DOI:
10.1039/D2RA00223J
(Paper)
RSC Adv., 2022,
12, 8841-8851
Copper(II) complexes with terpene derivatives of ethylenediamine: synthesis, and antibacterial, antifungal and antioxidant activity†
Received
12th January 2022
, Accepted 12th March 2022
First published on 23rd March 2022
Abstract
The synthesis of new chiral copper(II) complexes with terpene derivatives of ethylenediamine and the results of studying their antibacterial, antifungal and antioxidant activity in vitro are discussed. All studied copper complexes (1–4) showed significantly higher antifungal activity against the strains of C. albicans, S. salmonicolor and P. notatum compared to the activity of the clinical antifungal drug amphotericin. High antibacterial activity of copper complexes with terpene derivatives of ethylenediamine was revealed against the S. aureus (MRSA) strain, which is resistant to the reference antibiotic ciprofloxacin. Using various test systems, a comparative assessment of the antioxidant activity (AOA) of the synthesized copper complexes and the ligands was carried out. The salen-type complex 4, which has the highest AOA in the model of initiated oxidation of a substrate containing animal lipids, was superior to other copper complexes in the ability to protect erythrocytes under conditions of H2O2-induced hemolysis.
Introduction
Metal complexes with organic ligands are being actively investigated as pharmacological agents, some of which have already found application in medical practice.1–5 The idea of using a three-dimensional metal complex matrix to create a new drug is very promising, since a high degree of variability is possible.5 Using metals with variable valence, for example Cu(I/II), it is possible to ensure their active participation in redox biochemical processes. Moreover, ligands can stabilize one or another oxidation state of the metal. In general, by varying the nature of the metal center, it is possible to control the kinetic parameters (rate of ligand exchange) and thermodynamics (strength of metal–ligand binding, level of redox potential). The variation in the nature of the ligands is almost limitless. This also applies to the internal coordination sphere and the external one, which provides anion exchange. Organic compounds of various classes were studied as starting ligands.6 First of all, these are N-donor imines, amines, heterocyclic compounds, as well as ligands of the salen type and sulfur-containing ligands. In most works, the fact of a higher biological activity of the metal complex in comparison with the corresponding ligand was established. Metal complexes with a certain type of ligand can provide targeted pharmacological properties.
Screening for antimicrobial activity of a wide range of metal-containing compounds was carried out within the framework of an international project Community for Open Antimicrobial Drug Discovery.1 It was found that in the group of metal complexes the proportion of compounds with high efficiency is 9.9% in comparison with organic molecules (0.9%). The great interest of researchers in copper complexes is confirmed by the fact that in 2020 alone, more than 60 works were published devoted to the synthesis and study of the antimicrobial properties of these compounds. Our review is devoted to the analysis of these results.6
Copper complexes are characterized by great potential for biomedical applications, which is confirmed by numerous studies. A number of works report on the AOA of copper complexes.7–17 Recently, much attention has been paid to the development and use of copper complexes with the superoxide dismutase (SOD) mimetic activity.18–24 To test free-radical-scavenging properties of drugs is a promising protocol for enlarging the applicability of the clinic drugs.25 Copper stands out among transition metals for its remarkable affinity for biological ligands and redox properties that make it an ideal metal to drive biochemical reactions involving redox and oxygen chemistry. But the same chemical properties that make Cu a superb enzymatic co-factor render the metal potentially toxic, particularly for unicellular microbes.26
Analysis of the literature data allows us to conclude that the synthesis and study of the biological activity of copper complexes is very promising from the point of view of obtaining new pharmacological preparations. This work discusses the synthesis of chiral copper(II) complexes 1–4 with terpene derivatives of ethylenediamine and the results of studying their biological activity. Antibacterial and antifungal activity was determined by inhibiting cell growth of five bacteria types (Staphylococcus aureus, Pseudomonas aeruginosa, Mycobacterium vaccae, Bacillus subtilis, Escherichia coli) and three types of fungi (Candida albicans, Sporobolomyces salmonicolor, Penicillium notatum). These types of widespread bacteria and fungi possess multiple mechanisms of resistance to a number of clinical drugs, which makes actual the search for new bactericidal and fungicidal drugs. A comparative assessment of the AOA of the synthesized copper complexes was carried out in vitro using test systems of varying degrees of complexity (including mammalian erythrocytes).
Results and discussion
Synthesis of copper(II) complexes
Terpene ligands are successfully used in coordination chemistry for the synthesis of chiral metal complexes.27 In our work, we used terpene derivatives of ethylenediamine L1–L4 as polydentate ligands for the preparation of copper chelate complexes (Fig. 1). We have previously described the synthesis and characteristics of these compounds: L1,28 L2,29 L3,30 L4.31
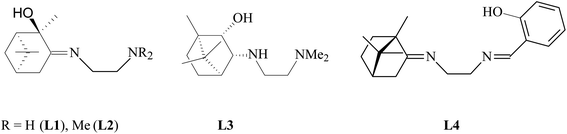 |
| Fig. 1 Ligands L1–L4 for the copper complexes synthesis. | |
The synthesis of copper complexes 1–4 (Fig. 2) was carried out by direct interaction of equimolar amounts of the starting ligand and copper chloride in methanol at room temperature. After removal of the solvent and recrystallization of the solid residue, substances were obtained in the form of powders. The structure of the obtained metal complexes was confirmed by the data of elemental analysis, mass spectrometry, and IR spectroscopy. The IR spectra show a shift in the band of stretching vibrations of the C
N bond upon passing from the free ligand to the corresponding complex, which indicates the participation of imine nitrogen in coordination. For complexes 1–4 in the region 410–490 cm−1 absorption bands are observed corresponding to stretching vibrations of Cu–N bonds. For complex 4, the participation of the phenol hydroxyl in coordination can be confirmed by the fact that the band due to O–H shifted from 3254 cm−1 (L4) to 3439 cm−1 (4). In the case of compounds 1 and 2, no such regularities were noted. The mass spectra of compounds 1–4 show the presence of peaks of molecular ions, the low intensity of which indicates the instability of the initial molecule under conditions of electron impact. The data obtained provide good agreement for the molecular formula of these complexes. To determine the metal complexes charge, the molar conductivity of complexes 1–4 measured in DMSO (10−3 M) at 25 °C. Low molar conductance values (18–35 S cm2 mol−1) suggests complexes are non-electrolytes in nature.32
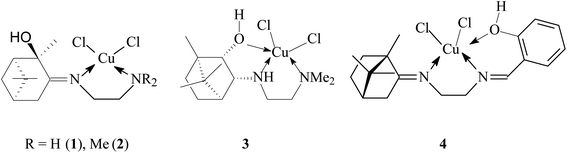 |
| Fig. 2 New copper complexes 1–4 with terpene derivatives of ethylenediamine. | |
According to X-ray diffraction (XRD) analysis, compound 3 (Fig. 3) crystallizes in a non-centrosymmetric space group, which confirms the chirality of the compound. The central copper ion coordinates two N atoms, the O atom of the OH group of the tridentate ligand L3, and two Cl atoms (coordination number 5). As a result of the coordination interaction of the Cu2+ ion with the ligand L3, two five-membered chelate rings CuC2N2 and CuC2NO are closed. In this case, the S-configuration of the chiral nitrogen N1 is fixed. The Cl2N2O coordination polyhedron has the shape of a trigonal bipyramid. This geometry for copper(II) complexes is not unique and has been noted in a number of studies.33 Angular structural parameter τ5, as general descriptor five-coordinate centric molecules,34 determined for 3. Its value is 0.6133. According to this criterion, the Cl2N2O coordination polyhedron in the solid state has a trigonal–bipyramidal like geometry.
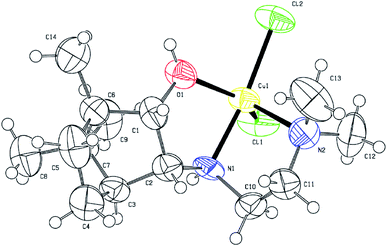 |
| Fig. 3 ORTEP drawing of the molecular structure 3. Thermal ellipsoids are shown at the 50% probability level. | |
Antibacterial and antifungal activity
Studies have shown that, in general, copper complexes 1–4 showed high antibacterial and antifungal activity (Table S1†). In particular, a high antibacterial activity of 1–4 was revealed against the methicillin-resistant S. aureus (MRSA) strain, which was also resistant to the reference antibiotic ciprofloxacin (Fig. 4). In relation to the S. aureus (511 B3) strain, the activity of complexes 1–4 is comparable to that of ciprofloxacin. All studied copper complexes (1–4) showed significantly higher antifungal activity against strains of Candida albicans, Sporobolomyces salmonicolor and Penicillium notatum compared to the activity of the clinical antifungal drug amphotericin (Fig. 5).
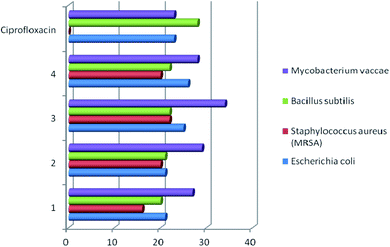 |
| Fig. 4 Antibacterial activity of copper complexes 1–4 vs. clinical antibiotic ciprofloxacin. Agar diffusion assay, inhibition zone diameter, mm. | |
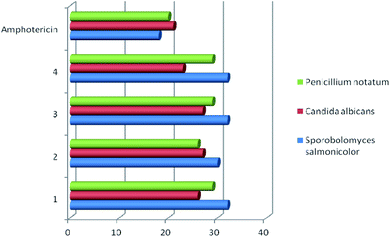 |
| Fig. 5 Antifungal activity of copper complexes 1–4 vs. clinical fungicide amphotericin. Agar diffusion assay, inhibition zone diameter, mm. | |
For comparison, the antimicrobial activity of free ligands L1–L4 was evaluated and it was found that they are inactive against the line of investigated strains of pathogenic microorganisms. The data obtained confirm the regularity established in many works – a higher antimicrobial activity of metal complexes compared to free ligands.11,19,35,36 Scientists explain this result by an increase in the lipophilicity of the Cu2+ ion due to coordination with the organic ligand. Subsequently, the penetration of the complexes into lipid membranes will be enhanced and then blocking the Cu(II) binding sites in the enzymes of microorganisms. The authors36 add that “the enhancement of the activity can occur not only due the increase of lipophilicity but alternatively it may be due to the formation of reactive oxygen species through the reduction of Cu(II) to Cu(I) in intracellular environment, which can cause the death of the microorganism”.
It is difficult to make a correct comparison of the literature data on the antimicrobial activity of copper complexes and our results. There are a lot of examples,6 however, the difficulty lies in the fact that the assessment of activity was carried out by various methods, the data are presented in various quantitative units. We have tried to make a small selection of comparable data.11,12 The results are included in the Table S1.†
A series of bivalent metal complexes 5a–c and 6a–c (Fig. S1†) with Schiff base ligands have been synthesized.12 The antimicrobial screening results displayed that the Schiff base ligands were moderately active against bacterial (P. aeruginosa, E. coli, S. aureus, B. amyloliquefaciens) and fungal (M. phaseolina, S. rolfsii) strains whereas the corresponding metal complexes 5a–c and 6a–c show higher activity than free Schiff base ligands. From the above results it is concluded that, among all, copper complexes 5c and 6c show highest potential activity against mentioned bacterial and fungal species.
Thermal reactions of Cu2+ ions with the two Schiff base ligands [N′-(1-(2-hydroxyphenyl)ethylidene)-2-phenylacetohydrazide] and [N′-((1-hydroxynaphthalen-2-yl)methylene)-2-phenylacetohydrazide] resulted in formation of the binuclear complexes 7a and 7b.11 The two ligands showed no activity towards either the bacteria (E. coli, S. aureus) or the fungi (A. flavus, C. albicans). On the other hand, the results indicated high antimicrobial activities for the complexes 7a, 7b and were found to be comparable with that of standards (ampicillin or amphotericin B). Accordingly, it could be concluded that the Cu2+ ions improved the antimicrobial activity of the compounds.
These examples indicate comparable antibacterial activity of the copper complexes 1–4 obtained by us and compounds 5c, 6c, 7a, 7b described in the literature11,12 against E. coli, P. aeruginosa, and S. aureus strains. The antifungal activity of complexes 1–4 against Candida albicans is twice as high than that established for compounds 7a and 7b.11
Antioxidant activity
For the obtained complex compounds of copper 1–4 (Fig. 2), as well as free ligands L1, L2 (Fig. 1), a comparative assessment of AOA was carried out using various test systems. We employ a common set of methods, which were successfully used by us in previous studies, to investigate the antioxidant properties of the prepared compounds.37–40
The AOA of compounds was assessed (in vitro) by the ability to inhibit lipid peroxidation (LPO) processes in a substrate containing lipids of the laboratory mice brain. In this case, two methods of LPO initiation were used – Fe2+/ascorbate or H2O2. The content of LPO secondary products reacting with 2-thiobarbituric acid (TBA-reactive substances, TBA-RS) was determined spectrophotometrically (Fig. 6 and Table S2†). From the analysis of the data obtained at a concentration of compounds of 500 μM, it follows that regardless of the method of LPO initiation in both pairs of ligand-complex, the highest inhibitory activity was observed for copper complexes. In particular, complex 1 is more active than ligand L1, complex 2 is more active than ligand L2. Copper complexes 3 and 4 are also characterized by a high AOA at the indicated concentration. Note that the results obtained upon initiation of LPO by various methods are closely correlated with each other (Spearman's correlation coefficient Rs = 0.75; significance level p = 0.05; sample size n = 7).
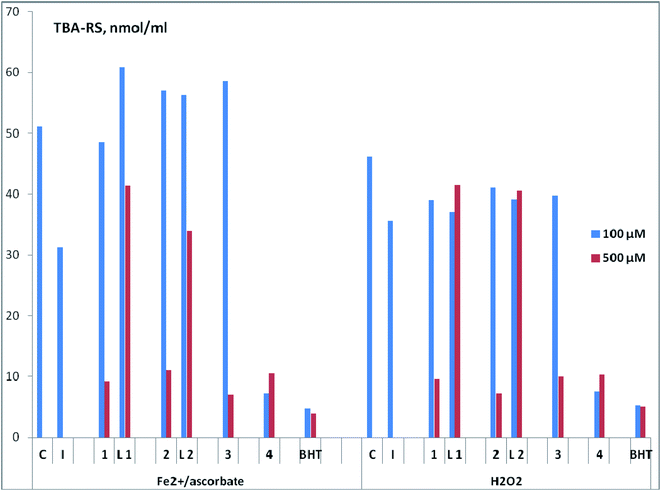 |
| Fig. 6 AOA of the test compounds (copper complexes 1–4, ligands L1 and L2) at concentrations of 100 and 500 μM. C – control without test compounds. I – intact samples (without initiated oxidation). BHT – standard antioxidant 2,6-di-tert-butyl-4-methylphenol. | |
With a decrease in concentration to 100 μM, complex 4 practically does not lose its ability to inhibit LPO and retains leading position (Fig. 6 and Table S2†). This compound is comparable in activity to the standard antioxidant 2,6-di-tert-butyl-4-methylphenol (BHT) for both methods of LPO initiation (Fe2+/ascorbate or H2O2). Structurally, the copper complex 4 has significant differences from compounds 1–3, since it contains N,N,O-donor tridentate ligand of salen type. It can be assumed that the high AOA 4 is provided by the presence of a phenolic fragment. For further research, we define this type of complexes as the most promising.
The absence of an increase (and even a slight decrease) in the antioxidant activity with an increase in the drug concentration, as is observed for complex 4, was repeatedly noted earlier for structures of another type.38,39,49 The authors attribute this to the possible pro-oxidant effect of these highly active compounds at high concentrations.
Before carrying out a comparative study of the membrane-protective activity (MPA) of compounds 1–4, L1, L2 on the model of oxidative hemolysis of erythrocytes, we assessed their toxicity by the degree of hemolysis of erythrocytes. It was established (Fig. 7 and Table S3†) that almost all tested compounds at 10 μM are characterized by low hemolytic activity – the death of erythrocytes in their presence slightly exceeds spontaneous. Only in the presence of complex 2 erythrocyte hemolysis exceeds the control values by about two times. Further studies of the biological activity of the compounds using blood cells were carried out at 1 μM.
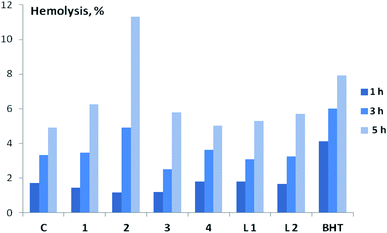 |
| Fig. 7 Hemolytic activity of the test compounds (copper complexes 1–4, ligands L1 and L2) at a concentration of 10 μM after 1, 3 and 5 h of incubation. C – control without test compounds. BHT – standard antioxidant 2,6-di-tert-butyl-4-methylphenol. | |
MPA of the tested compounds (copper complexes 1–4, ligands L1 and L2) was determined by the degree of inhibition of oxidative hemolysis in red blood cells. 2,2′-Azobis-(2-amidinopropane)hydrochloride (AAPH) or H2O2 was used to initiate oxidative hemolysis. In an aqueous medium at physiological temperatures AAPH generates the formation of peroxyl radicals that are unable to penetrate into the cell and act on the membrane from the outside.41,42 Oxidative hemolysis initiated by AAPH is widely used for the detection of AOA and MPA of various compounds and plant extracts.41–45 It was found that all studied compounds (copper complexes 1–4, ligands L1 and L2) have statistically significant MPA, which follows from the decrease in the rate of cell death in their presence under the influence of AAPH radicals (Table 1). Two hours after the induction of oxidative stress, the level of hemolysis in the control was 23.2%, in the presence of copper complexes 1–4 – only 6.2–10.9%, ligands L1 and L2 – 12.1–15.0%. The highest MPA among all studied compounds was found for complex 2, which was superior in activity not only to the corresponding ligand L2, but also to BHT, slowing down the rate of oxidative hemolysis during the experiment.
Table 1 MPA of the test compounds (copper complexes 1–4, ligands L1 and L2) at a concentration of 1 μM under the conditions of AAPH- and H2O2-induced hemolysis
Variant |
Hemolysis, % |
1 h |
2 h |
3 h |
4 h |
5 h |
C – control without test compounds. BHT – standard antioxidant 2,6-di-tert-butyl-4-methylphenol. |
AAPH-induced hemolysis |
Ca |
2.7 ± 0.0 |
23.2 ± 0.8 |
55.9 ± 0.7 |
75.6 ± 0.8 |
81.8 ± 0.4 |
1 |
2.8 ± 0.0 |
9.3 ± 0.2 |
42.4 ± 0.6 |
69.1 ± 0.8 |
81.3 ± 0.6 |
2 |
2.4 ± 0.1 |
6.2 ± 0.7 |
31.6 ± 0.1 |
62.0 ± 0.7 |
75.5 ± 0.7 |
3 |
2.8 ± 0.0 |
10.4 ± 0.6 |
47.1 ± 1.4 |
69.4 ± 1.1 |
81.2 ± 0.8 |
4 |
2.5 ± 0.0 |
10.9 ± 0.4 |
45.5 ± 0.6 |
70.8 ± 0.4 |
82.9 ± 0.4 |
L1 |
2.7 ± 0.0 |
12.1 ± 1.0 |
42.7 ± 0.6 |
66.7 ± 0.8 |
79.0 ± 0.7 |
L2 |
2.9 ± 0.2 |
15.0 ± 1.3 |
47.5 ± 1.2 |
68.7 ± 0.6 |
80.7 ± 1.7 |
BHTb |
3.0 ± 0.1 |
16.0 ± 0.8 |
46.2 ± 0.8 |
70.7 ± 0.8 |
81.0 ± 0.3 |
![[thin space (1/6-em)]](https://www.rsc.org/images/entities/char_2009.gif) |
H2O2-induced hemolysis |
C |
11.5 ± 0.7 |
23.1 ± 0.5 |
30.7 ± 0.7 |
36.7 ± 0.6 |
39.9 ± 0.4 |
1 |
7.1 ± 0.4 |
17.9 ± 0.6 |
24.8 ± 0.9 |
30.6 ± 0.8 |
34.5 ± 0.8 |
2 |
6.9 ± 0.5 |
18.5 ± 0.5 |
25.5 ± 0.3 |
29.4 ± 0.2 |
33.5 ± 0.4 |
3 |
11.0 ± 0.5 |
21.4 ± 0.6 |
29.1 ± 0.8 |
36.8 ± 0.4 |
40.5 ± 0.8 |
4 |
10.9 ± 0.6 |
16.4 ± 0.4 |
20.9 ± 0.5 |
25.0 ± 0.4 |
28.0 ± 0.7 |
L1 |
8.0 ± 0.5 |
22.6 ± 0.8 |
29.8 ± 1.5 |
34.1 ± 1.0 |
36.3 ± 1.0 |
L2 |
10.3 ± 0.6 |
21.8 ± 0.7 |
26.7 ± 0.5 |
29.7 ± 0.3 |
31.2 ± 0.3 |
BHT |
5.6 ± 0.4 |
9.2 ± 0.9 |
11.9 ± 0.5 |
18.4 ± 0.6 |
22.3 ± 1.1 |
Hydroperoxide (H2O2), along with AAPH, is also widely used to initiate oxidative hemolysis in the study of the antioxidant and membrane-protective properties of various compounds.44,46,47 Unlike AAPH, this compound easily penetrates into the erythrocyte, and its primary target is hemoglobin.42 The results of a comparative MPA assessment of the studied compounds (copper complexes 1–4, ligands L1 and L2) under H2O2-induced hemolysis are presented in Table 1. In this experiment, the highest activity among complexes 1–4 was found for the salen type compound 4, which contains a phenolic fragment and is distinguished by the highest AOA in the model of initiated oxidation of a substrate containing animal lipids.
In the study of the AOA of various compounds and extracts, the assessment of radical scavenging activity (RSA) by their ability to interact with the stable chromogen radical 2,2-diphenyl-1-picrylhydrazyl (DPPH) is widely used.48–51 In a number of works, this method was also used to characterize the AOA of copper complexes with ligands of various structures.7–12,16 In our studies, the largest RSA, comparable to that of BHT, was found for copper complexes 1 and 2, as well as their ligands L1 and L1 (Fig. 8 and Table S4†). For complex 2, this result is consistent with the data obtained in the test for inhibition of oxidative hemolysis initiated by AAPH radicals. It is possible that the high RSA of compounds 1 and 2 is due to the fact that they belong to the derivatives of the pinane series, which are more prone to oxidation reactions, in contrast to the bornane rings (3, 4).
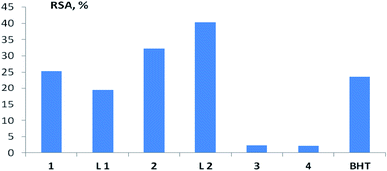 |
| Fig. 8 RSA of the test compounds (copper complexes 1–4, ligands L1 and L2) in the test with DPPH at a concentration of 100 μM. BHT – standard antioxidant 2,6-di-tert-butyl-4-methylphenol. | |
It should be noted that for the studied compounds (copper complexes 1–4, ligands L1 and L2), the RSA, assessed in the test with DPPH, does not correlate with the results obtained in biologically relevant test systems, and does not allow predicting the activity of the considered terpene ligands and their copper complexes in contact with living cells. Spearman's rank correlation coefficient (Rs) between RSA and AOA, assessed by the ability to inhibit LPO with both initiation methods, ranged from −0.18 to 0.43 (n = 7; p > 0.05), between RSA and erythrocyte survival under conditions of AAPH-induced hemolysis from −0.64 to 0.18 (n = 7; p > 0.05).
Significant differences in the results depending on the method used were noted in the study of AOA Schiff-base ligands containing carboxylate groups and their copper and nickel complexes.14 A similar result was noted for the complexes Pd(II), Fe(II), Zn(II), Mn(II), Cu(II) and VO(IV) c multifunctional Schiff base.16 Our data confirm the conclusions of other researchers41,52,53 that for a correct assessment of AOA one should not be limited to studies of RSA alone, it is desirable to use more relevant biological test systems.
Conclusion
New chelate complexes of copper(II) (1–4) with terpene derivatives of ethylenediamine have been synthesized and characterized. The structure of compound 3 was confirmed by XRD. A high antibacterial activity of copper complexes 1–4 was revealed against a methicillin-resistant strain of Staphylococcus aureus (MRSA), resistant, among other things, to the reference antibiotic ciprofloxacin. All studied copper complexes (1–4) showed significantly higher antifungal activity against the strains of Candida albicans, Sporobolomyces salmonicolor and Penicillium notatum compared to the activity of the clinical antifungal drug amphotericin. At the same time, no dependence on the structure of the initial ligand was revealed.
Using test systems of varying degrees of complexity, the AOA of copper complexes 1–4 and terpene ligands L1 and L2 were assessed. Regardless of the method of lipid peroxidation initiation (Fe2+/ascorbate or H2O2), in both ligand–complex pairs (L1-1 and L2-2), the highest inhibitory activity was observed for complexes 1 and 2. Salen type complex 4, characterized by the highest AOA in the model of initiated oxidation of a substrate containing animal lipids and showing no hemolytic activity even at a concentration of 10 μM, was superior to other copper complexes in their ability to protect erythrocytes under H2O2-induced hemolysis. It was found that all tested compounds at a concentration of 10 μM are characterized by low hemolytic activity – the death of erythrocytes in their presence slightly exceeds spontaneous, which allows them to be classified as low-toxic.
Experimental section
Synthesis of copper complexes
Materials and methods. All reagents and solvents were purchased from Sigma-Aldrich, Merck and Fluka. All solvents (ethanol, methanol, chloroform, dimethylsulfoxide, acetone, diethyl ether, hexane) were of reagent grade and used without further purification.The FT-IR spectra of the synthesized novel complexes were recorded in the 200–4000 cm−1 region on FT-IR spectrometer Shimadzu IR Prestige 21 on thin films or KBr tablets. Optical rotations were obtained with automatized digital polarimeter Optical Activity PolAAr 3001 England. Melting points were determined with Gallencamp-Sanyo apparatus. Elemental analyses were performed by using vario Micro cube apparatus. Mass spectra were recorded on a gas chromatography-mass spectrometer Shimadzu GCMS-QP2010 Plus. Molar conductance of the complexes in DMSO (10−3 M) measured by Elins Z-1000P Impedance Meter.
The following terpene derivatives of ethylenediamine were used as ligands, the synthesis of which was described by us earlier: (E)-N1-[(1R,2R,5R)-2-oxy-2,6,6-trimethylbicyclo[3.1.1]heptane-3-ylidene]ethane-1,2-diamine (L1);28 (E)-N1,N1-dimethyl-N2-[(1R,2R,5R)-2-oxy-2,6,6-trimethylbicyclo[3.1.1]heptane-3-ylidene]ethane-1,2-diamine (L2);29 N1,N1-dimethyl-N2-[(1R,2S,3R,4S)-2-oxy-1,7,7-trimethylbicyclo[2.2.1]heptane-3-yl]ethane-1,2-diamine (L3);30 (E,E)-N1-[(1S,4S)-1,7,7-trimethylbicyclo[2.2.1]heptane-2-ylidene]-N2-(2-hydroxybenzylidene)ethane-1,2-diamine (L4).31
General procedure for the synthesis of copper complexes 1–4. A solution of CuCl2·2H2O (0.5 mmol) and corresponding ligand L1–L4 (0.5 mmol) in 10 mL of methanol was stirred for 8 h at room temperature. Copper(II) complexes were obtained in the form of powders after removal of the solvent and recrystallization from a mixture of acetone – diethyl ether.
Physico-chemical and spectral characteristics of copper complexes 1–4. Dichloro{(E)-N1-[(1R,2R,5R)-2-oxy-2,6,6-trimethylbicyclo[3.1.1]heptane-3-ylidene]ethane-1,2-diamine-N1,N2}copper(II) (1): green powder; yield 84%; m.p.: 107 °C (decomp.); [α]D + 416.0 (c 0.05, EtOH); selected FT-IR data, ν, cm−1: 3229 broad (OH), 3132 broad (NH2), 1674 (C
N), 467, 457, 406 (Cu–N). Complex 1 dissolves in EtOH, DMSO. Elemental analysis (%) calcd for C12H22N2OCl2Cu·H2O: C 39.73; H 6.67; N 7.72; found: C 39.53; H 5.93; N 7.59. Molar conductance (DMSO, Λm, S cm2 mol−1): 32.4. MS (m/z): 343.2 [M]+ for C12H22N2OCl2Cu.Dichloro{(E)-N1,N1-dimethyl-N2-[(1R,2R,5R)-2-oxy-2,6,6-trimethylbicyclo[3.1.1]heptane-3-ylidene]ethane-1,2-diamine-N1,N2}copper(II) (2): green powder; yield 82%; m.p.: 86 °C (decomp.); [α]D + 487.1 (c 0.1, EtOH); selected FT-IR data, ν, cm−1: 3163 broad (OH), 1672 (C
N), 460, 432 (Cu–N). Complex 2 dissolves in EtOH, DMSO, acetone. Elemental analysis (%) calcd for C14H26N2OCl2Cu·H2O: C 43.02; H 7.22; N 7.17; found: C 43.61; H 7.10; N 7.08. Molar conductance (DMSO, Λm, S cm2 mol−1): 28.5. MS (m/z): 372.2 [M + H]+ for C14H26N2OCl2Cu.
Dichloro{N1,N1-dimethyl-N2-[(1R,2S,3R,4S)-2-oxy-1,7,7-trimethylbicyclo[2.2.1]heptane-3-yl]ethane-1,2-diamine-N1,N2,O}copper(II) (3): green powder; yield 66%; m.p.: 124 °C (decomp.); [α]D − 207.1 (c 0.04, EtOH). FT-IR, ν, cm−1: 3228 broad (OH), 3184 (NH), 443, 476 (Cu–N). Complex 3 dissolves in EtOH, DMSO. Elemental analysis calcd (%) for C14H28N2OCl2Cu: %: C 44.86; H 7.53; N 7.47; found: C 45.02; H 7.12; N 7.41. MS (m/z): 374.1 [M + H]+ for C14H28N2OCl2Cu.
Dichloro{(E,E)-N1-[(1S,4S)-1,7,7-trimethylbicyclo[2.2.1]heptane-2-ylidene]-N2-(2-hydroxybenzylidene)ethane-1,2-diamine-N1,N2,O}copper(II) (4): purple powder; yield 83%; m.p.: 182 °C (decomp.); [α]D + 68.0 (c 0.05, H2O); selected FT-IR data, ν, cm−1: 3439 broad (OH), 1695 (C
N), 1624 (C
N), 467, 422 (Cu–N). Complex 4 dissolves in H2O, DMSO. Elemental analysis (%) calcd for C19H26N2OCl2Cu: %: C 52.72; H 6.05; N 6.47; found: C 52.32; H 6.18; N 6.38. Molar conductance (DMSO, Λm, S cm2 mol−1): 34.5. MS (m/z): 432.1 [M]+ for C19H26N2OCl2Cu.
Crystal structure determination of complex 3. XRD experiment was accomplished on the base of centre collective using “SAOC” IOS UB RAS. XRD data were collected on the automated four-circle diffractometer with CCD “Xcalibur S” according to the standard procedure (MoKα-irradiation, graphite monochromator, ω-scans with 1° step at T = 295(2) K). Empirical absorption correction was applied. Using Olex2,54 the structure was solved with the SHELXS55 structure solution program using Direct Methods and refined with the SHELXL55 refinement package using Least Squares minimization in anisotropic approximation for non-hydrogen atoms. H-atoms were placed in the calculated positions and refined isotropically in the “rider” model. Crystal data for compound: crystal monoclinic, space group P21, a = 7.1957(10) Å, b = 12.0448(13) Å, c = 10.685(2) Å, β = 101.652(15)°, V = 907.0(2) Å3, μ = 1.496 mm−1. On the angles of the scattering 7.138° ≤ 2θ ≤ 61.772° 6643 reflections were collected, 4237 independent reflections (Rint = 0.0747). Final R-indexes: R1 = 0.1501, wR2 = 0.3056 (all data), R1 = 0.0985, wR2 = 0.2429 (c I > 2σ(I)), GooF = 1.028. Largest diff. peak/hole 1.77/−0.91 eÅ−3. XRD data were registered in the Cambridge Structural Database (CCDC 2113093).
τ5 = (β − α)/60° = (172.2 − 135.4)/60 = 0.6133 |
Evaluation of antimicrobial activity
The microorganisms used in this study are Pseudomonas aeruginosa (P. aeruginosa) [SG137 B7], P. aeruginosa [K799/61 B9], Escherichia coli (E. coli) [458 B4], Staphylococcus aureus (S. aureus) [511 B3], S. aureus (MRSA) [134/93 R9], Mycobacterium vaccae (M. vaccae) [10670 M4], Bacillus subtilis (B. subtilis) [6633 B1] as pathogenic bacterial strains and Sporobolomyces salmonicolor (S. salmonicolor) [549 H4], Candida albicans (C. albicans) [H8], Penicillium notatum (P. notatum) [JP36 P1] as pathogenic fungal strains.
The antimicrobial activity of Cu(II) complexes were screened by agar well diffusion method.56,57 Experimental compounds were dissolved in dimethyl sulfoxide (DMSO) at a concentration 1 mg mL−1. The bacteria were cultivated on standard I nutrient agar in Petri dishes at 37 °C. After inoculation, a disc (9 mm in diameter) was removed from the center of the Petri dish and 50 μL (corresponds to 50 μg of pure compound) of the test solution was added to the cavity. After 18 hours of incubation at the respective temperatures, growth inhibition or promotion occurred as a halo around the inoculation hole. Ciprofloxacin (5 μg mL−1 in deionized water) was used as the antibacterial reference compound. When studying the antifungal activity, the amphotericin (Amph) was used as a comparison drug. The diameter of the inhibition zones was measured (in mm) and documented as given in Table S1.† All biological assays done in triplicate.
Evaluation of the antioxidant activity
Materials and methods. In this work we used FeSO4 (Reachim Ltd, Moscow, Russian Federation), phosphate buffered saline (pH 7.4) (Sigma-Aldrich, USA); 2,2-diphenyl-1-picrylhydrazyl (CAS Number 1898-66-4, Alfa Aesar), 2,2′-azobis-(2-amidinopropane)hydrochloride (CAS Number 2997-92-4, Alfa Aesar), trichloroacetic acid (CAS Number 76-03-9, Alfa Aesar), thiobarbituric acid (CAS Number 504-17-6, Alfa Aesar), and ascorbic acid (CAS Number 50-81-7, ICN Biomedicals, USA).When studying the AOA of the compounds, we performed no experiments on animals: the analyzes were carried out exclusively in vitro. For these purposes, we used the erythrocytes and brain tissue of intact laboratory mice obtained from the scientific collection of experimental animals at the Institute of Biology, Komi SC, UB RAS (https://www.ckp-rf.ru/usu/471933/). The animals were kept in accordance with bioethical conditions (Protocol No. 1, dated 24.01.2017, approved by Academic Council of the Institute of Biology of the Komi Scientific Center of the Ural Branch of the RAS).
To study AOA, stock solutions of the studied compounds (copper complexes 1–4, ligands L1 and L2) in ethanol with a concentration of 5 mM were prepared.
Radical scavenging activity. The RSA of the compounds was evaluated by their ability to interact with the stable radical of DPPH.51 Test compound stock solution was added to 0.0015% DPPH solution in MeOH (final concentration of compounds 100 μM). Then, each mixture was shaken vigorously and kept in the dark at room temperature for 30 min. The absorption decrease was measured at λ 517 nm using a Thermo Spectromic Genesys 20 spectrophotometer. RSA was calculated as a percentage of DPPH discoloration using the following equation:
RSA, % = 100 × (1 − At/Ac), |
where At is the absorbance of the solution containing the test compound and Ac is the absorbance of the control sample with all reagents except for the compound.
The ability to inhibit lipid peroxidation. The AOA was studied (in vitro) by the ability to inhibit LPO processes in a substrate containing lipids of laboratory mice brain.58,59 Briefly, extracted brain was homogenized in physiological saline (pH = 7.4) and centrifuged. Then test compound stock solution was added to the supernatant (final concentration 100 μM or 500 μM) and LPO was initiated by FeSO4/ascorbic acid or H2O2. Samples were stirred gently for 1 h at 37 °C in a thermostatic shaker Biosan ES-20. Content of LPO secondary products reacting with TBA (TBA-RS, nmol mL−1) was determined spectrophotometrically at λ = 532 nm using the extinction coefficient of 1.56 × 105 M−1 cm−1.60
Hemolytic activity. Toxicity, AOA and MPA of the tested compounds (copper complexes 1–4, ligands L1 and L2), were investigated using a 0.5% (v/v) suspension of laboratory mice red blood cells (RBCs) in phosphate buffered saline (pH 7.4). The compounds ability to induce hemolysis defined their toxicity (in vitro). The solution of tested compound was added to a suspension of erythrocytes (final concentration 10 μM) and incubated at 37 °C for 5 h in a shaker Biosan ES-20. After 1, 3 and 5 h of incubation an aliquot was taken from the suspension, centrifuged for 5 min (1600 g); hemolysis was determined by the hemoglobin content in the supernatant on Thermo Spectromic Genesys 20 spectrophotometer at λ = 541 nm. The percentage of hemolysis was calculated relative to complete hemolysis of the sample, where it was triggered by addition of distilled water.MPA was determined by the degree of inhibition of oxidative hemolysis in RBCs. For this purpose, 30 min after the test compounds solution was added to the of the erythrocyte suspension hemolysis was initiated with H2O2 (0.006%) or AAPH (3 mM). Then the reaction mixture was incubated in a thermostatic shaker Biosan ES-20 with slow stirring at 37 °C temperature for 5 hours. Each hour an aliquot was taken from the incubated samples, centrifuged, hemolysis was determined by the hemoglobin content in the supernatant on Thermo Spectromic Genesys 20 spectrophotometer at λ = 524 nm.41
Statistical analysis. Each experiment was carried out in 4–11 replicates. Statistical analysis was done using Microsoft Office Excel 2007 and Statistica 6.0 software package. Experimental results are presented as arithmetic means with standard errors. Regression analyses were performed, and Spearman's rank correlation coefficient was calculated in order to evaluate the interrelation between certain parameters.
Conflicts of interest
There are no conflicts to declare.
Acknowledgements
The compounds synthesized were analysed using the equipment of the “Chemistry” Centre for Collective Use at the Institute of Chemistry, Komi Scientific Centre, Ural Branch of the Russian Academy of Sciences and the Centre of collective using “SAOC” IOS UB RAS. The assays of antioxidant and hemolytic activity were performed using the equipment of the Centre of Collective Usage “Molecular Biology”, Institute of Biology, Komi Scientific Centre, Ural Branch of the RAS. This work was financially supported by the Ministry of Science and Higher Education of the Russian Federation (state assignments No. AAAA-A21-121011190042-0, AAAA-A18-118011120004-5). This work has been supported by Russian Foundation for Basic Research (RFBR), project number 20-03-00027.
References
- A. Frei, J. Zuegg, A. G. Elliott, M. V. Baker, S. Braese and C. Brown, Metal Complexes as a Promising Source for New Antibiotics, Chem. Sci., 2020, 11, 2627–2639, 10.1039/c9sc06460e.
- S. Nasiri and F. Zobi, Recent Studies on the Antimicrobial Activity of Transition Metal Complexes of Groups 6–12, Chemistry, 2020, 2(2), 418–452, DOI:10.3390/chemistry2020026.
- C. N. Morrison, K. E. Prosser, R. W. Stokes, A. Cordes, N. Metzler-Nolte and S. M. Cohen, Expanding Medicinal Chemistry into 3D Space: Metallofragments as 3D Scaffolds for Fragment-Based Drug Discovery, Chem. Sci., 2020, 11, 1216–1225, 10.1039/c9sc05586j.
- K. J. Franz and N. Metzler-Nolte, Introduction: Metals in Medicine, Chem. Rev., 2019, 119, 727–729, DOI:10.1021/acs.chemrev.8b00685.
- K. D. Mjos and C. Orvig, Metallodrugs in Medicinal Inorganic Chemistry, Chem. Rev., 2014, 114, 4540–4563, DOI:10.1021/cr400460s.
- O. A. Zalevskaya and Y. A. Gur’eva, Recent Studies on the Antimicrobial Activity of Copper Complexes, Russ. J. Coord. Chem., 2021, 47, 861–880, DOI:10.1134/s1070328421120046.
- D. C. Onwudiwe and A. C. Ekennia, Synthesis, characterization, thermal, antimicrobial and antioxidant studies of some transition metal dithiocarbamates, Res. Chem. Intermed., 2017, 43, 1465–1485, DOI:10.1007/s11164-016-2709-2.
- N. Ribeiro, S. Roy, N. Butenko, I. Cavaco, T. Pinheiro, I. Alho, F. Marques, F. Avecilla, J. Costa Pessoa and I. Correia, New Cu(II) complexes with pyrazolyl derived Schiff base ligands: Synthesis and biological evaluation, J. Inorg. Biochem., 2017, 174, 63–75, DOI:10.1016/j.jinorgbio.2017.05.011.
- N. Ganji, R. Aveli, V. Narendrula and D. S. Sreenu, Copper(II) complexes with isoxazole Schiff bases: Synthesis, spectroscopic investigation, DNA binding and nuclease activities, antioxidant and antimicrobial studies, J. Mol. Struct., 2018, 1173, 173–182, DOI:10.1016/j.molstruc.2018.06.100.
- S. D. Oladipo, B. Omondi and C. Mocktar, Synthesis and structural studies of nickel(II)- and copper(II)-N,N,O-diarylformamidine dithiocarbamate complexes as antimicrobial and antioxidant agents, Polyhedron, 2019, 170, 712–722, DOI:10.1016/j.poly.2019.06.038.
- S. M. El-Medani, A. M. Abdelmoneim, M. Hussein, A. A. Manal, H. Matti and R. M. Ramadan, Spectroscopic, crystal structural, theoretical and biological studies of phenylacetohydrazide Schiff base derivatives and their copper complexes, J. Mol. Struct., 2020, 1208, 127860, DOI:10.1016/j.molstruc.2020.127860.
- G. Ramesh, S. Daravath, N. Ganji, A. Rambabu, K. Venkateswarlu and Shivaraj, Facile synthesis, structural characterization, DNA binding, incision evaluation, antioxidant and antimicrobial activity studies of Cobalt(II), Nickle(II) and Copper(II) complexes of 3-amino-5-(4-fluorophenyl) isoxazole derivatives, J. Mol. Struct., 2020, 1202, 127338, DOI:10.1016/j.molstruc.2019.127338.
- G. Psoma, Copper(II) and zinc(II) coordination compounds of non-steroidal anti-inflammatory drugs: Structural features and antioxidant activity, Coord. Chem. Rev., 2020, 412, 213259, DOI:10.1016/j.ccr.2020.213259.
- A. Boussadia, A. Beghidja, L. Gali, C. Beghidja, M. Elhabiri, P. Rabu and G. Rogez, Coordination properties of two new Schiff-base phenoxy-carboxylates and comparative study of their antioxidant activities, Inorg. Chim. Acta, 2020, 508, 119656, DOI:10.1016/j.ica.2020.119656.
- J. F. Guerreiro, M. A. G. B. Gomes, F. Pagliari, J. Jansen, M. G. Marafioti, C. Nistico, R. Hanley, R. O. Costa, S. S. Ferreira, F. Mendes, C. Fernandes, A. Horn, L. Tirinato and J. Seco, Iron and copper complexes with antioxidant activity as inhibitors of the metastatic potential of glioma cells, RSC Adv., 2020, 10, 12699, 10.1039/d0ra00166j.
- M. A. Said, A. Al-unizi, M. Al-Mamary, S. Alzahrani and D. Lentz, Easy coordinate geometry indexes, τ4 and τ5 and HSA study for unsymmetrical Pd(II), Fe(II), Zn(II), Mn(II), Cu(II) and VO(IV) complexes of a tetradentate ligand: Synthesis, characterization, properties, and antioxidant activities, Inorg. Chim. Acta, 2020, 505, 119434, DOI:10.1016/j.ica.2020.119434.
- I.-E. Boulguemh, A. Beghidja, L. Khattabi, J. Long and C. Beghidja, Monomeric and dimeric copper(II) complexes based on bidentate N′-(propan-2-ylidene) thiophene carbohydrazide Schiff-base ligand: Synthesis, structure, magnetic properties, antioxidant and anti-Alzheimer activities, Inorg. Chim. Acta, 2020, 507, 119519, DOI:10.1016/j.ica.2020.119519.
- A. K. Patel, R. N. Jadeja, H. Roy, R. N. Patel, S. K. Patel, R. J. Butcher, M. Cortijo and S. Herrero, Copper(II) hydrazone complexes with different nuclearities and geometries: Synthesis, structural characterization, antioxidant SOD activity and antiproliferative properties, Polyhedron, 2020, 186, 114624, DOI:10.1016/j.poly.2020.114624.
- A. Sakthivel, B. Thangagiri, N. Raman, J. Joseph, R. Guda, M. Kasula and L. Mitu, Spectroscopic, SOD, anticancer, antimicrobial, molecular docking and DNA binding properties of bioactive VO(IV), Cu(II), Zn(II), Co(II), Mn(II) and Ni(II) complexes obtained from 3-(2-hydroxy-3-methoxybenzylidene)pentane-2,4-dione, J. Biomol. Struct. Dyn., 2021, 39, 6500–6514, DOI:10.1080/07391102.2020.1801508.
- J. Valentová, S. Varényi, P. Herich, P. Baran, A. Bilková, J. Kožíšek and L. Habala, Synthesis, structures and biological activity of copper(II) and zinc(II) Schiff base complexes derived from aminocyclohexane-1-carboxylic acid. New type of geometrical isomerism in polynuclear complexes, Inorg. Chim. Acta, 2018, 480, 16–26, DOI:10.1016/j.ica.2018.04.058.
- D. Mo, J. Shi, D. Zhao, Y. Zhang, Y. Guan, Y. Shen, H. Bian, F. Huang and S. Wu, Synthesis and characterization of Fe/Co/Cu complexes with Schiff base ligand and their hybrid proteins, SOD activity and asymmetric catalytic oxidation of sulfides, J. Mol. Struct., 2021, 1223, e129229, DOI:10.1016/j.molstruc.2020.129229.
- M. Simunkova, P. Lauro, K. Jomova, L. Hudecova, M. Danko, S. Alwasel, I. M. Alhazza, S. Rajcaniova, Z. Kozovska, L. Kucerova, J. Moncol, L. Svorc and M. Valko, Redox-cycling and intercalating properties of novel mixed copper(II) complexes with non-steroidal anti-inflammatory drugs tolfenamic, mefenamic and flufenamic acids and phenanthroline functionality: Structure, SOD-mimetic activity, interaction with albumin, DNA damage study and anticancer activity, J. Inorg. Biochem., 2019, 194, 97–113, DOI:10.1016/j.jinorgbio.2019.02.010.
- Y. P. Singh and S. K. Patel, Molecular structures, spectral, electrochemical, DFT and antioxidant activities of copper(II) complexes with NNO donor Schiff base ligand, J. Mol. Struct., 2021, 1228, e129457, DOI:10.1016/j.molstruc.2020.129457.
- J. D. Siqueira, S. F. de Pellegrin and S. S. dos Santos, SOD activity of new copper (II) complexes with ligands derived from pyridoxal and toxicity in Caenorhabditis elegans, J. Inorg. Biochem., 2020, 204, e110950, DOI:10.1016/j.jinorgbio.2019.110950.
- Z. Q. Liu, Bridging free radical chemistry with drug discovery: A promising way for finding novel drugs efficiently, Eur. J. Med. Chem., 2020, 189, 112020, DOI:10.1016/j.ejmech.2019.112020.
- E. M. Culbertson and V. C. Culotta, Copper in infectious disease: Using both sides of the penny, Semin. Cell Dev. Biol., 2021, 115, 19–26, DOI:10.1016/j.semcdb.2020.12.003.
- O. A. Zalevskaya, Y. A. Gur’eva and A. V. Kutchin, Terpene ligands in the coordination chemistry: synthesis of metal complexes, stereochemistry, catalytic properties and biological activity, Russ. Chem. Rev., 2019, 88(10), 979–1012, DOI:10.1070/rcr4880.
- I. A. Dvornikova, E. V. Buravlev, L. L. Frolova, Y. V. Nelyubina, I. Y. Chukicheva and A. V. Kuchin, Synthesis of 1,2-diamine ligands based on natural monoterpenoids, Russ. J. Org. Chem., 2011, 47, 1130–1138, DOI:10.1134/s1070428011080021.
- Y. A. Gur'eva, I. N. Alekseev, A. V. Kutchin, O. A. Zalevskaya and P. A. Slepukhin, Synthesis of new bidentate ligands-terpene derivatives of ethylenediamine and their palladium complexes, Russ. J. Org. Chem., 2016, 52, 781–784, DOI:10.1134/s107042801606004x.
- O. A. Zalevskaya, Y. A. Gur'eva, A. V. Kutchin, Y. R. Aleksandrova, E. Y. Yandulova, N. S. Nikolaeva and M. E. Neganova, Palladium complexes with terpene derivatives of ethylenediamine and benzylamine: synthesis and study of antitumor properties, Inorg. Chim. Acta, 2021, 527, 120593, DOI:10.1016/j.ica.2021.120593.
- Y. A. Gur'eva, O. A. Zalevskaya, I. N. Alekseev, P. A. Slepukhin and A. V. Kutchin, Synthesis of new chiral palladium complexes with multidentate camphor Schiff bases, Russ. J. Org. Chem., 2018, 54, 1285–1289, DOI:10.1134/s1070428018090026.
- W. J. Geary, The use of conductivity measurements in organic solvents for the characterization of coordination compounds, Coord. Chem. Rev., 1971, 7, 81–122, DOI:10.1016/s0010-8545(00)80009-0.
- L. A. Bryleva, L. A. Glinskaya, K. S. Marenin, A. S. Bogomyakov, D. A. Piryazev, A. V. Tkachev and S. V. Larionov, Copper(II) complexes with chiral ligands containing fragments of monoterpenoids and amino acid esters, Russ. J. Coord. Chem., 2018, 44, 117–126, DOI:10.1134/s1070328418020033.
- A. W. Addison, N. T. Rao, J. Reedijk, J. van Rijn and G. C. Verschoor, Synthesis, structure, and spectroscopic properties of copper(II) compounds containing nitrogen–sulphur donor ligands; the crystal and molecular structure of aqua[1,7-bis(N-methylbenzimidazol-2′-yl)-2,6-dithiaheptane]copper(II) perchlorate, J. Chem. Soc., Dalton Trans., 1984, 7, 1349–1356, 10.1039/dt984000.613301349.
- A. T. Gordon, O. O. Abosede, S. Ntsimango, S. van Vuuren, E. C. Hosten and A. S. Ogunlaja, Synthesis, characterization, molecular docking and antimicrobial activity of copper(II) complexes of metronidazole and 1,10-phenanthroline, Inorg. Chim. Acta, 2020, 510, 119744, DOI:10.1016/j.ica.2020.119744.
- P. H. O. Santiago, F. S. Tiago, M. S. Castro, P. E. N. Souza, J. B. I. Martins and C. C. Gatto, DFT analysis, spectroscopic study and biological activity of a newly synthesized benzoylhydrazone binuclear Cu(II) complex, J. Inorg. Biochem., 2020, 204, 110949, DOI:10.1016/j.jinorgbio.2019.110949.
- E. V. Buravlev, O. G. Shevchenko, A. A. Anisimov and K. Y. Suponitsky, Novel Mannich bases of α- and γ-mangostins: synthesis and evaluation of antioxidant and membrane-protective activity, Eur. J. Med. Chem., 2018, 152, 10–20, DOI:10.1016/j.ejmech.2018.04.022.
- A. V. Samet, O. G. Shevchenko, V. V. Rusak, E. M. Chartov, A. Myshlyavtsev, D. Rusanov, M. N. Semenova and V. V. Semenov, Antioxidant Activity of Natural Allylpolyalkoxybenzene Plant Essential Oil Constituents, J. Nat. Prod., 2019, 82, 1451–1458, DOI:10.1021/acs.jnatprod.8b00878.
- I. S. Martakov, O. G. Shevchenko, M. A. Torlopov and P. A. Sitnikov, Immobilization of natural phenolic acids on AlOOH nanoparticles: effect on antioxidant, membrane-protective activity and colloid-chemical properties, J. Mol. Struct., 2022, 1248, 131471, DOI:10.1016/j.molstruc.2021.131471.
- M. A. Torlopov, O. G. Shevchenko, I. Y. Chukicheva and E. V. Udoratina, Effective low cytotoxic cell membranes protector based on amphiphilic conjugate of cellulose sulfate with isobornylphenol, React. Funct. Polym., 2020, 156, 104740, DOI:10.1016/j.reactfunctpolym.2020.104740.
- J. Takebayashi, A. Chen and A. Tai, A method for evaluation of antioxidant activity based on inhibition of free radical-induced erythrocyte hemolysis, in Advanced protocols in oxidative stress II. Methods in Molecular Biology (Methods and Protocols), ed. D. Armstrong, Humana Press, Totowa, NJ, 2010, 594, pp. 287–296, DOI:10.1007/978-1-60761-411-1_20.
- F. N. Ko, G. Hsiao and Y. H. Kuo, Protection of oxidative hemolysis by demethyl diisoeugenol in normal and β-thalassemic red blood cells, Free Radical Biol. Med., 1997, 22, 215–222, DOI:10.1016/s0891-5849(96)00295-x.
- C. G. Zou, N. S. Agar and G. L. Jones, Oxidative insult to human red blood cells induced by free radical initiator AAPH and its inhibition
by a commercial antioxidant mixture, Life Sci., 2001, 69, 75–86, DOI:10.1016/s0024-3205(01)01112-2.
- C. S. S. S. Reddy, M. V. V. Subramanyam, R. Vani and S. A. Devi, In vitro models of oxidative stress in rat erythrocytes: Effect of antioxidant supplements, Toxicol. In Vitro, 2007, 21, 1355–1364, DOI:10.1016/j.tiv.2007.06.010.
- E. Niki, Y. Yamamoto, M. Takahashi, K. Yamamoto, Y. Yamamoto, E. Komuro, M. Miki and M. Mino, Free radical-mediated damage of blood and its inhibition by antioxidants, J. Nutr. Sci. Vitaminol., 1988, 34, 507–512, DOI:10.3177/jnsv.34.507.
- C. M. Ajila and P. U. J. S. Rao, Protection against hydrogen peroxide induced oxidative damage in rat erythrocytes by Mangifera indica L. peel extract, Food Chem. Toxicol., 2008, 46, 303–309, DOI:10.1016/j.fct.2007.08.024.
- S. Rocha, E. Costa, S. Coimbra, H. Nascimento, C. Catarino, P. Rocha-Pereira, A. Quintanilha, L. Belo and A. Santos-Silva, Linkage of cytosolic peroxiredoxin 2 to erythrocyte membrane imposed by hydrogen peroxide-induced oxidative stress, Blood Cells, Mol., Dis., 2009, 43, 68–73, DOI:10.1016/j.bcmd.2009.03.002.
- M. N. Alam, N. J. Bristi and M. Rafiquzzaman, Review on in vivo and in vitro methods evaluation of antioxidant activity, Saudi Pharm. J., 2013, 21, 143–152, DOI:10.1016/j.jsps.2012.05.002.
- M. Carocho and I. C. F. R. Ferreira, A review on antioxidants, prooxidants and related controversy: Natural and synthetic compounds, screening and analysis methodologies and future perspectives, Food Chem. Toxicol., 2013, 51, 15–25, DOI:10.1016/j.fct.2012.09.021.
- E. D. Cömert and V. Gökmen, Evolution of food antioxidants as a core topic of food science for a century, Food Res. Int., 2018, 105, 76–93, DOI:10.1016/j.foodres.2017.10.056.
- B. T. Sevgi and C. Sarikurkcu, Antioxidant and DNA damage protection potentials of selected phenolic acids, Food Chem. Toxicol., 2015, 77, 12–21, DOI:10.1016/j.fct.2014.12.006.
- J. Tabart, C. Kevers, J. Pincemail, J.-O. Defraigne and J. Dommes, Comparative antioxidant capacities of phenolic compounds measured by various tests, Food Chem., 2009, 113, 1226–1233, DOI:10.1016/j.foodchem.2008.08.013.
- E. Niki, Assessment of Antioxidant Capacity in vitro and in vivo, Free Radical Biol. Med., 2010, 49, 503–515, DOI:10.1016/j.freeradbiomed.2010.04.016.
- O. V. Dolomanov, L. J. Bourhis, R. J. Gildea, J. A. K. Howard and H. Puschmann, OLEX2: a complete structure solution, refinement and analysis program, J. Appl. Crystallogr., 2009, 42, 339–341, DOI:10.1107/s0021889808042726.
- G. M. Sheldrick, A short history of SHELX, Acta Crystallogr., Sect. A: Found. Crystallogr., 2008, 64, 112–122, DOI:10.1107/s0108767307043930.
- W. W. Davis and T. R. Stout, Disc plate method of microbiological antibiotic assay: I. Factors influencing variability and error, Appl. Microbiol., 1971, 22, 659–665, DOI:10.1128/am.22.4.659-665.1971.
- W. W. Davis and T. R. Stout, Disc plate method of microbiological antibiotic assay. II. Novel procedure offering improved accuracy, Appl. Microbiol., 1971, 22, 666–670, DOI:10.1128/am.22.4.666-670.1971.
- S. N. Lim, P. C. K. Cheung, V. E. C. Ooi and P. O. Ang, Evaluation of Antioxidative Activity of Extracts from a Brown Seaweed, Sargassum siliquastrum, J. Agric. Food Chem., 2002, 50, 3862–3866, DOI:10.1021/jf020096b.
- S. T. Stefanello, A. S. Prestes, T. Ogunmoyole, S. M. Salman, R. S. Schwab, C. R. Brender, L. Dornelles, J. B. T. Rocha and F. A. A. Soares, Evaluation of in vitro antioxidant effect of new mono and diselenides, Toxicol. In Vitro, 2013, 27, 1433–1439, DOI:10.1016/j.tiv.2013.03.001.
- T. Asakawa and S. Matsushita, Coloring conditions of thiobarbituric acid test for detecting lipid hydroperoxides, Lipids, 1980, 15, 137–140, DOI:10.1007/bf02540959.
Footnote |
† Electronic supplementary information (ESI) available. CCDC 2113093. For ESI and crystallographic data in CIF or other electronic format see DOI: 10.1039/d2ra00223j |
|
This journal is © The Royal Society of Chemistry 2022 |
Click here to see how this site uses Cookies. View our privacy policy here.