DOI:
10.1039/D1RA08090C
(Paper)
RSC Adv., 2022,
12, 1968-1981
Biochemical characterization of a novel azo reductase named BVU5 from the bacterial flora DDMZ1: application for decolorization of azo dyes†
Received
4th November 2021
, Accepted 9th December 2021
First published on 12th January 2022
Abstract
One of the main mechanisms of bacterial decolorization and degradation of azo dyes is the use of biological enzymes to catalyze the breaking of azo bonds. This paper shows the expression and properties of a novel azo reductase (hybrid-cluster NAD(P)-dependent oxidoreductase, accession no. A0A1S1BVU5, named BVU5) from the bacterial flora DDMZ1 for degradation of azo dyes. The molecular weight of BVU5 is about 40.1 kDa, and it contains the prosthetic group flavin mononucleotide (FMN). It has the decolorization ability of 80.1 ± 2.5% within 3 min for a dye concentration of 20 mg L−1, and 53.5 ± 1.8% even for a dye concentration of 200 mg L−1 after 30 min. The optimum temperature of enzyme BVU5 is 30 °C and the optimum pH is 6. It is insensitive to salt concentration up to a salinity level of 10%. Furthermore, enzyme BVU5 has good tolerance toward some metal ions (2 mM) such as Mn2+, Ca2+, Mg2+ and Cu2+ and some organic solvents (20%) such as DMSO, methanol, isopentyl, ethylene glycol and N-hexane. However, the enzyme BVU5 has a low tolerance to high concentrations of denaturants. In particular, it is sensitive to the denaturants guanidine hydrochloride (GdmCl) (2 M) and urea (2 M). Analysis of the dye substrate specificity shows that enzyme BVU5 decolorizes most azo dyes, which is indicating that the enzyme is not strictly substrate specific, it is a functional enzyme for breaking the azo structure. Liquid chromatography/time-of-flight/mass spectrometry (LC-TOF-MS) revealed after the action of enzyme BVU5 that some intermediate products with relatively large molecular weights were produced; this illustrates a symmetric or an asymmetric rapid cleavage of the azo bonds by this enzyme. The potential degradation pathways and the enzyme-catalyzed degradation mechanism are deduced in the end of this paper. The results give insight into the potential of a rapid bio-pretreatment by enzyme BVU5 for processing azo dye wastewater.
1. Introduction
Azo dyes are organic compounds that color other substances in a molecular or dispersed state. They are used in many industries such as textiles, paper printing, and pharmaceuticals.1–3 Azo dyes are synthetic dyes with a high output and application rate in the textile industry. They account for more than 50% of all dyes synthesized.4,5 The molecular structure of azo dyes is characterized generally by one or more azo groups (–N
N–), which are the chromophore. Due to the chemical structure, large quantities of colored effluents are generated by the textile industry, which are a difficult to degrade and are highly toxic to the aquatic environment.6 Thus, it is of great importance to separate and degrade the azo dyes from the effluents, before the latter are discharged into the environment.
Biological treatment methods are usually cost-effective and environmentally friendly, if compared with physical or chemical methods. Microorganisms play a significant role in biological treatment methods. Microorganisms which can degrade azo dyes include fungi and bacteria. Among the bacteria, aerobic or anaerobic genera like Pseudomonas, Enterobacter and Bacillus are the most numerous. Due to the mechanism of action and growth in different environments, the decolorization rates of different types of microorganisms are quite different.7,8 Microorganisms degrade azo dyes by reducing the azo bonds, which leads to the formation of amines. Azo reductase enzymes are the key enzymes in the process degradation. Azo reductases cleave the azo bonds using the reductive poses of NADH or NADPH.9
In recent years, many azo reductases from different bacteria and fungi have been described. They originate from Enterococcus faecalis, Staphylococcus aureus, Escherichia coli, and Xenophilusazovorans KF46F, which have an azo reductase activity.10 If the strain Shewanella decolorationis S12 used formic acid, lactic acid or butyric acid as the electron donor, a considerable reduction of various azo dyes resulted. The reduction and decolorization rate of azo dyes reached more than 80% in 36 h.11 The results about the strain Shewanella aquimarina reducing the azo dye Acid Red 27 show that the reduction decolorization of Acid Red 27 was as high as 97% in 5 h, also a salinity of 5% NaCl was tolerated.12 In our work a large number of proteins expressed in the system degrade the dye reactive black 5 (RB5), and were screened through macro proteomics analysis. One azo reductase named BVU5 was expressed exogenously in E. coli through molecular cloning. Enzyme BVU5 is a heterozygous cluster NAD(P)-dependent oxidoreductase from the functional bacterial flora DDMZ1 (NCBI gene accession number MK583566) with a high-efficiency azo reductase activity.
Most studies are based on the decolorization during bacterial growth, which usually take several hours or even dozens of hours to achieve a satisfying decolorization effect.13 Compared with the rapid catalysis by enzymes such as BVU5, the efficiency is very low. Hence, purified azo reductase BVU5 was directly applied to the decolorization and degradation of azo dyes in this study. In addition, the enzyme BVU5 was selected from the dominant bacterial genera of the functional bacterial flora DDMZ1 through macroproteomics technology and then expressed and extracted. In this way the separation and purification of a single strain was avoided, and at the same time the screening range of azo reductase enzymes was expanded. But the mechanism of degradation of azo dyes and the properties of the enzyme are unclear. There are few reports on the effect of enzymes on bis-azo dyes with large molecules, complex structures and high concentrations, such as RB5.14 For this reason, the enzyme BVU5 was regarded as the main research object and in-depth related research was conducted.
The aim of the present study is to investigate the performance and mechanism of the novel azoreductase BVU5 on the degradation of azo dyes such as RB5. Consequently, the novel azoreductase BVU5 was purified. The effects of temperature, pH and salt concentration as well as the tolerance to certain metal ions, organic solvents and high concentrations of denaturants were explored. The dye degradation rate and the enzyme activity of BVU5 were assessed. In addition, the potential degradation pathways and the degradation mechanism are deduced. This study demonstrates that BVU5 is an enzyme with excellent properties. It is a promising candidate to degrade azo dyes efficiently in industrial wastewater and might give insight into a rapid bio-pretreatment.
2. Materials and methods
2.1 Materials
Reactive black 5 (RB5, CAS no. 17095-24-8, MW 991.82, λmax = 597 nm) was purchased from Sigma-Aldrich (USA). E. coli DH5α cells and E. coli BL21(DE3) were purchased from Sangon Biotech Co., Ltd (China). All chemicals were of analytical grade and were purchased from Sinopharm Chemical Reagent Co., Ltd (Shanghai). The basic medium contained (L−1): 0.2 g NH4Cl, 0.5 g Na2SO4, 2.66 g KH2PO4 and 3 g yeast extract. All culture media were sterilized using a pressure steam sterilizer at 115 °C and 0.12 MPa for 30 min.
Our laboratory had successfully expressed the hybrid-cluster NAD(P)-dependent oxidoreductase (accession no. A0A1S1BVU5, named BVU5) in recombinant E. coli. The gene of enzyme BVU5 was amplified from the bacterial flora DDMZ1. The bacterial flora DDMZ1 and the recombinant E. coli were stored in our laboratory.15 Previous study revealed the bacterial flora DDMZ1 could decolorize more than 90% of various azo dyes such as Reactive Black 5, Acid Red 112, Orange Yellow I, Congo Red and Acid Orange within 24 h. The predominant bacteria in bacterial flora DDMZ1 were Stenotrophomonas, Pseudomonas, Lactococcus, and Alcaligenes unclassified_f_Alcaligenaceae16 Through molecular cloning the target gene of enzyme BVU5 was obtained and amplified. The protein expression vector MBP-BVU5 had been designed, and the MBP tag was added to reduce the degradation of enzyme BVU5.
2.2 Expression and purification of enzyme BVU5
E. coli BL21 containing a recombinant plasmid was streaked on LB solid medium (30 μg mL−1 kanamycin) and cultured at 37 °C for 12 h. Multiple single colonies were picked and cultured in 5 mL LB liquid medium (30 μg mL−1 kanamycin) at 37 °C for 4–5 h, until the OD600 tide reached to 0.4–0.6. Isopropyl-β-D-1-thiogalactopyranoside (IPTG) with a final concentration of 1 mM was added to the culture medium at 37 °C and 100 × g for 4 h to induce the BVU5 expression. After that, bacterial cells were collected by high-speed centrifugation (4000 × g) at 4 °C for 5 min. Then induced cells were ruptured by a homogenizer (800 psi). After centrifugation (6000 × g) at 4 °C for 20 min the supernatant of the cell lysis solution was loaded onto a Ni-NTA column (5 mL, Gen Script, China). Unbound proteins were washed out with tris buffer (pH 8) containing imidazole (10, 20 and 30 mM). The bound BVU5 protein was eluted with imidazole (250, 500 mM) in tris buffer (pH 8). The purified enzyme BVU5 was analyzed by SDS-PAGE. After dialysis, an ultrafiltration tube (30 K) was used to concentrate the protein.
2.3 Prosthetic group and coenzyme preference of enzyme BVU5
The prosthetic group FMN in BVU5 was identified using LC-TOF-MS. With BC Grade powder of FMN to prepare FMN standard solution. Enzyme BVU5 needs NADH, NADPH or NADP+ as a coenzyme to degrade azo dyes. Different coenzymes were added to each system with dye RB5 (20 mg L−1) and enzyme BVU5 (0.3 μg) to reveal its performance for decolorization. The decolorization rates were determined at 25 °C after incubation for 3 min or 3 h.
2.4 Effect of different RB5 concentrations
A concentration series of dye RB5 (20, 40, 80, 100 and 200 mg L−1) was used to study the decolorization by enzyme BVU5. The systems contained 0.3 μg BVU5 and 2.5 mM NADH were incubated at 30 °C for 30 min. Aliquots from each culture were collected to examine RB5 decolorization. Three replicates were processed for each experiment.
The RB5 decolorizing effect was determined by a spectrophotometer (Hitachi U200, Tokyo, Japan) at λmax of 597 nm. The decolorization ratio was calculated according to the equation: decolorization (%) = (A0 − At)/A0 ×100, where A0 and At represent the initial absorbance of the dye and the absorbance after incubation.
2.5 Effects of temperature, pH and salt concentrations
To determine the optimum temperature for enzyme BVU5 for decoloring of RB5, the reaction solution was incubated in a water bath at temperatures ranging from 10 °C to 60 °C. Each system contained 0.3 μg BVU5, 2.5 mM NADH and 100 mg L−1 RB5. The decolorization was measured after 30 min. In order to determine the thermal stability of enzyme BVU5, it was incubated at different temperatures (ranging from 10 °C to 60 °C) for 1 h. Then the residual activity for decoloring RB5 was determined.
The optimal reaction pH was contested over a pH ranged from 4.0 to 10.0 with different buffers (PBS buffer (pH 4.0–7.0) and Tris–HCl buffer solution (pH 7.0–10.0)). Each assay contained 0.3 μg BVU5, 2.5 mM NADH and 100 mg L−1 RB5. The decolorization was measured after 30 min. In order to determine the acid–base stability of the enzyme BVU5, it was pre-incubated at different pH (ranging from 4.0 to 10.0) at 30 °C for 1 h. Then the residual activity for decoloring RB5 was determined.
The effect of different NaCl concentrations (5, 10, 15 and 20%, w/v) on the decolorization of RB5 was explored. Each system contained 0.3 μg BVU5, 2.5 mM NADH and 100 mg L−1 RB5. The decolorization was measured after 30 min. In order to evaluate the salt stability of the enzyme BVU5, it was pre-incubated at different salinities (ranging from 5% to 20%, w/v) at 30 °C for 1 h. Then the residual activity for decoloring RB5 was determined. Three replicates were processed for each experiment.
2.6 Effect of metal ions, organic solvents and denaturants
To estimate the effect of metal ions on the decolorization by enzyme BVU5, the reaction solution was mixed with different metal ions (FeCl3, MnCl2, MgCl2, CaCl2, CoCl2, CuSO4, NiCl2, ZnCl2, FeCl2 and CdCl2). Each system contained 2 mM of different metal ions, 0.3 μg BVU5, 2.5 mM NADH and 100 mg L−1 RB5. The decolorization was measured after 30 min. In order to detect the effect of metal ions on enzyme stability, the enzyme BVU5 was pre-incubated with different metal ions (2 mM, 10 mM) at 30 °C for 1 h. Then the residual activity on decoloring of RB5 was determined.
Organic solvents were added to study their effect on the decolorization of enzyme BVU5. The solvents of DMSO, acetone, ethanol, methanol, isopentyl, ethylene glycol, N-hexane and isopropanol were used. Each system contained 0.3 μg BVU5, 2.5 mM NADH and 100 mg L−1 RB5. The content of organic solvent was 10% (v/v). The decolorization was measured after 30 min. In order to clarify the effect of organic solvents on enzyme stability, the enzyme BVU5 was pre-incubated with organic solvents at 30 °C for 1 h. The final organic solvent concentrations were 10% or 20%. Then the residual activity for decoloring RB5 was determined.
To study the effect of denaturants on the decolorization efficiency of enzyme BVU5, three denaturants (0.1% SDS, 1 M urea, and 1 M GdmCl) were selected. Each system contained 0.3 μg BVU5, 2.5 mM NADH and 100 mg L−1 RB5. The decolorization was measured after 30 min. In order to test the effect of denaturants on enzyme stability. Enzyme BVU5 was pre-incubated with different denaturants 0.1% and 0.2% SDS, 1 M and 2 M urea, and 1 M and 2 M GdmCl at 30 °C for 1 h. Then the residual activity for decoloring of RB5 was determined. Three replicates were processed for each experiment.
2.7 Effect of different dye species
Dye substrate specificity of enzyme BVU5 was measured at the wavelength established for each dye (597 nm for dye (1) Reactive Black 5, 605 nm for dye (2) Trypan Blue, 328 nm for dye (3) Chlorazol Black E, 487 nm for dye (4) Acid Orange 7, 597 nm for dye (5) Acid Black 1, 529 nm for dye (6) Acid Red 112, 486 nm for dye (7) α-Naphthol Orange, 619 nm for dye (8) Acid Blue 40, 596 nm for dye (9) Reactive Blue 4, 596 nm for dye (10) Reactive Blue 19, 616 nm for dye (11) Malachite Green, 625 nm for dye (12) Brilliant Green, 543 nm for dye (13) Fuchsin Basic, 597 nm for dye (14) Crystal Violet. Each assay contained 0.3 μg BVU5, 2.5 mM NADH, and a dye concentration of 100 mg L−1. After incubation at 30 °C for 30 min, the decolorization was measured. Three replicates were processed for each experiment.
2.8 Kinetic properties of BVU5
The Michaelis constant gives important characteristics of enzyme. The Michaelis equation reflects the relationship between the reaction rate and the concentration of the substrate,17,18 as shown below:
The Km value is the Michaelis constant, Vmax represents the reaction, if the enzyme is saturated with the substrate, and [S] is the substrate concentration, which is calculated from the Lineweawer–Burk double reciprocal drawing method.19 The enzyme BVU5 is a redox enzyme which requires coenzyme NADH or NADPH for the reaction. It is a double substrate reaction mechanism, also known as sequential reaction or ping–pong reaction. The mechanism is as follows: ① Kc: E + C
EC, ② Ks: EC + S
ECS, ③ K: ECS
E + C + P. In this study, several concentrations of coenzyme NADH and different concentrations of dye RB5 were used as substrates. The reciprocal substrate concentration 1/[S] and the initial enzyme reaction rate 1/v were plotted reciprocally.20 According to the dynamic equation of the sequence mechanism, the following results:
V = (Vmax × [C][S])/([C][S] + [S]Kc,m + [C]Ks,m + Kc,mKs,m) |
where [C] means the concentration of coenzyme NADH and [S] is the concentration of substrate RB5. In a multi-substrate reaction, the Michaelis constant of one substrate changes with the concentration of the other substrate, where
Kc,m refers to the Michaelis constant of NADH if the concentration of dye RB5 reaches saturation.
Ks,m refers to the Michaelis constant of the RB5 substrate if the concentration of coenzyme NADH reaches saturation.
Vmax is the maximum reaction rate if both, the coenzyme and the substrate reach saturation.
To calculate the kinetic parameters such as Km and Vmax of enzyme BVU5, 20–200 mg L−1 of dye RB5 and 0.5–5 mM of coenzyme NADH were tested. 0.3 μg enzyme BVU5 was added and reacted at 30 °C for 30 min. The reduction of dye RB5 per unit time was used as the reaction rate of enzyme BVU5. The characteristic absorption peak of RB5 at 597 nm measured by spectrophotometery was used to determine the activity of enzyme BVU5. According to the Lineweawer–Burk double reciprocal plot, the kinetic parameters Km and Vmax were calculated.
2.9 Measurement of RB5 degradation metabolites
The enzyme BVU5 (0.3 μg) was incubated with NADH 2.5 mM under optimal conditions and used to decolorize 100 mg L−1 RB5. Assays were performed in triplicate. The decolored samples were taken at regular intervals and centrifuged at 4000 × g for 10 min. The supernatants were scanned over the UV-visible full spectrum from 200 nm to 800 nm. RB5 degradation metabolites were identified by FTIR and LC-TOF-MS according to previous study.21
3. Results and discussion
3.1 Expression and purification of enzyme BVU5
The protein expression vector MBP-BVU5 had been designed using Snap Gene. The MBP tag was added to reduce the degradation of enzyme BVU5 and enhance the purification efficiency (Fig. S1(A)†). The molecular weight of the MBP tag was about 40.4 kDa, and the molecular weight of enzyme BVU5 was about 40.1 kDa. So it was estimated that the molecular weight of MBP-BVU5 fusion protein was about 80.5 kDa (seen in Fig. S1(B)†). The gene was cloned into a pET28a (+) vector with a 6× His tag, and finally transformed to E. coli BL21 (DE3) for protein expression. The BVU5 protein had been expressed successfully as a soluble part after the induction of IPTG. After elution with 250 mM imidazole, the purified protein BVU5 was separated as a single band (seen in Fig. S1(B)†).
3.2 Cofactor identification and coenzyme preference
Since the enzyme BVU5 had light a yellow color after purification (shown in Fig. 1(A)), it was speculated that the protein way contains the prosthetic group FMN. The results by LC-TOF-MS analysis show that the molecular weight of FMN in the positive ion mode is 457.1119 (without sodium ions) or 479.0938 (with sodium ions) (Fig. 1(B)). Compared with the mass spectrum of the FMN standard solution (Fig. 1(C)), the spectrum of the enzyme BVU5 had the same mass peaks as those of the FMN standard solution. This confirms that the FMN is part of enzyme BVU5. This also was verified by previous experiment that only the coenzymes NADPH or NADH need for the catalytic reaction of enzyme BVU5 without FMN.
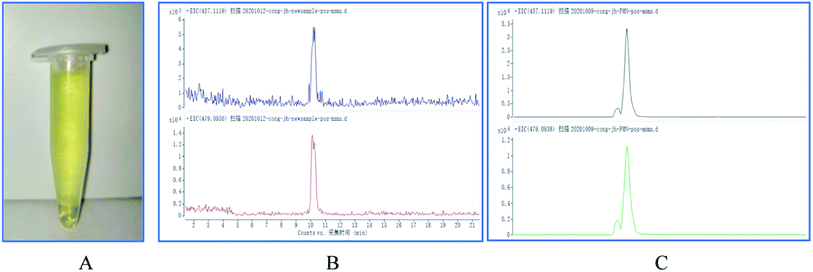 |
| Fig. 1 (A) The photo of purified enzyme BVU5; (B) spectra of enzyme BVU5; (C) mass spectra of FMN standard solution. | |
Enzyme BVU5 is a heterozygous cluster NAD(P)-dependent oxidoreductase. Its catalytic reaction requires coenzymes. Since coenzymes often are soluble components of the enzyme. The preference of enzyme BVU5 for coenzymes. Three coenzymes, NADP+, NADH and NADPH, were added as electron donors to catalyze the reaction and degrade the dye RB5. The effect is shown in Table 1.
Table 1 Decolorization effect of enzyme BVU5 with different coenzymesa
Time |
Sample |
1 |
2 |
3 |
4 |
5 |
6 |
7 |
8 |
Note: (1) RB5 + PBS; (2) RB5 + PBS + NADPH; (3) RB5 + PBS + NADP+; (4) RB5 + PBS + NADH; (5) RB5 + PBS + NADPH + BVU5; (6) RB5 + PBS + NADP+ + BVU5; (7) RB5 + PBS + NADH + BVU5; (8) RB5 + PBS + BVU5 (with 20 mg L−1 RB5, 0.3 μg BVU5, 2.5 mM coenzymes, incubation for 3 min or 3 h at 25 °C). |
3 min |
 |
3 h |
 |
The samples with the coenzymes NADPH or NADH decolorized RB5 within 3 min (the samples no. 5 and no. 7). But the addition of NADP+ had no effect (sample no. 6). The sample without BVU5 but with coenzyme NADP+ (sample no. 3) and the sample without coenzyme but only with enzyme BVU5 (sample no. 8), also did not decolorized RB5. Interestingly, after an incubation for 3 h, the samples containing coenzymes NADPH or NADH (the samples no. 2 and no. 4 in Table 1) had an obvious decolorization effect. Therefore, it can be deduced that enzyme BVU5 catalyze the decolorization of dye RB5 only with coenzymes NADPH or NADH. Also, the reducing coenzymes NADPH or NADH alone had a certain decolorizing effect on RB5, but together with the enzyme BVU5, the decoloration rate was improved significantly.22
3.3 Effect of different RB5 concentrations
The reaction speed of enzyme-catalyzed dye decolorization is very high. The photos in Table 2 show the color change intuitively, which indicates the decolorization performance of dye RB5 at different concentrations. Samples 4 and 5, which are the assays with enzyme BVU5 and coenzyme NADH or NADPH, had the best decolorization effect for any concentration of RB5 (Table 2). Within 3 min, the original blue or black color (depending on the concentration of RB5) changed to light purple or purple and stabilized within 30 min. The decolorization could reach up to 80.1 ± 2.5% (BVU5 + NADH system 4) or 75 ± 2.4% (BVU5 + NADPH system 5) if the dye concentration was 20 mg L−1 with a reaction time of 3 min (Fig. 2). If the dye concentration increased, the decolorization rate decreased, it still reached 53.5 ± 1.8% (BVU5 + NADH system 4) or 49 ± 2.1% (BVU5 + NADPH system 5) even at a dye concentration of 200 mg L−1 after 30 min reaction. At the same time, there was a little difference between different coenzymes in the decolorization of RB5. NADH acted was better than NADPH. Therefore, only NADH was used as the main coenzyme for the following experiments. The catalytic reduction of various dyes by the purified azo reductase in the presence of NADH as electron donor had usually been studied.23,24
Table 2 Decolorization of different concentrations of RB5 catalyzed by enzyme BVU5a
Sample |
Time |
3 min |
30 min |
1 |
2 |
3 |
4 |
5 |
6 |
1 |
2 |
3 |
4 |
5 |
6 |
Samples (1) PBS + RB5; 2. PBS + RB5 + NADPH; (3) PBS + RB5 + NADH; (4) PBS + RB5 + NADH + BVU5; (5) PBS + RB5 + NADPH + BVU5; (6) PBS + BVU5 (with 0.3 μg BVU5, 2.5 mM coenzymes, different concentrations of RB5, incubation for 30 min at 30 °C). |
20 (mg L−1) |
 |
 |
40 (mg L−1) |
 |
 |
80 (mg L−1) |
 |
 |
100 (mg L−1) |
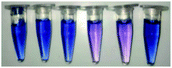 |
 |
200 (mg L−1) |
 |
 |
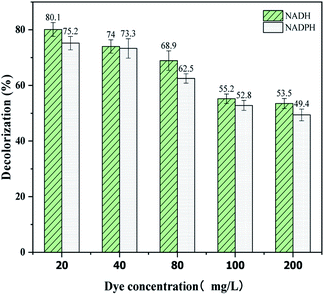 |
| Fig. 2 Decolorization of different concentrations of RB5 catalyzed by enzyme BVU5 with NADH or NADPH as coenzymes after incubation for 30 min. | |
3.4 Effects of temperature, pH and salt concentration
The effect of temperature on the decolorization and enzymatic activity of BVU5 were investigated from 10 °C up to 60 °C.25 As shown in Fig. 3(A), the enzyme at a temperature of 30 °C had the highest decolorization rate of 63.6 ± 2.6%. The thermal stability of enzyme BVU5 is shown in Fig. 3(B). BVU5 displayed the maximum activity at 30 °C after incubation for 1 h. From 10 °C up to 30 °C the relative activity of BVU5 increases linearly. However, for a temperature above 35 °C the relative activity of BVU5 decreases rapidly. These results indicate the optimum temperature for enzyme BVU5 is 30 °C.
 |
| Fig. 3 (A) Decolorization of 100 mg L−1 RB5 at different temperatures. Incubation 30 min. (B) Relative activity of enzyme BVU5 at different temperatures after incubation for 1 h. (C) Decolorization at different pH. Incubation 30 min at 30 °C, dye RB5 100 mg L−1. (D) Relative activity of enzyme BVU5 at different pH after incubation for 1 h. (E) Decolorization at different salt concentrations. Incubation 30 min at 30 °C, dye RB5 100 mg L−1. (F) Relative activity of enzyme BVU5 at different salt concentrations. Incubation 1 h. | |
The pH was an impact on the structure and function of enzyme BVU5. It also can affect the degradation of the dye RB5.26 In this experiment the effect of pH on decolorization and enzymatic activity of BVU5 were investigated over a pH from 4 up to 10. Fig. 3(C) shows that the decolorization has the highest value at pH 6. The decolorization had the highest value at this pH of 70.9 ± 2.1% within 30 min. Therefore, the optimal pH of enzyme BVU5 is 6. As the data in Fig. 3(D) shows that at pH 6, enzyme BVU5 has the highest relative activity. Yang27 reported that an azo reductase had the highest stability at pH 6.5 in sodium phosphate buffer and the activity decreased significantly in other buffers with an incubation time of only 1 h. Lu28 constructed a fusion enzyme GFA which was sensitive against pH changes. After an incubation of only 1 h the degradation activity of GFA decreased in different buffers. The residual activities of that GFA were around 50% or 20% in buffers with pH 5.0 or pH 8.0, respectively. In our study after incubation for 1 h BVU5 retained over 60% of the initial activity with pH ranging from 5.5 to 8.0.
Salts are often used as a solid tissue in printing processes. The conventional treatment guidance cannot remove all salts ions, this results in high concentrations of salts in wastewater such as NaCl and Na2SO4.29 Salts have been a significant factor in the decolorization of dyeing wastewater, which will affect microorganism growth. The content of salts will affect the activity of enzyme. Thus, the salt resistance of enzyme BVU5 was studied since it is vital for the decolorization of azo dye wastewater. Fig. 3(E) shows that the enzyme BVU5 is capable of decolorizing RB5 dye in a wide range of salt concentrations (0–20% NaCl, w/v). Although the highest decolorizing effect was observed at 0% salt concentration with RB5 and a decolorization efficiency of 61.7 ± 2% within 30 min, the enzyme BVU5 displayed a notable tolerance to high salinity and even decolorize 48.9 ± 1.8% RB5 with 5% NaCl (w/v), decolorize 48.3 ± 2.2% RB5 with 10% NaCl (w/v), and decolorize 34.7 ± 1.6% RB5 with 15% NaCl (w/v) within 30 min. The effect of salinity on enzyme activity is shown in Fig. 3(F). After an incubation for 1 h the highest enzyme activity was observed at 0% salt concentration (NaCl). 95% relative activity were noted at 5% salt concentration, and 78% at 10% salt concentration. Avinash30 found that a strain of Rhizobium sp. could tolerate salt concentrations up to 4%. The bacterium Gracilibacillus sp. GTY grows in the media containing 15% (w/v) of NaCl.31 Nevertheless, in this report, the enzyme BVU5 was found insensitive to salt concentration below 10% and showed a preferable applicability for a rapid treatment of high-salt dyeing wastewater.
3.5 Effects of metal ions, organic solvents and denaturants
Wastewater usually contains a variety of metal ions, which might affect the activity of enzymes.32,33 In order to detect the dependence of BVU5 enzyme on metals ions and their influence on the decolorization of RB5, the metal ions Mn2+, Mg2+, Ca2+, Fe2+, Fe3+, Cu2+, Ni2+, Zn2+, Co2+, and Cd2+ were added to the assay.34 The data for RB5 decolorization are shown in Fig. 4(A). The presence of Cd2+ ion (2 mM) markedly inhibited the RB5 decolorization by enzyme BVU5 (decolorization of 11.7 ± 1.5%), followed by Fe3+, Co2+, Zn2+, Ni2+ and Fe2+. Comparatively, the metal ions Mn2+, Ca2+ and Mg2+ had scarcely any inhibiting effect on RB5 decolorization. In the presence of Cu2+ ion (2 mM), the decolorization reached the highest value of 61.9 ± 4.1%, which was about 4% higher than the one of the control samples (decolorization 57.6 ± 2.4%). This indicates that a low concentration of Cu2+ stimulates the activity of enzyme BVU5. Moreover, the relative activity of enzyme BVU5 after incubation for 1 h with different metal ions was investigated. As shown in Fig. 4(B), Cd2+ had the highest inhibitory impact on enzyme BVU5 activity with a relative activity was 3.1 ± 2% (10 mM). Similarly, Fe3+, Co2+, Zn2+, Ni2+ and Fe2+ had a negative impact on BVU5. In contrast, other metal ions like Mn2+, Ca2+, Mg2+ and Cu2+ had little inhibition effect on BVU5. It could be known that the activity of enzyme BVU5 can be affected greatly by metal ions such as Cd2+, Fe3+, Co2+, Zn2+, Ni2+ and Fe2+, but less affected by metal ions such as Mn2+, Ca2+, Mg2+ and Cu2+. Metal ions possibly change the activity of enzyme, because the way to combined with it and form a stable or unstable protein.35 It has been reported that the addition of Cu2+ to the purified enzyme stimulates activity, but only at a low concentration of under 1 mM.36 The ions Ca2+, Mn2+, Mg2+ also have been reported to stimulate activity.37,38
 |
| Fig. 4 (A) Decolorization of RB5 by BUV5 in the presence of 2 mM different metal ions after an incubation for 30 min (RB5 100 mg L−1). (B) Relative activity of enzyme BVU5 incubation for 1 h during 2 mM and 10 mM different metal ions. (C) Decolorization of RB5 by BUV5 in the presence of 10% different organic solvents after an incubation for 30 min (RB5 100 mg L−1). (D) Relative activity of enzyme BVU5 incubation for1 h during 10% and 20% different organic solvents. (E) Decolorization of RB5 by BUV5 in the presence of different denaturants after an incubation for 30 min (RB5 100 mg L−1, 1 M urea, 1 M GdmCl and 0.1% SDS). (F) Relative activity of enzyme BVU5 after incubation for1 h during different denaturants. 1 T: 1 M urea, 1 M GdmCl and 0.1% SDS, 2 T: 2 M urea, 2 M GdmCl and 0.2% SDS. | |
Wastewater contains often a mixture of organic solvents, organic matter.39 Consequently, it is necessary to determine the effect of organic solvents on decolorization and enzymatic activity of BVU5. As shown in Fig. 4(C), the presence of the organic solvent isopropanol significantly inhibited the RB5 decolorization by enzyme (decolorization 12.61 ± 3.5%), acetone and ethanol. The solvent N-hexane scarcely had an inhibiting effect on RB5 decolorization (decolorization of 55.7 ± 4%), similar to ethylene glycol (decolorization of 50.71 ± 3.8%). Additionally, the relative activities of enzyme BVU5 after incubation for 1 h with different organic solvents were investigated and are displayed in Fig. 4(D). Isopropanol, acetone and ethanol had a great inhibitory effect. Only about 20% of the origin activity remained. The other organic solvents DMSO, methanol, isopentyl, ethylene glycol and N-hexane had little inhibitory effect on the relative activity of BVU5. Summarily, enzyme BVU5 had a good tolerance toward some organic solvents, which may be conducive to the degradation of azo dyes in organic solvents containing wastewater.
The effect of denaturants on decolorization of RB5 by enzyme BVU5 is presented in Fig. 4(E). All denaturants caused an inhibitory effect on decolorization of RB5, if compared to the control without any denaturant (PBS system, decolorization 57.7 ± 2.3%). The presence of the denaturant GdmCl (2 M), the decolorization decreased considerately to 14.4 ± 3.4% after incubation for 30 min, urea caused at 1 M, a decolorization of 20.3 ± 5.7% and the denaturant SDS at 0.1% produced a decolorization of 31.0 ± 6.4%. Furthermore, the relative activity of enzyme BVU5 after incubation for 1 h with different denaturants were investigated and are displayed in Fig. 4(F). A 22.6 ± 6.4% residual activity of enzyme BVU5 was reported after incubation with 2 M GdmCl. Similarly, 31.3 ± 4.6% residual activity was reported after incubation with 2 M urea and 49.7 ± 4.3% residual activity was reported for an incubation with 0.1% SDS. Only 6.2 ± 2.1% residual activity was reported for an incubation with 0.2% SDS. Verma26 found an azo reductase enzyme from Chromobacterium violaceum, which had 55% residual activity was reported with 2 M urea for a concentration, 50% activity at 2 M GdmCl and 60% residual activity offer 0.1% SDS treatment. Obviously, enzyme BVU5 has a low tolerance to high concentration of denaturants, especially it is sensitive to denaturants SDS and GdmCl.
3.6 Effect of different dyes
In order to explore the effect of different dyes with varied structure and composition on the decolorization by the enzyme BVU5, a total of 14 different dyes were tested. The dyes were azo dyes, anthraquinone dyes and triphenylmethane dyes.
After 30 min incubation remarkable differences in decolorization of the different dyes by enzyme BVU5 were noted (Table 3 and Fig. 5). As showed in Table 3, the differences agreed with their chemical structures and type of substitutions.40 Obviously, the removal efficiency for azo dyes and for triphenylmethane dyes were generally higher than those for anthraquinone dyes. Azo dyes, such as sample 1: Reactive Black 5, sample 4: Acid Orange 7, sample 5: Acid Black 1, sample 6: Acid Red 112, and sample 7: α-Naphthol Orange existed a significant color change and efficient decolorization (Fig. 6), especially α-Naphthol Orange (77.2 ± 2.5%), Reactive Black 5 (68.2 ± 3%) and Acid Red 112 (60.1 ± 1.9%). However, in the assay with sample 2: Trypan Blue and sample 3: Chlorazol Black E almost no color change was detected causing a low decolorization (Fig. 5). It has been reported that the structures of those azo dyes are complex, containing multiple bisphenyl rings and azo bonds. The latter may be difficult to degrade by the enzyme. The results reveal enzyme BVU5 can decolorize many azo dyes, but not all.
Table 3 Photos of the decolorization effect of enzyme for different dyes (100 mg L−1 dye, incubation 30 min at 30 °C)a
Time |
Dye |
Azo dyes |
Anthraquinone dyes |
Triphenylmethane dyes |
1 |
2 |
3 |
4 |
5 |
6 |
7 |
8 |
9 |
10 |
11 |
12 |
13 |
14 |
Note: azo dyes: (1) Reactive Black 5, (2) Trypan Blue, (3) Chlorazol Black E, (4) Acid Orange 7, (5) Acid Black 1, (6) Acid Red 112, (7) α-Naphthol Orange. Anthraquinone dyes: (8) Acid Blue 40, (9) Reactive Blue 4, (10) Reactive Blue 19. Triphenylmethane dyes: (11) Malachite Green, (12) Brilliantgreen, (13) Fuchsin Basic, (14) Crystal Violet. |
0 h |
 |
 |
 |
0.5 h |
 |
 |
 |
1 h |
 |
 |
 |
 |
| Fig. 5 Decolorization of different dyes (100 mg L−1 dye, incubation 30 min at 30 °C). | |
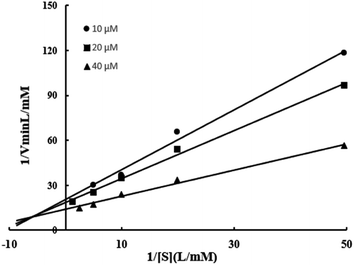 |
| Fig. 6 Relationship between 1/V and 1/[S] of BVU5 enzyme. | |
Interestingly, most of the triphenylmethane dye were degraded efficiently such as malachite green, brilliant green and fuchsin basic. In order to determine whether the decolorization is caused by enzyme BVU5 or only by coenzyme NADH, those dyes were reacted with coenzyme NADH only. The results are shown in Table S1.† Almost all azo and anthraquinone dyes had no color change, indicating that NADH alone had no effect. However, the triphenylmethane dyes showed a similar color change within 30 min with NADH alone as in the system with enzyme BVU5. Comparing these results, the conclusion can be drawn that the decolorization of triphenylmethane dyes may be caused mainly by the coenzyme NADH, and not by the enzyme BVU5.
3.7 Kinetic properties of BVU5
In this experiment, Km and Vmax of enzyme BVU5 were determined with different concentrations of RB5 and NADH. Three regression equations were calculated as y = 1.9994X + 20.684 (R2 = 0.9908), y = 1.5993x + 18.716 (R2 = 0.9951), and y = 0.8742x + 14.064 (R2 = 0.9932) according to Fig. 6. The kinetic parameters of the purified enzyme BVU5 for the degration of RB5 are shown in Table 4. The experiments used RB5 as a substrate, and NADH as an electron donor. The BVU5 enzyme conformed to the double-substrate ping–pong kinetic mechanism. As the concentration of RB5 increased, the reduction rate decreased. As can be seen from the result, RB5 could be a competitive inhibitor of NADH.
Table 4 Kinetic parameters of the purified enzyme BVU5 with substrate RB5
Kinetic parameter |
Value |
Vmax (mmol L−1 min) |
0.067 |
Ks,m (mmol L−1) |
0.092 |
Kc,m (mmol L−1) |
1.346 |
Kc,s (mmol L−1) |
0.203 |
Data in Table 4 indicates that if RB5 is used as saturation, the Michaelis constant for NADH (Kc,m) is 1.346 mmol L−1. If NADH is used as saturation, the Michaelis constant for RB5 (Ks,m) is 0.092 mmol L−1. The maximum reaction rate of enzyme BVU5 (Vmax) is 0.067 mmol L−1 min, if substrate RB5 and NADH are present at saturation concentration.
3.8 RB5 degradation pathway catalyzed by enzyme BVU5
3.8.1 UV-vis spectrophotometry analysis. The characteristic absorption peaks of dyes can be determined through UV-Vis spectrophotometry. The dye RB5 has a characteristic absorption peak at 597 nm. This is ascribed to the presence of chromophoric azo bonds as well as aryl, and naphthalene-like moieties.41 The spectra of RB5 after treatment by enzyme BVU5 from 3 min to 3 h displayed a notable decrease of the main peak (597 nm). On the other hand, a peak at 260 nm become visible (Fig. S2†). Fig. S3† shows that when NADH was added in the presence of the enzyme, the peak at 430 nm disappeared. Obviously, the chromophores in the RB5 molecule related with the reduced enzyme BVU5, causing them to be reduced and degraded for those intermediate aromatic amine compounds and other small molecules were producted.42
3.8.2 FTIR analysis. The atoms of chemical bonds or functional groups are constantly vibrating in organic molecules. If they are irradiated with infrared light, the vibration frequency is close to the vibration frequency of the atoms themselves.43,44 Therefore, chemical bonds and functional groups can be deduced based on the infrared spectra. The FTIR spectra showed absorption bands are 3000–2800 cm−1 in the region. The peaks at 2921 cm−1 and 2850 cm−1 are corresponding to symmetric and asymmetric C–H stretching vibrations of the –CH2– groups. They may result from methylene groups of an aromatic ring cleavage or of the dyes molecular structure degradation to –SO2–CH2CH2–OSO3Na (Fig. S4†). The intensity of the peak at 1635 cm−1 increased markedly, this indicates that a large amount of amide compounds were produced for RB5. The peak at 1261 cm−1 represents the stretching vibration of C–O, the peaks at 1078 cm−1 and 1063 cm−1 represents the vibration of CN in C–NH2, and the peak at 750 cm−1 represents the deformation vibration of CH in aromatic ring of hydrocarbons, (Fig. S5†).45–47 These results show that RB5 was degraded by enzyme BVU5 into aromatic amine compounds and other small substances.
3.8.3 LC-TOF-MS analysis. LC-TOF-MS was employed to identify the degradation products of RB5 after enzyme attack. The degradation products with the most probable structure are summarized in Table 5 according to their molecular weight. Four different products have been detected. The resulting possible reaction pathway for RB5 degradation, as catalyzed by enzyme BVU5 is proposed in Fig. 7.
Table 5 Degradation products detected by LC-TOF-MS of RB5 (100 mg L−1) after incubation with enzyme 0.3 μg BVU5 and 2.5 mM NADH at 30 °C for 30 min
Degradation products |
Chemical structure |
Mass-to-charge ratio (m/z) |
2,8-Diimino-1,7-naphthalenediol |
 |
188.19 |
2-(4-Aminobenzenesulfonyl) ethanol |
 |
201.24 |
4-Vinylsulfonyl-aniline |
 |
183.23 |
3,4,5-Trihydroxynaphthalene-2-sulfonate |
 |
255.22 |
 |
| Fig. 7 Probable degradation pathway of RB5 during incubation with enzyme BVU5. 100 mg L−1 RB5, reaction time 30 min. (*) Compounds were not detected but postulated from LC-TOF-MS results. | |
During the experiment, the color of the liquid turned to purple, after 30 min of enzyme action. Finally, the expected light-yellow color did not resume. Therefore, it seems that the RB5 molecule firstly underwent symmetric and asymmetric azo bond reductive cleavage as well as desulfonation to form those main substances, namely 3,5-diamino-4-hydroxynaphthalene-2-sulfonate (253.25 g mol−1) and 2,7,8-triaminonaphthalen-1-ol (189.22 g mol−1) (Fig. 7). Subsequently, the first converted into 3,4,5-trihydroxynaphthalene-2-sulfonate (255.22 g mol−1) by deamination, which had been detected as product in this experiment.48 Then 2,7,8-triaminonaphthalen-1-ol was converted to 2-amino-7,8-diimino-1-naphthol (187.20 g mol−1), and subsequently converted into 2,8-diimino-1,7-naphthalenediol (188.19 g mol−1) by deamination and hydroxylation in this experiment. On the other hand, 2-[(4-aminophenyl) sulfonyl] ethyl hydrogen sulfate was attacked further, resulting in the breakage of the S–O bond (i.e., desulfonation) to generate 2-(4-aminobenzenesulphonyl) ethanol (201.24 g mol−1). Afterwards, it was degraded to 4-vinylsulfonyl-aniline (183.23 g mol−1) by dehydroxylation and dehydrogenation. The intermediates 2-[(4-aminophenyl) sulfonyl] ethyl hydrogen sulfate, 2-(4-aminobenzenesulphonyl) ethanol and 4-vinylsulfonyl-aniline are often found as identifiers for RB5 degradation.49–51 This indicated that the enzyme BVU5 forms intermediate products with relatively large molecular weight by the symmetric or asymmetric cleavage of the azo bond. In comparison, bacteria can further degrade these intermediate products like Klebsiella sp. KL-1, who subsequently destroys the aromatic ring of 4-aminophenol and generates (Z)-4-amino-4-oxo-2-butenoic acid (115.09 g mol−1).21 Zhang52 found that the bacterial flora DDMZ1 produced many metabolites with low molecule in the presence of product.
The enzyme-catalyzed degradation of RB5 by enzyme BVU5 with the coenzyme NADH is deduced in Fig. 8. The catalysis of enzyme BVU5 occurs by electron transfer. The enzyme BVU5 transfer the electron from NADH to the azo bond. Through this catalysis the azo bonds are cleaved gradually to form the above discussed intermediates, 2,8-diimino-1,7-naphthalenediol, 4-vinylsulfonyl-aniline and 3,4,5-trihydroxynaphthalene-2-sulfonate. This process happens fast. The color of the dye changed significantly within 3 min, and became stable within 30 min. Thus, the enzyme BVU5 acting on RB5 mainly provides a rapid pretreatment method. It breaks quickly the key azo bonds, and provides the basis for a subsequent biological degradation.
 |
| Fig. 8 Proposed enzyme-catalyzed degradation mechanism for RB5 by enzyme BVU5 + NADH. | |
4. Conclusion
A novel azo reductase (enzyme BVU5) has been carefully investigated in this study. It was found to be capable of decolorizing RB5 under various conditions. The catalytic decolorization took place quickly, and about 80% of the RB5 could degrade within 3 min. The enzyme is usable in a wide range of temperatures, pH and salt concentrations. It has a good tolerance toward some metal ions and some organic solvents, but low tolerance to high concentrations of denaturants. It can decolorize most azo dyes, indicating it is a functional enzyme for the azo structure. It can cleave symmetric or asymmetric azo bonds to form intermediate products with relatively large molecular weight, which need subsequent biological degradation.
Conflicts of interest
There are no conflicts to declare.
Acknowledgements
The authors acknowledge the financial support by the National Key Research and Development Program of China (Grant No. 2019YFC0408304), the Fundamental Research Funds for the Central Universities (2232019D3-22), General Project of Shanghai Natural Science Foundation (21ZR1402100), Anhui Provincial Natural Science Foundation (1808085QE176), Outstanding academic and technical backbone of Suzhou University (2020XJGG06), Anhui Polytechnic University Startup Foundation for Introduced Talents (2020YQQ059), the Key Program of Anhui Polytechnic University (Xjky2020086).
References
- A. Vs, et al., An over review on recently developed techniques, mechanisms and intermediate involved in the advanced azo dye degradation for industrial applications, J. Mol. Struct., 2020, 1224, 129195 Search PubMed.
- T. A. Fang, et al., Enhanced azo dye biodegradation at high salinity by a halophilic bacterial consortium, Bioresour. Technol., 2021, 326, 124749 CrossRef PubMed.
- M. Dissanayake, et al., Mineralization of persistent azo dye pollutants by a microaerophilic tropical lake sediment mixed bacterial consortium, Environ. Adv., 2021, 3, 100038 CrossRef.
- G. Guo, et al., Development and characterization of a halo-thermophilic bacterial consortium for decolorization of azo dye, Chemosphere, 2021, 129916 CrossRef CAS PubMed.
- L. Dal Magro, et al., Pectin lyase immobilization using the glutaraldehyde chemistry increases the enzyme operation range, Enzyme Microb. Technol., 2020, 132, 109397 CrossRef CAS PubMed.
- C. D. Fernandes, et al., Fungal biosynthesis of lignin-modifying enzymes from pulp wash and Luffa cylindrica for azo dye RB5 biodecolorization using modeling by response surface methodology and artificial neural network, J. Hazard. Mater., 2020, 399, 123094 CrossRef CAS PubMed.
- A. U. Chaudhari, et al., Effective biotransformation and detoxification of anthraquinone dye reactive blue 4 by using aerobic bacterial granules, Water Res., 2017, 122, 603–613 CrossRef CAS PubMed.
- F. Tian, et al., Isolation, cloning and characterization of an azoreductase and the effect of salinity on its expression in a halophilic bacterium, Int. J. Biol. Macromol., 2019, 123, 1062–1069 CrossRef CAS PubMed.
- H. Suzuki, Remarkable diversification of bacterial azoreductases: primary sequences, structures, substrates, physiological roles, and biotechnological applications, Appl. Microbiol. Biotechnol., 2019, 103(10), 3965–3978 CrossRef CAS PubMed.
- K. Sarayu and S. Sandhya, Aerobic Biodegradation Pathway for Remazol Orange by Pseudomonas aeruginosa, Appl. Biochem. Biotechnol., 2010, 160(4), 1241–1253 CrossRef CAS PubMed.
- Y. Hong, et al., Effects of electron donors and acceptors on anaerobic reduction of azo dyes by Shewanella decolorationis S12, Appl. Microbiol. Biotechnol., 2007, 74(1), 230–238 CrossRef CAS PubMed.
- X. Meng, et al., Azo dye decolorization by Shewanella aquimarina under saline conditions, Bioresour. Technol., 2012, 114, 95–101 CrossRef CAS PubMed.
- S. A. Zahran, et al., Azoreductase activity of dye-decolorizing bacteria isolated from the human gut microbiota, Sci. Rep., 2019, 9(1), 1–14 CAS.
- J.-q. Hua and L. Yu, Cloning and characterization of a Flavin-free oxygen-insensitive azoreductase from Klebsiella oxytoca GS-4-08, Biotechnol. Lett., 2019, 41(3), 371–378 CrossRef CAS PubMed.
- A. Xz, et al., Deciphering the mechanism of carbon sources inhibiting recolorization in the removal of refractory dye: based on an untargeted LC–MS metabolomics approach, Bioresour. Technol., 2020, 307, 123248 CrossRef PubMed.
- Q. Zhang and X. Xie, Sugar sources as Co-substrates promoting the degradation of refractory dye: a comparative study, Ecotoxicol. Environ. Saf., 2019, 184, 109613 CrossRef CAS PubMed.
- F. Turkan, et al., Synthesis, biological evaluation and molecular docking of novel pyrazole derivatives as potent carbonic anhydrase and acetylcholinesterase inhibitors, Bioorg. Chem., 2019, 86, 420–427 CrossRef CAS PubMed.
- F. Erdemir, et al., 2-Hydroxyethyl substituted NHC precursors: synthesis, characterization, crystal structure and carbonic anhydrase, α-glycosidase, butyrylcholinesterase, and acetylcholinesterase inhibitory properties, J. Mol. Struct., 2018, 1155, 797–806 CrossRef CAS.
- F. Özbey, et al., Synthesis of diaryl ethers with acetylcholinesterase, butyrylcholinesterase and carbonic anhydrase inhibitory actions, J. Enzyme Inhib. Med. Chem., 2016, 31(sup2), 79–85 CrossRef PubMed.
- P. Pérez Galende, et al., Kinetics of Spanish broom peroxidase obeys a Ping-Pong Bi–Bi mechanism with competitive inhibition by substrates, Int. J. Biol. Macromol., 2015, 81, 1005–1011 CrossRef PubMed.
- X. Zheng, et al., Deciphering the mechanism of carbon sources inhibiting recolorization in the removal of refractory dye: based on an untargeted LC–MS metabolomics approach, Bioresour. Technol., 2020, 307, 123248 CrossRef CAS PubMed.
- K. Yoneda, et al., Structural and biochemical characterization of an extremely thermostable FMN-dependent NADH-indigo reductase from Bacillus smithii, Int. J. Biol. Macromol., 2020, 164, 3259–3267 CrossRef CAS PubMed.
- M. F. Abdul-Wahab, et al., Reduction of azo dyes by flavin reductase from Citrobacter freundii A1, J. Xenobiot., 2012, 3(1), 2 CrossRef.
- M. Shah, Purification and Analysis of Norcadia spp. Azoreductase, J. Appl. Environ. Microbiol., 2014, 2(5), 237–243 Search PubMed.
- R. T. Kapoor, et al., Exploiting microbial biomass in treating azo dyes contaminated wastewater: mechanism of degradation and factors affecting
microbial efficiency, J. Water Process. Eng., 2021, 43, 102255 CrossRef.
- K. Verma, et al., Biochemical characterization of a stable azoreductase enzyme from Chromobacterium violaceum: application in industrial effluent dye degradation, Int. J. Biol. Macromol., 2019, 121, 1011–1018 CrossRef CAS PubMed.
- Y. Yang, et al., Characterization of an efficient catalytic and organic solvent-tolerant azoreductase toward methyl red from Shewanella oneidensis MR-1, Environ. Sci. Pollut. Res., 2013, 20(5), 3232–3239 CrossRef CAS PubMed.
- L.-L. Lu, et al., Construction, expression and characterization of fusion enzyme containing azoreductase and glucose 1-dehydrogenase for dye removal, Int. Biodeterior. Biodegrad., 2014, 87, 81–86 CrossRef CAS.
- R. Al-Tohamy, et al., Ecofriendly biodegradation of Reactive Black 5 by newly isolated Sterigmatomyces halophilus SSA1575, valued for textile azo dye wastewater processing and detoxification, Sci. Rep., 2020, 10(1), 12370 CrossRef CAS PubMed.
- A. A. Raut, et al., Biotreatment of paper mill effluent using alkaliphilic Rhizobium sp. NCIM 5590 isolated from meteoric alkaline Lonar Lake, Buldhana District, Maharashtra, India, Lakes Reservoirs, 2018, 23(2), 130–138 CrossRef CAS.
- M. S. Uddin, et al., Biodecolorization of azo dye acid red B under high salinity condition, Bull. Environ. Contam. Toxicol., 2007, 79(4), 440–444 CrossRef CAS PubMed.
- U. M. Kocyigit, P. Taslimi and İ. Gulçin, Characterization and inhibition effects of some metal ions on carbonic anhydrase enzyme from Kangal Akkaraman sheep, J. Biochem. Mol. Toxicol., 2018, 32(8), e22172 CrossRef PubMed.
- M. Kucuk and İ. Gulcin, Purification and characterization of the carbonic anhydrase enzyme from Black Sea trout (Salmo trutta Labrax Coruhensis) kidney and inhibition effects of some metal ions on enzyme activity, Environ. Toxicol. Pharmacol., 2016, 44, 134–139 CrossRef CAS PubMed.
- C. Caglayan, et al., Purification and characterization of the carbonic anhydrase enzyme from horse mackerel (Trachurus trachurus) muscle and the impact of some metal ions and pesticides on enzyme activity, Comp. Biochem. Physiol., Part C: Toxicol. Pharmacol., 2019, 226, 108605 CAS.
- C. Zhang, et al., Purification and Characterization of a Temperature- and pH-Stable Laccase from the Spores of Bacillus vallismortis fmb-103 and Its Application in the Degradation of Malachite Green, J. Agric. Food Chem., 2013, 61(23), 5468–5473 CrossRef CAS PubMed.
- M. Lorenzo, et al., Inhibition of laccase activity from Trametes versicolor by heavy metals and organic compounds, Chemosphere, 2005, 60(8), 1124–1128 CrossRef CAS PubMed.
- S. Samir, et al., Performance of a newly isolated salt-tolerant yeast strain Sterigmatomyces halophilus SSA-1575 for azo dye decolorization and detoxification, Front. Microbiol., 2020, 11, 1163 CrossRef PubMed.
- Z. Ming, et al., Biodegradation of methyl red by Bacillus sp. strain UN2: decolorization capacity, metabolites characterization, and enzyme analysis, Environ. Sci. Pollut. Res. Int., 2014, 21(9), 6136–6145 CrossRef PubMed.
- V. García, et al., From waste treatment to resource efficiency in the chemical industry: recovery of organic solvents from waters containing electrolytes by pervaporation, J. Cleaner Prod., 2013, 39, 146–153 CrossRef.
- Z. Khan, et al., Microaerophilic degradation of sulphonated azo dye – reactive red 195 by bacterial consortium AR1 through co-metabolism, Int. Biodeterior. Biodegrad., 2014, 94, 167–175 CrossRef CAS.
- L. A. Adnan, et al., Metabolites characterisation of laccase mediated reactive black 5 biodegradation by fast growing ascomycete fungus Trichoderma atroviride F03, Int. Biodeterior. Biodegrad., 2015, 104, 274–282 CrossRef CAS.
- S. J. Eun, et al., Simple Preparation of Diaphorase/Polysiloxane Viologen Polymer Modified Electrode for Sensing NAD and NADH, J. Electrochem. Sci. Technol., 2011, 2(3), 163–167 CrossRef.
- R. Rathour, et al., Microaerophilic biodegradation of raw textile effluent by synergistic activity of bacterial community DR4, J. Environ. Manage., 2019, 250, 109549 CrossRef CAS PubMed.
- V. V. Dawkar, et al., Decolorization and detoxification of sulphonated azo dye Red HE7B by Bacillus sp. VUS, World J. Microbiol. Biotechnol., 2010, 26(5), 909–916 CrossRef.
- A. Dalvand, et al., Reactive Dye Adsorption from Aqueous Solution on HPEI-Modified Fe3O4 Nanoparticle as a Superadsorbent: Characterization, Modeling, and Optimization, J. Polym. Environ., 2018, 26(8), 3470–3483 CrossRef CAS.
- A. J. Méndez-Martínez, et al., Electrochemical reduction and oxidation pathways for Reactive Black 5 dye using nickel electrodes in divided and undivided cells, Electrochim. Acta, 2012, 59, 140–149 CrossRef.
- A. Naresh Kumar, Biomineralization of azo dye bearing wastewater in periodic discontinuous batch reactor: effect of microaerophilic conditions on treatment efficiency, Bioresour. Technol., 2015, 188, 56–64 CrossRef CAS PubMed.
- L. A. Adnan, et al., Metabolites characterisation of laccase mediated Reactive Black 5 biodegradation by fast growing
ascomycete fungus Trichoderma atroviride F03, Int. Biodeterior. Biodegrad., 2015, 104, 274–282 CrossRef CAS.
- R. C. Bonugli-Santos, et al., Enhanced textile dye decolorization by marine-derived basidiomycete Peniophora sp. CBMAI 1063 using integrated statistical design, Environ. Sci. Pollut. Res., 2016, 23(9), 8659–8668 CrossRef CAS PubMed.
- B. R. Shah and U. D. Patel, Reductive photocatalytic decolourization of an azo dye Reactive Black 5 using TiO2: mechanism and role of reductive species, Desalin. Water Treat., 2018, 130, 214–225 CrossRef CAS.
- A. Rehorek and A. Plum, Characterization of sulfonated azo dyes and aromatic amines by pyrolysis gas chromatography/mass spectrometry, Anal. Bioanal. Chem., 2007, 388(8), 1653–1662 CrossRef CAS PubMed.
- Q. Zhang, et al., Fructose as an additional co-metabolite promotes refractory dye degradation: performance and mechanism, Bioresour. Technol., 2019, 280, 430–440 CrossRef CAS PubMed.
Footnote |
† Electronic supplementary information (ESI) available. See DOI: 10.1039/d1ra08090c |
|
This journal is © The Royal Society of Chemistry 2022 |
Click here to see how this site uses Cookies. View our privacy policy here.