig. S1b.† While, pH 3 resulted in well resolved peaks with good shapes within a short analysis time. Therefore, pH 3 was selected for analysis.
3.1.4. Detection wavelength. DAD provided an advantage of analysis of each drug at its maximum wavelength which ensured high sensitivity and selectivity of assay. So, application of HPLC-Dual wavelength system was performed to measure MX at 300 nm and MT at 320 nm. Moreover, it provided peak purity plots to ensure purity of investigated drugs. Also, using fluorescence detector within complex biological matrixes ensures high sensitivity and selectivity of the method for analysis of MX at λex = 300 nm and λem = 460 nm.
3.2. System suitability parameters
The system suitability parameters are essential part for accurate chromatographic methods. They were valuable for assessment of efficiency of separation, peak symmetry, and resolution of peaks. As shown in Table S1 (ESI†), the peaks of investigated drugs were well separated with high efficiency of separation with short analysis time, where, MT and MX were eluted at retention time of 3.22 and 3.99 min, respectively (Fig. 2).
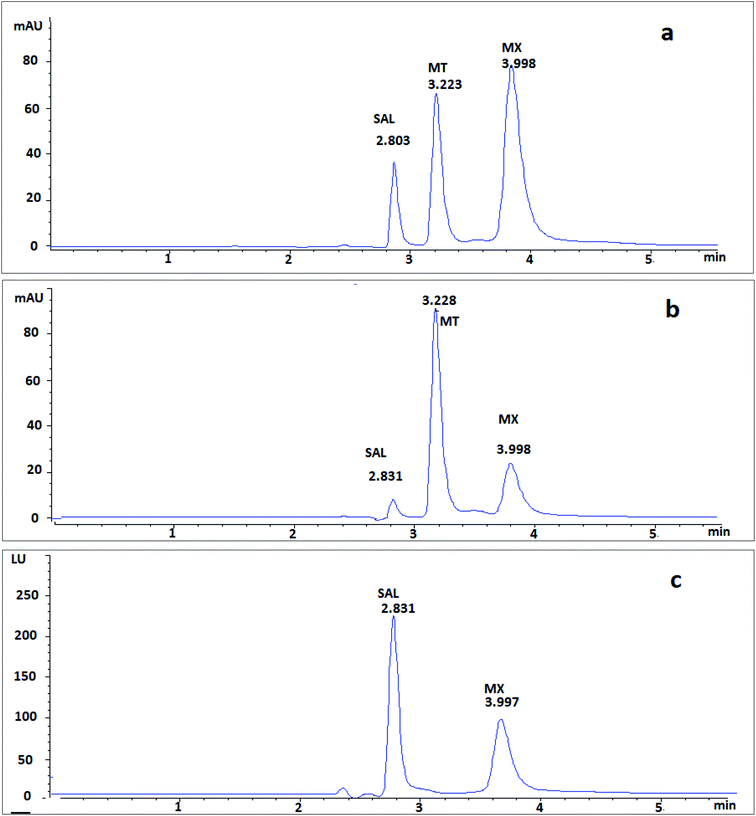 |
| Fig. 2 Typical HPLC chromatograms of a standard synthetic mixture containing 10 μg mL−1 SAL, 20 μg mL−1 MT and 20 μg mL−1 MX (a) using DAD at 300 nm, (b) using DAD at 320 nm and (c) using FLD at λex = 300 nm and λem = 460 nm. | |
3.3. Validation of the proposed method
Validation of the proposed method was performed depending on Bio-analytical Method Validation Guidance for Industry to determine MX and MT in biological fluids.12
3.3.2. Lower limit of quantitation (LLOQ). LLOQ were determined by analysis of five replicates of small concentrations of each analyte against blank of biological fluids. The lowest concentration of each analyte with high precision and accuracy were determined as LLOQ. Low LLOQ values were obtained by the proposed methods as demonstrated in Table S2† indicating the high sensitivity of the proposed methods.
3.3.3. Accuracy, precision and recoveries. The accuracy and precision were performed for the proposed method on the same day and on three different days to assess accuracy, intra-day precision and inter-day precision. Set of calibrators and quality control sample involving LLOQ, LQC, MQC and HQC have been analyzed with five replicates per each quality control level. The values obtained of %recovery, percentage error (%Er) and percentage relative standard deviation (%RSD) were lower than 15% for concentrations higher that LLOQ and 20% for LLOQ. These values showed high accuracy and precision of the proposed method for synthetic mixtures (Table S3, ESI†) and for quality control samples of different biological fluids (Table 2).
Table 2 Intra-day and inter-day precision and accuracy for simultaneous determination of MX and MT in different biological fluids using the proposed RP-HPLC method
Conc. (μg mL−1) |
Average% recovery ± SD |
%RSD |
%Er |
MX (DAD) |
MX (FLD) |
MT |
MX (DAD) |
MX (FLD) |
MT |
MX (DAD) |
MX (FLD) |
MT |
In human saliva |
Intra-day precision |
LLQC |
90.93 ± 13.01 |
90.62 ± 18.06 |
97.29 ± 11.09 |
14.31 |
19.92 |
11.40 |
−9.07 |
−9.38 |
−2.71 |
LQC |
96.11 ± 4.58 |
89.44 ± 6.93 |
97.73 ± 3.67 |
4.77 |
7.74 |
3.76 |
−3.90 |
−10.56 |
−2.27 |
MQC |
97.52 ± 6.10 |
97.95 ± 2.73 |
100.57 ± 0.77 |
6.26 |
2.79 |
0.77 |
−2.48 |
−2.05 |
0.57 |
HQC |
103.60 ± 4.89 |
99.53 ± 5.46 |
98.20 ± 1.43 |
4.72 |
5.49 |
1.46 |
3.60 |
−0.47 |
−1.80 |
Inter-day precision |
LLQC |
93.10 ± 10.77 |
114.26 ± 18.06 |
105.37 ± 0.69 |
11.47 |
15.80 |
0.66 |
−6.90 |
14.26 |
5.37 |
LQC |
102.69 ± 1.79 |
104.73 ± 13.72 |
103.30 ± 6.98 |
1.74 |
13.10 |
6.76 |
2.69 |
4.73 |
3.30 |
MQC |
101.49 ± 0.45 |
101.02 ± 1.30 |
98.73 ± 4.16 |
0.44 |
1.29 |
4.22 |
1.49 |
1.02 |
−1.27 |
HQC |
108.31 ± 12.86 |
95.47 ± 4.48 |
97.47 ± 1.82 |
11.87 |
4.69 |
1.85 |
8.31 |
−4.53 |
−2.53 |
![[thin space (1/6-em)]](https://www.rsc.org/images/entities/char_2009.gif) |
In human plasma |
Intra-day precision |
LLQC |
95.26 ± 1.89 |
108.55 ± 15.19 |
90.51 ± 5.52 |
1.99 |
13.99 |
6.10 |
−4.74 |
8.55 |
−9.49 |
LQC |
97.82 ± 2.84 |
97.68 ± 3.29 |
108.97 ± 1.79 |
2.90 |
3.37 |
1.64 |
−2.18 |
−2.32 |
8.97 |
MQC |
97.47 ± 1.20 |
100.49 ± 1.33 |
106.83 ± 4.42 |
1.23 |
1.32 |
4.14 |
−2.53 |
0.49 |
6.83 |
HQC |
101.04 ± 1.97 |
100.45 ± 0.45 |
101.90 ± 4.22 |
1.95 |
0.44 |
4.14 |
1.04 |
0.45 |
1.90 |
Inter-day precision |
LLQC |
104.73 ± 8.26 |
113.04 ± 13.04 |
91.86 ± 4.15 |
7.88 |
11.54 |
4.51 |
4.73 |
13.04 |
−8.14 |
LQC |
100.66 ± 3.28 |
97.68 ± 3.29 |
107.50 ± 10.08 |
3.26 |
3.37 |
9.38 |
0.66 |
−2.32 |
7.50 |
MQC |
102.33 ± 6.56 |
103.97 ± 5.59 |
106.48 ± 3.49 |
6.41 |
5.38 |
3.28 |
2.33 |
3.97 |
6.48 |
HQC |
99.78 ± 0.55 |
102.86 ± 3.95 |
99.33 ± 2.97 |
0.55 |
3.84 |
2.99 |
−0.22 |
2.86 |
−0.67 |
![[thin space (1/6-em)]](https://www.rsc.org/images/entities/char_2009.gif) |
In rat plasma |
Intra-day precision |
LLQC |
94.12 ± 5.69 |
105.88 ± 8.95 |
103.35 ± 3.91 |
6.05 |
8.45 |
3.78 |
−5.88 |
5.88 |
3.35 |
LQC |
102.36 ± 4.43 |
95.10 ± 6.67 |
90.52 ± 2.35 |
4.33 |
7.02 |
2.59 |
2.36 |
−4.90 |
−9.48 |
MQC |
98.16 ± 5.01 |
100.03 ± 2.93 |
98.01 ± 1.27 |
5.01 |
2.92 |
1.29 |
−1.84 |
0.03 |
−1.99 |
HQC |
99.88 ± 1.35 |
101.47 ± 3.09 |
100.35 ± 1.11 |
1.35 |
3.05 |
1.11 |
−0.12 |
1.47 |
0.35 |
Inter-day precision |
LLQC |
91.13 ± 3.36 |
103.43 ± 11.89 |
95.97 ± 2.69 |
3.69 |
11.49 |
2.80 |
−8.87 |
3.43 |
−4.03 |
LQC |
96.81 ± 5.40 |
103.92 ± 4.90 |
97.31 ± 5.01 |
5.58 |
4.71 |
5.15 |
−3.19 |
3.92 |
−2.69 |
MQC |
101.29 ± 0.82 |
105.72 ± 10.20 |
98.21 ± 1.62 |
0.81 |
9.65 |
1.65 |
1.29 |
5.72 |
−1.79 |
HQC |
100.07 ± 1.62 |
97.75 ± 8.62 |
101.29 ± 1.17 |
1.62 |
8.82 |
1.15 |
0.07 |
−2.25 |
1.29 |
3.3.4. Robustness. Robustness is a measure of the capacity of the developed method to remain unaffected by small but deliberate variations in used conditions. It provides an indication of the reliability of the developed method during normal usage. Applying slight variations in some chromatographic conditions provided no significant effect on resolution, peak shape or responses of peak area, which ensured by accepted values of %recovery and small values of %RSD (Table S4, ESI†). The involved parameters were buffer pH, buffer concentration and detection wavelength.
3.3.5. Stability. The stability studies were carried out on the stock solutions for each drug where the drugs showed high stability. Also, the studies were performed on QC samples with low and high concentration including long and short terms stability, freeze and thaw and post preparative stability. The obtained results were compared with freshly prepared solutions responses. As shown in Table S5 (ESI†) for spiked saliva and Table S6 (ESI†) for spiked plasma, %Er and %RSD values were within the accepted limits which proved the stability of drugs by application of different conditions.
3.3.6. Selectivity and matrix effect. During method development, it is essential to ensure that the determination is free of any interfering material of matrix component. The analysis of blank matrix (saliva, human plasma and rat plasma) from different sources showed that the biological matrix did not have any interference with the investigated drugs. Also, the purity of investigated drugs is ensured by peak purity plots of MX and MT as demonstrated in Fig. S2.† Moreover, detection of MX using FLD ensured the selectivity of the proposed method.
3.3.7. Sensitivity. The proposed method is highly sensitive method showing small values of LLOQ in different biological fluids (Table S2†). Moreover, detection of MX using FLD ensured the sensitivity of the proposed method.
3.4. Application to biological fluids
3.4.2. Plasma analysis (in vivo analysis). The proposed method was successfully used for sensitive determination of the selected drugs in rats. Fig. 4 demonstrated well separated peaks of MX and MT with high selectivity and no interference with the plasma matrix.
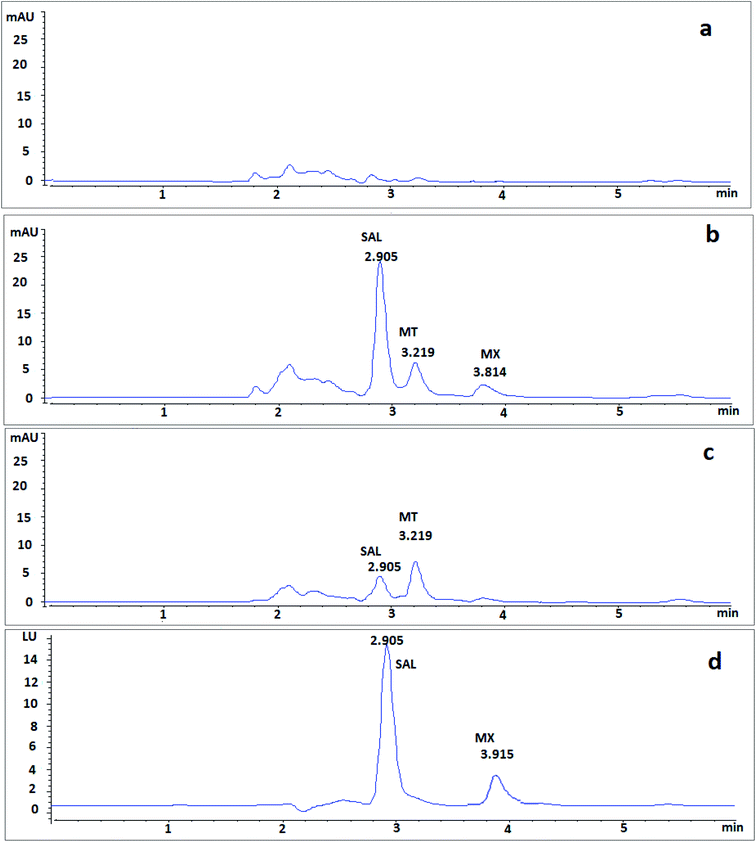 |
| Fig. 4 Typical HPLC chromatogram of (a) blank rat plasma using DAD at 300 nm, (b) 4 μg mL−1 MX and 4 μg mL−1 MT spiked in rat plasma in presence of 10 μg mL−1 SAL (IS) using DAD at 300 nm, (c) 4 μg mL−1 MX and 4 μg mL−1 MT spiked in rat plasma in presence of 10 μg mL−1 SAL (IS) using DAD at 320 nm and (d) 4 μg mL−1 MX and 4 μg mL−1 MT spiked in rat plasma in presence of 10 μg mL−1 SAL (IS) using FLD at λex = 300 nm and λem = 460 nm. | |
After 1 h of co-administration of single dose of MX (9.2 mg kg−1) and MT (7.5 mg kg−1), analysis of the plasma samples showed well-separated peaks of MX and MT (Fig. 5), where the recovered concentrations of MX and MT were 4.19 ± 0.45 μg mL−1 and 4.21 ± 0.64 μg mL−1, respectively.
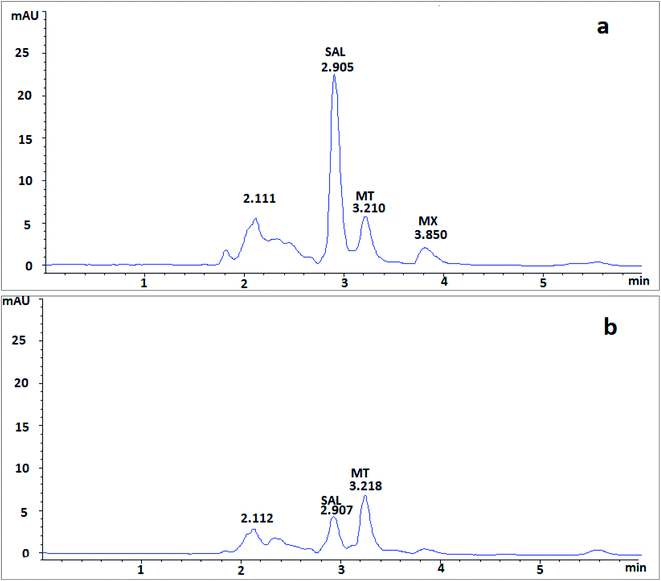 |
| Fig. 5 Typical chromatograms of single dose of co-administrated MX and MT in rat plasma after 1 h spiked with IS (a) using DAD at 300 nm and (b) using DAD at 320 nm. | |
Moreover, in order to assess the applicability of the proposed method in human plasma, the investigated drugs added to human plasma were analyzed using the proposed method. Results showed very similar elution and retention characteristics to those of rat plasma (Fig. 6).
 |
| Fig. 6 Typical HPLC chromatograms of (a) blank human plasma using DAD at 300 nm, (b) 20 μg mL−1 MX and 20 μg mL−1 MT spiked in human plasma in presence of 10 μg mL−1 SAL (IS) using DAD at 300 nm, (c) 20 μg mL−1 MX and 20 μg mL−1 MT spiked in human plasma in presence of 10 μg mL−1 SAL (IS) using DAD at 320 nm and (d) 20 μg mL−1 MX and 20 μg mL−1 MT spiked in human plasma in presence of 10 μg mL−1 SAL (IS) using FLD at λex = 300 nm and λem = 460 nm. | |
3.5. Comparison with reported methods
To the best of our knowledge, only two spectrophotometric studies were previously reported for the simultaneous analysis of MT and MX.10,11 Table 4 demonstrates a detailed comparison of our proposed method and the reported ones. Chromatographic analysis offers many advantages for the analysis of various compounds.13–27 Thus, our proposed RP-HPLC method shows many advantages over the reported ones. It shows higher selectivity and sensitivity. Also, the proposed method provides peak purity plots to ensure the purity of each drug that allows the determination of the studied drugs in complex biological matrixes. Moreover, using the fluorescence detector enhances the selectivity and sensitivity of the method. The present work offers several applications that not available in other reported methods. It is applied for the simultaneous determination of MT and MX in various biological fluids including saliva, and plasma that permits the fast therapeutic drug monitoring of such combination and studying the pharmacokinetics parameters and bioavailability of both co-administered drugs.
Table 4 Analytical comparison between the proposed RP-HPLC method and reported spectrophotometric methods for simultaneous determination of MX and MT
Parameter |
Proposed RP-HPLC method |
Reported method10 |
Reported method11 |
MX (DAD) |
MX (FLD) |
MT |
MX |
MT |
MX |
MT |
λnm (nm) |
300 |
λex = 300 |
320 |
200–500 |
295.4 |
231.8 |
λem = 460 |
Linearity range (μg mL−1) |
0.2–100 |
0.1–100 |
0.2–80 |
5–25 |
7–35 |
1–10 |
3–150 |
LOQ (μg mL−1) |
0.18 |
0.1 |
0.19 |
5.00 |
7.00 |
1.00 |
3.00 |
Application |
It was applied in variable biological fluids |
It was applied only in pharmaceutical formulations |
It was applied only in synthetic mixture |
It is the most sensitive method |
4. Conclusion
In this work, a selective and sensitive HPLC method has been developed and validated for the simultaneous determination of MX and MT in different biological fluids using dual DAD and FLD. MX and MT were determined by the DAD at 300 nm and at 320 nm, respectively and MX was determined using FLD at λex = 300 nm and λem = 460 nm. The proposed method was successfully applied for the simultaneous determination of MT and MX in saliva and plasma samples. Thus, this method is valuable for clinical studies and therapeutic monitoring of both drugs, simultaneously or as single components. Also, it introduces a facile analytical method for the fast therapeutic drug monitoring of both co-administered drugs.
Ethical statement
All animal experiments were conducted according to a protocol approved by our institutional animal care and use committee and in compliance with the Guide for Care and use of Laboratory Animals of the Institute for Laboratory Animal Research of the National Academy of Sciences, USA.
Conflicts of interest
There are no conflicts of interest to declare.
References
- National Center for Biotechnology Information, PubChem Compound Summary for CID 152946 MRJ, 2021 from https://pubchem.ncbi.nlm.nih.gov/compound/Moxifloxacin Search PubMed.
- National Center for Biotechnology Information, PubChem Compound Summary for CID 4173 MRJ, 2021 from https://pubchem.ncbi.nlm.nih.gov/compound/Metronidazole Search PubMed.
- G. Weiss, P. Reimnitz, B. Hampel, E. Muehlhofer and H. Lippert, Moxifloxacin for the treatment of patients with complicated intra-abdominal infections (the AIDA Study), J. Chemother., 2009, 21(2), 170–180 CrossRef CAS PubMed.
- S. H. van den Elsen, L. M. Oostenbrink, S. K. Heysell, D. Hira, D. J. Touw and O. W. Akkerman, et al., Systematic review of salivary versus blood concentrations of antituberculosis drugs and their potential for salivary therapeutic drug monitoring, Ther. Drug Monit., 2018, 40(1), 17 CrossRef CAS PubMed.
- Nord C. E., Pharmacology of moxifloxacin—absorption, distribution, metabolism and excretion, First International Moxifloxacin Symposium, Springer, 2000 Search PubMed.
- E. R. Pähkla, T. Koppel, M. Saag and R. Pähkla, Metronidazole concentrations in plasma, saliva and periodontal pockets in patients with periodontitis, J. Clin. Periodontol., 2005, 32(2), 163–166 CrossRef PubMed.
- S. Suryawati and B. Santoso, Pharmacokinetics of metronidazole in saliva, Int. J. Clin. Pharmacol., Ther. Toxicol., 1991, 29(12), 474–478 Search PubMed.
- E. D. Ralph, J. T. Clarke, R. D. Libke, R. P. Luthy and W. M. Kirby, Pharmacokinetics of metronidazole as determined by bioassay, Antimicrob. Agents Chemother., 1974, 6(6), 691–696 CrossRef CAS PubMed.
- C. M. Culley, M. K. Lacy, N. Klutman and B. Edwards, Moxifloxacin: clinical efficacy and safety, Am. J. Health-Syst. Pharm., 2001, 58(5), 379–388 CrossRef CAS PubMed.
- G. P. Ertokuş and Ü. M. ÇELİK, Chemometric Analysis of Moxifloxacin and Metronidazole in Binary Drug Combinations With Spectrophotometric Method, Eastern Anatolian Journal of Science, 2017, 3(2), 30–37 Search PubMed.
- P. Patel, B. Suhagia and M. Patel, Simultaneous spectrophotometric determination of Moxifloxacin and Metronidazole in synthetic mixture by simultaneous equations method, Indian Drugs, 2005, 2(3), 155–157 Search PubMed.
- U. FDA, Bioanalytical method validation guidance for industry. US Department of Health and Human Services Food and Drug Administration Center for Drug Evaluation and Research and Center for Veterinary Medicine, 2018 Search PubMed.
- A. F. El-Yazbi, M. G. Guirguis, M. M. Bedair and T. S. Belal, Simultaneous Quantitation of Paracetamol and Lornoxicam in the Presence of Five Related Substances and Toxic Impurities by a Selective HPLC–DAD Method, J. AOAC Int., 2022, 1–7 Search PubMed.
- A. F. El-Yazbi, F. M. Aboukhalil, E. F. Khamis, R. M. Youssef and M. A. El-Sayed, Greenness assessment of a stability indicating simple inexpensive high-performance thin-layer chromatography–dual wavelength method for simultaneous determination of mometasone furoate and salicylic acid in complex matrix using analytical eco-scale, J. Planar Chromatogr.--Mod. TLC, 2021, 34, 455–466 CrossRef CAS.
- A. F. El-Yazbi, N. E. Elashkar, H. M. Ahmed, W. Talaat and K. M. Abdel-Hay, Cost-effective green chromatographic method for the simultaneous determination of four commonly used direct-acting antiviral drugs in plasma and various pharmaceutical formulations, Microchem. J., 2021, 168, 106512 CrossRef CAS.
- A. F. El-Yazbi, Green methods for the simultaneous analysis of pharmaceutical mixtures present in disparate concentration ranges: Application to antidiabetic mixtures, Microchem. J., 2021, 164, 106073 CrossRef CAS.
- A. F. El-Yazbi, F. M. Aboukhalil, E. F. Khamis, R. M. Youssef and M. A. El-Sayed, Simultaneous determination of Mometasone Furoate and salicylic acid in complex matrix using green analytical method, Microchem. J., 2021, 163, 105900 CrossRef CAS.
- A. F. El-Yazbi, Y. Khalifa, M. A. W. Elkhatib and A. F. El-Yazbi, Green Analytical Method for the Determination of Sofosbuvir, Ledipasvir, Ribavirin and Complex Silymarin Flavonoids Simultaneously in Biological Fluids, Microchem. J., 2021, 105964 CrossRef CAS.
- A. F. El-Yazbi, M. G. Guirguis, M. M. Bedair and T. S. Belal, Validated specific HPLC-DAD method for simultaneous estimation of paracetamol and chlorzoxazone
in the presence of five of their degradation products and toxic impurities, Drug Dev. Ind. Pharm., 2020, 46(11), 1853–1861 CrossRef CAS PubMed.
- E. I. El-Kimary, H. A. Khalil, T. S. Belal and A. F. El-Yazbi, Green stability-indicating capillary electrophoretic method for simultaneous determination of Lesinurad and Allopurinol in tablet dosage form: Degradation kinetics investigation, Microchem. J., 2020, 158, 105199 CrossRef CAS.
- A. F. El-Yazbi, N. E. Elashkar, K. M. Abdel-Hay, W. Talaat and H. M. Ahmed, An Eco-friendly HPTLC-dual wavelength spectrodensitometric method for simultaneous determination of sofosbuvir and ledipasvir in biological and pharmaceutical samples: Stability indicating application, Microchem. J., 2020, 154, 104584 CrossRef CAS.
- A. F. El-Yazbi, E. I. El-Kimary and R. M. Youssef, Hantzsch pre-column derivatization for simultaneous determination of alendronate sodium and its pharmacopoeial related impurity: Comparative study with synchronous fluorometry using fluorescamine, J. Food Drug Anal., 2019, 27, 208–220 CrossRef CAS PubMed.
- F. Ibrahim, A. F. El-Yazbi, M. M. Wagih and M. A. Barary, Chromatographic determination of zonisamide, topiramate and sulpiride in plasma by a fluorescent ‘turn-on’ chemosensor, Bioanalysis, 2017, 9(14), 1049–1064 CrossRef CAS PubMed.
- A. F. El-Yazbi, Comparative Validation of the Determination of Sofosbuvir in Pharmaceuticals by Several Inexpensive Ecofriendly Chromatographic, Electrophoretic, and Spectrophotometric Methods, J. AOAC Int., 2017, 100(4), 1000–1007 CrossRef CAS PubMed.
- A. F. El-Yazbi and A. El-Hawiet, Novel Chromatographic Methods for Simultaneous Quantification of Fish and Wheat Germ Oils Mixture in Pharmaceutical Dosage Forms, J. Chromatogr. Sci., 2017, 55, 497–507 CAS.
- F. Ibrahim, A. F. El-Yazbi, M. A. Barary and M. M. Wagih, Sensitive inexpensive HPLC determination of four antiepileptic drugs in human plasma: application to PK studies, Bioanalysis, 2016, 8(21), 2219–2234 CrossRef CAS PubMed.
- A. F. El-Yazbi and R. M. Youssef, Eco-friendly HPTLC method for assay of Eszopiclone in pharmaceutical preparation: Investigation of its water-induced degradation kinetics, Anal. Methods, 2015, 7, 7590–7595 RSC.
|
This journal is © The Royal Society of Chemistry 2022 |
Click here to see how this site uses Cookies. View our privacy policy here.