DOI:
10.1039/D1RA08874B
(Paper)
RSC Adv., 2022,
12, 3365-3371
Synthesis and properties of wax based on waste cooking oil†
Received
6th December 2021
, Accepted 14th January 2022
First published on 25th January 2022
Abstract
In this work, a cost-effective wax was synthesized from waste cooking oil (WCO), and its properties including melting point, color, hardness, combustion performance and micro-morphology were tested and analyzed. The obtained results showed that the epoxy waste cooking oil had lighter color, higher melting point and hardness than that of original WCO, which could be used as wax. Moreover, introducing stearic acid further improved the performances of WCO-based wax. The WCO-based wax made of epoxy waste cooking oil and stearic acid (containing ≥50 wt% stearic acid) displayed a relatively high melting point (≥46 °C), light color (Lovibond color code Y ≤ 16.1, R ≤ 2.3), good hardness (needle penetration index ≤2.95 mm) and long combustion time (≥227 min), and could achieve the required national standard and be used as a substitute for the commercially available soybean wax. Together with many additional benefits such as low synthesis cost, mild reaction conditions, convenient synthesis route, and no secondary pollution, producing wax based on WCO could provide a new path for WCO recycling in economically trailing regions.
1. Introduction
Waste cooking oil (WCO) is one of the inedible waste resources which pollute the environment, causing serious harm to human health, and affecting quality of life.1,2 This indicates that standardized management of WCO has to be set out to harness its impacts. In the last two decades, several recycling and WCO utilization methods have been developed and a variety of products including biodiesel,3–6 biolubricants,7,8 alkyd resins,9 washing products,10,11 and others were reported. Among these, biodiesel production from WCO has become the most effective way for WCO utilization.12 The low cost, environmental impacts, and availability of WCO make WCO-based biodiesel more economical relative to other biodiesels produced from natural oil sources such as palm oil, soybean oil, peanut oil and olive oil.13–16 Furthermore, WCO-based biodiesel production requires lower energy consumption and emits minimum green-house gas as reported in the literature.17–19
However, there are various factors which became bottlenecks for large-scale production of WCO-to-biodiesel. The first challenge is associated to the raw materials supply since sustainable and massive source is required to scale-up the biodiesel manufacture. Since WCO sources such as restaurants, hotels, and house-holds are spread all over the city, establishing a stable raw material supply is not an easy job especially in economically backward regions due to absence of strong policy, financial support, and optimum supply chain.20,21 The requirement of high quality oil, with fewer free fatty acids, and appropriate fatty acid compositions,22–25 can also be stated as another challenge in massive biodiesel production. Due to their unsteady components, not all WCO are suitable for biodiesel production, even those WCO as biodiesel's feedstock require cumbersome pre-treatment steps such as decolorization, deodorization, degumming, filtration, clarification or dehydration, which not only increases the production cost, but also affect the quality of the product.26 Catalysts are also required for large-scale biodiesel production.3 Utilizing homogeneous catalyst may lead to more by-products formation and difficult to separate,2,13,27 while the heterogeneous catalyst often require high calcination temperature, long preparation time, and related complex equipments.17,28–30 Enzyme catalyst has good catalytic efficiency but needs very high preparation cost.31,32 Overall, large-scale biodiesel production is suitable for economically strong areas but not of good choice where the oil source and economy are scarce.14 Therefore, a simple, convenient, and low-cost method has to be developed for the conversion of WCO-to-biodiesel. Particularly, synthetic strategies which use low-quality WCO and feasible in economically lagging regions, such as producing solid alcohol using low iodine value WCO have to be promoted.33
On the other hand, plant wax materials, such as SW, can be used to produce essential oil candles, crayons or wax figures, which are considered to be renewable and biodegradable alternatives to paraffin wax with broad market prospects.34,35 Compared to biodiesel, plant wax material has relatively smaller market share, require simple equipment and flexible production control. Therefore, WCO-to-wax conversion may be one of the advantageous ways of WCO recycling. At present, most of commercial plant waxes are the hydrogenation products of plant oil. However, hydrogenation of plant oil usually needs relatively high temperature (>300 °C), high H2 pressure (>1 MPa), expensive catalysts and safety facilities, which surge the investment and production costs and hence, not suitable for WCO-based wax production with small market scale,36–39 which indicate that developing a low-cost WCO-to-wax conversion technique is necessary.
Epoxidation is also proved to be an effective way of plant oil modification, which can be carried out under gentle reaction conditions (<100 °C and atmospheric pressure).40–43 In this study, a new “epoxidation & combination” method of producing low-cost WCO-based wax was proposed for the first time. In this approach, WCO was epoxidized to form E-WCO, and then combined with special modifier, SA, to form cost-effective wax material that can replace the commercial SW (Fig. 1). The melting point, color, hardness, combustion performance and micro-morphology of the obtained WCO-based wax were tested and analyzed. It has to be also mentioned that, synthesis of wax using WCO as a precursor has not been reported before to the best of the researchers' knowledge.
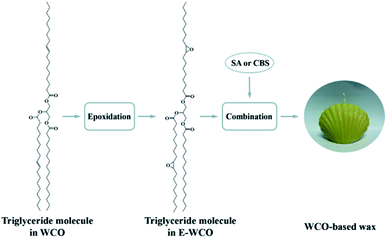 |
| Fig. 1 Schematic diagram of the synthesis of WCO-based wax. WCO = waste cooking oil; E-WCO = epoxy waste cooking oil; SA = stearic acid; CBS = cocoa butter substitute. | |
2. Experiment section
2.1 Materials
The WCO (iodine value: 45.20) was collected from ZhengXin Chicken fast food restaurant in Guilin, China. The CBS (96% purity, iodine value: 5.39), hydrogen peroxide (analytical grade, 30 wt% in H2O) and sulfuric acid (analytical grade) were purchased from Xiya Chemical Technology Co., Ltd (Shandong, China). The SA (98% purity), glacial acetic acid (99.5% purity), urea (99% purity), and sodium bicarbonate (99.5% purity) were purchased from McLean Company (Shanghai, China). The commercial SW (iodine value: 49.68) was purchased from Xu's wax Co., Ltd (Hebei, China).
2.2 Synthesis of wax based on WCO
Raw oil with low iodine value is required to synthesize WCO-based wax. Thus, the collected WCO was treated by centrifugation as reported in our previous report33 to obtain a processed WCO (iodine value: 20.24) as a raw material for subsequent preparations. The obtained WCO was a kind of dark brown soft solid with rancidity odor.
The processed WCO was then epoxidized to generate E-WCO as follows. At first, 620 g 30% H2O2, 160 g glacial acetic acid, 4 g concentrated sulfuric acid and 4 g urea were mixed in a lightproof container, and placed in an oven at 40 °C for 12 h to prepare an epoxidation reagent. Then, 1000 g WCO was placed in a glass stirred reactor and heated at 40 °C, and the initially prepared epoxidation reagent was slowly added to the reactor within 2 h. After that, the reaction mixture was heated to 70 °C and further stirred at this temperature for 3 h. The mixture was allowed to cool, kept over 12 h, and layered. Then, the upper oil layer was collected, washed once with 5 wt% sodium bicarbonate solution and twice with deionized water at 60 °C, and evaporated at 70 °C under vacuum for 2 h. After cooling, the E-WCO was obtained as a kind of odorless light yellow solid with an epoxy value of 2.56.
Finally, the obtained E-WCO was mixed with SA or CBS in a mass ratio of 1
:
3, 1
:
1 or 3
:
1 (Table 1), and then heated at 80 °C to form a homogeneous light yellow melt which can be poured into mold to shape. After cooling and demoulding, products of WCO-based wax, including candle, crayon or wax figure were obtained (Fig. 2). The synthesis recipe of wax based on WCO is listed in Table 1.
Table 1 Synthesis recipe of wax based on WCOa
Code |
E-WCO (g) |
SA (g) |
CBS (g) |
Note: SA = stearic acid; CBS = cocoa butter substitute; A1-A3 represent WCO-based wax samples with different amounts of SA; B1–B3 represent WCO-based wax samples with different amounts of CBS. |
A1 |
120 |
40 |
— |
A2 |
80 |
80 |
— |
A3 |
40 |
120 |
— |
B1 |
120 |
— |
40 |
B2 |
80 |
— |
80 |
B3 |
40 |
— |
120 |
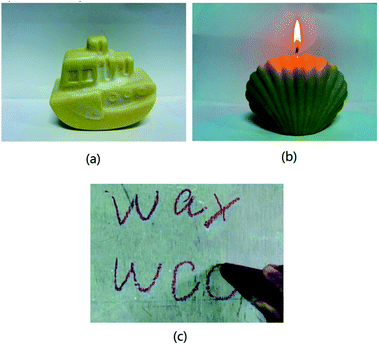 |
| Fig. 2 The photographs of a ship-like ornament (a), a shell-like candle (b), and a red crayon (containing 0.5 wt% carmine) (c) produced by WCO-based wax (A2 sample). | |
2.3 Characterization
The iodine value of the oil and the epoxy value of the E-WCO were tested according to the China Standards GB/T 5532-2008 and GB/T 1677-2008, respectively. The melting point of the wax was tested by an X-5 microscope melting point detector. The Lovibond color of the waxes was tested by a LABO-HUB WSL-2 Lovibond tintometer. The needle penetration index of the wax product was recorded by a FY-2801 C penetrometer at 25 °C. The IR spectra was recorded as KBr pellets at a range of 400–4000 cm−1 on a Nicolet 5700 FT-IR spectrometer with a spectral resolution of 4.00 cm−1. The micro-morphology image of the wax was recorded on a Scope A1 microscope with transmission mode. The powder X-ray diffraction (PXRD) pattern of the wax was obtained with X'Pert PRD diffractometer with Cu Kα radiation (λ = 1.54056 Å) at 40 kV and 40 mA at a scan speed of 4° min−1 (2θ). The moisture content of the wax was tested with XY-100 MW-1 moisture content tester.
The combustion performance of the wax was carried out using a cube candle (size: 5 × 5 × 5 cm). Such candles were repeatedly prepared for several parallel tests. The candle was lit in an open, breezeless environment without lateral support. The combustion time of the candle from ignition to burnout was recorded. Each of the combustion times presented in this paper represents the average measurement of four samples having the same composition.
3. Results and discussion
3.1 Melting point
As shown in Fig. 3 and Table S1 in ESI,† as raw material, the WCO itself is a soft solid at room temperature with low melting point ranged from 34–37 °C. It is difficult to maintain its shape at room temperature, and it cannot be used to produce wax products such as candle and wax figure. Compared to WCO, the E-WCO exhibited a higher melting point range (38–41 °C), its shape is stable and can be maintained at room temperature which make it ideal to produce wax products. The introduction of SA could significantly increase the melting point of WCO-based waxes, and as the SA dosage increases, the melting points of wax samples also showed an increasing trend and approached to the melting point of SA (57–58 °C). The results also showed that WCO-based wax with 75 wt% SA (A3 sample) showed a higher melting point (54–56 °C) than that of commercial SW (51–54 °C).
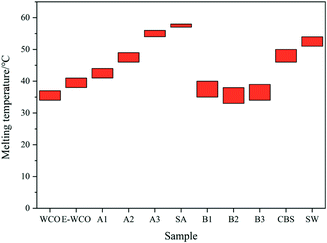 |
| Fig. 3 The melting points of WCO-based waxes and other control samples. WCO = waste cooking oil; E-WCO = epoxy waste cooking oil; SA = stearic acid; CBS = cocoa butter substitute; SW = soybean wax; A1–A3 represent WCO-based wax samples with different amounts of SA; B1–B3 represent WCO-based wax samples with different amounts of CBS. | |
Unlike SA, addition of CBS on E-WCO form wax materials with low melting points. As can be seen from the results (Fig. 3), the melting point of the wax samples (B1–B3) initially decreases and then slowly increase, as the CBS dosage increases. This might be due to the existence of some kind of co-melting compounds with low melting points. The melting point of WCO-based wax 50 wt% CBS (B2 sample, 33–38 °C) was close to that of WCO. However, B2 sample displayed better shape stability and hardness at room temperature than that of WCO.
3.2 Color
Plant wax is generally used to prepare high artistic essential oil candle or wax figure. Therefore, a good plant wax requires light color to facilitate subsequent coloring and other processing. According to China Standard GB/T 30392-2013, the Lovibond color codes of plant wax materials are mainly reflected in yellow color code (Y) and red color code (R), with a requirement of Y ≤ 35 and R ≤ 4, which display a color of light yellow to white visually. The Lovibond color codes of WCO-based waxes and other control samples are shown in Fig. 4 and Table S2 in ESI.† The results showed that the dark brown solid WCO exhibited very high Lovibond color value (Y = 51.1, R = 24.3). After epoxidation, the color of E-WCO became lighter and turned into yellow, with a lower Lovibond color value of Y = 34.0 and R = 10.0.
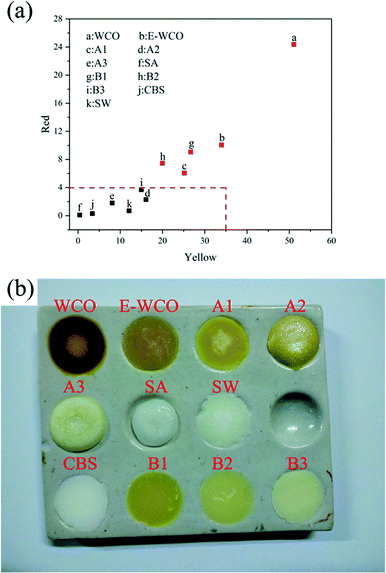 |
| Fig. 4 (a) The Lovibond color codes of WCO-based waxes and other control samples. The points within the red box represents the color codes of corresponding samples that can meet the requirement of China Standard GB/T 30392-2013; (b) the photographs showing the colors of WCO-based waxes and other control samples. WCO = waste cooking oil; E-WCO = epoxy waste cooking oil; SA = stearic acid; CBS = cocoa butter substitute; SW = soybean wax; A1–A3 represent WCO-based wax samples with different amounts of SA; B1–B3 represent WCO-based wax samples with different amounts of CBS. | |
Due to their nearly pure white color, introducing either SA or CBS can effectively improve the colors of the WCO-based waxes. As more amounts of SA or CBS are added, the corresponding Y and R color code of WCO-based waxes decreases. The results also revealed that the wax products comprising ≥50 wt% SA (samples A2 and A3) or ≥75 wt% CBS (sample B3) meet the requirements of China Standard (Fig. 4(a)). Compared with CBS, SA containing WCO-based wax could achieve light color more effectively. The wax product with 75 wt% SA (sample A3) displayed a similar light color (Y = 8.1, R = 1.8) to that of commercial SW (Y = 12.1, R = 0.7) (Fig. 4(a) and (b)).
3.3 Hardness
Hardness of wax products based on WCO were characterized using the needle penetration index and the results are presented in Fig. 5 and Table S3 in ESI.† Since the WCO was too soft, its needle penetration index was not detected. After the epoxidation process, the hardness of E-WCO was improved, with a needle penetration index of 22.68 mm. Introduction of SA enhanced, significantly, the hardness of WCO-based wax. For instance, the wax comprising only 25 wt% SA (sample A1) displayed similar needle penetration index (5.67 mm) to commercial SW (5.46 mm). Hardness of the wax samples A1–A3 showed a similar increasing trend with the SA dosage. In general, WCO-based waxes comprising SA are generally hard at room temperature and suitable for producing wax figure or crayon. On the other hand, introducing of CBS did not improve the hardness, effectively, and as the CBS dosage increases, the needle penetration index of the wax samples (B1–B3) increased first and then decreased, but all larger than that of pure E-WCO.
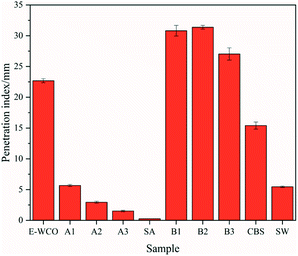 |
| Fig. 5 The penetration indexes of WCO-based waxes and other control samples. WCO = waste cooking oil; E-WCO = epoxy waste cooking oil; SA = stearic acid; CBS = cocoa butter substitute; SW = soybean wax; A1–A3 represent WCO-based wax samples with different amounts of SA; B1–B3 represent WCO-based wax samples with different amounts of CBS. | |
3.4 Combustion performance
Combustion performances of the synthesized waxes were investigated and the corresponding combustion times of the candles made of the WCO-based wax are presented (Fig. 6 and Table S4 in ESI†). The WCO itself was too soft to maintain its shape at room temperature, and could not produce a candle. Due to its higher melting point and hardness, relatively, E-WCO was used to produce a free-standing candle, which can give a steady, bright flame without black smoke. However, its melting speed was quiet fast exhibited short combustion time (42 min). Upon SA introduction, the combustion time of the candles get improved, significantly, and further enhanced as SA dosage increases. For example, the wax candle containing 50 wt% SA (sample A2) displayed similar combustion time (227 min) to commercial SW (276 min), while the candle containing 75 wt% SA (sample A3) displayed even longer combustion time (335 min). In the case of CBS addition, as the CBS dosage increases, the combustion time (samples B1–B3) increased initially, and then decreased, and finally showed an increasing trend. As a whole, introducing CBS did not improve the combustion properties of E-WCO relative to SA.
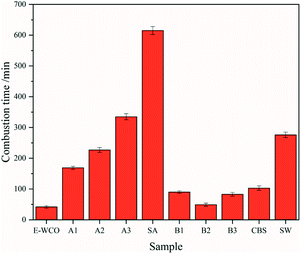 |
| Fig. 6 The combustion times of cube candles of WCO-based waxes and other control samples. WCO = waste cooking oil; E-WCO = epoxy waste cooking oil; SA = stearic acid; CBS = cocoa butter substitute; SW = soybean wax; A1–A3 represent WCO-based wax samples with different amounts of SA; B1–B3 represent WCO-based wax samples with different amounts of CBS. | |
3.5 IR spectra
To learn the available functional groups in the products, their corresponding IR spectra were recorded as shown in the ESI† (Fig. S1). As can be seen, the raw WCO displayed double bond stretching vibration peaks at 1638 cm−1 and 888 cm−1. After epoxidation, the E-WCO showed an epoxy absorption peaks at 843 cm−1 and 898 cm−1, while the double bond peak at 1638 cm−1 was disappeared, indicating that the WCO molecule was epoxidated successfully. In IR spectra of other WCO-based waxes, with the increase of SA or CBS dosage, the peaks of epoxy group at 843 cm−1 and 898 cm−1 were covered by the absorption peaks of SA or CBS and became indistinct.
3.6 Micro-morphology
The micro-morphology study results of WCO-based waxes are shown in Fig. 7. From the images (Fig. 7) and the XRD diffraction patterns (Fig. S2 in ESI†), it can be seen that the WCO solid has irregular fine particles with poor crystallization performance with a considerable number of circular oil droplets. Unlike WCO, E-WCO solid consists of uniform fine grains without oil droplets, whose grain size was much smaller than that of commercial SW. The micro-morphology of pure SA displayed regularly arranged, large long-rod crystalline crystals. This seems why SA addition significantly enhances the grain sizes of WCO-based wax, and as the SA dosage increases, long-rod crystals of SA increase and gradually formed 3D crossing networks. Compared with that of SA, the grain size of CBS is finer and the WCO-based waxes containing CBS all displayed similar fine grain micro-morphologies.
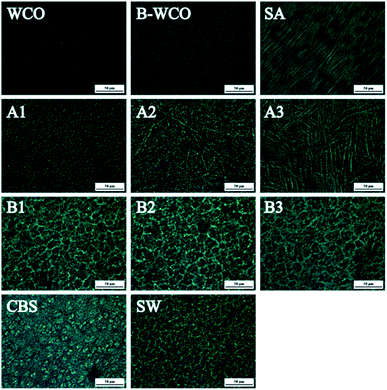 |
| Fig. 7 The 500× microphotographs of the WCO-based waxes and other control samples. WCO = waste cooking oil; E-WCO = epoxy waste cooking oil; SA = stearic acid; CBS = cocoa butter substitute; SW = soybean wax; A1–A3 represent WCO-based wax samples with different amounts of SA; B1–B3 represent WCO-based wax samples with different amounts of CBS. | |
3.7 The effect of epoxidation and combination with SA or CBS on the wax
A good quality plant-based wax has to have light color, odorless, certain hardness and melting point, and its candle product should have sufficient combustion time. The WCO consists of mainly mixed triglycerides with a certain amount of double bonds, as well as many kinds of colored or odorous organic impurities. The unsaturated double bonds would lead to a loose molecular arrangement. This is in good agreement with the micro-morphology results (Fig. 7) which showed that the WCO composed of irregular fine particles and even liquid oil drops which may cause the low melting point and very weak hardness of WCO. Together with its dark color and rancidity odor of its various impurities, WCO is not a good candidate as wax raw material.
The experimental results indicated that, epoxidation reaction could be the basis for WCO-to-wax conversion. First, the epoxidation reagent containing peracetic acid was produced by the reaction of hydrogen peroxide and glacial acetic acid. As strong oxidant, excess peracetic acid would cause peroxidative degradation to various colored and odorous organic impurities in WCO, and eliminate the rancidity odor and improve its color. Second, unsaturated double bonds in WCO were epoxidated to form epoxy groups. Compared with that in WCO, the epoxy triglycerides in E-WCO could form more regular grains without oil droplets (Fig. 7). Thus, E-WCO displayed higher melting point and hardness than that of WCO, which could have maintained its shape at room temperature or initial combustion stage. Furthermore, E-WCO has relatively light color and is odorless, and hence, can be used as wax material.
However, the color of E-WCO doesn't completely fit the required China standards. Moreover, due to too small grains in its solid, the E-WCO displayed lower melting point and weaker hardness than that of commercial SW, and its candle was soft and displayed short combustion time during the combustion process. Thus, introducing a compound with relatively high melting point, good hardness and light color could further modify the WCO-based wax. It was observed that SA was very suitable for making WCO-based wax with better performance. The large long-rod crystals of SA could form a 3D stable crossing network (Fig. 7), which effectively solve the constraints associated to E-WCO grain size. This enhances melting point and hardness of the WCO-based wax, increases combustion time of its candle during combustion process. Furthermore, light colored SA can also effectively reduce the color code of the WCO-based wax. In general, the WCO-based wax containing ≥50 wt% SA (e.g. samples A2 and A3) showed similar performance to commercial SW and fulfill the national standard's requirements. Therefore, SA induced WCO-based wax can be used to make real-world wax products like candle, crayon or wax figure. Due to the application of WCO as a raw material, WCO-based wax is cost-effective and competitive in the market. The estimated total synthesis costs of wax samples are $0.64 per kg for A2 and $0.88 per kg for A3, which is about 1/4 and 1/3 of the commercial SW ($2.35 per kg), respectively.
Compared with SA, CBS could also reduce the color code of the WCO-based wax effectively. However, as a kind of oil, the CBS could not form large, highly crystalline crystals like that of SA, which indicate that it cannot fill the E-WCO grains size issue. As a result, the WCO-based waxes containing CBS displayed relatively low melting point, hardness and combustion time, and their overall performances were not as well as SA comprising WCO-based waxes.
4. Conclusions
In this paper, we report the synthesis and properties of low-cost wax based on WCO, and discussed the effect of epoxidation and addition of SA or CBS on the wax quality. The results showed that, epoxidation reaction could produce E-WCO with lighter color, higher melting point and hardness than that of the original WCO. On the other hand, combining appropriate modifier with E-WCO has further improved the WCO-based wax performances, and SA was proved to be an ideal modifier. The WCO-based wax candle made of E-WCO and SA showed light color, odorless, good hardness, appropriate melting point, and relatively long combustion time, which fulfill the national standard requirements, and hence, can substitute commercial one made of SW. Moreover, WCO-based wax has many additional benefits, such as low synthesis cost, gentle reaction condition, convenient synthesis route, and no secondary pollution. Compared to common WCO-to-biodiesel conversion strategies, the current WCO-to-wax conversion strategy displayed relatively smaller industrial scale and market value, but can be carried out with much lower plant and equipment investment and more flexible production control. Since the production of WCO-based wax is based on effective utilization of WCO with low iodine value, and high impurity contents that is unsuitable for the production of biodiesel, it is conceivable that a stage wise treatments of WCO and a simultaneous synthesis of wax and biodiesel could be a better solution for WCO recycling, especially in economically lagging regions.
Conflicts of interest
There are no conflicts to declare.
Abbreviations
WCO | Waste cooking oil |
E-WCO | Epoxy waste cooking oil |
SA | Stearic acid |
CBS | Cocoa butter substitute |
SW | Soybean wax |
Symbols
A1–A3 | WCO-based wax sample containing different amounts of SA |
B1–B3 | WCO-based wax sample containing different amounts of CBS |
Acknowledgements
This work was supported by grants of National Nature Science Foundation of China (No. 51763007), Guangxi Natural Science Foundation Program (No. 2015GXNSFBA139033), Basic Ability Improvement Program for Guangxi Young and Middle-aged Teachers (No. KY2016YB186), Sharing Foundation of Guangxi Key Laboratory of Optical and Electronic Materials and Devices (No. 20 AA-15 and 20 AA-9), as well as Sharing Foundation of Guangxi Key Laboratory of Geomechanics and Geotechnical Engineering (No. 17-J-21-7). The authors would like to express their gratitude to EditSprings (https://www.editsprings.cn/) for the expert linguistic services provided.
References
- J. C. C. Santana, A. C. Miranda, L. Souza, C. L. K. Yamamura, D. F. Coelho, E. B. Tambourgi, F. T. Berssaneti and L. L. Ho, Sustainability, 2021, 13, 9185 CrossRef CAS.
- H. H. Bandbafha, C. Li, X. Chen, W. Peng, M. Aghbashlo, S. S. Lam and M. Tabatabaei, J. Hazard. Mater., 2021, 127636 Search PubMed.
- V. C. Ravelo and J. S. Rodriguez, J. Environ. Manage., 2018, 228, 117–129 CrossRef PubMed.
- N. S. A. Zamanhuri, M. F. Hanafi and N. Sapawe, Mater. Today: Proc., 2020, 31, A122–A125 Search PubMed.
- T. K. Sahu, S. Sarkar and P. C. Shukla, Fuel, 2021, 283, 119262 CrossRef CAS.
- S. S. Bargole, R. Bhoi, S. George and V. K. Saharan, Advanced Technology for the Conversion of Waste into Fuels and Chemicals, 2021, vol. 1, pp. 231–272 Search PubMed.
- W. Zhang, H. Ji, Y. Song, S. Ma, W. Xiong, C. Chen, B. Chen and X. Zhang, J. Cleaner Prod., 2020, 274, 122918 CrossRef CAS.
- R. Z. K. Hussein, N. K. Attia, K. F. Mai and S. T. Elsheltawy, Biomass Bioenergy, 2021, 144, 105850 CrossRef CAS.
- L. M. Orozco, S. Cardona, C. Gomez, H. Inciarte, Y. Villada and L. Rios, Prog. Org. Coat., 2021, 161, 106467 CrossRef CAS.
- K. Sharma, S. S. Toor, J. Brando, T. H. Pedersen and L. A. Rosendahl, J. Cleaner Prod., 2021, 294, 126214 CrossRef CAS.
- A. Pospisilova, I. Novackova and R. Prikryl, Bioresour. Technol., 2021, 326, 124683 CrossRef CAS PubMed.
- B. H. H. Goh, C. T. Chong, Y. Ge, H. C. Ong, J. H. Ng, B. Tian, V. Ashokkumar, S. Lim, T. Seljak and V. Jozsa, Energy Convers. Manage., 2020, 223, 113296 CrossRef CAS.
- B. Changmai, C. Vanlalveni, A. P. Ingle, T. Noor, R. Bhagat and S. L. Rokhum, RSC Adv., 2020, 10, 41625 RSC.
- D. Singh, D. Sharma, S. L. Soni, C. S. Inda, S. Sharma, P. K. Sharma and A. Jhalani, J. Cleaner Prod., 2021, 307, 127299 CrossRef CAS.
- R. S. Nursal, A. Khalid, I. S. Abdullah, N. Jaat, N. Darlis and H. Koten, Fuel, 2021, 306, 121695 CrossRef.
- I. S. Abdullah, A. Khalid, N. Jaat, R. S. Nursal, H. Koten and Y. Karagoz, Fuel, 2021, 297, 120706 CrossRef.
- M. Mohadesi, B. Aghel, M. Maleki and A. Ansari, Fuel, 2020, 263, 116659 CrossRef CAS.
- B. Panchal, Z. Zhu, S. Qin, T. Chang, Q. Zhao, Y. Sun, C. Zhao, J. Wang, K. Bian and S. Rankhamb, Renew. Energy, 2022, 181, 341–354 CrossRef CAS.
- N. F. Sulaiman, N. I. Ramly, M. H. A. Mubinc and S. L. Lee, RSC Adv., 2021, 11, 21781 RSC.
- J. Yang and H. Shan, J. Cleaner Prod., 2021, 282, 125331 CrossRef.
- Y. Zhao, C. Wang, L. Zhang, Y. Chang and Y. Hao, Renewable Sustainable Energy Rev., 2021, 140, 110661 CrossRef CAS.
- P. Sharma, M. Usman, E. S. Salama, M. Redina, N. Thakur and X. Li, Waste Manag., 2021, 136, 219–229 CrossRef CAS PubMed.
- A. S. Yusuff, A. O. Gbadamosi and L. T. Popoola, J. Environ. Chem. Eng., 2021, 9, 104955 CrossRef CAS.
- W. H. Foo, W. Y. Chia, D. Y. Y. Tang, S. S. N. Koay, S. S. Lim and K. W. Chew, J. Hazard. Mater., 2021, 417, 126129 CrossRef CAS PubMed.
- H. M. Khan, T. Iqbal, C. H. Ali, A. Javaid and I. I. Cheema, Fuel, 2020, 277, 118091 CrossRef.
- A. R. Mohammed and C. Bandari, Biofuels, 2018, 9, 567–574 CrossRef.
- F. Zaman, M. W. Ishaq, N. Ul-Haq, W. U. Rahman, M. M. Ali, F. Ahmed and A. ul Haq, ChemBioEng Rev., 2022, 9(no. 1), 1–16 Search PubMed.
- M. A. Gonçalves, E. K. L. Mares, J. R. Zamian, G. N. d. R. Filho and L. R. V. d. Conceição, Fuel, 2021, 304, 121463 CrossRef.
- A. Gouran, B. Aghel and F. Nasirmanesh, Fuel, 2021, 295, 120542 CrossRef CAS.
- H. Kumar, A. A. Renita and S. A. Jabasingh, Environ. Prog. Sustainable Energy, 2021, 40, 13632 Search PubMed.
- J. H. C. Wancura, A. L. Fantinel, G. A. Ugalde, F. F. Donato, J. V. d. Oliveira, M. V. Tres and S. L. Jahn, J. Cleaner Prod., 2021, 285, 124838 CrossRef CAS.
- J. Patchimpet, B. K. Simpson, K. Sangkharak and S. Klomklao, Renew. Energy, 2020, 153, 861–869 CrossRef CAS.
- Y. Xiong, W. F. Miao, N. N. Wang, H. M. Chen, X. R. Wang, J. Y. Wang, Q. L. Tan and S. P. Chen, Waste Manag., 2019, 85, 295–303 CrossRef CAS PubMed.
- K. Rezaei, W. Tong and L. A. Johnson, J. Am. Oil Chem. Soc., 2002, 79, 1241–1247 CrossRef CAS.
- T. Fei, K. Ren and T. Wang, J. Am. Oil Chem. Soc., 2020, 97, 1141–1150 CrossRef CAS.
- R. X. Chen and W. C. Wang, Renew. Energy, 2019, 135, 819–835 CrossRef CAS.
- P. Dujjanutat and P. Kaewkannetra, Renew. Energy, 2020, 147, 464–472 CrossRef CAS.
- P. Ji, Z. Fan, M. Niu, L. Pan, G. Jing, R. Tian and W. Li, React. Kinet., Mech. Catal., 2021, 132, 751–769 CrossRef CAS.
- H. Iida, K. Takahashi, A. Yanagisawa, H. Hashimoto and A. Igarashi, Food Chem., 2021, 340, 127927 CrossRef CAS PubMed.
- P. T. Wai, P. Jiang, Y. Shen, P. Zhang, Q. Gu and Y. Leng, RSC Adv., 2019, 9, 38119 RSC.
- P. D. Jadhav, A. V. Patwardhan and R. D. Kulkarni, Mol. Catal., 2021, 511, 111748 CrossRef CAS.
- M. J. Jalil, A. Hadi and I. S. Azmi, Mater. Chem. Phys., 2021, 270, 124754 CrossRef CAS.
- S. Bashiri, B. Ghobadian, M. D. Soufi and S. Gorjian, Mater. Sci. Energy Technol., 2021, 4, 119–127 CAS.
Footnote |
† Electronic supplementary information (ESI) available. See DOI: 10.1039/d1ra08874b |
|
This journal is © The Royal Society of Chemistry 2022 |
Click here to see how this site uses Cookies. View our privacy policy here.