DOI:
10.1039/D2RA00796G
(Review Article)
RSC Adv., 2022,
12, 11233-11254
A comprehensive review on the decontamination of lead(II) from water and wastewater by low-cost biosorbents
Received
7th February 2022
, Accepted 22nd March 2022
First published on 12th April 2022
Abstract
The disadvantages of conventional methods in water and wastewater management including the demand for high energy consumption, the creation of secondary toxic sludge, and operation cost are much too high for developing countries. However, adsorption using low-cost biosorbents is the most efficient non-conventional technique for heavy metals removal. The high adsorption capacities, cost-effectiveness, and the abundance of agricultural waste materials in nature are the important parameters that explain why these biosorbents are economical for heavy metals removal. The present investigation sought to review the biosorption of lead [Pb(II)] onto low-cost biosorbents to understand their adsorption mechanism. The review shows that biosorption using low-cost biosorbents is eco-friendly, cost-effective, and is a simple technique for water and wastewater treatment containing lead(II) ions. The batch biosorption tests carried out in most studies show that Pb(II) biosorption by the low-cost biosorbents is dependent on biosorption variables such as pH of the aqueous solution, contact time, biosorbent dose, Pb(II) initial concentration, and temperature. Furthermore, batch equilibrium data have been explored in many studies by evaluating the kinetics, isothermal and thermodynamic variables. Most of the studies on the adsorptive removal of Pb(II) were found to follow the pseudo-second kinetic and Langmuir isotherm models with the thermodynamics variables suggesting the feasibility and spontaneous nature of Pb(II) sequestration. However, gaps exist to increase biosorption ability, economic feasibility, optimization of the biosorption system, and desorption and regeneration of the used agricultural biosorbents.
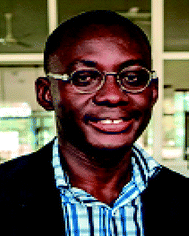 Jonas Bayuo | Jonas Bayuo is a PhD Scholar at the Nelson Mandela African Institution of Science and Technology, Arusha-Tanzania. He completed his bachelor's degree in Science Education (Chemistry and Physics options) from the University for Development Studies, Tamale-Ghana and obtained his Master's degree in Physical Chemistry from the same University. He works at the C. K. Tedam University of Technology and Applied Sciences, Navrongo-Ghana. His research interests are adsorption of heavy metals, phenols, dyes, modeling, and optimization of adsorption variables. Currently, he is interested in the decontamination of toxic pollutants from water and wastewater using natural and waste materials. |
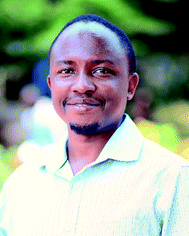 Mwemezi Rwiza | Dr Rwiza holds a PhD in Earth Sciences and Environmental Engineering from Gwangju Institute of Science and Technology (GIST), South Korea. He also holds a master's degree in environmental studies and Sustainability Science from Lund University, Sweden. He works at the Nelson Mandela African Institution of Science and Technology (NM-AIST) where he lectures and researches in the fields of water and wastewater treatment; remediation of mine sites; biomaterial synthesis, characterization, and application; environmental pollution, monitoring, protection and remediation. |
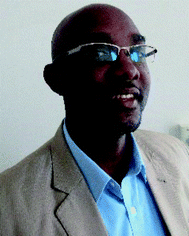 Kelvin Mtei | Kelvin Mtei is an associate professor of agriculture and environmental sciences with vast experience in research and academics for about eighteen years. He has been delivering lectures in various universities both at undergraduate and postgraduate levels. His research emphasizes application of science and technology in social-ecological context with a focus on sustainable farming systems; remediation of degraded land; agricultural water management and re-use. He has received several research grants through which he has produced more than 70 publications in peer review journals, conference proceedings and books. |
1. Introduction
Agricultural and industrial activities have become pollutant sources in recent times, primarily concerning the bioaccumulation of toxic metals in the ecosystem. This results in a significant escalation of the amount of these metals in surface and ground waters thereby contaminating the aquatic bodies. Heavy metals are hazardous and detrimental to living species especially when they could be distributed through the food chain.1 The manifestation of toxic ions in the aquatic environment is a fundamental universal concern attributable to their continuous-release, venomous nature and other deadly effects on the receiving aqueous media.
Lead(II) is commonly present in effluents and sewages from industries such as paint, pesticides, battery, mine, and smelting. The various sources of Pb(II) contamination in the environment are depicted in Fig. 1. Reports indicate that grown-ups engross 5–15% of lead(II) and nearly 5% of it is being retained and the existence of 0.5–0.8 μg per mL of lead(II) in the blood of living organisms leads to numerous health conditions.2 The toxicity of lead(II) leads to widespread health disorders including pregnancy miscarriages in women, severe stomachache, hypertension, impaired blood synthesis, brain, and kidney damage.3 Besides, lead(II) is a universal pollutant on earth, and aqueous media and its exposure cause feebleness in ankles, fingers, and wrists.4 Some of the toxic effects of Pb(II) on plants, animals, and humans are shown in Fig. 2.
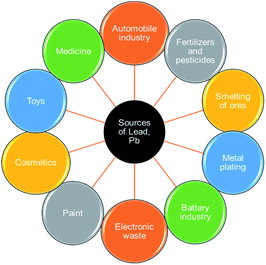 |
| Fig. 1 Various sources of Pb(II) pollution in the environment (this figure has been adapted from Mohd et al.7 with permission from Springer, copyright 2021). | |
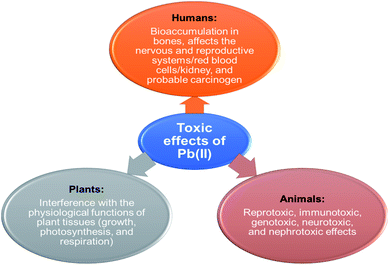 |
| Fig. 2 Toxic effects of Pb(II) on plants, animals, and humans (this figure has been adapted from Abidli et al.8 with permission from Elsevier, copyright 2021). | |
Many treatment systems have been probed for heavy metals reduction from wastewater and water; however, many of these management processes are significantly pricy and incompetent of eliminating low-level concentrations of heavy metals. In comparison, adsorption (biosorption) appears to be more resourceful and used worldwide owing to the greater yield and cost-effectiveness of the biosorbents.5 Biosorption is a simple and efficient reversible reaction that takes place between heavy metals and biomass functional groups. Several studies have demonstrated Pb(II) adsorption potentials by natural and agro-based materials leading to multiple sorption mechanisms involving ion exchange, precipitation with inorganic components, interactions with π electrons, and complexation with oxygen-containing functional groups.6
The objective of the present study is to conduct a review on the biosorption of lead(II) ions from water media on low-cost biosorbents especially those obtained from natural and agro-based materials. The effects of biosorption variables as well as biosorption isotherms, kinetics, and thermodynamic variables evaluated from the adsorption equilibrium data were also reviewed.
2. Heavy metals
In the environment, trace metals exist naturally, particularly in the Earth's crust, and add to the balancing of the planet.9 At the non-anthropogenic origin, heavy metals always exist at background concentration levels where their presence in soils could be due to weathering of parent rocks. In the ecosystem, heavy metals occur naturally with large variations in concentration. Heavy metals pollution emanates from metals purification including various sources. Especially, copper smelting, nuclear fuels preparation, and electroplating of metals have led to the production of chromium and cadmium.10 Other sources of metal ions pollution include wet and dry fallouts of atmospheric particulate matter; dead and decomposing vegetation, animal matter, as well as from man's activities.9,11 Anthropogenic activities that result in toxic metal pollution are cement industry and mining operations, smelting procedures, steelworks, burning of coal and oil.12 Wuana and Okieimen13 indicated that agricultural fields could be contaminated due to improper treatments and disposal of mine drainages. Heavy metal ions can be in existence for a lengthy time in different inorganic and organic colloids before being exposed to living species.14 They are indestructible and do not decay with time. Most metal ions are present at trace levels in soils, vegetation, and in humans, plants, and other living species as micro-elements.15 Traces of heavy metals are not lethal in plants and animals at low concentration levels. However, there are few exemptions, cadmium, chromium, mercury, and lead are poisonous at very low concentrations.16
Toxic metals can enter the human system via food, air, and dermal contact absorption.17 They also accumulate in aquatic biota, soils, and plants.9 Biomagnification of metal ions may occur when an organism ejects it slower than it ingests and this can be detrimental to living organisms.18 The bioaccumulation of heavy metal ions tends to be very hazardous as a result of their extended biological half-lives.19
As shown in Fig. 3, heavy metals can pollute the ecosystem due to various pathways through natural processes [1], and anthropogenic activities [2], leading to detrimental effects in the environment including direct pollution of the aquatic environment [3], plants [4], as well as triggering toxic effects on humans [5] through different intake paths such as food chain (drinking water and food), inhalation, and skin contact [6].
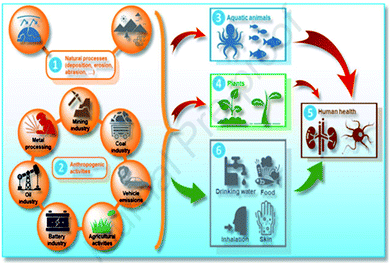 |
| Fig. 3 Schematic illustration of the pathway of heavy metals and metalloids pollutants in the ecosystem (this figure has been adapted from Abidli et al.8 with permission from Elsevier, copyright 2021). | |
2.1 Heavy metals presence and pollution in the ecosystem
The discharge of metal ions into the ecosystem occurs over wide-ranging processes and pathways including to the surface waters via runoff, transport to soil; hence, into groundwaters and crops as well as to air during combustion.20,21 Heavy metal ions occurrence in the soils could be accredited to different environmental factors, including, but not restricted to soil characteristics, parent materials, and human activities for example farming and irrigation.22 Toxic metals in soil can influence crop growth negatively and as well as interferes with the degeneration of major cell organelles, metabolic processes in plants, inhibition of respiration and photosynthesis.23
Furthermore, the production and usage of synthetic products such as paints, pesticides, and batteries can lead to heavy metal pollution of agricultural soils and the environment at large.24 Contamination of soils by heavy metals is primarily due to the use of wastewaters obtained from mining operations to irrigate paddy fields.25 Heavy metals have the potential of polluting groundwater because of their high rate of transfer in soil profiles. The potentiality of metal ions accumulation and bioaccumulation in soils leads to serious food chain contamination.26 In Ghana, organochlorine pesticides are widely been used for public health and agricultural purposes for more than 40 years with their remains found in sediments, water, humans, and crops.27 For instance, crop and vegetable farming had led to misuse and excessive use of pesticides. In Ghana, pesticides form part of agriculture and it has been estimated that about 87.00% of farmers depend on pesticides to prevent diseases and pests on vegetable farms, rice fields, cotton, and cocoa plantations and among others.28 It has also been reported that several toxic metals are found to be present in drinking water media in some mining areas in the country.
Global worries have suddenly increased over toxic metals discharged from various sources into the environment due to their inability to degrade and so they linger in the environment.22 Metal circulation between soil and vegetation is a very important issue in evaluating the effect of metals on the environment.29
The main sources of heavy metals in the environment from anthropogenic activities and their implication on human beings and other life forms are presented in Fig. 4.
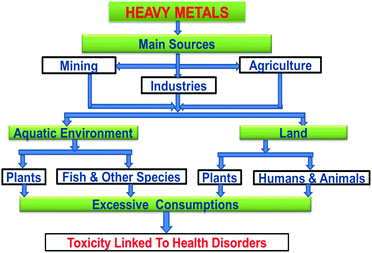 |
| Fig. 4 Sources of heavy metals and their impact on living organisms. | |
2.2 Effect of heavy metals
The toxicity of metal is mainly defined based on its concentration required to cause an acute response generally a sub-lethal response or death. Several trace elements are recognized to be noxious to human beings and other living species when consumed beyond their concentration required for healthy growth. Some of these heavy metals [arsenic (As), chromium (Cr), cadmium (Cd), lead (Pb), nickel (Ni), mercury (Hg), and zinc (Zn)] and their harmful effects in living organisms when bio-accumulated in higher concentration than their acceptable levels are presented in Fig. 5. There could be a high tendency of metal ions concentration build-up in living tissues once they become part of the food chain and cause severe health disorders when ingestion exceeds the permitted concentration.30 Heavy metal toxicity has been found to have an inhibitory influence on enzymatic functions, plant development, stoma roles, photosynthesis processes as well as the destruction of the root system.31 The health risks assessment of metal ions pollution of potable water bodies are most imperative due to their lethal effects and capability of bioaccumulation via the food chain.32 Different metals show alterations in the level of harmfulness, some are carcinogenic though other effects may include cardiovascular,33 epidermal systems alteration,34 and hematological, neurological, gastrointestinal, immunological, and musculoskeletal effect.35 Fig. 6 depicts the human organs usually affected as a result of the discharge of excessive heavy metal ions into the environment from different sources. Highly toxic metal ions cause destructive effects even at small concentration levels.36 The dangers of heavy metals have become the most current environmental health concern because they are potentially dangerous due to bioaccumulation through the food chain.25 Mostly, heavy metals are distributed, concentrated, and chemically modified through human activities that may increase their toxicity. These activities result in greater concentrations of metal ions relative to their normal background concentrations.25 Contamination with metal ions poses a serious threat to agriculture and other sources of food for humanity, reducing plant growth and reducing the resistance to pests and diseases. This affects the quality of groundwater, plant growth, food, and microbes.10 The effects of heavy metals on microbes can lead to reduced waste degradation and nitrogen fixation, inefficient nutrient circulation, and impaired enzyme synthesis.25 Poisonous ions persist in the ecosystem for a prolonged time and do not decompose.37 Rapid exposure to toxic trace elements in marine and terrestrial organisms can have a negative toxic effect.38 For example, coastal fish and seabirds are often observed for the presence of toxins, such as exposure to poisonous metals due to the food chain. It is generally difficult to predict heavy metals exposure effects on species since these metals may be essential or non-essential and very low concentrations of essential metals can be as harmful as high concentrations.
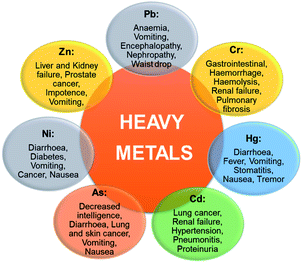 |
| Fig. 5 The effects of heavy metals on human health (this figure has been adapted from Mohd et al.7 with permission from Springer, copyright 2021). | |
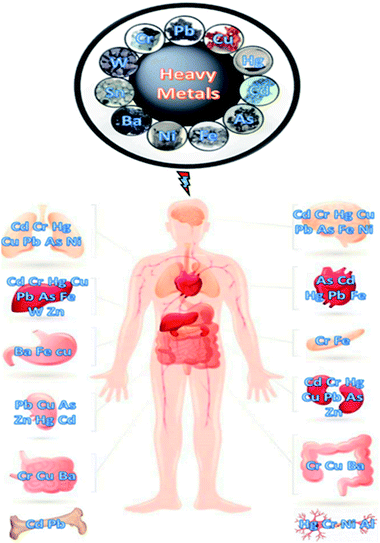 |
| Fig. 6 Illustration of human organs affected due to heavy metals toxicity (this figure has been reproduced from Perumal et al.40 with permission from MDPI, copyright 2021). | |
Table 1 depicts the Maximum Allowable Limits (MAL) and health implications of different heavy metals.22,39
Table 1 Maximum allowable limits (MAL) and health implications of heavy metals
Heavy metal |
MAL for effluent discharge from industries (mg L−1) |
MAL for drinking water (mg L−1) |
Health implication |
WHO |
Inland surface water bodies |
Marine coastal regions |
WHO |
European Union standard |
USEPA |
Arsenic |
— |
0.2 |
0.01 |
0.01 |
0.01 |
Cancerous and gastrointestinal disorder |
Cadmium |
0.1 |
2.0 |
0.003 |
0.005 |
0.005 |
Causes cancer, dyspnoea, and lung fibrosis |
Copper |
0.05–1.5 |
3.0 |
2.0 |
0.2 |
1.3 |
Causes stomach pain, irritation of the eyes, nasal cavity, mouth, and headache |
Chromium |
— |
2.0 |
0.05 |
0.05 |
0.1 |
Causes tumor in lungs and cancer |
Iron |
0.1–1.0 |
3.0 |
0.2 |
0.2 |
0.3 |
Causes hypertension, contraction of the blood vessel and pulse rate |
Lead |
0.1 |
2.0 |
0.01 |
0.01 |
0.15 |
Causes anemia, joint and muscle ache, elevated blood pressure, kidney inflammation, and carcinogen |
Manganese |
0.05–0.5 |
2.0 |
0.5 |
0.05 |
0.05 |
Fatigue, blindness, and sexual impotence |
Mercury |
— |
0.01 |
0.001 |
0.001 |
0.02 |
Diarrhea, headache, abdominal effects, loss of appetite, and paralysis |
Nickel |
— |
5.0 |
0.02 |
0.02 |
0.1 |
Cancer of the lung and acute bronchitis |
Zinc |
5.0–15.0 |
15.0 |
3.0 |
— |
5.0 |
Causes discomfort and metal fume fever |
2.3 Application of conventional adsorbents in heavy metals decontamination
Earlier adsorbents used for metal ions removal were commercial adsorbents and have been used globally and extensively for controlling water contamination. In literature, the commonly utilized conventional adsorbents are activated carbons, ion-exchange resins (polymeric organic resins), and inorganic materials such as activated alumina, silica gel, zeolites, and molecular sieves. However, only four types of generic adsorbents have enormous surface areas and have dominated the commercial use of adsorption: activated carbons > zeolites > silica gel > activated aluminas.41
Silica gel is worldwide recognized as an inorganic polymer that is used in chromatographic columns. Its high porous surface topology and enormous specific surface area are key for effective heavy metal ion interaction. In furtherance, silica gel is easily available as a substrate for immobilizing various chemical functional groups.42 Several silicate-derived gels have been explored to extract, stabilize, and/or decontaminate arsenic and other heavy metal ions from water and wastewater.43 Due to its strong physical strength, endurance, and high chemical stability, silica gel has been utilized in the sequestration of certain contaminants including solid wastes.43,44 El-Moselhy et al.44 were successful in developing hydrated iron oxide nanoparticles using silicate matrix, resulting in high arsenic detoxification. Mesoporous silica materials of MCM-41 type were efficiently utilized by Zhu et al.45 in the removal of Cu(II), Pb(II), and Cd(II) from aqueous solutions. More so, a silica gel material modified with nitrilotriacetic acid (NTA-silica gel) was applied for the elimination of Cu(II), Cd(II), and Pb(II) from the aqueous system, which indicates high removal efficiency of the three heavy metal ions.42 Furthermore, silica gel polymer was proven to be effective in Ni(II) removal from aqueous solution.46
Zeolites are micro-porous crystal-like solids with definite structures and are naturally-occurring materials comprising hydrated aluminosilicate with maximum cation exchangeability with metal ions.47 Zeolite has a highly porous structure in three-dimensional crystal lattices that display strong cation interaction and ion exchange ability with heavy metal ions.48 Due to their high porosity and sieving characteristics, zeolites are a good choice for removing heavy metal ions from untreated wastewater. They also have a high ion exchange ability, and the exchangeable ions (Na+, Ca2+, and K+ ions) are largely harmless.49 Based on their quick advancements in properties, zeolites are gaining in popularity and are being used in a variety of innovative applications. Biomedical, radioprotection, wastewater purification, biosensor, pure, and applied chemical applications are all driving interest in them.
When using zeolites in sorption, the surface area is not a matter of concern because it is a selective process and it is reversible.50 Zeolites are used practically in the fuel industries as water softeners, manufacture of detergents, catalysts, and making molecular sieves. Quite a lot of zeolites have been employed as adsorbents for the treatment of contaminants in water and wastewater. A zeolite produced from fly ash was capable of decontaminating Hg(II) and Pb(II) ions from aqueous media.51 Chen et al.52 designed and prepared zeolite cotton as a form of filter for household water treatment. More so, zeolite composite adsorbents were applied to simultaneously detoxicate heavy metals and total coliforms from wastewater.53 A high-quality zeolite type A was produced and utilized successfully in removing heavy metals from water systems.54 Besides, zeolite-alginate composites were found to be promising in the Pb(II) removal from contaminated water solutions.55 Makki56 employed zeolite A4 for Cd(II) and Pb(II) decontamination in water. Also, Cu(II), Ni(II), and Pb(II) ions in an acid mine drainage were removed using Philippine natural zeolite.57
Activated alumina is one of the most extensively utilized adsorbents for heavy metals sequestration due to its high affinity for metal ions.58 It consists of porous aluminum oxide with a wide surface area that is resilient to thermal stress and abrasion. It does not swell, shrink, soften, or disintegrate throughout the adsorption process.59 Furthermore, activated alumina has outstanding adsorption capabilities and a high ion uptake capacity, as well as being cost-effective, safe, and ecologically friendly, making it suitable for use in wastewater treatment.60 Activated alumina has a good surface area and is frequently applied to get rid of oxygenates and mercaptans of hydrocarbons and especially, fluorides in water.61 A nano-alumina was produced and used effectively in As(V) decontamination from an aqueous solution.62 Furthermore, novel γ-alumina nanoparticles were developed for Ni(II) decontamination from the solvent phase and found proficient.63 In a study, a mesoporous carbon stabilized alumina was employed in removing Cd(II) and Pb(II) ions from aqueous media.64 Besides, an alumina composite was shown to be effective in the removal of both Cr(VI) and methylene blue from aqueous solutions.65
Globally, the elimination of noxious pollutants in water has been attributed to activated carbons. The well-known and lasting adsorbent in water and wastewater treatment is charcoal. The preparation of activated charcoal involves three stages; dehydration, carbonization, and activation. Due to the high surface area of activated carbons, they are highly recognized and recommended as proficient adsorbents for water contaminants treatment and gas purification.66,67 Activated carbons are black and made up of solid carbonaceous materials with high porosity, internal surface area, and mechanical strength. Granular activated carbon, powdered activated carbon, and activated carbon cloth are among the different forms of activated carbon used to remove contaminants from water and wastewater.68 The constant utilization of activated carbons has produced fruitful outcomes in contaminant management processes.41 Hu et al.69 report the applicability of humic acid-impregnated activated carbon in removing Cu(II). Meanwhile, Jjagwe et al.70 previously reviewed the synthesis and application of granular activated carbon from biomass waste materials for water treatment. Besides, Manjuladevi et al.71 investigated Cr(VI), Ni(II), Cd(II), and Pb(II) ions removal from aqueous solutions using activated carbon prepared from Cucumis melo peel. Fan and Anderson72 carried out a related study using granulated activated carbon to eradicate Cu(II) and Cd(II). Also, activated carbon has been applied in the decontamination of Pb(II) as recounted by many studies.73,74
Regardless of the capabilities and prolific application of commercial activated carbons, there are some constraints on usage attributable to its high cost. For that reason, attention has been directed towards the preparation and production of cost-effective adsorbents from natural materials that are readily available, carbonaceous, and easy to activate.75 To produce activated carbon, an organic material undergoes thermal degradation for subsequent decomposition into carbon granules.70
2.4 Application of agricultural waste materials in heavy metals removal
Commercially activated carbons are expensive adsorbents regardless of their widespread use in water and wastewater treatments. This has generated an increased interest in the production of safe, cheap, and economical adsorbents, which are more economical than commercially available activated carbon aimed at removing heavy metals from polluted resources.76,77 Natural and waste materials of agriculture and industries are extensively being modified and converted into activated carbons aimed at heavy metals cleansing from wastewater.75 For instance, Fig. 7 demonstrates the application of activated carbons as adsorbents derived from agricultural wastes for water and wastewater treatment. An effective adsorbent should have a wide adsorption capability, a fast rate of sorption, be easy to regenerate or retrieve from water, have high porosity, and have a small pore diameter, because adsorption ability is proportional to the amount of surface area available.78 Typical adsorption properties of these materials are derived polymers complexes such as lignin, simple sugars, hemicellulose, proteins, lipids, and starch with diverse functional groups. These constituents play a vital role in the adsorption of heavy metal ions as they can form complexes and chelates with heavy metal ions. They are capable of replacing hydrogen ions with hazardous heavy metal ions dissolved in the aqueous media or donating a pair of electrons to bind these metal ions.79
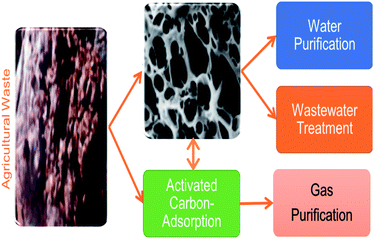 |
| Fig. 7 Application of low-cost activated carbons derived from agricultural wastes for environmental remediation (this figure has been adapted from Heidarinejad et al.85 with permission from Springer, copyright 2020). | |
The functional groups that are present in agricultural wastes include carbonyl, acetamido, phenolic, amido, carboxyl, amino, alcohols, esters, and sulphydryl.80 It is these groups, which possess an affinity for complexation with metals. During adsorption studies, various studies have confirmed the existence of functional groups and the binding ability of these groups with metal ions through spectroscopy analysis.81–83
Toxic metals elimination by dint of low-cost biosorbents is regarded as more promising and cost-effective since there are numerous natural resources available locally and plentifully, which could be used as low-cost biosorbents.84 The development of agro-based materials into less expensive biosorbents is well acknowledged as a prospective and economical for the treatments of pollutants in water. The sources of agro-based biosorbents are presented in Fig. 8. The increasing number of studies or researches and publications on the adsorption process (as shown in Fig. 9) using low-cost adsorbents conclude and recommend the increasing interest in the search for more low-cost adsorbents that are suitable for removing pollutants. As a result, several studies have reported the uses of agro-based adsorbents in eliminating heavy metals from water, wastewater, or effluents. Some of these studies are summarized in Table 2.
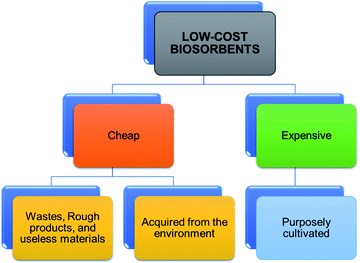 |
| Fig. 8 Sources of agro-based biosorbents. | |
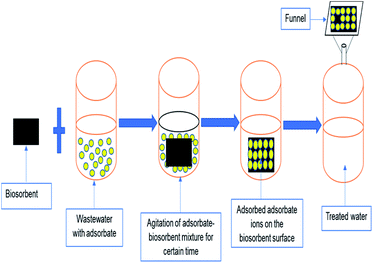 |
| Fig. 9 Adsorption process to clean up polluted water using low-cost adsorbents. | |
Table 2 Agricultural waste bioadsorbents for heavy metal ions sequestration
S/N |
Bioadsorbent |
Metal ion |
Removal efficiency or Qmax |
References |
1 |
Thiolated saw dust |
Pb(II) and Co(II) |
2.87 mg g−1 |
84 |
2 |
Groundnut shell |
Cr(VI), Pb(II) |
87.6 and 96.61%, respectively |
82 and 86 |
3 |
Shea fruit biomass |
Cd(II) |
76.86% |
76 |
4 |
Coffee waste-derived biochars |
Cd(II) and Pb(II) |
11.41 and 1.18 mg g−1, respectively |
122 |
5 |
Banana peels |
Cu(II) and Pb(II) |
99.79 and 88.94%, respectively |
123 |
6 |
Spent tea leaves |
As(V) |
87% |
124 |
7 |
Coconut shell char |
Ni(II) |
0.58 mg g−1 |
125 |
8 |
Olive stones |
Cd(II), Cu(II), Pb(II) and Cr(VI) |
77.4, 80.5, and 94.5%, respectively |
79 |
9 |
Brewed tea waste |
Pb(II), Zn(II), Ni(II), and Cd(II) |
1.197, 1.457, 1.163 and, 2.468 mg g−1, respectively |
126 |
10 |
Orange peel |
Cd(II) |
128.23 mg g−1 |
127 |
11 |
Jute stick activated carbon |
Cd(II) |
73.53 mg g−1 |
128 |
12 |
Oil palm fibers |
Pb(II), Cu(II), Fe(II) and Zn(II) |
16.67, 16.59, 16.65 and 16.54 mg g−1, respectively |
129 |
13 |
Sunflower waste carbon |
Cd(II) |
99.8% |
130 |
14 |
Modified corn stalks biochar |
Cr(VI) |
138.89 mg g−1 |
131 |
15 |
Dialium guineense seed shell |
As(III) |
47.08 mg g−1 |
132 |
16 |
Sagwan sawdust |
Cr(VI) |
9.62 mg g−1 |
133 |
17 |
Saccharomyces cerevisiae biomass |
As(III) and As(V) |
66.2 and 15.8%, respectively |
134 |
18 |
Activated carbon from sugarcane bagasse |
Hg(II) |
61% |
135 |
19 |
Succinylated hay |
Cd(II) and Ni(II) |
75.19 mg g−1 and 57.77 mg g−1, respectively |
136 |
20 |
Carbon derived from corn straw |
Cr(V) |
175.44 mg g−1 |
137 |
21 |
Modified chicken feather |
88.9% |
88.9% |
138 |
22 |
Albizia lebbeck pods |
Pb(II), Cd(II), Zn(II) and Cu(II) ions |
7.17, 7.81, 8.26, and 8.68 mg g−1, respectively |
88 |
23 |
Fe-modified rice straw biochars |
As(V) |
69.6% |
88 |
24 |
Spent tea leaves |
Cr(III) |
95.42% |
139 |
25 |
Bagasse biochar |
Pb(II) |
75.376% |
91 |
26 |
Corn and rice husks |
Pb(II) |
>90% |
140 |
27 |
Rice husk ash |
Pb(II) |
75% |
141 |
28 |
Corn silk |
Cu(II) and Zn(II) |
15.35 and 13.98 mg g−1, respectively |
142 |
29 |
Mung bean husk |
As(V) |
98.75% |
143 |
30 |
Typha angustifolia and Salix matsudana branches |
Cd(II) and Pb(II) |
90% |
144 |
31 |
Mustard waste biomass |
Pb(II), Zn(II), and Cd(II) |
94.56, 96.15 and 76.48%, respectively |
145 |
32 |
Banana peel |
Cd(II) and Pb(II) |
93.2 and 83.78%, respectively |
146 |
33 |
Coco-peat biomass |
Pb(II), Cd(II), Cu(II), and Ni(II) |
0.484, 0.151, 0.383 and, 0.181 mmol g−1, respectively |
147 |
34 |
Coffee residues |
Pb(II) and Zn(II) |
96 and 44%, respectively |
148 |
2.5 Elimination of Pb(II) ions using low-cost adsorbents
Bayuo et al.86 studied the optimization of adsorption factors to eliminate lead(II) from an aqueous medium by groundnut husk. The influences of three factors (contact time, pH, and initial metal Pb(II) concentration) on two responses (removal efficiency and adsorption capacity) were explored by Central Composite Design. The optimal operative conditions for Pb(II) removal were established at 90 min contact time, pH of 8 and 75 mg L−1 Pb(II) initial concentration by a 0.966 desirability. At this operating condition, the optimal percent removal of Pb(II) and adsorption capacities were 90.26% and 3.428 mg g−1 respectively. The equilibrium data agreed with the Langmuir isotherm and pseudo-second-order kinetic model.
Hydroxyapatite nanostructures were synthesized by microwave irradiation from eggshells by Safatian et al.87 This nano adsorbent was applied to eliminate lead(II) from wastewater. The impacts of solution pH, contact time, dosage, Pb(II) initial concentration, and temperature on the elimination of lead(II) were examined revealing Pb(II) depollution dependence on these parameters. Langmuir and Freundlich's models were tried and the results show good fitness to Langmuir isotherm. The synthesized nano adsorbent displays great adsorption abilities for removing lead(II) from water media.
Mustapha et al.88 produced a biosorbent using Albizia lebbeck pods to remove Pb(II), Cd(II), Zn(II), and Cu(II) ions from aqueous media. The influences of many parameters including solution pH, contact time, dosage, initial concentration, and temperature were studied by batch mode. Equilibrium and kinetic experiments were conducted as well as change in enthalpy entropy and free energy was determined. The study confirmed that Albizia lebbeck pods are capable of removing metals from the aqueous environment. The experimental data fitted the Langmuir model with a high correlation coefficient (R2 > 0.94); however, the kinetic data obeyed the pseudo-second-order model. The adsorption thermodynamic variables attested that the biosorption of these metals was endothermic, non-spontaneous, and spontaneous at lower and higher temperatures respectively.
Boulaiche et al.89 reported the use of Posidonia oceanica in removing Pb(II), Cd(II), Ni(II), Cu(II), and Zn(II) using the batch procedure. The influence of pH, contact time, initial metal ion concentration, and the temperature was considered. Sorption kinetics and isotherm models were verified for the biosorption of the metals. The concentrations of the metals adsorbed were found to be 48.33, 43.9, 41.02, 37.90, and 30.22 mg g−1 for Pb(II), Cu(II), Ni(II), Zn(II), and Cd(II), respectively. The biosorption kinetic data conformed to the pseudo-second-order model while the isotherm equilibrium data rightly fitted Langmuir models for all the metals investigated. The biosorption of Pb(II) was endothermic and spontaneous as suggested by the thermodynamic variables evaluated.
A novel natural adsorbent obtained from thorns was utilized by Alatabe and Kariem90 to remove lead(II) from wastewater. The influence of diverse factors (pH, contact time, initial concentration, and different electrolytes) was investigated by batch technique to optimize conditions for which optimal biosorption can be achieved. The experimental data were scrutinized with isotherm and kinetic equations. The study revealed that the adsorbent has a high ability to eliminate Pb(II) and at a pH of 6, optimum biosorption was accomplished. The removal concentration was dependent on the time of equilibrium. At higher temperatures of the isotherm studies, Langmuir model was obeyed. The Pb(II) ions adsorption was influenced by raising electrolyte concentrations. According to the experimental data, the pseudo-second-order model illustrates better applicability indicating chemical sorption. The thermodynamic data point out Pb(II) depollution to be endothermic and spontaneous.
Manjuladevi et al.71 examined Pb(II), Ni(II), Cr(VI), and Cd(II) eradication from aqueous media using Cucumis melo peel-activated carbon (CMAC). The biosorption characteristics of the ions on CMAC were evaluated utilizing kinetic models. The biosorption kinetic data showed a better fit to the pseudo-first-order than the pseudo-second-order model. The optimum removal efficiencies of the ions by the CMAC were 98.78, 98.55, 97.95, and 97.96% for Ni(II), Pb(II), Cr(VI), and Cd(II), respectively at 250 mg dosage of CMAC. The maximum values for the parameters for which optimum removal was achieved at 250 mg dosage, 250 mg L−1 for all metals, pH of 6.0 for Ni(II), Cd(II), and Pb(II), and pH of 3.0 for Cr(VI) using 180 min contact time.
Bagasse is agro-based biomass that was employed by Poonam et al.91 for investigating its possibility in removing lead(II) from effluents of the battery industry. Kinetics and batch experiments comprising several factors (pH, contact time, and dosage) were investigated using biochar produced from the bagasse waste. The maximum removal was attained at a pH of 5, 140 min contact time, and room temperature (25 ± 3 °C) using 5 g biochar. At the optimum pH of 5, the optimum percent removal and the amount of Pb(II) adsorbed were 75.38% and 12.741 mg g−1 correspondingly. The equilibrium data were well represented by Langmuir suggesting monolayer and homogenous biosorption of Pb(II). The pseudo-second-order model indicates conformity to the experimental data implying chemisorption.
Mahdi et al.92 studied lead(II) removal from aqueous media onto biochars obtained from the biomass of date seed. The impacts of solution pH, contact time, and initial Pb(II) concentration were conducted. Aqueous solution pH exhibited an influence on the biosorption capability of the biochar and maximum removal occurred at a pH of 6.0. The equilibrium data were effectively described by both Freundlich and Langmuir isotherms. The experimental data conformed well to the pseudo-second-order kinetic model. The study indicated that date seed-based biochar has high removal potential for Pb(II) in comparison to other plant-derived biochars.
Gaur et al.93 examined the ability of soya bean as a biosorbent in the purification of lead (Pb) and arsenic (As) in wastewater. Batch tests were conducted and the influence of some biosorption parameters (pH, contact time, adsorbent dose, and temperature) was determined. It was found that the maximum elimination of Pb(II) and As(III) was achieved at 37 °C and 3.0 g per 100 mL. At a pH of 2.0 and 4.0 ± 0.26, maximum decontamination of As(III) and Pb(II) were attained respectively at a shaking time of 60 min. Langmuir and Freundlich's models were tested and Langmuir suitably fit equilibrium data. Thermodynamics studies suggest the biosorption of Pb(II) using the soya bean biosorbent was exothermic.
Ferreira et al.94 studied the application of a modified cashew nutshell (Anacardium occidentale) (CNS) in the depollution of Pb(II), Cd(II), and Cr(III) from an aqueous environment. Batch tests were conducted to determine the optimal conditions of adsorption parameters. The cashew nutshell displayed high decontamination of Pb(II), Cd(II), and Cr(III). The study discovered that the maximum biosorption took place at a pH of 5.0, 4 g of the biosorbent, and a contact time of 40 min. The equilibrium data demonstrated a good agreement with Langmuir and Freundlich isotherms suggestive of the biosorption taking place on mono and multilayers.
Klapiszewski et al.95 combined lignin and silica to produce a multifunctional adsorbent with substantial sorption abilities. Batch experiments were conducted using silica/lignin hybrid in eliminating lead(II) from water media. It was found that the efficiency of the biosorption rest on removal time, pH, and adsorbent dosage with a maximum adsorption capacity of 89.02 mg g−1. The experimental data of lead(II) obtained by SiO2/lignin biosorbent show the best fit for Langmuir and pseudo-second-order models.
Ghogomu et al.96 utilized activated carbons produced from Raphia hookeri fruit epicarps using ZnCl2 (CAZn), H3PO4 (CAH), and KOH (CAK) as activation agents to remove lead(II) from wastewater. Batch tests were performed to determine the impacts of contact time, solution pH, dosage, and initial Pb(II) concentration at 27 °C. The results disclosed that optimum removal of Pb(II) occurred at 80 min contact time for CAZn and CAH and 100 min for CAK, pH of 6 for all samples with biosorption capacities of 66.37 mg g−1 for CAZn, 56.30 mg g−1 for CAH and 28.0 mg g−1 for CAK, respectively. The experimental data illustrate a good fit using the Langmuir model with monolayer biosorption capacities of 72.62, 58.62, and 12.16 mg g−1 for CAZn, CAH, and CAK correspondingly. The kinetic investigations have shown that the biosorption system fits the pseudo-second-order model. Raphia hookeri based activated carbons (CAZn and CAH) were revealed to be capable of removing lead(II) from an aqueous environment.
Nnaji and Emefu97 conducted experiments to investigate lead(II) decontamination using different mesh sizes of activated sawdust of two species of timber. The best particle sizes were 0.85 and 1.18 mm for Khaya ivorensis and Pycanthus angolensis, respectively. The equilibrium data were applied to isotherm and kinetic models. The biosorption of lead(II) using Khaya ivorensis and Pycanthus angolensis followed Langmuir and Freundlich models while experimental data conformed to the pseudo-first-order model.
Nordine et al.98 studied lead(II) elimination from synthetic wastewater using natural materials such as pine, beech, and fir sawdust. Several biosorption variables (pH, sawdust dosage, contact time, initial Pb(II) concentration, stirring speed, and temperature) were investigated. The study established that the pine sawdust show higher lead(II) removal (15.5 mg g−1) at pH of 5.45 ± 0.05, 10 g L−1 of pine sawdust, initial Pb(II) concentration of 100 mg L−1, and 23 ± 2 °C temperature. The Langmuir model showed a good representation of the experimental data describing the Pb(II) biosorption mechanism as a monolayer.
Yahaya and Akinlabi99 investigated cocoa pod husk in lead(II) decontamination. The adsorbent was activated chemically by treating it with dilute HNO3 for 24 hours. The adsorbent was modified with 7% (v/v) sulphuric acid (H2SO4) for 90 minutes. An additional modification of the adsorbent was done using 0.3 dm3 of pyridine and 5.67 g EDTA under reflux for 24 hours. At the maximum time of 50 min, the removal percentages of lead were 94.6% and 98.2%, respectively for the original and improved cocoa pod husk. The best-fitted model was pseudo-second-order with a high correlation coefficient ranging from 0.9776 to 1.0000. From the thermodynamic studies, the adsorption process was feasible, increase in randomness and endothermic.
Activated carbon produced using sugarcane bagasse (SCBA) and its possibility to adsorb lead(II) from synthetic water was studied by Kane et al.100 Batch mode trials were conducted to probe the impacts of pH and dosage of SCBA on the biosorption of Pb(II). The batch results demonstrated Pb(II) biosorption dependence on the pH and SCBA dosage. The optimum depollution of Pb(II) was obtained at pH 5.0 and 10 g L−1 SCBA dose with a removal efficiency of 87.3%. The isotherm data indicated good agreement with the Langmuir model and the maximal biosorption capacity determined from the model was 23.4 mg g−1.
Khandanlou et al.5 prepared nanocomposites (RS/Fe3O4-NCs) using rice straw to eliminate Pb(II) and Cu(II) from water. The influences of three parameters, removal time, adsorbent dose, and initial ion concentration were examined on Pb(II) and Cu(II) removal possibilities. The optimum removal conditions of Pb(II) and Cu(II) were found at removal times of 41.96 and 59.35 min; initial ion concentrations of 100 and 60 mg L−1 and 0.13 g of the biosorbent for both ions, respectively. The optimum percent removal of Pb(II) and Cu(II) was attained at 96.25% and 75.54%, respectively. The isotherm and kinetic data obeyed the Langmuir isotherm and pseudo-second-order models for both ions.
Shi et al.101 investigated heavy metals [Pb(II), Co(II), and Cu(II)] elimination in water media using arborvitae leaves. Single and competitive component absorption experiments were performed and the adsorption capability of the leaf was found to be dependent on the pore diameter and surface. The optimal uptake capacities of Pb(II), Co(II), and Cu(II) at an equilibrium time of 120 min were 7.64, 1.84, and 3.38 mg g−1, respectively. The single-component adsorption showed that the decontamination of the ions depended on increasing order of electronegativity, Pb (2.33) > Cu (1.90) > Co (1.88). Conversely, the ternary component biosorption process revealed that the uptake of Pb(II), Co(II), and Cu(II) ions onto the biosorbent declined from 35.84 to 9.32 mg g−1, 6.78 to 1.54 mg g−1, and 7.94 to 3.07 mg g−1, respectively. At pH 5.5, maximum decontamination of the ions was achieved and the equilibrium data correlated well with the Langmuir model.
Paliulis102 conducted a study using peat to remove Pb(II) from aqueous solutions. The study revealed an increase in the uptake capability of peat with the rising contact time, initial concentration of Pb(II) and was remarkably affected at a pH range of 4.0–7.0. At pH of 6.0, a contact time of 360 min and Pb(II) initial concentration of 100 mg L−1, maximum Pb(II) biosorption (9.489 mg g−1) was achieved by the peat. The Langmuir and Freundlich isotherms were employed in the analysis of the biosorption properties of Pb(II) with Freundlich being the best fit model.
Misihairabgwi et al.103 studied heavy metals decontamination from water media utilizing activated carbon obtained from agroforestry wastes including rice husk, baobab shells, pigeon pea husk, macadamia nut shells, marula stones, and Moringa oleifera husks. The uptake capacity was increased when the pH was raised from 4.0 to 6.0 but at a pH of 6.0, 60% uptake of Hg(II) was achieved for all the activated carbons. The elimination of Pb(II) was observed to increase by 22% in removal efficiency in comparison to activated carbons of Moringa oleifera husks, pigeon pea husk, baobab shells, and marula stones. The well-correlated isotherm was the Langmuir model and the adsorbents with the best adsorption capacity were baobab shells and pigeon pea husk as compared to the others.
A chemically modified adsorbent derived from okra was explored in removing toxic metals [Pb(II), Cd(II), Cu(II), and Zn(II)] from synthetic wastewater.104 Batch technique tests were used to determine the impact of removal time, temperature, pH, and metal particle fixation. The experimental data conformed to Dubinin–Radushkevich and Langmuir isotherms. The values of ΔH0 and ΔG0 obtained from the thermodynamic study showed that the metals uptake on the chemically modified cellulosic biopolymer prepared from okro is unrestricted and exothermic.
Ogunleye et al.105 explored banana stalk usage in the decontamination of Pb(II) ions from wastewater. Phosphoric acid (H3PO4) was used to modify the adsorbent at 1
:
1 (w/v). Besides, the mixture was dehydrated at 105 °C for 12 hours and further at 800 °C without air for 2 hours leading to the occurrence of carbonization. A 0.1 M HCl was applied to treat the carbonized biosorbent for 1 h. At 120 min equilibrium time, the optimum percentage removal was 97.9% however; at a pH of 8.0 and 250 mg L−1 Pb(II) initial concentration, the optimum capacity attained was 200 mg g−1. The best kinetic model applied in analyzing the kinetic data was pseudo-second-order while the isotherm model that depicted good agreement to the equilibrium data was the Langmuir model.
Hikmat et al.106 examined Pb(II) biosorption by absorbents obtained from agro-based materials including petiole and palm tree fiber. The uptake of Pb(II) unto the bio-sorbents exhibited dependence on shaking time, pH, biosorbent weight, and Pb(II) initial concentration. The isotherm data best fitted Langmuir than that of the Freundlich with a high correlation coefficient of 0.9801 and 0.9974 for fiber and petiole correspondingly. The kinetic data indicated that the pseudo-first-order fitted well with high coefficients of determination for fiber (0.9773) and petiole (0.9720), respectively.
The potential of natural bentonite and activated carbon for Pb(II) depollution from water media was studied by Yarkandi.107 The study revealed that the amount of Pb(II) removed upsurges with pH, contact time, and initial concentration but declines with adsorbent dose and temperature. The pseudo-second-order was the best fitted kinetic model while both Langmuir and Freundlich isotherms show good conformity to equilibrium data. Adsorption thermodynamic parameters suggested endothermic, spontaneous, and increase in randomness. The study concluded that bentonite is more efficient in removing Pb(II) than activated carbon.
Vieira et al.108 conducted kinetic studies on Pb(II) and Cu(II) ions utilizing rice husk ash (RHA) in a fixed column bed. The study has shown that RHA could be applied as a cost-effective biosorbent for eliminating Pb(II) and Cu(II) ions from effluents. The optimal removal capacities attained for Pb(II) and Cu(II) were 0.0561 and 0.0682 mmol g−1 respectively at a temperature of 20 °C. Biosorption isotherm data were well-represented using Langmuir isotherm. The thermodynamic parameters evaluated showed exothermic and spontaneous adsorption of Pb(II) whereas the adsorption system of Cu(II) was endothermic and spontaneous.
Chandrasekaran and Jeyakumar4 prepared activated carbons using green Ulva fasciata sp. to decontaminate Pb(II) ions from the marine. The impact in the variation of parameters such as pH, contact time, Pb(II) concentration, and biosorbent dose was investigated. Isotherm and kinetic models have been employed and the study indicated that the Langmuir isotherm and pseudo-second-order as the best fit biosorption models.
The depollution of Pb(II) from the water was investigated in an acidic medium (pH = 4) by Kouakou et al.109 using commercial activated carbons produced from wood and coconut shells. The study disclosed that the powdered activated carbon obtained from the wood showed a remarkably high uptake capability than the activated carbon of coconut shells. The powdered and granular activated carbons exhibited diverse sorption abilities. At 25 °C, the maximum Pb(II) adsorbed on powdered, 830-granular, and 123-granular activated carbons were 44.58, 38.96, and 39.06 mg g−1, respectively. Langmuir's model showed a good representation of the isotherm data.
Hikmat et al.106 examined Pb(II) elimination from the aqueous environment using leaf and fiber of a palm tree. The biosorption factors indicated that the removal time for Pb(II) was a bit fast and equilibrium was accomplished at approximately 30 and 40 min by the leaf and fiber, respectively. At a pH range of 6.5–7.0, optimal Pb(II) adsorption was attained using 0.5 g of the adsorbent. However, at high temperatures, Pb(II) removal by the adsorbents declined to signify exothermic biosorption. The isotherm and kinetic data of Pb(II) obtained using the two biosorbents conformed to Langmuir and pseudo-first-order than other models. It was found that the adsorption system was exothermic, feasible, and spontaneous.
Ghasemi and Gholami110 studied Pb(II) elimination from synthetic water making use of Myrtaceae sawdust. The influence of pH, contact time, dosage, and initial Pb(II) concentration was investigated using the batch technique. At a pH of 7.0, with the contact time of 30 min and 10 g L−1 dosage, the maximal removal efficiency of Pb(II) was 97.49%. The biosorption of Pb(II) upsurges with increasing initial concentration. The equilibrium data demonstrated good correspondence with the two models, Langmuir and Freundlich. The kinetic data analyzed show conformity with the second-degree kinetic equation.
Mokaddem et al.111 reported Pb(II) sorption from aqueous media using agar beads. The impact of solution pH, biomass dosage, contact time, and initial lead(II) concentration was examined. The study recommended agar beads for the decontamination of Pb(II) from aqueous solutions. The experimental results have shown that biosorption equilibrium was reached below 120 min at a pH of 5.0. The Freundlich isotherm was found to show best fit the isotherm data.
Haloi and Chakravarty112 conducted a study to examine the ability of heartwood charcoal of Areca catechu (HCAC) in depolluting Pb(II) ions from water. Batch tests were piloted to find out the biosorption characteristics at varied pH, contact times, adsorbent doses, and initial concentrations. At an equilibration time of 25 min, optimal biosorption of Pb(II) was attained at a pH of 5.0 and 0.5 g biomass dosage. The experimental data was found to be well-fitted to the Freundlich isotherm model implying that the HCAC surface was heterogeneous while the kinetic data was better described by the pseudo-second-order model.
Athar et al.113 considered biomass obtained from Trifolium resupinatum in removing Pb(II). The influences of several factors for example removal time, initial Pb(II) concentration and pH have been explored. The study discovered that 25 min and 3.0 as optimum removal time and pH, respectively. Also, increasing the biomass dosage increased the binding aptitude of the biosorbent. The equilibrium data were analyzed with isotherm and kinetic models for which, the Langmuir and pseudo-second-order models showed the best representation of the data.
Maize husk was utilized by Adeogun et al.114 for efficient removal of Pb(II) and Mn(II) using a batch system. The study has shown that the elimination of Pb(II) and Mn(II) depended on pH, biomass dose, initial concentration, and temperature, respectively. The equilibrium data showed a good fit to the Langmuir isotherm with 7.38 and 8.52 mg g−1 maximum sorption capacities for Pb(II) and Mn(II) correspondingly on the raw biomass. However, maximum sorption capacities obtained by acid-modified biomass were 9.33 and 9.00 mg g−1 for Pb(II) and Mn(II), respectively. The adsorption thermodynamics revealed that Pb(II) and Mn(II) biosorption onto maize husk is feasible, spontaneous, and exothermic.
Azouaou et al.115 examined Pb(II) removal from synthetic by using untreated orange barks. The impacts of initial pH, dosage, removal time, and initial Pb(II) concentration were considered. It was advocated that the orange barks are efficient and economical biosorbent in eliminating Pb(II). The study indicated that sorption equilibrium was realized in <30 min. The analysis of the equilibrium data suggested that the Langmuir isotherm correlated best.
Mitic-Stojanovic et al.116 studied Pb(II), Cd(II), and Zn(II) ions decontamination from water using Lagenaria vulgaris shell. The study employed batch experiments and the impacts of contact time, initial pH, temperature, and stirring speed were investigated. The biosorption of the metals ions considered was rapid, attaining equilibrium after around 5 to 10 min. The biosorption showed dependence on pH with optimal pH ranging at 4.5–6.0. The influence of temperature established that the biosorption of the metal is a chemical process.
Qaiser et al.117 utilized groundnut hulls in the decontamination of Pb(II) from wastewater. Batch experiments were piloted to determine the influence of biosorption factors including pH, initial Pb(II) concentration, and temperature. At an optimal pH 5 ± 0.1 and 20 ± 2 °C temperature, the optimum Pb(II) biosorption was 31.54 ± 0.63 mg g−1. The equilibrium data was better described by the Langmuir model with a coefficient of determination >0.98. The kinetics of biosorption followed the pseudo-second-order kinetic model.
Bhattacharyya and Sharma118 conducted a study to remove Pb(II) ion from water media by well-developed Azadirachta indica leaves. The biosorbent gave a maximum removal of 93% with a 300 min contact time. The pseudo-second-order model was the well-fitted than the pseudo-first-order model while the two isotherms (Langmuir and Freundlich) agreed better to the experimental data with a coefficient of determination of 0.99.
Abdulkarim and Al-Rub119 produced activated carbon and chemically modified activated carbon from date pits to remove Pb(II) from aqueous media. The variation of different factors was considered. The study indicated that both carbons exhibited high Pb(II) uptake. Lead(II) biosorption was found to rise by increasing pH and initial Pb(II) concentration until at pH of 5.2 where maximum elimination of Pb(II) was attained. The Langmuir and Freundlich isotherm models obeyed the experimental data using both biosorbents while the kinetic data followed the pseudo-second-order equation.
Wong et al.120 examined lead (Pb) elimination using batch experiments by tartaric acid modified rice husk. The adsorption system showed dependence on pH and demonstrated characteristics of exothermal adsorption. The pseudo-second-order model showed a good relationship with the equilibrium data than that of the pseudo-first-order model.
The adsorption potential of some agricultural adsorbents in removing Pb(II) ions from an aqueous solution is summarized in Table 3.
Table 3 Adsorption potential of some agricultural bioadsorbents for Pb(II) ions removal from aqueous solution
Adsorbent |
Modifying agent |
Experimental variable considered |
Constants |
Suited equilibrium model analysis (EMA) |
EMA, R2 values |
Kinetics model analysis (KMA) |
KMA, R2 value |
Optimum conditions |
% Removal or Qmax |
References |
Wood biomass |
— |
Concentration (C), contact time (CT), dosage (D) |
Agitation speed, pH, temperature |
Langmuir |
0.986 |
Pseudo-second-order |
0.9988 |
C = 100 mg L−1, CT = 4 h, D = 0.5 g L−1 |
100% |
149 |
Walnut and almond shells |
— |
Contact time (CT), dosage (D), pH |
Agitation speed, concentration, temperature |
Langmuir |
0.9952 and 0.9884, respectively |
Pseudo-second-order |
0.9902 and 0.9879, respectively |
CT = 240 min, D = 7 mg L−1, pH = 6 |
— |
150 |
Cotton shells |
— |
Contact time (CT), concentration (C), dosage (D) |
pH, temperature |
Freundlich |
0.99 |
— |
— |
CT = 90 min, C = 1 mg L−1, D = 1 g L−1 |
90% |
151 |
Pomelo leaves |
— |
Contact time (CT), pH |
Temperature, concentration dosage |
Sips |
0.9769 |
Pseudo-second-order |
0.9982 |
CT = 150 min, pH = 4 |
207.2 mg g−1 |
152 |
Fish scale and bean husk |
— |
Contact time (CT), pH, dosage (D), concentration (C), temperature (T) |
Agitation speed |
Temkin and Langmuir, respectively |
0.5178 and 0.8978, respectively |
Pseudo-second-order |
1.000 and 0.9999, respectively |
CT = 30 min, pH = 7, D = 0.2 and 6 g, respectively, C = 60 and 80 mg L−1, respectively and T = 321 and 318 K, respectively |
90% |
153 |
Coconut waste |
HCl |
pH, agitation time (AT), concentration (C) |
Agitation speed dosage, temperature |
Langmuir |
0.969 |
Pseudo-second-order |
0.999 |
pH = 6, T = 1440 min, C = 125 mg L−1 |
50.33 mg g−1 |
154 |
Winemaking waste |
— |
Stirring rate (SR), temperature (T), pH, dosage (D) |
Contact time, temperature |
Langmuir |
0.9989 |
Pseudo-second-order |
0.9999 |
SR = 500–750 rpm, T = 288 K, pH = 4, D = 0.25 g L−1 |
58 mg g−1 |
155 |
Lobeira fruit |
— |
pH, dosage (D), contact time (CT) |
Agitation speed, concentration, temperature |
Langmuir |
0.9611 |
— |
— |
pH = 2, D = 250 mg, CT = 60 min |
93% |
156 |
Eggplant peel |
— |
Contact time (CT), pH, dosage (D) concentration (C), and temperature (T) |
Agitation speed |
Langmuir |
0.9996 |
Pseudo-second-order |
0.9998 |
CT = 60 min, pH = 4, C = 70 ppm, D = 0.01 g mL−1, T = 25 °C |
88.33 mg g−1 |
157 |
Shrimp shells chitosan |
NaOH |
Contact time (CT), pH |
Temperature, concentration dosage |
— |
— |
— |
— |
CT = 90 min, pH = 4 |
99.88% |
158 |
Sugarcane bagasse |
— |
Concentration (C), dosage (D), contact time (CT) |
Agitation speed, pH, temperature |
Freundlich |
0.999 |
— |
— |
C = 7 ppm, D = 1 g, CT = 15 min |
90–98% |
159 |
Coffee husk |
— |
Dosage (D), contact time (CT), and concentration (C) |
Agitation speed, pH, temperature |
Freundlich |
0.9800 |
Pseudo-second-order |
1.000 |
D = 2 g L−1, CT = 60, C = 400 mg L−1 |
98% |
160 |
Cucumber peel |
— |
pH, contact time (CT), and temperature (T) |
Agitation speed, dosage, concentration |
Langmuir |
0.999 |
Pseudo-second-order |
1.000 |
pH = 5, CT = 60 min, T = 20–35 °C |
90% |
161 |
Black walnut husk |
— |
pH, contact time (CT), concentration (C), dosage, and temperature (T) |
Agitation speed |
Freundlich |
0.964 |
Pseudo-second-order |
0.999 |
pH = 4, CT = 60 min, D = 0.5 g L−1, T = 308–343 K |
96% |
162 |
Sugarcane bagasse derived activated carbon |
H2SO4 |
pH, contact time (CT), concentration (C) |
Agitation speed, temperature, dosage |
Langmuir |
0.9508 |
Pseudo-second-order |
0.9942 |
pH = 5, CT = 180 min, C = 50 mg L−1 |
23.4 mg g−1 |
163 |
Spirulina platensis biomass |
— |
pH, dosage (D), temperature (T), concentration (C), and contact time (CT) |
Agitation speed |
Freundlich |
0.9700 |
— |
— |
pH = 3, D = 2 g L−1, T = 26 °C, C = 100 mg L−1, CT = 60 min |
>91% |
164 |
Pineapple waste |
NaOH |
pH, contact time (CT), temperature (T) |
Agitation speed, dosage, concentration |
— |
— |
— |
— |
pH = 4, CT = 30 min, T = 60 °C |
>95% |
165 |
2.6 Adsorption kinetic studies
Adsorption kinetic modeling is conducted to determine the rate of biosorption and expressions for a certain reaction. Adsorption kinetics are significant in the treatment of wastewater as it provides valued information on the reaction pathways and in the mechanism of the adsorption process.114 The adsorption kinetics has key parameters for describing the basic properties of a good adsorbent. It offers cherished information about the system and regulates the adsorbate reactions in the solid–solute interface at different times.1,91 Many kinetic models had been applied in the elimination of pollutants in water and wastewater including pseudo-first-order, pseudo-second-order, Elovich, and intraparticle diffusion models.
For the present review, the linearized adsorption kinetic models commonly utilized in the elimination of Pb(II) from water and wastewater are presented in Table 4.
Table 4 Linearized adsorption kinetics model equations
Model |
Linear form |
Plot |
References |
Pseudo-first-order |
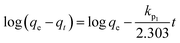 |
log(qe − qt) vs. t |
2, 3 and 166 |
Pseudo-second order |
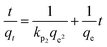 |
 |
2, 76 and 167 |
Elovich |
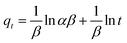 |
qt vs. ln t |
89, 168 and 169 |
Intra-particle diffusion |
qt = kidt1/2 + C |
qt vs. t1/2 |
4, 168 and 170 |
2.7 Adsorption isotherm studies
Adsorption isotherms are investigated to describe the adsorption system. Adsorption isotherm models signify the link between the concentration of adsorbate adsorbed on the biosorbent and the concentration of adsorbate retained in the adsorption medium.86,113,121
Many isotherm models are frequently used to fit the adsorption equilibrium data to get a linear regression for the prediction of the maximal sorption capacity of the employed adsorbent.91 In most of the numerous studies reviewed, the adsorption processes were justified by applying linear forms of one-parameter adsorption isotherm model (Henry), two-parameter adsorption isotherm models (Langmuir, Freundlich, Dubinin–Radushkevich, Temkin, Harkin–Jura, and Elovich), and three-parameter adsorption isotherm models (Redlich–Peterson and Jossens) as summarized in Table 5.
Table 5 Linearized adsorption isotherm model equations
Isotherm |
Linear form |
Plot |
Significance |
References |
Henry |
qe = KHECe |
qe = KHECe |
For very low concentrations |
2 and 86 |
Langmuir |
(I) 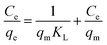 |
 |
For monolayer adsorption on homogeneous surfaces |
2, 86, 121 and 171 |
(II) 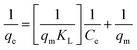 |
 |
(III) 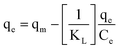 |
qe vs. Ce |
(IV) 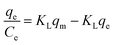 |
 |
Freundlich |
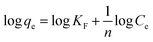 |
log qe vs. log Ce |
For dilute solutions, over a small concentration range |
2, 86, 121 and 172 |
Dubinin–Radushkevich (D–R) |
ln qe = ln qs − KDε2 |
ln qe vs. ε2 |
Describes adsorption with a Gaussian energy distribution onto a heterogeneous surface |
2, 86 and 173 |
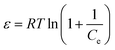 |
Temkin |
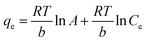 |
qe vs. ln(Ce) |
Based on the adsorbent–adsorbate interactions |
2, 86 and 174 |
or qe = B ln A + B ln Ce |
Where  |
Harkin–Jura |
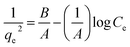 |
 |
For multilayer adsorption on the absorbent surface with heterogeneous pore distribution |
86 and 175 |
Elovich |
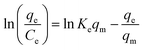 |
 |
Based on chemical adsorption kinetics of adsorbate unto biomaterials |
86 and 176 |
Redlich–Peterson (R–P) |
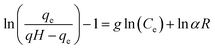 |
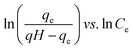 |
It can be applied either in homogeneous or heterogeneous systems |
2, 86, 177 and 178 |
Jossens |
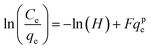 |
 |
For energy dissemination of solute–solid interactions |
86, 177 and 179 |
3. Conclusions
The review of the various studies on Pb(II) biosorption indicates that low-cost biosorbents are eco-friendly, cost-effective, and simple techniques for water and wastewater treatment containing lead(II) ions. Pb(II) biosorption with these low-cost biosorbents was found to show dependence on several adsorption factors such as pH, contact time, adsorbent dose, initial Pb(II) concentration, and temperature. Furthermore, the efficiency of the biosorption procedures was subjected to physicochemical characteristics of the biomass used as the biosorbent. In most of the studies, batch adsorption data have been modeled by determining the kinetic, isothermal, and thermodynamic parameters to elucidate the mechanism of the adsorption system. Most of the studies on the adsorptive removal of Pb(II) were found to follow the pseudo-second kinetic and Langmuir isotherm models with the thermodynamics variables suggesting the feasibility and spontaneous nature of Pb(II) sequestration. However, gaps exist to increase biosorption ability, economic feasibility, optimization of the biosorption system, and desorption and regeneration of the used agricultural biosorbents.
4. Future perspectives
Various laboratory-scale investigations have demonstrated that the capability of unconventional biosorbents in terms of effectiveness for the removal of Pb(II) from aqueous systems is extremely high. However, because of the lack of confidence in the engineering of these materials and practicality, they are not utilized on an industrial scale. Therefore, it is critical to reconsider multidimensional and multidisciplinary research approaches in biosorbent production for commercial and industrial applications in the near future to minimize metal burdens in aquatic systems.
Another consideration is that industrial effluents do not contain a single contaminant, but rather a large mixture of heterogeneous contaminants that may have a larger influence on adsorption systems and selectivity. As a result, significant measures should be taken to develop and apply novel solid materials, such as biomass, synthetic nanomaterials, and chitosan as low-cost biosorbents, for the decontamination of various toxic heavy metals from water systems. Therefore, deeper studies into the adsorption mechanisms are required, with a particular emphasis on the simultaneous detoxification of multiple heavy metals ions using cheap and single adsorbents. Besides, future research should look into different combinations of agro-industrial wastes and by-products that can effectively remove heavy metal ions from aqueous media.
The literature review also uncovered a current trend in this area of study, with a significant shift toward adopting more sustainable techniques and new technologies that prioritize sustainability over relying solely on the efficiency and performance of the technology in treating wastewater containing heavy metals ions. Finding the right balance among heavy metals decontamination and recovery would be one of those sustainability issues. As a result of this development, the attention and motivation have shifted away from simple wastewater treatment to the retrieval and recycling of heavy metals as well as the spent biosorbents for further use. Therefore, to avoid further environmental pollution, other feasible alternatives and dependable procedures should be employed for heavy metals recovery, regeneration of exhausted biosorbents, and safe disposal of spent biosorbents.
Author contributions
Jonas Bayuo: conceptualization, investigation and writing – original draft. Mwemezi Rwiza: reviewing, editing, and supervision. Kelvin Mtei: editing and supervision.
Conflicts of interest
The authors declare that there is no conflict of interest.
Acknowledgements
The authors are grateful for the support of the Partnership for Applied Sciences, Engineering, and Technology (PASET), which enabled them to complete this study.
References
- J. Bayuo, M. A. Abukari and K. B. Pelig-Ba, Optimization using central composite design (CCD) of response surface methodology (RSM) for biosorption of hexavalent chromium from aqueous media, Appl. Water Sci., 2020, 10(6), 1–12, DOI:10.1007/s13201-020-01213-3
. - A. Kaushal, Adsorption Phenomenon and its Application in Removal of Lead from Waste Water: A Review, Int. J. Hydrol., 2017, 1(2), 38–47 CrossRef
. - J. Bayuo, K. B. Pelig-ba and M. A. Abukari, Optimization of adsorption parameters for effective removal of lead(II) from aqueous solution, Phys. Chem.: Indian J., 2019, 14(1), 1–25 Search PubMed
. - R. P. Jeyakumar and V. Chandrasekaran, Adsorption of lead(II) ions by activated carbons prepared from marine green algae: equilibrium and kinetics studies, Int. J. Ind. Chem., 2014, 5(1), 1–9 CrossRef
. - R. Khandanlou, M. B. Ahmad, H. R. F. Masoumi, K. Shameli, M. Basri and K. Kalantari, Rapid adsorption of copper(II) and lead(II) by rice straw/Fe3O4 nanocomposite: optimization, equilibrium isotherms, and adsorption kinetics study, PLoS One, 2015, 10(3), 1–19 CrossRef PubMed
. - L. Han, L. Qian, R. Liu, M. Chen, J. Yan and Q. Hu, Lead adsorption by biochar under the elevated competition of cadmium and aluminum, Sci. Rep., 2017, 7(1), 1–11 CrossRef PubMed
. - I. Mohd, I. Ahamed and E. Lichtfouse, Water Pollution and Remediation: Heavy Metals, Environmental Chemistry for a Sustainable World, 2021, vol. 53, pp. 1–581 http://link.springer.com/10.1007/978-3-030-54723-3 Search PubMed
. - A. Abidli, Y. Huang, Z. Ben Rejeb, A. Zaoui and C. B. Park, Sustainable and efficient technologies for removal and recovery of toxic and valuable metals from wastewater: recent progress, challenges, and future perspectives, Chemosphere, 2021, 292, 133102, DOI:10.1016/j.chemosphere.2021.133102
. - E. A. Obodai, Concentrations of heavy metals in two Ghanaian Lagoons, Arch. Appl. Sci. Res., 2011, 3(3), 177–187 CAS
. http://scholarsresearchlibrary.com/aasr-vol3-iss3/AASR-2011-3-3-177-187.pdf. - A. Ene, A. Boşneagă and L. Georgescu, Determination of heavy metals in soils using XRF technique, Rom. Rep. Phys., 2010, 55(7–8), 815–820 CAS
. - P. J. Agya, Levels of Heavy Metals in Gubi Dam Water Bauchi, Nigeria, Global J. Environ. Sci., 2009, 8(2), 29–37 Search PubMed
. - F. Mireles, J. I. Davila, J. L. Pinedo, E. Reyes, R. J. Speakman and M. D. Glascock, Assessing urban soil pollution in the cities of Zacatecas and Guadalupe, Mexico by instrumental neutron activation analysis, Microchem. J., 2012, 103, 158–164 CrossRef CAS
, http://www.sciencedirect.com/science-/article/pii/S0026265X12000434. - R. A. Wuana and F. E. Okieimen, Heavy Metals in Contaminated Soil, ISRN Ecol., 2011, 2011, 1–20 CrossRef
, http://www.hindawi.com/journals/isrn/2011/402647/. - B. Adelekan and K. Abegunde, Heavy metals contamination of soil and groundwater at automobile mechanic villages in Ibadan, Nigeria, Int. J. Phys. Sci., 2011, 6(5), 1045–1058 CAS
. http://www.academicjournals.org/journal-/IJPS/article-full-text-pdf/E2C345627083. - S. Onder, S. Dursun, S. Gezgin and A. Demirbas, Determination of heavy metal pollution in grass and soil of City Centre Green areas (Konya, Turkey), Pol. J. Environ. Stud., 2007, 16(1), 145–154 CAS
. - I. Suciu, C. Cosma, M. Todica, S. D. Bolboaca and L. Jantschi, Analysis of soil heavy metal pollution and pattern in Central Transylvania, Int. J. Mol. Sci., 2008, 9(4), 434–453 CrossRef CAS
. - V. Rajaganapathy, F. Xavier, D. Sreekumar and P. K. Mandal, Heavy metal contamination in soil, water and fodder and their presence in livestock and products: a review, J. Environ. Sci. Technol., 2011, 4(3), 234–249 CrossRef CAS
. - P. S. Kumar, S. Ramalingam, R. Vijayakumar, S. D. Kirupha, A. Murugesan and S. Sivanesan, et al., Adsorption of metal ions onto the chemically modified agricultural waste, Clean: Soil, Air, Water, 2012, 40(2), 188–197 CAS
. - O. J. Aderinola, E. O. Clarke, O. M. Olarinmoye, V. Kusemiju and M. A. Anatekhai, Heavy Metals in Surface Water, Sediments, Fish and Perwinklesof Lagos Lagoon, Environ. Sci., 2009, 5(5), 609–617 CAS
. - M. M. El Bouraie, A. A. El Barbary, M. M. Yehia and E. A. Motawea, Heavy metal concentrations in surface river water and bed sediments at Nile Delta in Egypt, Suo, 2010, 61(1), 1–12 Search PubMed
. - L. Jarup, Hazards of Heavy Metals Contamination, Br. Med. Bull., 2003, 68, 167–182 CrossRef PubMed
. - P. C. Emenike, D. O. Omole, B. U. Ngene and I. T. Tenebe, Potentiality of agricultural adsorbent for the sequestering of metal ions from wastewater, Global J. Environ. Sci. Manage., 2016, 2(4), 411–442 CAS
. - K. Begum, K. M. Mohiuddin, H. M. Zakir, M. M. Rahman and M. Nazmul, Heavy Metal Pollution and Major Nutrient Elements Assessment in the Soils of Bogra City in Bangladesh, Can. Chem. Trans., 2014, 2(3), 316–326 Search PubMed
. available from: ISSN 2291-6466. - B. Wei and L. Yang, A review of heavy metal contaminations in urban soils, urban road dusts and agricultural soils from China, Microchem. J., 2010, 94(2), 99–107, DOI:10.1016/j.microc.2009.09.014
. - B. Adelekan, K. Abegunde, B. A. Adelekan and K. D. Abegunde, Heavy Metals Contamination of Soil and Groundwater at Automobile Mechanic Villages in Ibadan, Nigeria, Int. J. Phys. Sci., 2011, 6(5), 1045–1058 CAS
. http://www.academic-journals.org/journal/IJPS/article-full-text-pdf/E2C345627083. - E. T. Puttaih, Heavy Metal Contamination In Soil Under The Application Of Polluted Sewage Water Across Vrishabhavathi River, Int. J. Eng. Res. Appl., 2012, 2(6), 1666–1671 Search PubMed
. https://www.ijera.com. - W. J. Ntow, Organochlorine pesticides in water, sediment, crops, and human fluids in a farming community in Ghana, Arch. Environ. Contam. Toxicol., 2001, 40, 557–563 CrossRef CAS PubMed
. - B. Dinham, Growing vegetables in developing countries for local urban populations and export markets: problems confronting small-scale producers, Pest Manage. Sci., 2003, 59(5), 575–582 CrossRef CAS PubMed
. - F. O. Abulude and H. Adebusoye, Characterization of heavy metal pollutant around cassava processing factory using atomic absorption spectrophotometer, Res. J. Appl. Sci., 2006, 1(1–4), 16–18 CAS
. - S. Babel and T. T. A. Kurniawan, Cr(VI) removal from synthetic wastewater using coconut shell charcoal and commercial activated carbon modified with oxidizing agents and/or chitosan, Chemosphere, 2004, 54(7), 951–967 CrossRef CAS PubMed
. - A. Güne, M. Alpaslan and A. Inal, Plant Growth and Fertilizer, Ankara University of Agriculture publication, 2004, vol. 2000 Search PubMed
. - E. A. Agyapong, M. Fuseini and B. IFei-baffoe, Removal of Arsenic and Lead from Aqueous Solutions by Palm Kernel Cake and Shea Nut Cake Adsorbents, Int. J. Sci. Technol., 2015, 4(3), 150–155 Search PubMed
. - E. M. Alissa and G. A. Iferns, Heavy metal poisoning and cardiovascular disease, J. Toxicol., 2011, 2011, 1–21 CrossRef PubMed
. - B. Sharma, S. Singh and N. J. ISiddiqi, Biomedical implications of heavy metals induced imbalances in redox systems, BioMed Res. Int., 2014, 2014, 1–26 Search PubMed
. - P. B. Tchounwou, C. G. Yedjou, A. K. Patlolla and D. J. ISutton, Heavy metals toxicity and the environment, Mol. Clin. Environ. Toxicol., 2012, 101, 133–164 Search PubMed
. http://www.scopus.com/inward/record.url?eid=2-s2.0-77950880787%26partnerID=tZOtx3y1. - A. Yahaya, A. A. Adegbe and J. E. IEmurotu, Assessment of heavy metal content in the surface water of Oke-Afa Canal Isolo Lagos, Nigeria, Arch. Appl. Sci. Res., 2012, 4(6), 2322–2326 CAS
. - H. Lalhruaitluanga, K. Jayaram, M. N. V. Prasad and K. K. IKumar, Lead(II) adsorption from aqueous solutions by raw and activated charcoals of Melocanna baccifera Roxburgh (bamboo)-A comparative study, J. Hazard. Mater., 2010, 175, 311–318 CrossRef CAS PubMed
. - D. Yildiz, I. Kula, G. AY, S. Baslar and Y. IDogan, Determination of Trace Elements in the Plants of Mt. Bozdag, Izmir, Turkey, Arch. Biol. Sci., 2010, 62(3), 731–738 CrossRef
. - Lenntech, Water treatment solutions, Int. Paperworld, 2005, 28 Search PubMed
. http://www.lenntech.com/. - S. Perumal, R. Atchudan, T. N. J. Immanuel Edison, R. S. Babu, P. Karpagavinayagam and C. Vedhi, A short review on recent advances of hydrogel-based adsorbents for heavy metal ions, Metals, 2021, 11(6), 1–13 CrossRef
. - G. Crini, E. Lichtfouse, L. D. Wilson and N. Morin-Crini, Conventional and non-conventional adsorbents for wastewater treatment, Environ. Chem. Lett., 2019, 17(1), 195–213, DOI:10.1007/s10311-018-0786-8
. - Y. Li, J. He, K. Zhang, T. Liu, Y. Hu and X. Chen, et al., Super rapid removal of copper, cadmium and lead ions from water by NTA-silica gel, RSC Adv., 2019, 9(1), 397–407 RSC
. - Y. Li, X. Zhu, X. Qi, B. Shu, X. Zhang and K. Li, et al., Removal and immobilization of arsenic from copper smelting wastewater using copper slag by in situ encapsulation with silica gel, Chem. Eng. J., 2020, 394, 124833, DOI:10.1016/j.cej.2020.124833
. - M. M. El-Moselhy, A. Ates and A. Çelebi, Synthesis and characterization of hybrid iron oxide silicates for selective removal of arsenic oxyanions from contaminated water, J. Colloid Interface Sci., 2017, 488, 335–347 CrossRef CAS PubMed
. - W. Zhu, J. Wang, D. Wu, X. Li, Y. Luo and C. Han, et al., Investigating the Heavy Metal Adsorption of Mesoporous Silica Materials Prepared by Microwave Synthesis, Nanoscale Res. Lett., 2017, 12(1), 1–19 CrossRef PubMed
. - H. He, Q. Gan and C. Feng, Preparation and application of Ni(II) ion-imprinted silica gel polymer for selective separation of Ni(II) from aqueous solution, RSC Adv., 2017, 7(25), 15102–15111 RSC
. - E. Chmielewská, Chapter 4-natural zeolite: alternative adsorbent in purification or post-treatment of waters, in Modified Clay and Zeolite Nanocomposite Materials: Environmental and Pharmaceutical Applications, Elsevier, Amsterdam, The Netherlands, 2019, pp. 87–112 Search PubMed
. - C. G. Flores, H. Schneider, N. R. Marcilio, L. Ferret and J. C. P. Oliveira, Potassic zeolites from Brazilian coal ash for use as a fertilizer in agriculture, Waste Manag., 2017, 70, 263–271 CrossRef CAS PubMed
. - S. S. Obaid, D. K. Gaikwad, M. I. Sayyed, K. Al-Rashdi and P. P. Pawar, Heavy metal ions removal from waste water bythe natural zeolites, Mater. Today: Proc., 2018, 5(9), 17930–17934, DOI:10.1016/j.matpr.2018.06.122
. - U. Singh and R. K. Kaushal, Treatment of Waste Water With Low Cost Adsorbent – a Review, VSRD Int. J. Tech. Non-Tech. Res., 2013, 4(33), 33–42 Search PubMed
. - Y. Kobayashi, F. Ogata, T. Nakamura and N. Kawasaki, Synthesis of novel zeolites produced from fly ash by hydrothermal treatment in alkaline solution and its evaluation as an adsorbent for heavy metal removal, J. Environ. Chem. Eng., 2020, 8(2), 103687, DOI:10.1016/j.jece.2020.103687
. - X. Chen, L. Yu, S. Zou, L. Xiao and J. Fan, Zeolite Cotton in Tube: A Simple Robust Household Water Treatment Filter for Heavy Metal Removal, Sci. Rep., 2020, 10(1), 1–9, DOI:10.1038/s41598-020-61-776-8
. - F. T. Fanta, A. A. Dubale, D. F. Bebizuh and M. Atlabachew, Copper doped zeolite composite for antimicrobial activity and heavy metal removal from waste water, BMC Chem., 2019, 13(3), 1–12, DOI:10.1186/s13065-019-0563-1
. - Q. Meng, H. Chen, J. Lin, Z. Lin and J. Sun, Zeolite A synthesized from alkaline assisted pre-activated halloysite for efficient heavy metal removal in polluted river water and industrial wastewater, J. Environ. Sci., 2017, 56, 254–262, DOI:10.1016/j.jes.2016-.10.010
. - M. Kragović, S. Pašalić, M. Marković, M. Petrović, B. Nedeljković and M. Momčilović, et al., Natural and modified zeolite—alginate composites. Application for removal of heavy metal cations from contaminated water solutions, Minerals, 2018, 8(1), 1–16 CrossRef
. - H. F. Makki, Removal of Cadmium (II) and Lead(II) Ions from Aqueous Solution by Zeolite A4 Supported on Natural Carbon, Int. J. Sci. Technol., 2014, 3(7), 391–399 Search PubMed
. - E. Olegario-Sanchez and C. M. Pelicano, Characterization of philippine natural zeolite and its application for heavy metal removal from acid mine drainage (AMD), Key Eng. Mater., 2017, 737, 407–411 Search PubMed
. - M. Deravanesiyan, M. Beheshti and A. Malekpour, Alumina nanoparticles immobilization onto the NaX zeolite and the removal of Cr(III) and Co(II) ions from aqueous solutions, J. Ind. Eng. Chem., 2015, 21, 580–586 CrossRef CAS
. - A. Mondal, B. Banerjee, A. Bhaumik and C. Mukhopadhyay, Activated Alumina Balls under Neat Conditions: A Green Catalyst for the Synthesis of Spiro-Heterocyclic Scaffolds by Ring-Opening versus Annulation of the Isatin Moiety, ChemCatChem, 2016, 8(6), 1185–1198 CrossRef CAS
. - X. Wang, G. Zheng, T. Chen, E. Nie, Y. Wang and X. Shi, et al., Application of ceramsite and activated alumina balls as recyclable bulking agents for sludge composting, Chemosphere, 2019, 218, 42–51, DOI:10.1016/j.chemosphere.2018.11.103
. - K. U. Ahamad, R. Singh, I. Baruah, H. Choudhury and M. R. Sharma, Equilibrium and kinetics modeling of fluoride adsorption onto activated alumina, alum and brick powder, Groundw. Sustain. Dev., 2018, 7, 452–458, DOI:10.1016/j.gsd.2018.06.005
. - R. Prabhakar and S. R. Samadder, Use of adsorption-influencing parameters for designing the batch adsorber and neural network-based prediction modelling for the aqueous arsenate removal using combustion synthesised nano-alumina, Environ. Sci. Pollut. Res., 2020, 27(21), 26367–26384 CrossRef CAS PubMed
. - S. Agarwal, I. Tyagi, V. K. Gupta, M. H. Dehghani, J. Jaafari and D. Balarak, et al., Rapid removal of noxious nickel(II) using novel γ-alumina nanoparticles and multiwalled carbon nanotubes: kinetic and isotherm studies, J. Mol. Liq., 2016, 224, 618–623, DOI:10.1016/j.molliq.2016.10.032
. - W. Yang, Q. Tang, J. Wei, Y. Ran, L. Chai and H. Wang, Enhanced removal of Cd(II) and Pb(II) by composites of mesoporous carbon stabilized alumina, Appl. Surf. Sci., 2016, 369(Ii), 215–223, DOI:10.1016/j.apsusc.2016.01.151
. - G. B. Kunde, B. Sehgal and A. K. Ganguli, Synthesis of mesoporous rebar MWCNT/alumina composite (RMAC) nodules for the effective removal of methylene blue and Cr(VI) from an aqueous medium, J. Hazard. Mater., 2019, 374, 140–151, DOI:10.1016/j.jhazmat.2019.03.099
. - M. Z. A. Zaimee, M. S. Sarjadi and M. L. Rahman, Heavy metals removal from water by efficient adsorbents, Water, 2021, 13(19), 1–22 CrossRef
. - J. a. Abudaia, M. O. Sulyman, K. Y. Elazaby and S. M. Ben-Ali, Adsorption of Pb(II) and Cu(II) from Aqueous Solution onto Activated Carbon Prepared from Dates Stones, Int. J. Environ. Sci. Dev., 2013, 4(2), 191–195 CrossRef CAS
. http://www.ijesd.org/index.php?m=content%26c=index%26a=show%26catid=42%26id=620. - W. S. Chai, J. Y. Cheun, P. S. Kumar, M. Mubashir, Z. Majeed and F. Banat, et al., A review on conventional and novel materials towards heavy metal adsorption in wastewater treatment application, J. Cleaner Prod., 2021, 296, 126589, DOI:10.1016/j.jclepro.2021.126589
. - Y. Hu, X. Liu, J. Bai, K. Shih, E. Y. Zeng and H. Cheng, Assessing heavy metal pollution in the surface soils of a region that had undergone three decades of intense industrialization and
urbanization, Environ. Sci. Pollut. Res., 2013, 20(9), 6150–6159 CrossRef CAS PubMed
. - J. Jjagwe, P. W. Olupot, E. Menya and H. M. Kalibbala, Synthesis and Application of Granular Activated Carbon from Biomass Waste Materials for Water Treatment: A Review, J. Bioresour. Bioprod., 2021, 6(4), 292–322, DOI:10.1016/j.jobab.2021.03.003
. - M. Manjuladevi, R. Anitha and S. Manonmani, Kinetic study on adsorption of Cr(VI), Ni(II), Cd(II) and Pb(II) ions from aqueous solutions using activated carbon prepared from Cucumis melo peel, Appl. Water Sci., 2018, 8(1), 1–8, DOI:10.1007/s13201-018-0674-1
. - H. J. Fan and P. R. Anderson, Copper and cadmium removal by Mn oxide-coated granular activated carbon, Sep. Purif. Technol., 2005, 45(1), 61–67 CrossRef CAS
. - A. A. Alghamdi, A. B. Al-Odayni, W. S. Saeed, A. Al-Kahtani, F. A. Alharthi and T. Aouak, Efficient adsorption of lead(II) from aqueous phase solutions using polypyrrole-based activated carbon, Materials, 2019, 12(12), 1–16 CrossRef PubMed
. - M. Karnib, A. Kabbani, H. Holail and Z. Olama, Heavy metals removal using activated carbon, silica and silica activated carbon composite, Energy Procedia, 2014, 50, 113–120 CrossRef CAS
. http://linkinghub.elsevier-.com/retrieve/pii/S1876610214007504. - Y. Zhang, X. Song, P. Zhang, H. Gao, C. Ou and X. Kong, Production of activated carbons from four wastes via one-step activation and their applications in Pb2+ adsorption: insight of ash content, Chemosphere, 2020, 245, 125587, DOI:10.1016/j.chemosphere.2019.125587
. - J. Bayuo, Decontamination of cadmium(II) from synthetic wastewater onto shea fruit shell biomass, Appl. Water Sci., 2021, 11(5), 1–8, DOI:10.1007/s13201-021-01416-2
. - X. Liu, X. Xu, X. Dong and J. Park, Competitive adsorption of heavy metal ions from aqueous solutions onto activated carbon and agricultural waste materials, Pol. J. Environ. Stud., 2020, 29(1), 749–761 CrossRef CAS
. - S. M. Abegunde, K. S. Idowu, O. M. Adejuwon and T. Adeyemi-Adejolu, A review on the influence of chemical modification on the performance of adsorbents, Res. Environ. Sustain., 2020, 1, 100001 Search PubMed
. - M. B. Amar, K. Walha and V. Salvadó, Evaluation of Olive Stones for Cd(II), Cu(II), Pb(II) and Cr(VI) Biosorption from Aqueous Solution: Equilibrium and Kinetics, Int. J. Environ. Res., 2020, 14(2), 193–204, DOI:10.1007/s41742-020-00246-5
. - R. Baby, B. Saifullah and M. Z. Hussein, Palm Kernel Shell as an effective adsorbent for the treatment of heavy metal contaminated water, Sci. Rep., 2019, 9(1), 1–11 Search PubMed
. - A. Banchhor, M. Pandey and P. K. Pandey, Optimization of Adsorption Parameters for Effective Removal of Hexavalent Chromium Using Simarouba glauca from Aqueous Solution, Water Conserv. Sci. Eng., 2021, 6(3), 127–144, DOI:10.1007/s41-101-021-00106-z
. - J. Bayuo, K. B. Pelig-Ba and M. A. Abukari, Adsorptive removal of chromium(VI) from aqueous solution unto groundnut shell, Appl. Water Sci., 2019, 9(4), 1–11, DOI:10.1007/s13201-019-0987-8
. - Ş. Parlayici and E. Pehlivan, Comparative study of Cr(VI) removal by bio-waste adsorbents: equilibrium, kinetics, and thermodynamic, J. Anal. Sci. Technol., 2019, 10(1), 1–8 CrossRef
. - N. Dhiman, Binary adsorption of [Pb(II) + Co(II)] from aqueous solution using thiolated saw dust, Water Sci. Technol., 2021, 84(9), 2591–2600 CrossRef CAS PubMed
. - Z. Heidarinejad, M. H. Dehghani, M. Heidari, G. Javedan, I. Ali and M. Sillanpää, Methods for preparation and activation of activated carbon: a review, Environ. Chem. Lett., 2020, 18(2), 393–415, DOI:10.1007/s10311-019-00955-0
. - J. Bayuo, K. B. Pelig-ba, M. Abdullaiabukari and M. A. Abukari, Isotherm Modeling Of Lead(II) Adsorption From Aqueous Solution Using Groundnut Shell As A Low-Cost Adsorbent, IOSR J. Appl. Chem., 2018, 11(11), 18–23 CAS
. - F. Safatian, Z. Doago, M. Torabbeigi, H. Rahmani Shams and N. Ahadi, Lead ion removal from water by hydroxyapatite nanostructures synthesized from egg sells with microwave irradiation, Appl. Water Sci., 2019, 9(4), 1–6, DOI:10.1007/s13201-019-0979-8
. - S. Mustapha, D. T. Shuaib, M. M. Ndamitso, M. B. Etsuyankpa, A. Sumaila and U. M. Mohammed, et al., Adsorption isotherm, kinetic and thermodynamic studies for the removal of Pb(II), Cd(II), Zn(II) and Cu(II) ions from aqueous solutions using Albizia lebbeck pods, Appl. Water Sci., 2019, 9(6), 1–11, DOI:10.1007/s13201-019-1021-x
. - W. Boulaiche, B. Belhamdi, B. Hamdi and M. Trari, Kinetic and equilibrium studies of biosorption of M(II) (M = Cu, Pb, Ni, Zn and Cd) onto seaweed Posidonia oceanica fibers, Appl. Water Sci., 2019, 9(8), 1–11, DOI:10.1007/s13201-019-1062-1
. - M. J. A. Alatabe and N. O. Kariem, Thorns, a Novel Natural Plants for Adsorption of Lead(II) Ions from Wastewater: Equilibrium, Isotherm, Kinetics and Thermodynamics, Eurasian J. Anal. Chem., 2019, 14(2), 163–174 CAS
. - B. S. K. Poonam and N. Kumar, Kinetic study of lead (Pb2+) removal from battery manufacturing wastewater using bagasse biochar as biosorbent, Appl. Water Sci., 2018, 8(4), 1–13, DOI:10.1007/s13201-018-0765-z
. - Z. Mahdi, Q. J. Yu and A. El Hanandeh, Removal of lead(II) from aqueous solution using date seed-derived biochar: batch and column studies, Appl. Water Sci., 2018, 8(6), 1–13, DOI:10.1007/s13201-018-0829-0
. - N. Gaur, A. Kukreja, M. Yadav and A. Tiwari, Adsorptive removal of lead and arsenic from aqueous solution using soya bean as a novel biosorbent: equilibrium isotherm and thermal stability studies, Appl. Water Sci., 2018, 8(4), 1–12, DOI:10.1007/s13201-018-0743-5
. - G. F. Coelho, A. C. Gonçalves, D. Schwantes, E. Á. Rodríguez, C. R. T. Tarley and D. Dragunski, et al., Removal of Cd(II), Pb(II) and Cr(III) from water using modified residues of Anacardium occidentale L, Appl. Water Sci., 2018, 8(3), 1–21, DOI:10.1007/s13201-018-0724-8
. - Ł. Klapiszewski, P. Bartczak, T. Szatkowski and T. Jesionowski, Removal of lead(II) ions by an adsorption process with the use of an advanced SiO2/lignin biosorbent, Pol. J. Chem. Technol., 2017, 19(1), 48–53 CrossRef
. - J. N. Ghogomu, S. N. Muluh, D. L. Ajifack, A. A. Alongamo and D. T. Noufame, Adsorption of Lead(II) from Aqueous Solution using Activated Carbon Prepared from Raffia Palm (Raphia Hookeri) Fruit Epicarp, IOSR J. Appl. Chem., 2016, 9(7), 74–85 CAS
. - C. C. Nnaji and S. C. Emefu, A comparative study of lead adsorption by activated Khaya Ivoresnsis and Pycanthus angolensis sawdust, J. Water Sanit. Hyg. Dev., 2016, 6(4), 593–601 CrossRef
. - N. Nordine, Z. El Bahri, H. Sehil, R. I. Fertout, Z. Rais and Z. Bengharez, Lead removal kinetics from synthetic effluents using Algerian pine, beech and fir sawdust's: optimization and adsorption mechanism, Appl. Water Sci., 2016, 6(4), 349–358, DOI:10.1007/s13201-014-0233-3
. - L. E. Yahaya and A. K. Akinlabi, Equilibrium sorption of Lead(II) in aqueous solution onto EDTA-modified Cocoa (Theobroma cacao) Pod husk residue, Iran J. Energy Environ., 2016, 7(1), 58–63 CAS
. - S. N. Kane, A. Mishra and A. K. Dutta, Adsorption of Lead ions onto Activated Carbon derived from Sugarcane bagasse, IOP Conf. Ser.: Mater. Sci. Eng., 2016, 755(1), 1–7 Search PubMed
. - J. Shi, Z. Fang, Z. Zhao, T. Sun and Z. Liang, Comparative study on Pb(II), Cu(II), and Co(II) ions adsorption from aqueous solutions by arborvitae leaves, Desalin. Water Treat., 2015, 1–8, DOI:10.1080/19443994.2015.1089421
. - D. Paliulis, Adsorptive removal of Pb2+ ions from aqueous solutions by Peat, Pol. J. Environ. Stud., 2015, 24(3), 1213–1218, DOI:10.15244/pjoes/31223
. - J. M. Misihairabgwi, A. Kasiyamhuru, P. Anderson, C. J. Cunningham, T. a Peshkur and I. Ncube, Adsorption of heavy metals by agroforestry waste derived activated carbons applied to aqueous solutions, African J. Biotechnol., 2014, 13(14), 1579–1587 CrossRef CAS
. - A. S. Singha and A. Guleria, Chemical modification of cellulosic biopolymer and its use in removal of heavy metal ions from wastewater, Int. J. Biol. Macromol., 2014, 67, 409–417, DOI:10.1016/j.ijbiomac.2014.03.046
. - O. Ogunleye, M. Ajala and S. Agarry, Evaluation of biosorptive capacity of banana (Musa paradisaca) stalk for lead(II) removal from aqueous solution, J. Environ. Prot., 2014, 5(15), 1451–1465 CrossRef
. - N. A. Hikmat, B. B. Qassim and M. T. Khethi, Thermodynamic and Kinetic Studies of Lead Adsorption from Aquesous Solution onto Petiole and Fiber of Palm Tree, Am. J. Chem., 2014, 4(4), 116–124 Search PubMed
. - N. H. Yarkandi, Removal of lead(II) from waste water by adsorption, Int. J. Curr. Microbiol. Appl. Sci., 2014, 3(4), 207–228 Search PubMed
. - M. G. A. Vieira, A. F. De Almeida Neto, M. G. C. Da Silva, C. N. Carneiro and A. A. M. Filho, Adsorption of lead and copper ions from aqueous effluents on rice husk ash in a dynamic system, Brazilian J. Chem. Eng., 2014, 31(2), 519–529 CrossRef
. - Y. U. Kouakou, A. S. Ello, A. J. Yapo, I. M. Gouli-Bi and A. Trokourey, Mechanistic Study of Lead Adsorption on Activated Carbon, Langmuir, 2018, 34(45), 13565–13573 CrossRef PubMed
. - S. Ghasemi and R. M. Gholami, Kinetic and Isotherm Study of Lead Adsorption from Synthetic Wastewater by Myrtaceae Sawdust, Bull. Environ., Pharmacol. Life Sci., 2014, 3, 157–165 Search PubMed
. - H. Mokaddem, N. Azouaou, Y. Kaci and Z. Sadaoui, Study of lead adsorption from aqueous solutions on agar beads with eps produced from paenibacillus polymyxa, Chem. Eng. Trans., 2014, 38, 31–36 Search PubMed
. - N. Haloi, H. P. Sarma and P. Chakravarty, Biosorption of lead(II) from water using heartwood charcoal of Areca catechu: equilibrium and kinetics studies, Appl. Water Sci., 2013, 3(3), 559–565 CrossRef CAS
. - M. Athar, U. Farooq, M. Aslam and M. Salman, Adsorption of Pb(II) ions onto biomass from Trifolium resupinatum: equilibrium and kinetic studies, Appl. Water Sci., 2013, 3(3), 665–672 CrossRef CAS
. - A. I. Adeogun, M. A. Idowu, A. E. Ofudje, S. O. Kareem and S. A. Ahmed, Comparative biosorption of Mn(II) and Pb(II) ions on raw and oxalic acid modified maize husk: kinetic, thermodynamic and isothermal studies, Appl. Water Sci., 2013, 3(1), 167–179 CrossRef CAS
. - N. Azouaou, M. Belmedani, H. Mokaddem and Z. Sadaoui, Adsorption of lead from aqueous solution onto untreated orange barks, Chem. Eng. Trans., 2013, 32, 55–60 Search PubMed
. - D. L. Mitic-Stojanovic, A. Zarubica, M. Purenovic, D. Bojic, T. Andjelkovic and A. L. Bojic, Biosorptive removal of Pb2+Cd2+ and Zn2+ ions from water by lagenaria vulgaris shell, Water SA, 2011, 37(3), 303–312 CrossRef CAS
. - S. Qaiser, A. R. Saleemi and M. Umar, Biosorption of lead(II) and chromium(VI) on groundnut hull: equilibrium, kinetics and thermodynamics study, Electron. J. Biotechnol., 2009, 12(4), 1–9 Search PubMed
. - K. G. Bhattacharyya and A. Sharma, Adsorption of Pb(II) from aqueous solution by Azadirachta indica (Neem) leaf powder, J. Hazard. Mater., 2004, 113(1–3), 97–109 CrossRef CAS PubMed
. - M. Abdulkarim and F. A. Al-Rub, Adsorption of lead ions from aqueous solution onto activated carbon and chemically-modified activated carbon prepared from date pits, Adsorpt. Sci. Technol., 2004, 22(2), 119–134 CrossRef CAS
. - K. K. Wong, C. K. Lee, K. S. Low and M. J. Haron, Removal of Cu and Pb by tartaric acid modified rice husk from aqueous solutions, Chemosphere, 2003, 50(1), 23–28 CrossRef CAS PubMed
. - J. Bayuo, M. A. Abukari and K. B. Pelig-ba, Equilibrium isotherm studies for the sorption of hexavalent chromium(VI) onto groundnut shell, IOSR J. Appl. Chem., 2019, 11(12), 40–46 Search PubMed
. - R. Carnier, A. R. Coscione, C. A. d. Abreu, L. C. A. Melo and A. F. d. Silva, Cadmium and lead adsorption and desorption by coffee waste-derived biochars, Soil Plant Nutr., 2021, 81, 1–15 Search PubMed
. - F. O. Afolabi, P. Musonge and B. F. Bakare, Bio-sorption of a bi-solute system of copper and lead ions onto banana peels: characterization and optimization, J. Environ. Heal Sci. Eng., 2021, 19(1), 613–624 CrossRef CAS PubMed
. - I. H. Dakhil, G. F. Naser and A. H. Ali, Response Surface Modeling of Arsenic Adsorption by Modified Spent Tea Leaves, IOP Conf. Ser.: Mater. Sci. Eng., 2021, 1090(1), 012129 CAS
. - M. S. Abesekara, K. N. R. Kosvinna and B. M. W. P. K. Amarasinghe, Adsorption and desorption studies of Ni2+ ions on to coconut shell char, IOP Conf. Ser. Earth Environ. Sci., 2020, 427(1), 1–9 Search PubMed
. - H. Çelebi, G. Gök and O. Gök, Adsorption capability of brewed tea waste in waters containing toxic lead(II), cadmium(II), nickel(II), and zinc(II) heavy metal ions, Sci. Rep., 2020, 10(1), 1–12, DOI:10.1038/-s41598-020-74553-4
. - T. F. Akinhanmi, E. A. Ofudje, A. I. Adeogun, P. Aina and I. M. Joseph, Orange peel as low-cost adsorbent in the elimination of Cd(II) ion: kinetics, isotherm, thermodynamic and optimization evaluations, Bioresour. Bioprocess., 2020, 7(1), 1–16, DOI:10.1186/s40643-020-00320-y
. - R. K. Ghosh, D. P. Ray, S. Chakraborty, B. Saha, K. Manna and A. Tewari, et al., Cadmium removal from aqueous medium by jute stick activated carbon using response surface methodology: factor optimisation, equilibrium, and regeneration, Int. J. Environ. Anal. Chem., 2020, 1–18, DOI:10.1080/03067319.2019.1700964
. - J. O. Ighalo, A. G. Adeniyi, O. A. A. Eletta and L. T. Arowoyele, Competitive adsorption of Pb(II), Cu(II), Fe(II) and Zn(II) from aqueous media using biochar from oil palm (Elaeis guineensis) fibers: a kinetic and equilibrium study, Indian Chem. Eng., 2020, 1–11, DOI:10.1080/00194506.2020.1787870
. - M. Jain, V. K. Garg, R. Paliwal, K. Kadirvelu and S. Chaudhry, Optimization of cadmium(II) removal from water using sunflower waste carbon–a statistical approach, Toxin Rev., 2020, 1–10, DOI:10.1080/15569543.2020.1718163
. - Y. Yu, Q. An, L. Jin, N. Luo, Z. Li and J. Jiang, Unraveling sorption of Cr(VI) from aqueous solution by FeCl3 and ZnCl2-modified corn stalks biochar: implicit mechanism and application, Bioresour. Technol., 2020, 297(174), 1–7 Search PubMed
. - J. U. Ani, A. E. Ochonogor, K. G. Akpomie, C. S. Olikagu and C. C. Igboanugo, Abstraction of arsenic(III) on activated carbon prepared from Dialium guineense seed shell: kinetics, isotherms and thermodynamic studies, SN Appl. Sci., 2019, 1(10), 1–11, DOI:10.1007-/s42452-019-1335-1
. - G. K. Gupta and M. K. Mondal, Mechanism of Cr(VI) uptake onto sagwan sawdust derived biochar and statistical optimization via response surface methodology, Biomass Convers. Biorefinery., 2020, 1–17 Search PubMed
. - M. R. Hadiani, K. Khosravi-Darani and N. Rahimifard, Optimization of As(III) and As (V) removal by Saccharomyces cerevisiae biomass for biosorption of critical levels in the food and water resources, J. Environ. Chem. Eng., 2019, 7(2), 102949, DOI:10.10-16/j.jece.2019.102949
. - K. Javidi Alsadi and N. Esfandiari, Synthesis of activated carbon from sugarcane bagasse and application for mercury adsorption, Pollution, 2019, 5(3), 585–596 Search PubMed
. - P. Lin, J. Wu, J. Ahn and J. Lee, Adsorption characteristics of Cd(II) and Ni(II) from aqueous solution using succinylated hay, Int. J. Miner., Metall. Mater., 2019, 26(10), 1239–1246 CrossRef CAS
. - H. Ma, J. Yang, X. Gao, Z. Liu, X. Liu and Z. Xu, Removal of chromium(VI) from water by porous carbon derived from corn straw: influencing factors, regeneration and mechanism, J. Hazard. Mater., 2019, 369, 550–560, DOI:10.1016/j.jhazmat.2019.02.063
. - N. K. Mondal, S. Basu and B. Das, Decontamination and optimization study of hexavalent chromium on modified chicken feather using response surface methodology, Appl. Water Sci., 2019, 9(3), 1–15, DOI:10.1007/s13201-019-0930-z
. - M. Nur-E-Alam, M. Abu Sayid Mia, F. Ahmad and M. Mafizur Rahman, Adsorption of chromium (Cr) from tannery wastewater using low-cost spent tea leaves adsorbent, Appl. Water Sci., 2018, 8(5), 1–7, DOI:10.1007/s13201-018-0774-y
. - M. J. Rwiza, S. Y. Oh, K. W. Kim and S. D. Kim, Comparative sorption isotherms and removal studies for Pb(II) by physical and thermochemical modification of low-cost agro-wastes from Tanzania, Chemosphere, 2018, 195(2), 135–145, DOI:10.1016/j.chemosphere.2017.12.043
. - C. N. Chidozie, J. E. Chinwe and I. U. Emmanuel, Physicochemical Conditions for Adsorption of Lead from Water by Rice Husk Ash, Bioresources, 2017, 12, 799–818 Search PubMed
. - M. Petrović, T. Šoštarić, M. Stojanović, J. Petrović, M. Mihajlović and A. Ćosović, et al., Mechanism of adsorption of Cu2+ and Zn2+ on the corn silk (Zea mays L.), Ecol. Eng., 2017, 99, 83–90 CrossRef
. - S. Mondal, K. Aikat and G. Halder, Biosorptive uptake of arsenic(V) by steam activated carbon from mung bean husk: equilibrium, kinetics, thermodynamics and modeling, Appl. Water Sci., 2017, 7(8), 4479–4495 CrossRef CAS
. - C. Tang, Y. Shu, R. Zhang, X. Li, J. Song and B. Li, et al., Comparison of the removal and adsorption mechanisms of cadmium and lead from aqueous solution by activated carbons prepared from Typha angustifolia and Salix matsudana, RSC Adv., 2017, 7(26), 16092–16103 RSC
. - L. Nemeş and L. Bulgariu, Optimization of process parameters for heavy metals biosorption onto mustard waste biomass, Open Chem., 2016, 14(1), 175–187 Search PubMed
. - M. Motaghi and P. Ziarati, Adsorptive removal of cadmium and lead from Oryza sativa rice by banana peel as bio-sorbent, Biomed. Pharmacol. J., 2016, 9(2), 739–749 CrossRef
. - K. Vijayaraghavan, S. Rangabhashiyam, T. Ashokkumar and J. Arockiaraj, Mono- and multi-component biosorption of lead(II), cadmium(II), copper(II) and nickel(II) ions onto coco-peat biomass, Sep. Sci. Technol., 2016, 51(17), 2725–2733 CrossRef CAS
. - C. H. Wu, C. Y. Kuo and S. S. Guan, Adsorption kinetics of lead and zinc ions by coffee residues, Pol. J. Environ. Stud., 2016, 24(2), 761–767 Search PubMed
. - M. R. Boni, A. Chiavola and S. Marzeddu, Remediation of Lead-Contaminated Water by Virgin Coniferous Wood Biochar Adsorbent: Batch and Column Application, Water Air Soil Pollut., 2020, 231(4), 9–16 CrossRef
. - A. Das, N. Bar and S. K. Das, Pb(II) adsorption from aqueous solution by nutshells, green adsorbent: adsorption studies, regeneration studies, scale-up design, its effect on biological indicator and MLR modeling, J. Colloid. Interface. Sci., 2020, 580, 245–255, DOI:10.1016/j.jcis.2020.07.017
. - M. Akram, B. Khan, M. Imran, I. Ahmad, H. Ajaz and M. Tahir, et al., Biosorption of lead by cotton shells powder: characterization and equilibrium modeling study, Int. J. Phytoremediation, 2019, 21(2), 138–144, DOI:10.1080/15226514.2018.1488810
. - L. B. L. Lim, N. Priyantha, Y. C. Lu and N. A. H. Mohamad Zaidi, Adsorption of heavy metal lead using Citrus grandis (Pomelo) leaves as low-cost adsorbent, Desalin. Water Treat., 2019, 166, 44–52 CrossRef CAS
. - C. T. Onwordi, C. C. Uche, A. E. Ameh and L. F. Petrik, Comparative study of the adsorption capacity of lead(II) ions onto bean husk and fish scale from aqueous solution, J. Water Reuse Desalin., 2019, 9(3), 249–262 CrossRef CAS
. - A. R. A. Rahim, N. E. Rabat, K. Johari, N. Saman and H. Mat, Removal of lead(II) ions from aqueous solution using desiccated coconut waste as low-cost adsorbent, Chem. Eng. Trans., 2019, 72, 169–174 Search PubMed
. - F. J. Alguacil, L. Alcaraz, I. García-Díaz and F. A. López, Removal of Pb2+ in wastewater via adsorption onto an activated carbon produced from winemaking waste, Metals, 2018, 8(9), `1–15 CrossRef
. - C. S. T. Araújo, I. L. S. Almeida, H. C. Rezende, S. M. L. O. Marcionilio, J. J. L. Léon and T. N. de Matos, Elucidation of mechanism involved in adsorption of Pb(II) onto lobeira fruit (Solanum lycocarpum) using Langmuir, Freundlich and Temkin isotherms, Microchem. J., 2018, 137, 348–354, DOI:10.1016/j.microc.2017.11.009
. - D. M. H. Karimi, S. M. Davoodi, A. Y. Dursun, M. R. Ehsani, I. Karimpour and E. Ameri, Application of treated eggplant peel as a low-cost adsorbent for water treatment toward elimination of Pb2+: kinetic modeling and isotherm study, Adsorpt. Sci. Technol., 2018, 36(3–4), 1112–1143 CrossRef
. - R. Pratiwi and P. D. Prinajati, Adsorption for Lead Removal by Chitosan from Shrimp Shells, Indones. J. Urban. Environ. Technol., 2018, 2(1), 35 CrossRef
. - G. M. Shah, M. Nasir, M. Imran, H. F. Bakhat, F. Rabbani and M. Sajjad, et al., Biosorption potential of natural, pyrolysed and acid-assisted pyrolysed sugarcane bagasse for the removal of lead from contaminated water, PeerJ, 2018, 2018(9), 1–20 Search PubMed
. - B. G. Alhogbi, Potential of coffee husk biomass waste for the adsorption of Pb(II) ion from aqueous solutions, Sustainable Chem. Pharm., 2017, 6, 21–25, DOI:10.1016/j.scp.2017.06.004
. - M. Basu, A. K. Guha and L. Ray, Adsorption of Lead on Cucumber Peel, J. Cleaner Prod., 2017, 151, 603–615, DOI:10.1016/j.jclepro.2017.03.028
. - O. S. Lawal, O. S. Ayanda, O. O. Rabiu and K. O. Adebowale, Application of black walnut (Juglans nigra) husk for the removal of lead(II) ion from aqueous solution, Water Sci. Technol., 2017, 75(10), 2454–2464 CrossRef CAS PubMed
. - I. Umar Salihi, S. Rahman Mohamed Kutty and M. Hasnain Isa, Equilibrium and kinetic studies on lead(II) adsorption by sugarcane bagasse derived activated carbon, Int. J. Eng., Trans. B, 2017, 30(11), 1647–1653 Search PubMed
. - A. A. Al-Homaidan, A. F. Al-Abbad, A. A. Al-Hazzani, A. A. Al-Ghanayem and J. A. Alabdullatif, Lead removal by Spirulina platensis biomass, Int. J. Phytorem., 2016, 18(2), 184–189, DOI:10.1080/152265-14.2015.1073673
. - R. Mopoung and N. Kengkhetkit, Lead and cadmium removal efficiency from aqueous solution by NaOH treated pineapple waste, Int. J. Appl. Chem., 2016, 12(1), 23–35 Search PubMed
. - S. Lagergren, Zur theorie der sogenannten adsorption geloster stoffe, K. Sven. Vetenskapsakad. Handl., 1898, 24, 1–39 Search PubMed
. - Y. Ho, Isotherms for the sorption of lead onto peat: comparison of linear and non-linear methods, Pol. J. Environ. Stud., 2006, 15(1), 81–86 CAS
. - M. S. Rahman and K. V. Sathasivam, Heavy metal adsorption onto kappaphycus sp. from aqueous solutions: the use of error functions for validation of isotherm and kinetics models, BioMed Res. Int., 2015, 2015, 1–13 Search PubMed
. - M. J. D. Low, Kinetics of Chemisorption of Gases on Solids, Chem. Rev., 1960, 60(3), 267–312, DOI:10.1021/cr60205a-003
. - W. Weber and F. DiGiano, Process dynamics in Environmental Systems: Environmental Science and Technology Service, Wiley Sons, New York, 1996, pp. 89–94 Search PubMed
. - I. Langmuir, The constitution and fundamental properties of solids and liquids, J. Am. Chem. Soc., 1916, 38(11), 2221–2295 CrossRef CAS
. - H. Freundlich, Über die Adsorption in Lösungen, Z. Phys. Chem., 1907, 57(1), 385–470 CrossRef CAS
. - M. M. Dubinin, L. V. Radushkevich and E. D. Zaverina, Sorption and structure of active carbons, J. Phys. Chem., 1947, 2, 1351–1362 Search PubMed
. - K. K. H. Choy, G. McKay and J. F. Porter, Sorption of acid dyes from effluents using activated carbon, Resour., Conserv. Recy., 1999, 27(1–2), 57–71 CrossRef
. - K. Y. Foo and B. H. Hameed, Insights into the modeling of adsorption isotherm systems, Chem. Eng. J., 2010, 156(1), 2–10 CrossRef CAS
. - A. Achmad, J. Kassim, T. K. Suan, R. C. Amat and T. L. Seey, Equilibrium, kinetic and thermodynamic studies on the adsorption of direct dye onto a novel green adsorbent developed from Uncaria gambir extract, J. Phys. Sci., 2012, 23(1), 1–13 CAS
. - J. Bayuo, M. A. Abukari and K. B. Pelig-Ba, Optimization using central composite design (CCD) of response surface methodology (RSM) for biosorption of hexavalent chromium from aqueous media, Appl. Water Sci., 2020, 10(6), 1–12, DOI:10.1007/s13201-020-01213-3
. - J. Toth, State Equations of Solid-Gas Interface Layers, Acta Chim. Acad. Sci. Hung., 1971, 69(3), 311 CAS
. - M. F. Dilekoglu, Use of genetic algorithm optimization technique in the adsorption of phenol on banana and grapefruit peels, J. Chem. Soc. Pak., 2016, 38(6), 1252–1262 CAS
.
|
This journal is © The Royal Society of Chemistry 2022 |
Click here to see how this site uses Cookies. View our privacy policy here.