DOI:
10.1039/D2RA00241H
(Paper)
RSC Adv., 2022,
12, 18722-18727
Transient directing group enabled Pd-catalyzed C–H oxygenation of benzaldehydes and benzylic amines†
Received
13th January 2022
, Accepted 11th June 2022
First published on 27th June 2022
Abstract
We report a general protocol for <i>ortho</i>-C–H fluoroalkoxylation of benzaldehydes and benzylic amines utilizing an inexpensive amino amide as a transient directing group. In the presence of an electrophilic fluorinating bystanding oxidant and fluorinated alcohols, a wide range of benzaldehydes and benzylic amines could be oxygenated selectively at the ortho positions to afford fluoroalkyl aryl ethers. This elegant approach would provide appealing strategies for synthesis of drug molecules and natural products.
Introduction
The directed activation of carbon–hydrogen bonds (C–H) is a topic of increasing importance with a wide range of applications in organic synthesis.1 However, one of the major challenges in selective, catalytic functionalization of C–H bonds is to enable the use of native substrates without covalently installing external directing groups. Transient directing groups (TDGs), which form reversible linkages with the substrates in situ, complement a promising alternative approach to direct metal-catalysed or -mediated C–H functionalization, thereby avoiding the additional installation and removal of directing groups.2 While most of the achievements for TDG-mediated and Pd-catalyzed C(sp2)–H functionalization are restricted in C(sp2)–H arylation,3 alkylation,4 and alkenylation,5 efforts to develop Pd-catalyzed C(sp2)–O and C(sp2)–N bond forming reactions have recently afforded new advances.6 Recently, the seminal examples of these rare reactions, which were reported by Erik J. Sorensen and co-workers, featured the utilization of 4-chloroanthranilic acid as the bidentate transient directing group (BiTDG), and 1-fluoro-2,4,6-trimethylpyridnium triflate as the bystanding oxidant.7 Soon after, ortho-C–H methoxylation and fluoroalkoxylation of benzaldehydes were also realized by employing monodentate directing groups8a and BiTDG respectively which form N, O-bis-coordinated complexes with Pd(II) catalyst and promote the C–H activation process.8b Nevertheless, mild and selective transformations of this type are still largely undeveloped and the new catalytic TDGs, e.g., N, N-bidentate coordination, remain to be further exploited. Therefore, the investigation of new TDGs allowing flexible and diverse reactivities is of great importance and highly desirable.
The use of the peptide backbone in site-selective C–H oxygenations of tripeptides presented catalytic action by N, N-bidentate coordination of amide groups with Pd (Scheme 1A).9 Significantly, a bulky, amino amide transient directing group was developed to selectively promote the benzylic C(sp3)–H acetoxylation with low yield (29%) (Scheme 1B).10 Based on this particular investigation and our previous studies of using amino amide as catalytic transient directing group enabled C(sp2)–H activation via N, N-bidentate coordination with Pd catalyst,3d,k we speculated that an amino amide could serve as a suitable TDG which could form a N, N-coordinated complex with Pd(II) to enable subsequent C(sp2)–H oxidation in a manner similar to the N,N-bidentate coordination in the tripeptides, and decided to explore the feasibility of oxidation of benzaldehydes using amino amide transient directing group. Herein, we report an amino amide as TDG utilized to promote C(sp2)–H oxygenation of broad range of benzaldehydes and benzylic amines using N-fluoro-2,4,6-trimethylpyridinium tetrafluoroborate as the bystanding oxidant (Scheme 1C), the protocol features a one-step coupling between a wide range of benzaldehydes and benzylic amines and alcohols via C–O bond formation at the unactivated positions.11
 |
| Scheme 1 Controlled site-selective C–H bond oxygenation. | |
To establish the viability of the C(sp2)–H oxygenation of benzaldehydes, diverse molecules containing amino groups were investigated their role in palladium-catalyzed oxygenation of benzaldehyde (1a) with trifluoroethanol (2a) in the presence of 10 mol% of Pd(OAc)2, 2 equiv. of TFA and 2 equiv. of K2S2O8 (Table 1). First, commercially available glycinamides, such as 2-aminoacetamide hydrochloride (A1) and 2-aminopropanamide hydrochloride (A2), and hydrazides, such as picolinohydrazide (A3) and acetohydrazide (A4), were employed as TDGs for C(sp2)–H oxygenation and all afforded low yield. To further explore the efficiency of TDG, the screening of selected short peptides was conducted. It turned out that the reaction failed with 2-(2-aminoacetamido)acetic acid (A5) as the TDG, inversely its analogue, 2-(2-(2-aminoacetamido)acetamido)- acetic acid (A6), exhibited moderate catalytic activity. In contrast, all substituted peptides (A7–A9) led to the formation of the desired oxidation products (3a) compared with A5, and A9 afforded the best result. Then, a control experiment without a TDG afforded no desired product, indicating that TDGs are crucial to this reaction.
Table 1 TDG evaluation for the C(sp2)–H oxygenation of benzaldehydesa
Reaction conditions: 1a (0.2 mmol), TFE (2 mL), Pd(OAc)2 (10 mol%), TDG (50 mol%), K2S2O8 (0.4 mmol), TFA (2 equiv.) and stirred at 80 °C for 12 h. The per-centages under the chemical structures are their isolated yields. |
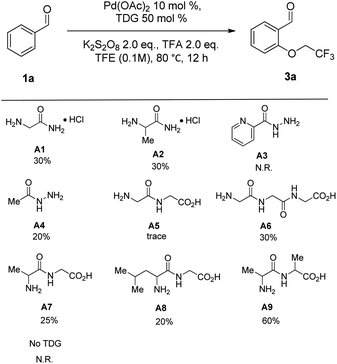 |
With the best TDG in the presence of K2S2O8 identified, we tested different oxidants and found that 1-fluoro-2,4,6-trimethylpyridinium tetrafluoroborate F1 resulted in a better yield (Table 2, entries 1–5). Screening of the amount of TFA indicated that either low or high loading was not beneficial for the reaction (Table 2, entries 6–10), and 2 equiv. of TFA were still preferred. Other reaction parameters, including reaction temperature and solvents, were examined. Decreases in the reaction temperature led to an obvious drop in the yield (Table 2, entries 11 and 12). Compared with trifluoroethanol, other solvents, such as toluene and dichloromethane, were not applicable to the reaction (Table 2, entries 13–17). Additionally, 2-aminopropanoic acid was used as a contrast TDG under the optimum conditions, only 35% yield was achieved (Table 2, entry 18). Therefore, it can be presumed that the short peptides rather than its, hydrolysate can coordinate with Pd(II) via N, N-bidentate coordination and further promote the C–H activation process. Interestingly, a lower yield was observed with either increased or decreased loading of the A9 (Table 2, entries 19 and 20). Lastly, control experiments show both Pd(OAc)2 and the oxidant F1 are essential for this transformation (Table 2, entry 21 and 22).
Table 2 Optimization of the reaction conditionsa

|
Entry |
Oxidant |
TFA (equiv.) |
Yieldb(%) |
Reaction conditions: 1a (0.2 mmol), TFE (2 mL), [Pd] (10 mol%), TDG (50 mol%), oxidant (0.4 mmol), TFA (2 equiv.) and stirred at 80 °C for 12 h. Isolated yield. Other oxidants: (NH4)2S2O8, Na2S2O8, PhI(OAc)2, AgTFA, AgOAc, Ag2CO3, Ag2O. 60 °C. 40 °C. Solvent: TFE/Toluene (v/v, 1 : 1). Solvent: TFE/DCM (v/v, 1 : 1). DCM as solvent, 10 equiv. TFE. DCM as solvent, 20 equiv. TFE. DCM as solvent, 30 equiv. TFE. 2-Aminopropanoic acid instead of A9. Reaction performed with 0.3 equiv. of A9. Reaction performed with 0.7 equiv. of A9. without Pd(OAc)2. |
1 |
F1 |
2 |
88 |
2 |
F2 |
2 |
60 |
3 |
F3 |
2 |
45 |
4 |
F4 |
2 |
60 |
5 |
Other oxidantsc |
2 |
<30 |
6 |
F1 |
0 |
30 |
7 |
F1 |
1 |
50 |
8 |
F1 |
3 |
80 |
9 |
F1 |
5 |
78 |
10 |
F1 |
10 |
78 |
11d |
F1 |
2 |
75 |
12e |
F1 |
2 |
60 |
13f |
F1 |
2 |
Trace |
14g |
F1 |
2 |
30 |
15h |
F1 |
2 |
Trace |
16i |
F1 |
2 |
Trace |
17j |
F1 |
2 |
25 |
18k |
F1 |
2 |
35 |
19l |
F1 |
2 |
30 |
20m |
F1 |
2 |
72 |
21n |
F1 |
2 |
N.R. |
22 |
— |
2 |
N.R. |
Under optimal conditions, we next investigated the substrate scope of substrates of benzaldehydes and fluorinated alcohols (Scheme 2). Firstly, the substrate scope of the various benzaldehydes was examined for the C(sp2)–H bond trifluoroethoxylation. We were delighted to find that different benzaldehydes bearing the alkyl group, halogen and the electron-rich methoxy group substituents were well tolerated to give the desired products in moderate to good yields (3a–3i).
 |
| Scheme 2 Scope of aldehydes and fluorinated alcohols.a a Reaction conditions: 1 (0.2 mmol), fluorinated alcohols (2 mL), Pd(OAc)2 (10 mol%), A9 (50 mol%), F1 (0.4 mmol), TFA (2 equiv.) and stirred at 80 °C for 12 h. All yields given are those for the isolated products. | |
When we changed the placement of the methyl or methoxyl group from the para-position to the meta-position, satisfactory results were also obtained (3b, 3c, 3g and 3h). It is noteworthy that 2-ethylbenzaldehyde also showed reaction activity in spite of the steric hindrance effect, affording the corresponding product in moderate yields (3d). Whereas, strong electron-deficient benzaldehydes with ester and nitro substituents only provided trace amounts of the product (3j and 3k). Furthermore, we also screened the other fluorinated alcohols under the standard conditions for obtaining fluorinated compounds. Gratifyingly, polyfluoroalkoxylation of benzaldehyde with other polyfluoro-alcohols proceeded smoothly with completely selectivity in moderate yields (3l–3n).12 Significantly, the ortho-C–H di-polyfluoroalkoxylation products could not be detected, demonstrating that the transformation showed good selectivity for the mono-fluoroalkoxylation. Moreover, 3-methylbenz-aldehyde and 3, 4-dimethoxy-benzaldehyde also afforded the corresponding mono-polyfluoroalkoxylated products in 49–64% yields (3o, 3p and 3s). Notably, secondary fluorinated alcohols such as 1,1,1,3,3,3-hexafluoro-2-propanol were also effective nucleophiles towards the benzaldehyde with steric hindrance, affording the highly hindered fluoroalkyl ethers in 55% isolated yields (3r).
Since the current reaction conditions were oxidative, we next wondered whether benzylic amines could be oxidized and serve as effective coupling partners for this reaction. Benzylamine instead of benzaldehyde was used as a substrate, and reacted with trifluoroethanol under the optimal reaction conditions (Table 3). To our delight, the C–N and ortho-C(sp2)–H consecutive oxidation of benzylamine could also be accomplished in one pot, albeit with lower efficiency (Table 3, entries 1). Upon evaluation of various oxidants, we discovered that although the [F+] bystanding oxidants gave inferior yields of the corresponding products in less than 10% (Table 3, entry 3–5), K2S2O8 was identified as potentially effective oxidant for this reaction, affording the desired product in 70% yield (Table 3, entry 2). Interestingly, the reaction with dual oxidant (F1/F4) system could be further optimized up to 80% isolated yield (Table 3, entry 6–10).
Table 3 Optimization of the consecutive oxidation of benzylamine

|
Entry |
Oxidant |
Temperature(°C) |
Yielda (%) |
Isolated yields. Benzaldehyde was formed as major product. F1 (0.2 mmol), K2S2O8 (0.2 mmol), under air. F1 (0.2 mmol), F4 (0.2 mmol), under air. F1 (0.2 mmol), F4 (0.2 mmol), under N2. |
1 |
F1 |
80 |
18% |
2 |
K2S2O8 |
80 |
70% |
3 |
F2 |
80 |
Trace |
4 |
F3 |
80 |
Trace |
5 |
F4 |
80 |
10%b (86%) |
6c |
F1+ K2S2O8 |
80 |
78% |
7d |
F1+F4 |
80 |
80% |
8d |
F1+F4 |
60 |
70% |
9d |
F1+F4 |
50 |
55% |
10e |
F1+F4 |
80 |
78% |
On the basis of this strategy, a variety of amines were investigated under new conditions. N-benzyl primary amines having methyl group, halogens and the electron-rich methoxy group on the aromatic ring all underwent the desired oxidation reaction in 45–80% yields (Scheme 3, entry 1–6). Unfortunately, N-benzyl secondary amines, such as N-methyl-1-phenylmethanamine and N-benzylpropan-1-amine, only provided trace amounts of yield, which indicated that the secondary amines are not suitable for this process (Scheme 3, entry 7–10). Remarkably, N-benzyl tertiary amines were competent to undergo the reaction in moderate yields (Scheme 3, entry 11–17).
 |
| Scheme 3 Scope of benzylic amines.a a Reaction conditions: 4 (0.2 mmol), TFE (2 mL), Pd(OAc)2 (10 mol%), A9 (50 mol%), F1 (0.2 mmol), F4 (0.2 mmol), TFA (2 equiv.) and stirred at 80 °C for 12 h. All yields given are those for the isolated products. | |
Based on our experiments and related literature,3d,k,6–8 a putative mechanism was proposed for the palladium-catalyzed ortho-C(sp2)–H oxidation of benzaldehydes (Scheme 4). Benzyl amines get converted into imine intermediate by the [F+] bystanding oxidant, which in turn could be hydrolyzed to the aldehydes. Condensation of benzaldehydes with the ligand 2-(2-aminopropanamido)propanoic acid provides the imine intermediate A. Coordination of this imine to a palladium species followed by cyclopalladation process generates the [5, 5]-bicyclic palladium intermediate C via a site-selective C–H bond activation process, and oxidative addition of the intermediate C with fluorinating bystanding oxidant generates the palladium(IV) species D. Next, the fluoride anion is displaced by the fluorinated alcohol to provide intermediate E. Reductive elimination of this palladium complex followed by a ligand dissociation process provides the α-imino amide, which releases the desired product, and ligand 2-(2-aminopropanamido)propanoic acid.
 |
| Scheme 4 Proposed mechanism. | |
Conclusions
In summary, we have developed a general method for the C(sp2)–H fluoroalkoxylation of benzaldehydes and benzylic amines using 2-(2-aminopropanamido)propanoic acid as the transient directing group and the [F+] bystanding oxidant. Moderate to good yields were obtained for a broad substrate scope under mild conditions. Given the efficient and selective processes for the functionalization of C(sp2)–H bonds, the approach will greatly enrich the toolbox and provide alternatively method for transformation of advanced synthetic intermediates in a single step. More importantly, the approach should find broad applications in synthesis of ubiquitous structural units in biologically active natural products and pharmaceuticals. Detailed mechanistic studies and new applications of this TDG strategy are underway in our laboratory.
Experimental
Typical procedure for palladium-catalyzed C(sp2)–H polyfluor-oalkoxylation of benzaldehydes
An 8 mL vial equipped with a stir bar was charged with Pd(OAc)2 (4.5 mg, 0.02 mmol, 10 mol%), A9 (16.0 mg, 0.1 mmol, 50 mol%), 1-fluoro-2,4,6-trimethylpyrdinium triflate (F1) (90.8 mg, 0.4 mmol, 2.0 equiv.), and benzaldehydes (0.2 mmol, 1.0 equiv.), followed by the addition of fluorinated alcohols (2.0 mL) and TFA (2.0 equiv.). The flask was then sealed and the mixture was stirred at room temperature for 10 min before being heated to 80 °C for 12 h. The reaction mixture was cooled to room temperature, followed by the addition of water and ethyl acetate. The organic layer was separated and the aqueous layer was extracted with ethyl acetate. The combined organic layer was washed with brine and dried over anhydrous MgSO4, filtrated and concentrated in vacuo, the residue was purified through column chromatography on silica gel to give the desired products.
Typical procedure for the C–N and ortho-C(sp2)–H consecutive oxidation of benzylamines
Selectfluor (F4) (70.8 mg, 0.2 mmol, 1.0 equiv.) and 1-fluoro-2,4,6-trimethylpyrdinium triflate (F1) (90.8 mg, 0.2 mmol, 1.0 equiv.) were added to a solution of benzylamines (0.2 mmol, 1.0 equiv.) in fluorinated alcohols (2.0 mL). The reaction mixture was stirred at 80 °C for 30 min, followed by the addition of Pd(OAc)2 (4.5 mg, 0.02 mmol, 10 mol%), A9 (16.0 mg, 0.1 mmol, 50 mol%), and TFA (2.0 equiv.). The reaction mixture was stirred at the same temperature for 12 h. Upon completion, the reaction mixture was cooled to room temperature, followed by the addition of water and ethyl acetate. The organic layer was separated and the aqueous layer was extracted with ethyl acetate. The combined organic layer was washed with brine and dried over anhydrous MgSO4, filtrated and concentrated in vacuo, the residue was purified through column chromatography on silica gel to give the desired products.
Conflicts of interest
There are no conflicts to declare.
Acknowledgements
This research was financially supported by the Scientific Research Foundation of Yunnan Provincial Education Department (No. 2019J1061), Applied Basic Research Project of Yunnan (No. 202001AU070096).
Notes and references
- For examples on functionalization of C-H bond:
(a) M. C. Whisler, S. MacNeil, V. Snieckus and P. Beak, Angew. Chem., Int. Ed., 2004, 43, 2206–2225 CrossRef CAS PubMed;
(b) O. Daugulis, H. Q. Do and D. Shabashov, Acc. Chem. Res., 2009, 42, 1074–1086 CrossRef CAS PubMed;
(c) K. M. Engle, T. S. Mei, M. Wasa and J. Q. Yu, Acc. Chem. Res., 2012, 45, 788–802 CrossRef CAS PubMed;
(d) X. Chen, K. Engle, D. Wang and J. Yu, Angew. Chem., Int. Ed., 2009, 48, 5094–5115 CrossRef CAS PubMed;
(e) D. Colby, R. Bergman and J. Ellman, Chem. Rev., 2010, 110, 624–655 CrossRef CAS PubMed;
(f) T. Lyons and M. Sanford, Chem. Rev., 2010, 110, 1147–1169 CrossRef CAS PubMed;
(g) G. Rouquet and N. Chatani, Angew. Chem., Int. Ed., 2013, 52, 11726–11743 CrossRef CAS;
(h) F. L. Zhang, K. Hong, T. J. Li, H. Park and J. Q. Yu, Science, 2016, 351, 252 CrossRef CAS;
(i) V. K. Tiwari and M. Kapur, Org. Biomol. Chem., 2019, 17, 1007–1026 RSC;
(j) R. Bisht and B. Chattopadhyay, J. Am. Chem. Soc., 2016, 138, 84–87 CrossRef CAS PubMed;
(k) J. He, M. Wasa, K. S. L. Chan, Q. Shao and J. Q. Yu, Chem. Rev., 2017, 117, 8754 CrossRef CAS;
(l) Y.-Q. Chen, S. Singh, Y. Wu, Z. Wang, W. Hao, P. Verma, J. X. Qiao, R. B. Sunoj and J. Q. Yu, J. Am. Chem. Soc., 2020, 142, 9966 CrossRef CAS;
(m) L. J. Xiao, K. Hong, F. Luo, L. Hu, W. R. Ewing, K. S. Yeung and J. Q. Yu, Angew. Chem., Int. Ed., 2020, 59, 9594 CrossRef CAS;
(n) P. A. Provencher, J. F. Hoskin, J. J. Wong, X. Chen, J. Q. Yu, K. N. Houk and E. J. Sorensen, J. Am. Chem. Soc., 2021, 143, 20035 CrossRef CAS;
(o) P. A. Provencher, K. L. Bay, J. F. Hoskin, K. N. Houk, J. Q. Yu and E. J. Sorensen, ACS Catal., 2021, 11, 3115 CrossRef CAS;
(p) Y.-H. Li, Y. Ouyang, N. Chekshin and J. Q. Yu, J. Am. Chem. Soc., 2022, 144, 4727 CrossRef CAS PubMed;
(q) I. Higham and J. A. Bull, Angew. Chem., Int. Ed., 2022, e202202933 Search PubMed;
(r) J.-T. Cheng, L.-J. Xiao, S.-Q. Qian, Z. Zhuang, A. Liu and J.-Q. Yu, Angew. Chem., Int. Ed., 2022, 61, e202117233 CAS.
- For examples on directing groups:
(a) V. G. Zaitsev, D. Shabashov and O. Daugulis, J. Am. Chem. Soc., 2005, 127, 13154–13155 CrossRef CAS PubMed;
(b) L. V. Desai, H. A. Malik and M. S. Sanford, Org. Lett., 2006, 8, 1141–1144 CrossRef CAS PubMed;
(c) P. J. Cabrera, M. Lee and M. S. Sanford, J. Am. Chem. Soc., 2018, 140, 5599–5606 CrossRef CAS PubMed;
(d) N. R. Deprez and M. S. Sanford, J. Am. Chem. Soc., 2009, 131, 11234–11241 CrossRef CAS;
(e) S. R. Neufeldt and M. S. Sanford, Acc. Chem. Res., 2012, 45, 936–946 CrossRef CAS PubMed;
(f) Y. Xu, M. C. Young, C. Wang, D. M. Magness and G. Dong, Angew. Chem., Int. Ed., 2016, 55, 9084–9087 CrossRef CAS PubMed;
(g) J. Wang, R. Li, Z. Dong, P. Liu and G. Dong, Nat. Chem., 2018, 10, 866–872 CrossRef CAS PubMed . For selected reviews on transient directing groups:;
(h) N. Goswami, T. Bhattacharya and D. Maiti, Nat. Rev. Chem., 2021, 5, 646–659 CrossRef CAS;
(i) C. Jacob, B. U. W. Maes and G. Evano, Chem. - Eur. J., 2021, 27, 13899–13952 CrossRef CAS;
(j) B. Zu, Y. Guo, J. Ke and C. He, Synthesis, 2021, 53, 2029–2042 CrossRef CAS;
(k) M. I. Lapuh, S. Mazeh and T. Besset, ACS Catal., 2020, 10, 12898–12919 CrossRef CAS;
(l) J. Das, S. Guin and D. Maiti, Chem. Sci., 2020, 11, 10887–10909 RSC;
(m) J. I. Higham and J. A. Bull, Org. Biomol. Chem., 2020, 18, 7291–7315 RSC;
(n) D. Bag, P. K. Verma and S. D. Sawant, Chem.–Asian J., 2020, 15, 3225–3238 CrossRef CAS PubMed;
(o) G. Liao, T. Zhang, Z. K. Lin and B.-F. Shi, Angew. Chem., Int. Ed., 2020, 59, 19773–19786 CrossRef CAS;
(p) B. Niu, K. Yang, B. Lawrence and H. Ge, ChemSusChem, 2019, 12, 2955–2969 CrossRef CAS PubMed;
(q) O. K. Rasheed and B. Sun, ChemistrySelect, 2018, 3, 5689–5708 CrossRef CAS;
(r) S. St John-Campbell and J. Bull, Org. Biomol. Chem., 2018, 16, 4582–4595 RSC;
(s) P. Gandeepan and L. Ackermann, Chem, 2018, 4, 199–222 CrossRef CAS;
(t) Z. Qun, P. Thomas, P. Xavier and B. Tatiana, Synthesis, 2017, 49, 4808–4826 CrossRef.
- For examples on TDG enabled C(sp2)–H (hetero)arylations:
(a) X.-H. Liu, H. Park, J.-H. Hu, Y. Hu, Q.-L. Zhang, B.-L. Wang, B. Sun, K.-S. Yeung, F.-L. Zhang and J.-Q. Yu, J. Am. Chem. Soc., 2017, 139, 888–896 CrossRef CAS PubMed;
(b) J. Xu, Y. Wang, Y. Li, X. Xu and Z. Jin, Org. Lett., 2017, 19, 1562–1565 CrossRef CAS;
(c) N. Thrimurtulu, A. Dey, A. Singh, K. Pal, D. Maiti and C. M. R. Volla, Adv. Synth. Catal., 2019, 361, 1441–1446 CrossRef CAS;
(d) J. Wang, C. Dong, L. Wu, M. Xu, J. Lin and K. Wei, Adv. Synth. Catal., 2018, 360, 3709–3715 CrossRef CAS;
(e) Y. Cheng, J. Zheng, C. Tian, Y. He, C. Zhang, Q. Tan, G. An and G. Li, Asian J. Org. Chem., 2019, 8, 526–531 CrossRef CAS;
(f) J. Xu, Y. Liu, J. Zhang, X. Xu and Z. Jin, Chem. Commun., 2018, 54, 689–692 RSC;
(g) G. Liao, H.-M. Chen, Y.-N. Xia, B. Li, Q.-J. Yao and B.-F. Shi, Angew. Chem., Int. Ed., 2019, 58, 11464–11468 CrossRef CAS PubMed;
(h) S. St John-Campbell, A. Ou and J. Bull, Chem, 2018, 24, 17838–17843 CrossRef CAS PubMed;
(i) B. Li, K. Seth, B. Niu, L. Pan, H. Yang and H. Ge, Angew. Chem., Int. Ed., 2018, 57, 3401–3405 CrossRef CAS;
(j) D.-Y. Wang, S.-H. Guo, G.-F. Pan, X.-Q. Zhu, Y.-R. Gao and Y.-Q. Wang, Org. Lett., 2018, 20, 1794–1797 CrossRef CAS PubMed;
(k) L.-F. Wu, J.-W. Yao, X. Zhang, S.-Y. Liu, Z.-N. Zhuang and K. Wei, Org. Lett., 2021, 23, 6237–6241 CrossRef CAS PubMed.
- For examples on TDG enabled C(sp2)–H alkylations:
(a) X.-Y. Chen and E. J. Sorensen, J. Am. Chem. Soc., 2018, 140, 2789–2792 CrossRef CAS;
(b) G. Liao, B. Li, H.-M. Chen, Q.-J. Yao, Y.-N. Xia, J. Luo and B.-F. Shi, Angew. Chem., Int. Ed., 2018, 57, 17151–17155 CrossRef CAS PubMed;
(c) H.-M. Chen, S. Zhang, G. Liao, Q.-J. Yao, X.-T. Xu, K. Zhang and B.-F. Shi, Organometallics, 2019, 38, 4022–4028 CrossRef CAS;
(d) P. W. Tan, N. A. B. Juwaini and J. Seayad, Org. Lett., 2013, 15, 5166–5169 CrossRef CAS;
(e) G. Li, J. Jijun, H. Xie and J. Wang, Chem. - Eur. J., 2019, 25, 4688–4694 CrossRef CAS;
(f) H.-M. Chen, G. Liao, C.-K. Xu, Q.-J. Yao, S. Zhang and B.-F. Shi, CCS Chem., 2021, 3, 455–465 CrossRef CAS.
- For examples on TDG enabled C(sp2)–H alkenylation:
(a) K. M. Engle and J.-Q. Yu, J. Org. Chem., 2013, 78, 8927–8955 CrossRef CAS PubMed;
(b) Q. Shao, K. Wu, Z. Zhuang, S. Qian and J.-Q. Yu, Acc. Chem. Res., 2020, 53, 833–851 CrossRef CAS PubMed;
(c) S. Bag, S. Jana, S. Pradhan, S. Bhowmick, N. Goswami, S. K. Sinha and D. Maiti, Nat. Commun., 2021, 12, 1393 CrossRef CAS PubMed;
(d) Q.-J. Yao, S. Zhang, B.-B. Zhan and B.-F. Shi, Angew. Chem., Int. Ed., 2017, 56, 6617–6621 CrossRef CAS;
(e) U. Dhawa, C. Tian, T. Wdowik, J. C. A. Oliveira, J. Hao and L. Ackermann, Angew. Chem., Int. Ed., 2020, 59, 13451–13457 CrossRef CAS PubMed.
-
(a) X.-Y. Chen, S. Ozturk and E. J. Sorensen, Org. Lett., 2017, 19, 6280–6283 CrossRef CAS PubMed;
(b) J. Jiang, D. Yuan, C. Ma, W. Song, Y. Lin, L. Hu and Y. Zhang, Org. Lett., 2021, 23, 279–284 CrossRef CAS PubMed;
(c) L. Jin, X.-L. Zhang, R.-L. Guo, M.-Y. Wang, Y.-R. Gao and Y.-Q. Wang, Org. Lett., 2021, 23, 1921–1927 CrossRef CAS PubMed.
- X. Chen, S. Ozturk and E. Sorensen, Org. Lett., 2017, 19, 6280–6283 CrossRef CAS PubMed.
-
(a) F. Li, Y. Zhou, H. Yang, Z. Wang, Q. Yu and F. Zhang, Org. Lett., 2019, 21, 3692–3695 CrossRef CAS PubMed;
(b) L. Jin, X. Zhang, R. Guo, M. Wang, Y. Gao and Y. Wang, Org. Lett., 2021, 23, 1921–1927 CrossRef CAS PubMed . During our preparation of manuscript, a related
report occurred, which form N, O-bis-coordinated complexes with Pd(II) catalyst and promote the C–H activation process. see:J. Zhou, D. Liu, C. Bai, A. Bao, T. Muschin, M. Baiyin and Y. S. Bao, Org. Chem. Front., 2021, 8, 5975–5981 RSC.
- W. Gong, G. Zhang, T. Liu, R. Giri and J. Yu, J. Am. Chem. Soc., 2014, 136, 16940–16946 CrossRef CAS PubMed.
- H. Park, P. Verma, K. Hong and J. Yu, Nat. Chem., 2018, 10, 755–762 CrossRef CAS PubMed.
- For examples on preparation of alkyl aryl ethers
(a) R. Szpera, P. G. Isenegger, M. Ghosez, N. J. W. Straathof, R. Cookson, D. C. Blakemore, P. Richardson and V. Gouverneur, Org. Lett., 2020, 22, 6573–6577 CrossRef CAS PubMed;
(b) Z. Chen, Y. Jiang, L. Zhang, Y. Guo and D. Ma, J. Am. Chem. Soc., 2019, 141, 3541–3549 CrossRef CAS;
(c) J. A. Terrett, J. D. Cuthbertson, V. W. Shurtleff and D. W. MacMillan, Nature, 2015, 524, 330–334 CrossRef CAS PubMed;
(d) K. Zhang, X.-H. Xu and F.-L. Qing, J. Fluorine Chem., 2017, 196, 24–31 CrossRef CAS;
(e) D.-Y. Wang, Z.-K. Yang, C. Wang, A. Zhang and M. Uchiyama, Angew. Chem., Int. Ed., 2018, 57, 3641–3645 CrossRef CAS PubMed;
(f) H. Zhang, P. Ruiz-Castillo and S. L. Buchwald, Org. Lett., 2018, 20, 1580–1583 CrossRef CAS PubMed;
(g) S. D. Laffoon, V. S. Chan, M. G. Fickes, B. Kotecki, A. R. Ickes, J. Henle, J. G. Napolitano, T. S. Franczyk, T. B. Dunn, D. M. Barnes, A. R. Haight, R. F. Henry and S. Shekhar, ACS Catal., 2019, 9, 11691–11708 CrossRef;
(h) J. Irurre, J. Casas and A. Messeguer, Bioorg. Med. Chem. Lett., 1993, 3, 179–182 CrossRef CAS.
- Nonfluorinated alcohols and other long chain fluorinated alcohols, such as methanol, ethanol, and 3,3,3-trifluoropropan-1-ol, not suitable substrates under the current reaction conditions, probably due to their different pka values.
|
This journal is © The Royal Society of Chemistry 2022 |
Click here to see how this site uses Cookies. View our privacy policy here.