DOI:
10.1039/D2RA01578A
(Paper)
RSC Adv., 2022,
12, 10534-10539
Beshanzuamide A, an unprecedented prenylated indole alkaloid produced by Aspergillus sp. Y-2 from the critically endangered conifer Abies beshanzuensis†
Received
10th March 2022
, Accepted 30th March 2022
First published on 6th April 2022
Abstract
A structurally unprecedented prenylated indole alkaloid, beshanzuamide A (1), together with five known analogues (2–6) were isolated and identified from the endophytic fungus derived from the needles of the critically endangered conifer Abies beshanzuensis. The new structure was determined by extensive spectroscopic methods and quantum chemical calculations of NMR and electronic circular dichroism (ECD) data. Compound 1 features a unique N,O-spiroketal/δ-lactone motif connected to a pyranoindole-derived bicyclo[2.2.2]diazaoctane ring. A plausible biogenetic pathway for the assembly of 1 was proposed.
Introduction
Rare and endangered plants (REPs) have proven to be a better donor for innovative drugs than other plant resources.1,2 It is necessary to give priority to the protection and utilization of these endangered plants. In recent years, relevant research on the REPs endemic to China have been launched by our group.3 However, in many cases, it is not easy to investigate endangered species due to the difficulties in collecting the rare plant materials. Fortunately, a large number of endophytic fungi, bacteria and actinomycetes contained in each plant species may be able to synthesize related plant metabolites.4 Consequently, exploring the secondary metabolites of endophytes related to endangered plants could become an alternative strategy for the discovery of new drugs, which may also be conductive to the protection and sustainable use of these fragile plant resources.
The Chinese fir tree Abies beshanzuensis M. H. Wu (family Pinaceae) has been categorized as one of the twelve critically endangered plant species all over the world by the International Union for Conservation of Nature (IUCN).5,6 Seven of such trees were first discovered in 1963 but only three currently remain in the wild, distributed on the summit of Baishanzu Mountain in Zhejiang Province of China.5–7 This conifer species has been nationally protected as the ‘first-grade’ in China since 1999.8 In a phytochemical investigation on this critically endangered conifer, a few rare enone-containing Abies sesquiterpenoids with considerable inhibition on protein tyrosine phosphatase 1B (PTP1B) were obtained.9 Subsequent researches on the microbial resources of this fragile species have been carried out.10,11 A previous investigation on the endophytic fungus Aspergillus sp. Y-2 (GenBank accession no. MN103998), which was isolated from the fresh needles, led to the isolation of several aflatoxins with cytotoxic activities.10 In a further chemical investigation on the culture, a structurally unprecedented prenylated indole alkaloid, named beshanzuamide A (1), along with five known analogues (2–6) (Fig. 1) was isolated and identified. Herein, we report their isolation, structural elucidation, and bioactivity evaluations.
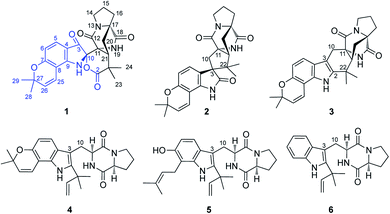 |
| Fig. 1 The structures of compounds 1–6. | |
Results and discussion
Beshanzuamide A (1) was isolated as an orange amorphous powder. It has the molecular formula of C26H27N3O6 (fifteen degrees of unsaturation), based on a protonated ion at m/z 478.1977 [M + H]+ (calcd 478.1973) in the positive HR-ESI-MS in conjunction with its 13C NMR data. The 1H NMR spectrum of 1 exhibited signals ascribable for four olefinic protons [δH 7.37 (1H, d, J = 8.4 Hz, H-4), 6.56 (1H, d, J = 10.0 Hz, H-25), 6.35 (1H, d, J = 8.4 Hz, H-5), 5.73 (1H, d, J = 10.0 Hz, H-26)] and four methyl singlets [δH 1.47 (3H, s, H3-29), 1.46 (3H, s, H3-28), 1.33 (3H, s, H3-23), 1.23 (3H, s, H3-24)] (Table 1). The 13C NMR spectrum of 1 in CD3OD displayed 26 well-resolved carbon resonances (Table 1), classified by HSQC experiment as four carbonyl carbons (δC 193.3, 178.4, 175.0 and 168.2), eight olefinic carbons (δC 164.0, 158.8, 129.9, 127.9, 116.3, 112.3, 111.9, and 106.2), and 14 saturated carbons. The latter consists of four methyls (δC 28.6, 28.5, 26.5 and 22.5), four methylenes (δC 45.3, 31.6, 30.0 and 25.4), one methine (δC 42.3), one quaternary carbon (δC 43.4), three heteroatom-bearing tertiary carbons (δC 79.4, 68.2 and 61.7), and the carbon resonating at δC 90.5 typical for a N,O-spiro-ketal group. The above spectroscopic data showed great similarity to those of notoamide O (Fig. S1†), a prenylated indole alkaloid featuring a unique hemiacetal/hemiaminal ether functionality isolated from a marine-derived Aspergillus sp.12 The most appreciable difference is, resonances for the hemiacetal group (δC 104.8/δH 6.19) in notoamide O was absent in compound 1; while instead, an additional lactone carbonyl group (δC 178.4, C-2) was observed. This lactone carbonyl was adjacent to C-22, which was readily evidenced from its HMBC correlations (Fig. 2) with the geminal methyl groups of H3-23 and H3-24. Meanwhile, the N,O-ketal carbon was undoubtedly assigned to C-10 based on the HMBC correlations of 1-NH with C-3/C-8/C-9, and of 19-NH with C-10/C-11/C-17. The remaining one more degree of unsaturation then required the formation of a δ-lactone ring between C-10 and C-2.
Table 1 1H and 13C NMR spectroscopic data (δ in ppm) of 1
No. |
Type |
In CD3OD |
In DMSO-d6 |
δCa |
δHb (mult., J in Hz) |
δCa |
δHc (mult., J in Hz) |
Measured at 125 MHz. Measured at 600 MHz. Measured at 400 MHz. |
1 |
NH |
|
|
|
7.61 (s) |
2 |
C |
178.4 |
|
176.2 |
|
3 |
C |
193.3 |
|
192.3 |
|
4 |
CH |
127.9 |
7.37 (d, 8.4) |
126.5 |
7.29 (d, 8.4) |
5 |
CH |
111.9 |
6.35 (d, 8.4) |
109.9 |
6.30 (d, 8.4) |
6 |
C |
164.0 |
|
161.8 |
|
7 |
C |
106.2 |
|
104.8 |
|
8 |
C |
158.8 |
|
157.7 |
|
9 |
C |
112.3 |
|
111.2 |
|
10 |
C |
90.5 |
|
89.5 |
|
11 |
C |
61.7 |
|
59.8 |
|
12 |
C |
168.2 |
|
165.7 |
|
13 |
N |
|
|
|
|
14 |
CH2 |
45.3 |
3.56 (m) |
43.8 |
3.43 (m) |
3.49 (m) |
3.40 (m) |
15 |
CH2 |
25.4 |
2.08 (m) |
24.1 |
2.02 (m) |
1.95 (m) |
1.84 (m) |
16 |
CH2 |
30.0 |
2.65 (m) |
28.5 |
2.47 (m) |
1.93 (m) |
1.82 (m) |
17 |
C |
68.2 |
|
66.1 |
|
18 |
C |
175.0 |
|
172.8 |
|
19 |
NH |
|
|
|
8.95 (s) |
20 |
CH2 |
31.6 |
2.15 (dd, 13.7, 10.5) |
2.07 (dd, 13.7, 10.4) |
2.00 (dd, 13.7, 5.2) |
2.07 (dd, 13.7, 5.2) |
21 |
CH |
42.3 |
3.75 (dd, 10.5, 5.2) |
40.1 |
3.55 (dd, 10.4, 5.2) |
22 |
C |
43.4 |
|
41.7 |
|
23 |
CH3 |
26.5 |
1.33 (s) |
25.9 |
1.21 (s) |
24 |
CH3 |
22.5 |
1.23 (s) |
21.6 |
1.13 (s) |
25 |
CH |
116.3 |
6.56 (d, 10.0) |
115.7 |
6.82 (d, 10.0) |
26 |
CH |
129.9 |
5.73 (d, 10.0) |
128.0 |
5.70 (d, 10.0) |
27 |
C |
79.4 |
|
77.8 |
|
28 |
CH3 |
28.5 |
1.46 (s) |
27.8 |
1.40 (s) |
29 |
CH3 |
28.6 |
1.47 (s) |
27.9 |
1.43 (s) |
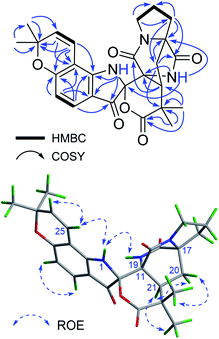 |
| Fig. 2 Key HMBC, 1H–1H COSY, and ROE correlations of 1. | |
In terms of determination of the relative spatial configuration for 1, interpretation of the ROESY correlations (Fig. 2) or proton–proton coupling constants (Table 1) could not provide us with enough information for this complex structure. Given the high rigidity of the bicyclo[2.2.2]diazaoctane ring (C-11–C-21), the two chiral canters C-11 and C-17 must be changed simultaneously. Hence there are four possible relative configurations for 1 [(10S*,11R*,17S*,21S*)-1, (10S*,11R*,17S*,21R*)-1, (10R*,11R*,17S*,21S*)-1, and (10R*,11R*,17S*,21R*)-1] (Fig. S2 in ESI†). The quantum calculations of 13C NMR chemical shifts of the above four possible isomers were performed using the GIAO (Gauge Independent Atomic Orbital) method, and the data processing followed the reported STS protocol.13 As shown in Table 2, compared with the experimental data of 1, (10S*,11R*,17S*,21S*)-1 exhibited the minimum values of mean absolute error (MAE) and root mean square (RMS), with a Prel probability of 99.12%. This unequivocally defined the relative configuration of 1 as (10S*,11R*,17S*,21S*). Consistent with this, the relative configuration assignment of (11R*,17S*,21S*) was congruent with the co-occurring prenylated indole alkaloids notoamide B (2) and stephacidine A (3).14
Table 2 DFT GIAO 13C NMR chemical shifts of the four possible isomers of 1 were calculated on ωB97X-D/6-31G*//B3LYP-D3(BJ)/TZVP (IEFPCM CH3OH) level following the STS strategy,13 resulting the mean absolute error (MAE), root mean square (RMS), and the statistic values of Pmean and Prel
|
MAE (ppm) |
RMS (ppm) |
Pmean (%) |
Prel (%) |
Calc. (10S,21S)-1 |
1.02 |
1.31 |
40.82 |
99.12 |
Calc. (10S,21R)-1 |
1.06 |
1.33 |
34.04 |
0.88 |
Calc. (10R,21S)-1 |
1.30 |
1.52 |
25.16 |
0.00 |
Calc. (10R,21R)-1 |
1.42 |
1.77 |
18.50 |
0.00 |
Finally, the absolute configuration of 1 was determined by the electronic circular dichroism (ECD) calculations using the time-dependent density functional theory (TDDFT) method at the B3LYP/6-31+g(d,p) level (details shown in ESI†). A pair of eligible enantiomers (10S,11R,17S,21S)-1 and (10R,11S,17R,21R)-1 were employed for calculation. As shown in Fig. 3, the calculated ECD curve of (10S,11R,17S,21S)-1 resembled the experimental ECD spectrum of 1, allowing its absolute configuration determined as depicted.
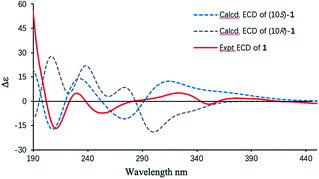 |
| Fig. 3 Experimental ECD spectra of 1, and calculated ECD spectra of (10S)-1 and (10R)-1 in MeCN. | |
In addition, the five known prenylated indole alkaloids (2–6) were identified as notoamide B (2),14 stephacidine A (3),14 notoamide E (4),15 asperochramide A (5)16 and deoxybrevianamide E (6),17 respectively, by comparing the spectroscopic data and physicochemical properties with those reported in the literature.
Structurally, beshanzuamide A (1) belongs to the family of p prenylated indole alkaloid, possessing a unique N,O-spiroketal motif connected to a pyranoindole-derived bicyclo[2.2.2]diazaoctane ring. A proposed biogenetic pathway for the assembly of compound 1 is shown in Scheme 1. The co-isolated stephacidine A (3)14 might be a precursor for the construction of 1 via the intermediacy of notoamide R.12 Oxidation cleavage of the Δ2(3) double bond in notoamide R would generate (i), which upon attack from N-1 to C-10 yields (ii). After amide hydrolysis, compound ii would convert to (iii), which would then dehydrate into (iv). Finally, compound 1 is afforded through Mannich-type N,O-ketalization.
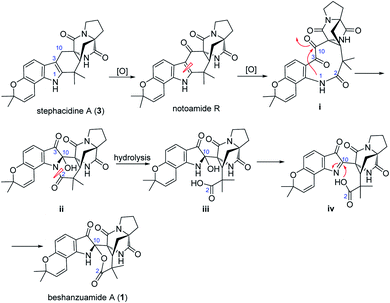 |
| Scheme 1 Plausible biogenetic pathway of 1 originated from 3. | |
All the isolates were evaluated for their cytotoxic activities against the human lung adenocarcinoma A549 cells and the human cervical carcinoma HeLa cells. However, none of them showed apparent cytotoxicities (IC50 > 50 μM). These prenylated indole alkaloids were also found to be inactive (IC50s > 20 μM) in the bioassays against PTP1B, ATP-citrate lyase (ACL), and acetyl-CoA carboxylate 1 (ACC1), which are potential drug targets associated with the glycolipid metabolic disorders.3,9
Conclusions
In conclusion, an unprecedented prenylated indole alkaloid, beshanzuamide A (1), was produced by an endophytic fungus Aspergillus sp. associated with the critically endangered conifer A. beshanzuensis. Featuring a unique azaspiro/δ-lactone functionality hitherto unknown among the family of prenylated indole alkaloid, beshanzuamide A may attract some attention of chemists and biologists who interested in its distinctive structure and synthesis. Further studies related to its bioactivities and biosynthetic mechanisms are warranted.
Experimental
General experimental procedure
NMR spectra were acquired using a Bruker Avance III 600 MHz spectrometer. Chemical shifts are indicated in δ (ppm) and referenced to the residual solvent signals. HR-ESI-MS was obtained on an AB 5600+ Q TOF spectrometer. Optical rotations were measured on a Rudolph Autopol IV-T polarimeter. HPLC was performed on a Shimadzu LC-20A HPLC system equipped with a Prominence SPD-20A UV–vis detector and an XBridge C18 column (5 μm, 250 × 10 mm; flow rate: 3.0 mL min−1). Column chromatography was operated using silica gel (100–200, 200–300 mesh, Jiang-You silysia Chemical Ltd, Yantai, China), MCI (CHP20P, 75-150 μm, Mitsubishi Chemical Industries, Tokyo, Japan) and Sephadex LH-20 (GE Healthcare Bio-Sciences AB, Uppsala, Sweden). TLC detection was measured by silica gel precoated plates (GF254, 0.2 ± 0.03 mm, Jiang-You silysia Chemical Ltd). Spots were visualised using UV light (254, 302 and/or 365 nm) and by spraying with 5% (v/v) H2SO4–EtOH followed by heating to 120 °C.
Isolation and identification of fungus
The fungal strain Aspergillus sp. Y-2 was isolated from the fresh leaves of A. beshanzuensis collected from the Baishanzu Mountain in Lishui, Zhejiang Province of China in April 2014 with the authorised permission. The plant was identified by Dr Ke-Jun Cheng (Lishui Institute of Agricultural science, China) with its taxonomic keys and samples are stored in biological ice packs and airlifted to the laboratory within 24 hours. The endophytic fungus Y-2 was presented by Dr Jin-Feng Hu's lab of Fudan University and identified as Aspergillus sp. by the morphological method and further corroborated by sequencing the ITS region. The ITS sequence of the strain (GenBank accession no. MN103998) manifested a high similarity with the known Aspergillus sp. isolate LN898693.1 (GenBank accession no. MK605980.1) on the BLSAT provided by NCBI (max score: 1002; Query cover: 100%; percent identity: 100.00%).
Fermentation, extraction, and isolation
Aspergillus sp. Y-2 was cultivated in rice medium for 30 days at 28 °C. The solid rice culture medium (3.2 kg) was soaked and extracted 3 times with EtOAc to give an EtOAc filtrate. Then, concentrate the filtrate under vacuum to obtain a dark brown oil extract (30.1 g). The crude extract was subjected to silica gel column (100–200 mesh) chromatography eluted with petroleum ether–EtOAc (20
:
1, 10
:
1, 5
:
1, 2
:
1, 1
:
1, 0
:
1, v/v) and EtOAc–MeOH (5
:
1, 1
:
1, 0
:
1, v/v) to obtain five fractions (Fr. 1–Fr. 5). Fr. 2 (2.3 g) was divided into five subfracticons (Fr. 2a–2e) by Sephadex LH-20 (in MeOH). Compounds 1 (tR = 18.6 min, 0.7 mg; MeOH/H2O, 60
:
40, v/v) was isolated from Fr. 2b by purification on semi-preparative HPLC. Fr. 4 (6.85 g) was further chromatographed on an MCI gel column with gradients of MeOH–H2O (7
:
3, 8
:
2, 9
:
1, 1
:
0) to give three fractions (Fr. 4a–4c). Fr. 4a was purified by semi-preparative reversed-phase HPLC (MeCN/H2O, 45
:
55, v/v) to obtain compound 5 (tR = 15.3 min, 1.0 mg). Compounds 6 (tR = 13.2 min, 8.5 mg) and 3 (tR = 19.1 min, 8.6 mg) were obtained from Fr. 4b by purification on HPLC (MeOH/H2O, 65
:
35, v/v), whereas compounds 2 (tR = 12.1 min, 8.5 mg) and 4 (tR = 17.3 min, 7.0 mg) were purified from Fr. 4c by semi-preparative HPLC (MeOH/H2O, 60
:
40, v/v).
Beshanzuamide A (1): orange amorphous powder; [α]25D −164.2 (c 0.50, MeOH); UV (MeOH) λmax (log
ε) 261 (4.32), 339 (3.21), 396 (2.81) nm; ECD (0.08, MeOH) λmax (Δε) 213 (−15.8), 235 (2.7), 253 (−7.2), 324 (5.1),352 (−1.8), 377 (1.9) nm. 1H and 13C NMR data, see Table 1; HR-ESI-MS m/z: 478.1977 [M + H]+ (calcd for C26H27N3O6, 478.1973).
Quantum chemistry calculations
The software Crest18 was used to search the four possible relative configurations for 1 [(10S*,11R*,17S*,21S*)-1 (1a), (10S*,11R*,17S*,21R*)-1 (1b), (10R*,11R*,17S*,21S*)-1 (1c), and (10R*,11R*,17S*,21R*)-1 (1d)] on GFNFF19 level of theory, followed by optimization on GFN2-XTB20 level with 4 kcal mol−1 energy window to remove high energy conformers. Optimization and frequency calculation of each conformer was performed on B3LYP-D3(BJ)/TZVP (IEFPCM, CH3OH) level of theory. DFT GIAO 13C NMR calculation was calculated on the ωB97xD/6-31G* (IEFPCM, CH3OH) level, and the data processing followed the reported STS protocol.13 The calculated shielding tensors of conformers were Boltzmann averaged based on Gibbs free energy. All DFT calculations were performed by Gaussian 16 software package.21
ECD calculation
Monte Carlo conformational searches were carried out by means of the Spartan's 10 software using Merck Molecular Force Field (MMFF). The conformers with Boltzmann-population of over 0.5% were chosen for ECD calculations, and then the conformers were initially optimized at B3LYP/6-31g level in gas. The theoretical calculation of ECD was conducted in MeOH using Time-dependent Density functional theory (TD-DFT) at the B3LYP/6-31+g(d,p) level for all conformers of compounds (10S,11R,17S,21S)-1 and (10R,11S,17R,21R)-1. Rotatory strengths for a total of 40 excited states were calculated. ECD spectra were generated using the program SpecDis 1.6 (University of Würzburg, Würzburg, Germany) and GraphPad Prism 5 (University of California San Diego, USA) from dipole-length rotational strengths by applying Gaussian band shapes with sigma = 0.3 eV.
Cytotoxicity assay
The cytotoxicity of compounds 1–6 against A549 and HeLa cell lines was evaluated by CCK8 method.22 Doxorubicin was used as a positive control.
Bioassays against ACL, ACC1 and PTP1B
The bioassays of compounds 1–6 against ACL, ACC1 and PTP1B were evaluated according to the protocols as described previously.23–26
Conflicts of interest
There are no conflicts to declare.
Acknowledgements
This work was supported by the NSFC grant (No. 21937002). The authors thank Associate Prof. Li Jing (Department of Pharmacy, Xiangya Hospital, Central South University, Changsha, PR China) for his kind suggestions and assistance with the NMR quantum computations.
Notes and references
- F. Zhu, C. Qin, L. Tao, X. Liu, Z. Shi, X. Ma, J. Jia, Y. Tan, C. Cui, J. Lin, C. Tan, Y. Jiang and Y. Chen, Proc. Natl. Acad. Sci. U. S. A., 2011, 108, 12943–12948 CrossRef CAS PubMed.
- M. A. Ibrahim, M. Na, J. Oh, R. F. Schinazi, T. R. McBrayer, T. Whitaker, R. J. Doerksen, D. J. Newman, L. G. Zachos and M. T. Hamann, Proc. Natl. Acad. Sci. U. S. A., 2013, 110, 16832–16837 CrossRef CAS PubMed.
- For the most recent studies on REPs, see: J. Xiong, P.-J. Zhou, H.-W. Jiang, T. Huang, Y.-H. He, Z.-Y. Zhao, Y. Zang, Y.-M. Choo, X. Wang, A. G. Chittiboyina, P. Pandey, M. T. Hamann, J. Li and J.-F. Hu, Angew. Chem., Int. Ed., 2021, 60, 22270–22275 Search PubMed , (part XXI of the ‘Phytochemical and biological studies on rare and endangered plants endemic to China’ series)..
- R.-X. Tan and W.-X. Zou, Nat. Prod. Rep., 2001, 18, 448–459 RSC.
- S. L. Shao, Z. F. Jin and Y. H. Weng, J. Wood. Sci., 2008, 54, 81–86 CrossRef CAS.
- Y. Yang, D. Zhang, D. Luscombe, W. Liao, A. Farjon, T. Katsuki, Q. Xiang and N. Li, Abies beshanzuensis, The IUCN Red List of Threatened Species 2013, eT32318A2814360, RLTS.T32318A2814360.en, DOI:10.2305/IUCN.UK.2013-1.
- L.-K. Fu and J.-M. Jin, China Plant Red Data Book - Rare and Endangered plants I, Science Press, Beijing, New York, 1992 Search PubMed.
- The State Forestry Administration and the Ministry of Agriculture. List of Wild Plants of National Priority Protection I, 1999, http://www.gov.cn/gongbao/content/2000/content_60072.htm Search PubMed.
- C.-L. Hu, J. Xiong, J.-Y. Li, L.-X. Gao, W.-X. Wang, K.-J. Cheng, G.-X. Yang, J. Li and J.-F. Hu, Eur. J. Org. Chem., 2016, 10, 1832–1835 CrossRef.
- Y.-X. Zhu, C. Peng, S. Yu, J.-F. Hu and J.-Y. Li, Nat. Prod. Res., 2021, 19, 3248–3253 CrossRef PubMed.
- Y.-X. Zhu, C. Peng, W. Ding, J.-F. Hu and J.-Y. Li, Nat. Prod. Res., 2020 DOI:10.1080/14786419.2020.1844700.
- S. Tsukamoto, H. Umaoka, K. Yoshikawa, T. Ikeda and H. Hirota, J. Nat. Prod., 2010, 73, 1438–1440 CrossRef CAS PubMed.
- J. Li, J.-K. Liu and W.-X. Wang, J. Org. Chem., 2020, 85, 11350–11358 CrossRef CAS PubMed.
- T. J. Greshock, A. W. Grubbs, S. Tsukamoto and R. M. Williams, Angew. Chem., Int. Ed., 2007, 46, 2262–2265 CrossRef CAS.
- A. W. Grubbs, G. D. I. Artman, S. Tsukamoto and R. M. Williams, Angew. Chem., Int. Ed., 2007, 46, 2257–2261 CrossRef CAS PubMed.
- H. Wen, X. Liu, Q. Zhang, Y. Deng, Y. Zang, J. Wang, J. Liu, Q. Zhou, L. Hu and H. Zhu, Chem. Biodivers., 2018, 15, e1700550 CrossRef PubMed.
- H. Li, W. Sun, M. Deng, Q. Zhou, J. Wang, J. Liu, C. Chen, C. Qi, Z. Luo, Y. Xue, H. Zhu and Y. Zhang, J. Org. Chem., 2018, 83, 8483–8492 CrossRef CAS.
- P. Pracht, F. Bohle and S. Grimme, Phys. Chem. Chem. Phys., 2020, 22, 7169–7192 RSC.
- S. Spicher and S. Grimme, Angew. Chem., Int. Ed., 2020, 59, 15665–15673 CrossRef CAS PubMed.
- C. Bannwarth, S. Ehlert and S. Grimme, J. Chem. Theory Comput., 2019, 15, 1652–1671 CrossRef CAS PubMed.
- M. J. Frisch, G. W. Trucks, H. B. Schlegel, G. E. Scuseria, M. A. Robb, J. R. Cheeseman, G. Scalmani, V. Barone, G. A. Petersson, H. Nakatsuji, X. Li, M. Caricato, A. V. Marenich, J. Bloino, B. G. Janesko, R. Gomperts, B. Mennucci, H. P. Hratchian, J. V. Ortiz, A. F. Izmaylov, J. L. Sonnenberg, D. Williams-Young, F. Ding, F. Lipparini, F. Egidi, J. Goings, B. Peng, A. Petrone, T. Henderson, D. Ranasinghe, V. G. Zakrzewski, J. Gao, N. Rega, G. Zheng, W. Liang, M. Hada, M. Ehara, K. Toyota, R. Fukuda, J. Hasegawa, M. Ishida, T. Nakajima, Y. Honda, O. Kitao, H. Nakai, T. Vreven, K. Throssell, J. A. Montgomery Jr, J. E. Peralta, F. Ogliaro, M. J. Bearpark, J. J. Heyd, E. N. Brothers, K. N. Kudin, V. N. Staroverov, T. A. Keith, R. Kobayashi, J. Normand, K. Raghavachari, A. P. Rendell, J. C. Burant, S. S. Iyengar, J. Tomasi, M. Cossi, J. M. Millam, M. Klene, C. Adamo, R. Cammi, J. W. Ochterski, R. L. Martin, K. Morokuma, O. Farkas, J. B. Foresman, and D. J. Fox, Gaussian 16, Revision C.01, Gaussian, Inc., Wallingford CT, 2016 Search PubMed.
- H. Tominaga, M. Ishiyama, F. Ohseto, K. Sasamoto, T. Hamamoto, K. Suzuki and M. Watanabe, Anal. Commun., 1999, 36, 47–57 RSC.
- S. K. Koerner, J.-I. Hanai, S. Bai, F. E. Jernigan, M. Oki, C. Komaba, E. Shuto, V. P. Sukhatme and L. J. Sun, Eur. J. Med. Chem., 2017, 126, 920–928 CrossRef CAS PubMed.
- N. J. Halbrook, R. V. Lawrence, R. L. Dressler, R. C. Blackstone and W. Herz, J. Org. Chem., 1964, 29, 1017–1021 CrossRef CAS.
- G. Harriman, J. Greenwood, S. Bhat, X. Y. Huang, R. Y. Wang, D. Paul, L. Tong, A. K. Saha, W. F. Westlin, R. Kapeller and H. J. Harwood, Proc. Natl. Acad. U. S. A., 2016, 113, E1796–E1805 CrossRef CAS PubMed.
- J. Xiong, Z.-L. Hong, L.-X. Gao, J. Shen, S.-T. Liu, G.-X. Yang, J. Li, H. Q. Zeng and J.-F. Hu, J. Org. Chem., 2015, 80, 11080–11085 CrossRef CAS PubMed.
Footnotes |
† Electronic supplementary information (ESI) available: The structures of notoamide O and compound 1; GIAO 13NMR data computational details for 1; ECD computational details for 1; original HR-ESIMS and 1D/2D NMR spectra of 1. See DOI: 10.1039/d2ra01578a |
‡ These authors contributed equally to this work. |
|
This journal is © The Royal Society of Chemistry 2022 |
Click here to see how this site uses Cookies. View our privacy policy here.