DOI:
10.1039/D1RA07321D
(Paper)
RSC Adv., 2022,
12, 1051-1061
Immobilization of Ag(0) nanoparticles on quaternary ammonium functionalized polyacrylonitrile fiber as a highly active catalyst for 4-nitrophenol reduction†
Received
1st October 2021
, Accepted 26th November 2021
First published on 5th January 2022
Abstract
Ag(0) nanoparticles were immobilized on various pyridine salt, imidazole salt and quaternary ammonium functionalized polyacrylonitrile fibers (PANFs) to prepare Ag(0)-immobilized fiber catalysts. The catalytic activities of these immobilized catalysts for 4-nitrophenol (4-NP) reduction were detected. Among them, the quaternary ammonium fiber with butyl group immobilized Ag(0) nanoparticle catalyst PANQA-C4F-Ag(0) showed the best catalytic activity, and can effectively catalyze 4-nitrophenol (4-NP) reduction with a high conversation rate of 99.6%. Furthermore, PANQA-C4F-Ag(0) can be easily recovered, and it was reused 20 times with little decrease in catalytic activity and moderate Ag retention (53.5%). Notably, the cationic groups in the functionalized fibers can stabilize Ag(0) nanoparticles through electrostatic interactions and steric effects, and play an important role in phase transfer catalysis. Accordingly, possible mechanisms for the 4-NP reduction catalyzed by PANQA-C4F-Ag(0) were proposed.
1. Introduction
Nitrophenol is usually used as a raw material for the preparation of pesticides and dyes.1–4 Due to its high toxicity and difficulty in degradation, nitrophenol has become a common organic pollutant in waste water.5–8 Notably, 4-NP is an important kind of nitrophenol compound. It has carcinogenicity, teratogenicity and embryonic cytotoxicity, which can damage the blood, organs and nervous systems of animals, thereby endangering human health.9–12 In recent years, 4-NP has been regarded as a hazardous pollutant and toxic waste by the U.S. environmental protection agency, and it also became one of the 68 pollutants that are prioritized for treatment in China.
According to recent research, many methods were used to treat 4-NP sewage, including photocatalytic degradation,13,14 catalytic oxidation,15 catalytic reduction,16,17 biodegradation,18 etc. Among them, researchers discovered that 4-NP can be reduced to less toxic 4-aminophenol (4-AP) through using appropriate reducing agents and nano metal catalysts. Therefore, catalytic reduction treatment of 4-NP has high value in environmental protection.
Compared with various precious metals, silver has the advantages of cheap and easy to obtain. Additionally, the supported Ag(0) catalysts have the advantages of high dispersion of Ag(0) nanoparticles, high catalytic activity and easy recovery. Therefore, supported Ag(0) catalysts are more suitable for catalyzing the reduction reaction of 4-NP. Recently, many different materials including SBA-15,19 GO,20 polymers,21,22 MCM-41,23,24 magnetic Fe3O4,25,26 SiO2 (ref. 27 and 28) and MOFs29,30 have been used as supports for preparation of immobilized silver catalysts. These heterogeneous silver catalysts have many advantages, such as high efficiency and simple post-treatment steps. Therefore, preparation of novel heterogeneous Ag(0) nanoparticle catalysts has high research value.
It has been verified that quaternary ammonium salts can effectively stabilize different metal nanoparticles.31–35 Quaternary ammonium salts also can stabilize Ag(0) nanoparticles through electrostatic interactions and steric effects.36–40 In this work, various of pyridine salt, imidazole salt and quaternary ammonium functionalized PANFs were prepared by amination and quaternization of PANF. Ag(I) and Ag(0) nanoparticles were then supported into these pre-functionalized fibers through chelating by –CN in PANF and then reduction. The structures of various functionalized fibers were shown in Scheme 1. The catalytic activities of these Ag(0)-functionalized fibers were tested, and the best fiber catalyst was characterized.
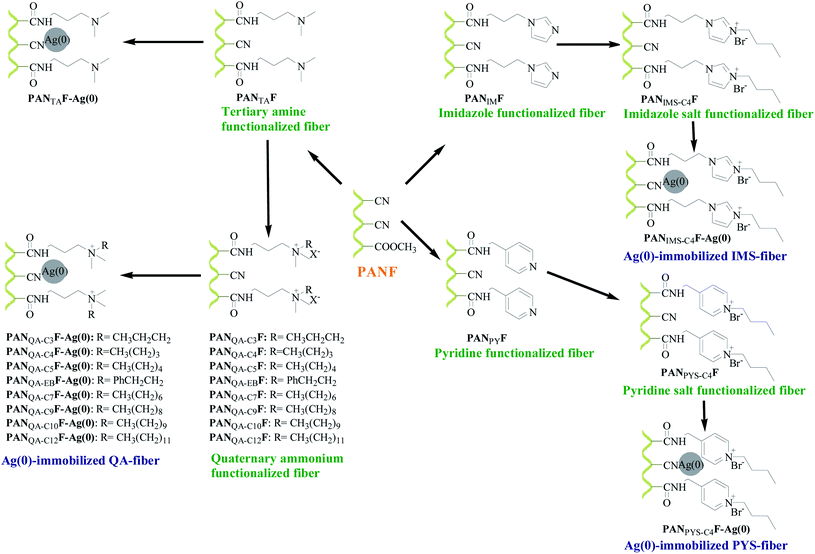 |
| Scheme 1 Various PANF-immobilized silver catalystsa. a PANTAF is tertiary amine functionalized PANF; PANPYF is pyridine functionalized fiber; PANIMF is imidazole functionalized fiber; PANPYSF is pyridine salt functionalized fiber; PANIMSF is imidazole salt functionalized fiber; PANQA-C3F, PANQA-C4F, PANQA-C5F, PANQA-EBF, PANQA-C7F, PANQA-C9F, PANQA-C10F and PANQA-C12F are ammonium functionalized fibers. PANTAF-Ag(0), PANIMSF-Ag(0), PANPYSF-Ag(0) and PANQAF-Ag(0) are the corresponding fiber-supported Ag(0) nanoparticle catalysts. | |
2. Results and discussion
2.1 Preparation and characterization of PANF-immobilized Ag(0) catalysts
Different of PANF-immobilized Ag(0) catalysts have been successfully prepared by chelation of various functionalized PANFs and AgNO3. The functionality of tertiary aminated fiber support was accurately quantified by acid–base titration. The functionalities of PANIMF, PANPYF, PANQAF, PANIMSF and PANPYSF were estimated by w%/[M × (1 + w%)] × 1000, where M is the molecular weight of corresponding moieties and w% is the weight gain of pre-functionalized PANFs including PANF, PANTAF, PANIMF and PANPYF. Additionally, the functionalities of Ag(0) nanoparticles functionalized PANFs were exactly measured by inductively coupled plasma optical emission spectrometry (ICP-AES). The measured functionality of silver-functionalized PANFs were 5.946–21.700 × 10−3 mmol g−1, which verified that Ag(0) nanoparticles were successfully immobilized into surface of fibers. The results of functionality of different fibers are shown in Table 1.
Table 1 The functionalities of the various functionalized fibers
Entry |
Functionalized fibers |
Amount of functionality (mmol g−1) |
Entry |
Functionalized fibers |
Amount of functionality (10−3 mmol g−1) |
1 |
PANTAF |
1.985 |
14 |
PANTAF-Ag(0) |
6.296 |
2 |
PANIMF |
1.010 |
15 |
PANQA-C3F-Ag(0) |
7.222 |
3 |
PANPYF |
1.214 |
16 |
PANQA-C4F-Ag(0) |
5.946 |
4 |
PANQA-C3F |
1.883 |
17 |
PANQA-C4F-Ag(0) |
17.892 |
5 |
PANQA-C4F |
1.757 |
18 |
PANQA-C4F-Ag(0) |
28.775 |
6 |
PANQA-C5F |
1.432 |
19 |
PANQA-C5F-Ag(0) |
8.610 |
7 |
PANQA-EBF |
1.523 |
20 |
PANQA-EBF-Ag(0) |
12.010 |
8 |
PANQA-C7F |
1.664 |
21 |
PANQA-C7F-Ag(0) |
9.260 |
9 |
PANQA-C9F |
1.304 |
22 |
PANQA-C9F-Ag(0) |
8.980 |
10 |
PANQA-C10F |
1.288 |
23 |
PANQA-C10F-Ag(0) |
13.89 |
11 |
PANQA-C12F |
1.310 |
24 |
PANQA-C12F-Ag(0) |
21.700 |
12 |
PANIM-C4F |
0.869 |
25 |
PANIM-C4F-Ag(0) |
9.800 |
13 |
PANPY-C4F |
1.064 |
26 |
PANPY-C4F-Ag(0) |
13.900 |
Fourier transform infrared spectroscopy (FTIR) was used to investigate the fiber structures. The FTIR spectra of PANF, PANTAF, PANQA-C4F and PANQA-C4F-Ag(0) are shown in the Fig. 1. The strong adsorption peak at 2243 cm−1 was owned to the stretching vibration of C
N. Compared with PANF, the adsorption peak of C
N in the functionalized fibers were weakened but did not disappear, showing that the functionalized steps did not damage the skeleton structure of PANF (curve a). The original fiber has an adsorption peak at 1734 cm−1 which is attributed to the stretching vibration of C
O in methoxycarbonyl. After modification, PANTAF has new adsorption peak at 1670 cm−1 which is attributed to C
O stretching vibration in carbamoyl, suggesting that the amide was generated by aminolysis of methoxycarbonyl and cyano groups (curves a, b). Each functionalized fiber has an adsorption peak at 3000–3500 cm−1, which is contributed to the stretching vibration of N–H in the amide (curve b–d). Moreover, the FTIR spectrum of PANQA-C4F is similar to that of PANTAF because the quaternary ammonium groups do not have characteristic IR absorption (curve c). Furthermore, compared with PANQA-C4F, the spectrum of PANQA-C4F-Ag(0) did not change significantly, indicating that PANQAF can maintain the integral structure after modification (curve d).
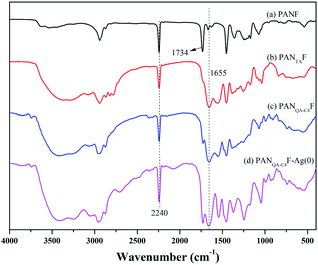 |
| Fig. 1 The FTIR spectra of (a) PANF, (b) PANTAF, (c) PANQA-C4F and (d) PANQA-C4F-Ag(0). | |
The X-ray diffraction (XRD) was utilized to detect the crystal structure of the fibers and the XRD spectra of PANF, PANQA-C4F-Ag(0) are shown in Fig. 2. Both original fiber and functional fiber have two strong diffraction peaks at 17.0° and 29.5°, which are attributed to the (1 0 0) and (1 1 0) crystallographic planes of hexagonal lattice of PANF (curve a–c). Moreover, the weak peak at 2θ = 38.1° are attributed to (1 1 1) facets of Ag(0) nanoparticle, which indicates that the Ag(0) nanoparticle was successfully supported into fiber (curve b). Noteworthily, the characteristic diffraction peak of silver still exists in the recycled catalyst, which proves that the Ag(0) nanoparticles in PANQA-C4F-Ag(0) do not lost seriously after 20th runs (curve c).37
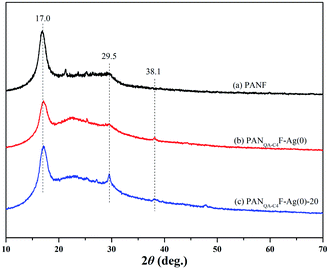 |
| Fig. 2 The XRD spectra of (a) PANF, (b) PANQA-C4F-Ag(0) and (c) PANQA-C4F-Ag(0)-20. | |
The elemental analysis (EA) was used to determine the composition of elements of different fibers and the EA data of PANF, PANTAF, PANQA-C4F and PANQA-C4F-Ag(0) are shown in Table 2. Compared with original fiber, PANTAF has less C and N contents and higher H content, the reason is that the immobilized CONHCH2CH2CH2N(CH3)2 moiety has lower C and N contents and more H content (entries 1 and 2). PANQA-C4F has lower C, H, and N contents than PANTAF because of the increased proportion of Br (entry 3). The C, H and N contents of PANQA-C4F-Ag(0) slightly increased because Br− was partially replaced by NO3− (entry 4). EA data proves that fiber has been successfully immobilized.
Table 2 The elemental analysis data
Entry |
Fiber |
Elemental analysis data |
C (%) |
H (%) |
N (%) |
1 |
PANF |
66.19 |
5.98 |
24.38 |
2 |
PANTAF |
58.69 |
7.17 |
20.65 |
3 |
PANQA-C4F |
51.42 |
6.59 |
15.88 |
4 |
PANQA-C4F-Ag(0) |
53.90 |
7.17 |
16.81 |
The X-ray photoelectron spectroscopy (XPS) was applied to detect the chemical properties of the surface of PANQA-C4F-Ag(0), and the result is shown in Fig. 3. The survey spectrum of PANQA-C4F-Ag(0) has obvious peaks of Ag, Br, N, O and C (Fig. 3a). The peaks at 373.4 and 367.4 eV correspond to Ag 3d3/2 and Ag 3d5/2 of Ag(0), respectively, proving that the Ag(0) nanoparticles have been successfully modified into the fiber (Fig. 3b).37 Additionally, the corresponding Br 3d spectrum of PANQA-C4F-Ag(0) has a peak at 67.7 eV, which can be attributed to the Br− in PANQA-C4F-Ag(0) (Fig. 3c). Moreover, there are two peaks at 402.3 and 399.5 eV corresponding to N 1s XPS spectrum of PANQA-C4F-Ag(0), which can be owned to the O
C–N and C–N bonds of the PANQA-C4F-Ag(0), respectively (Fig. 3d). Furthermore, the peak at 529–535 eV was attributed to the O 1s in PANQA-C4F-Ag(0) (Fig. 3e). The peaks at 288.2, 286.4 and 284.8 eV corresponding to C 1s XPS spectrum of PANQA-C4F-Ag(0) owes to the N–C
O, N–H and C
N bonds, respectively (Fig. 3f), and the peaks at 980 eV corresponds to C (KLL) Auger electron spectrum (Fig. 3a). XPS spectra further verifies the successfully immobilization of PANQA-C4F-Ag(0).41
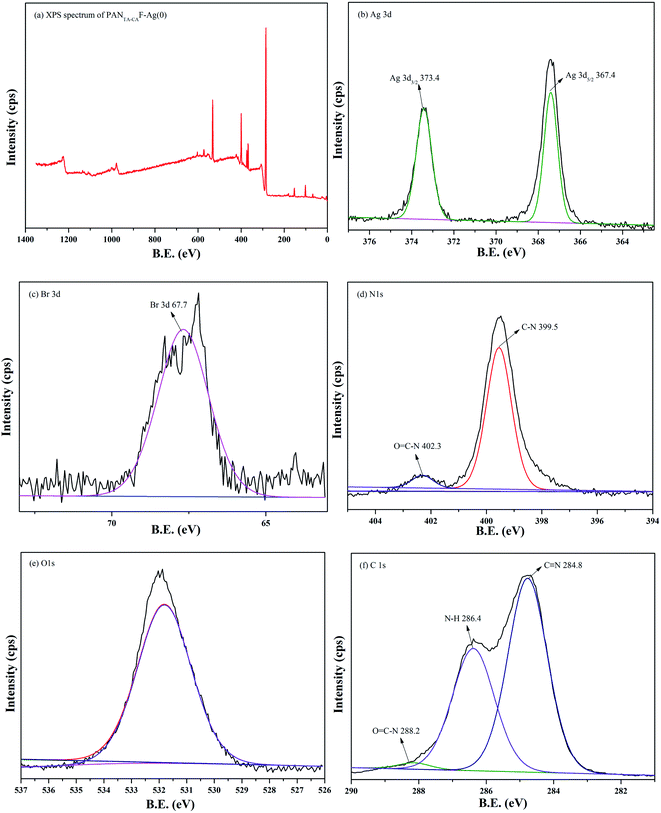 |
| Fig. 3 (a) XPS survey spectrum of PANQA-C4F-Ag(0), (b) Ag 3d spectra of PANQA-C4F-Ag(0), (c) Br 3d spectra of PANQA-C4F-Ag(0), (d) N 1s spectra of PANQA-C4F-Ag(0), (e) O 1s spectra of PANQA-C4F-Ag(0) and (f) C 1s spectra of PANQA-C4F-Ag(0). | |
Scanning electron microscopy (SEM) images was used to observe the microscopic appearance of the fiber surfaces, so the SEM spectra of various functionalized PANFs are shown in Fig. 4. The functionalized fibers has slightly rough surface under 20
000× magnification, confirming that its structure was almost not damaged (Fig. 4b–d). Compared with fresh PANQA-C4F-Ag(0), the PANQA-C4F-Ag(0) reused twenty times was not significant broken under 20
000× magnification, confirming that PANQA-C4F-Ag(0) can be recycled multiple times because of its good strength (Fig. 4e).
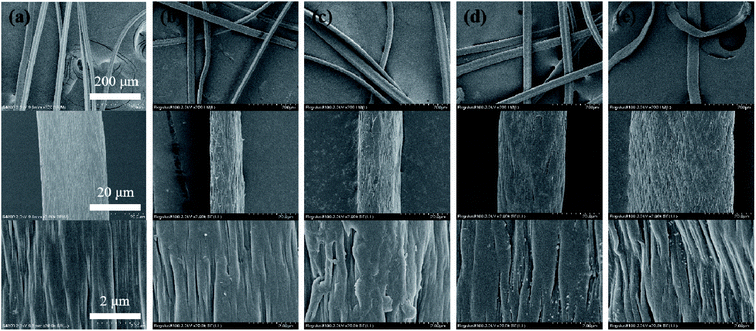 |
| Fig. 4 The SEM images of (a) PANF, (b) PANTAF, (c) PANQA-C4F, (d) PANQA-C4F-Ag(0) and (e) PANQA-C4F-Ag(0)-20. Top row 200×, middle row 2000× and bottom row 20 000× magnification. | |
Transmission electron microscopy (TEM) was applied to observe the particle size of the Ag(0) nanoparticles in the PANQA-C4F-Ag(0). The TEM images of PANQA-C4F-Ag(0)-98
:
1 (the molar ratio of the quaternary ammonium group to the Ag(0) nanoparticle in the catalyst PANQA-C4F-Ag(0) is 98
:
1) under different magnifications are shown in Fig. 5. The particle size of Ag(0) nanoparticle is about 20–30 nm in fresh PANQA-C4F-Ag(0), and the size of Ag(0) nanoparticles in PANQA-C12F-Ag(0) is about 10–25 nm, indicating that the fiber catalyst with hydrophobic aliphatic chains is beneficial to stabilize Ag(0) nanoparticles (Fig. S1†). The size of Ag(0) nanoparticles in PANQA-C4F-Ag(0) used 20 times is still less than 100 nm, suggesting that the nano-sized Ag(0) functionalized fiber catalyst was successfully prepared and has good recyclability.
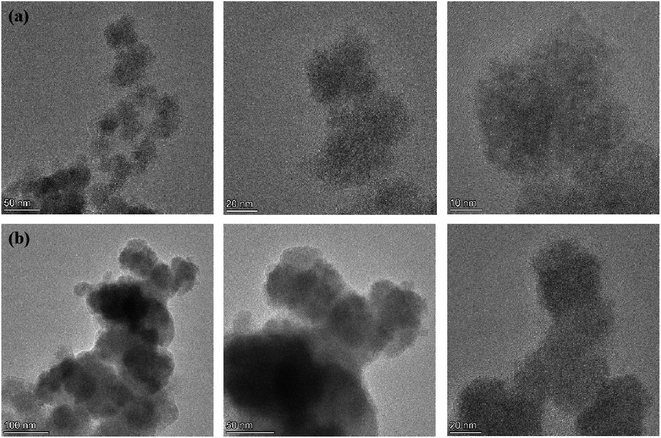 |
| Fig. 5 The TEM images of PANQA-C4F-Ag(0)-98 : 1, (a) the fresh fiber catalyst and (b) the fiber catalyst used 20 times. | |
The thermal stability of PANF, PANTAF, PANQA-C4F and PANQA-C4F-Ag(0) were detected by thermogravimetry analysis (TGA) (Fig. 6). All of the functionalized fibers exhibit lower thermal weight loss and excellent stability under 200 °C, indicating that the fiber catalyst is suitable for general conditions of many reactions (Fig. 6b–d).
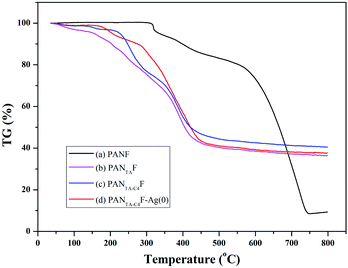 |
| Fig. 6 The thermal stability of (a) PANF, (b) PANTAF, (c) PANQA-C4F and (d) PANQA-C4F-Ag(0). | |
2.2 Catalytic activity of PANF-supported Ag catalysts
2.2.1 Catalyst activity of the functionalized fibers. Various of fiber-supported Ag(0) nanoparticle catalysts were used to catalyze the reduction of 4-NP, and the results are shown in Table 3. Functionalized fibers with similar quaternary ammonium salt/silver ratios according to close to 300
:
1, 200
:
1, 100
:
1, 60
:
1 are classified into groups one (entries 1–3), group two (entries 4–6), group three (entries 7–12) and group four (entries 13–15), respectively, and the catalytic activities of these fiber catalysts have been detected. The result shows that the quaternary ammonium fiber with butyl group immobilized Ag(0) nanoparticles catalyst PANQA-C4F-Ag(0) showed the best catalytic activity (entries 2, 4, 8 and 13). Compared with PANTAF-Ag(0), PANQA-C4F-Ag(0) has higher catalytic activity, which proves that introduction of quaternary ammonium groups improves the catalytic activity of the fiber catalyst (entries 2, 3, 4, 8 and 13). Through adjusting the ratio of quaternary ammonium salt group and Ag(0) nanoparticle in fiber PANQA-C4F-Ag(0), we found that the fiber PANQA-C4F-Ag(0) has the best catalytic activity when the ratio of quaternary ammonium salt group to silver is close to 100
:
1 (98
:
1) (entry 8). Additionally, different fibers are used to adsorb 4-NP without NaBH4, the result shows that fiber catalysts with short fatty chains have better adsorption effect. The reason can be explainer that 4-NP has good hydrophilicity under alkaline conditions, it is not conducive to the enrichment of 4-NP when the surface layer of the fiber is too hydrophobic (entries 16–22). Above experiments can explain why the PANQA-C4F has the best catalytic activity. Through the above discussion, the catalyst PANQA-C4F-Ag(0)-98
:
1 (the ratio of quaternary ammonium salt to Ag(0) nanoparticle is close to 98
:
1) was selected for subsequent experiments.
Table 3 The catalytic activities of different fiber catalysts for the reduction of 4-NPab
Entry |
Catalyst |
The content of Ag(0) (10−3 mmol g−1) |
The content of QA (mmol g−1) |
The ratio of QA to Ag |
Conv. (%) |
General conditions: 0.5 mol% catalyst, 50 eq. NaBH4, 40 mL, 1 mmol L−1 4-NP (aq), and the reaction was carried out in a single neck flask at 25 °C for 6 h. The yields were detected by the standard curve method of UV spectroscopy. General conditions. |
1 |
PANQA-C3F-Ag(0) |
7.222 |
1.883 |
261 : 1 |
23.4 |
2 |
PANQA-C4F-Ag(0) |
5.160 |
1.757 |
296 : 1 |
24.9 |
3 |
PANTAF-Ag(0) |
6.296 |
1.985 |
311 : 1 |
13.5 |
4 |
PANQA-C4F-Ag(0) |
9.100 |
1.757 |
193 : 1 |
36.5 |
5 |
PANQA-C5F-Ag(0) |
8.610 |
1.432 |
166 : 1 |
8.5 |
6 |
PANQA-C7F-Ag(0) |
9.260 |
1.664 |
180 : 1 |
12.3 |
7 |
PANTAF-Ag(0) |
15.480 |
1.985 |
128 : 1 |
16.2 |
8 |
PANQA-C4F-Ag(0) |
17.892 |
1.757 |
98 : 1 |
62.1 |
9 |
PANQA-EBF-Ag(0) |
12.010 |
1.523 |
127 : 1 |
3.5 |
10 |
PANQA-C9F-Ag(0) |
8.980 |
1.304 |
145 : 1 |
4.6 |
11 |
PANQA-C10F-Ag(0) |
13.890 |
1.288 |
93 : 1 |
33.2 |
12 |
PANIMS-C4F-Ag(0) |
9.800 |
0.869 |
89 : 1 |
17.7 |
13 |
PANQA-C4F-Ag(0) |
28.760 |
1.757 |
61 : 1 |
51.2 |
14 |
PANQA-C12F-Ag(0) |
21.700 |
1.310 |
60 : 1 |
6.7 |
15 |
PANPY-C4F-Ag(0) |
13.900 |
1.064 |
77 : 1 |
0.3 |
16c |
PANTAF |
6.296 |
1.985 |
311 : 1 |
N. R. |
17c |
PANQA-C3F-Ag(0) |
7.222 |
1.883 |
261 : 1 |
21.2 |
18c |
PANQA-C4F-Ag(0) |
9.100 |
1.757 |
193 : 1 |
27.8 |
19c |
PANQA-C5F-Ag(0) |
8.610 |
1.432 |
166 : 1 |
7.1 |
20c |
PANQA-C7F-Ag(0) |
9.260 |
1.664 |
180 : 1 |
8.9 |
21c |
PANQA-C10F-Ag(0) |
13.890 |
1.288 |
93 : 1 |
5.7 |
22c |
PANQA-C12F-Ag(0) |
21.700 |
1.310 |
60 : 1 |
2.3 |
2.2.2 4-NP reduction catalyzed by PANQA-C4F-Ag(0). The conditions of 4-NP reduction reaction catalyzed by PANQA-C4F-Ag(0) were then optimized, and the results are shown in Table 4. Using different amounts of catalyst to catalyze the 4-NP reduction, the optimized removal rate reaches 99.6% when the catalyst loading is 1 mol% and 1.5 mol% (entries 5–7). In addition, the low (50 eq.) or high (200 eq.) dosage of NaBH4 is not conducive to the progress of the reaction, and the best usage of NaBH4 is 100 eq. (entries 3, 5 and 8). The 4-NP conversion rate is low when there is no Ag(0) nanoparticles in the fiber (entry 13). Neither high nor low temperature is not conducive to the reaction, and the best temperature is 25 °C (entries 8–10). Additionally, corresponding control experiments were conducted. The PANQA-C4F-Ag(0) shows adsorption rate of 18.9% and 27.8% for 4-NP at 25 °C and 35 °C, which proves that high temperature is not conducive to the accumulation of 4-NP on the surface of the fiber and reduces the catalytic activity of PANQA-C4F-Ag(0) (entries 11 and 12). However, when 100 eq. NaBF4 existed in reaction system, the adsorption capacity of PANQA-C4F-Ag(0) for 4-NP decreased significantly, which indicates that competing anions (BF4−) in solution can interfere with the enrichment of 4-NP on the fiber(entry 14), and indirectly explains why the catalytic efficiency of PANF decreases when the amount of NaBH4 is too much (entry 8). In summary, the reaction could reach the highest removal rate of 99.6% under the conditions of 1 mol% catalyst loading, 100 eq. NaBH4, 25 °C and 90 min (entry 5).
Table 4 The catalytic activities of PANQA-C4F-Ag(0) in the different reaction conditions for the reduction of 4-NP
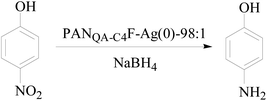
|
Entry |
Catalyst |
Catalyst loading |
Dosage of NaBH4 |
Temp. (°C) |
Time (min) |
Removal rate (%) |
The pH value of the 4-NP solution is pH = 10. 100 eq. NaBF4 was added in the reaction system. |
1 |
PANQA-C4F-Ag(0) |
0.1 mol% |
50 eq. |
25 |
360 |
3.7 |
2 |
PANQA-C4F-Ag(0) |
0.5 mol% |
50 eq. |
25 |
360 |
62.1 |
3 |
PANQA-C4F-Ag(0) |
1 mol% |
50 eq. |
25 |
360 |
97.2 |
4 |
PANQA-C4F-Ag(0) |
1.5 mol% |
50 eq. |
25 |
360 |
99.1 |
5 |
PANQA-C4F-Ag(0) |
1 mol% |
100 eq. |
25 |
90 |
99.6 |
6 |
PANQA-C4F-Ag(0) |
0.5 mol% |
100 eq. |
25 |
90 |
97.7 |
7 |
PANQA-C4F-Ag(0) |
1.5 mol% |
100 eq. |
25 |
45 |
99.6 |
8 |
PANQA-C4F-Ag(0) |
1 mol% |
200 eq. |
25 |
150 |
99.6 |
9 |
PANQA-C4F-Ag(0) |
1 mol% |
100 eq. |
15 |
150 |
97.9 |
10 |
PANQA-C4F-Ag(0) |
1 mol% |
100 eq. |
35 |
110 |
99.6 |
11a |
PANQA-C4F-Ag(0) |
1 eq. |
— |
25 |
360 |
27.8 |
12a |
PANQA-C4F-Ag(0) |
1 eq. |
— |
35 |
360 |
18.9 |
13 |
PANQA-C4F |
1 eq. |
100 eq. |
25 |
600 |
14.1 |
14ab |
PANQA-C4F-Ag(0) |
1 eq. |
— |
25 |
360 |
n.r |
2.2.3 Reaction kinetic of PANQA-C4F-Ag(0) for 4-NP. The reaction kinetic of 4-NP reduction catalyzed by PANQA-C4F-Ag(0) has been tested. As shown in Fig. 7, the catalysis process conducted slowly within the beginning to 10 min, which can be explained that the 4-NP hardly enrich from the solution to the surface of the fiber within short time. The conversion rate of 4-NP catalyzed by PANQA-C4F-Ag(0) increased rapidly within 10 min to 45 min, and then leveled off after 45 min. The reaction can reach the highest conversion rate of 4-NP (99.6%) at 25 °C. The UV spectra of the 4-NP under different reaction time are shown in Fig. 7b. It can be observed that the absorption peak at 400 nm corresponding to 4-NP decreases significantly as time goes by.
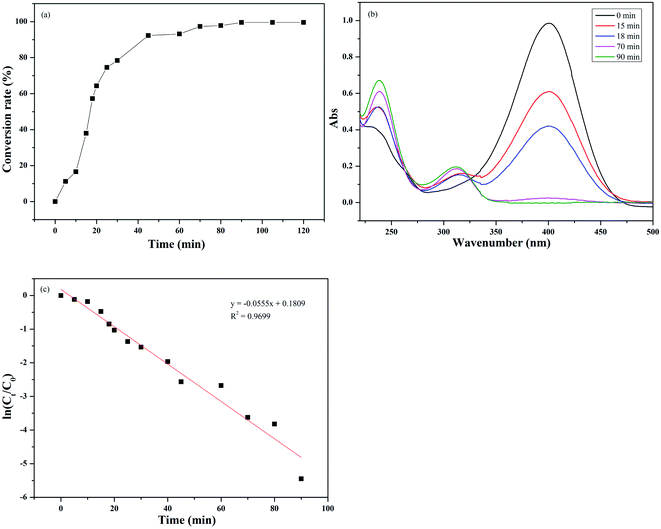 |
| Fig. 7 (a) Catalysis kinetic of PANQA-C4F-Ag(0) for the reduction of 4-NP at room temperature, (b) UV spectra of reaction system at different times, (c) the plot of ln(Ct/C0) vs. time. | |
Furthermore, the reaction process can be regarded as a first-order kinetic reaction, and the related equations are shown in eqn (1) and (2).
|
ln(Ct/C0) = ln(At/A0) = −kappt
| (2) |
where
kapp is the apparent rate constant (10
−3 min
−1). The plots of ln(C
t/
C0)
vs. time are shown in
Fig. 7c, and the
kapp of the reaction can be calculated to be 55.5 (10
−3 min
−1), suggesting that the PAN
QA-C4F-Ag(0) has good catalytic activity.
2.2.4 Reusability experiment. Reusability is an important factor in evaluating the performance of heterogeneous catalysts. The reduction of 4-NP was chosen as a model reaction to detect the recycle ability of the catalyst PANQA-C4F-Ag(0). As shown in Fig. 8, after the catalyst was used 20 times, the conversion rate of 4-NP was higher than 99%. As shown in Fig. S3,† the reaction kinetic of 4-NP reduction catalyzed by PANQA-C4F-Ag(0)-20 has been tested, the reaction kinetic curve reaches equilibrium within 90 minutes. The functionality of Ag in PANQA-C4F-Ag(0)-20 detected by ICP-AES is 9.579 × 10−3 mmol g−1, which is moderately lower than that of fresh catalyst PANQA-C4F-Ag(0)-20 (17.892 × 10−3 mmol g−1). The excellent catalytic activity of 4-NP reduction and the moderate Ag-retention (53.5%) shows that the PANF-supported Ag(0) nanocatalyst has moderate stability and reusability.
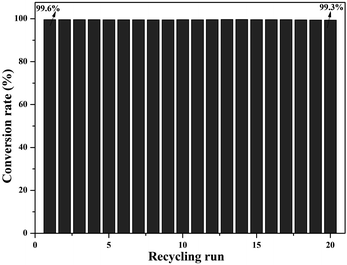 |
| Fig. 8 Reusability experiment of PANQA-C4F-Ag(0)-98 : 1a. a Reaction conditions: 4-NP (1.00 mmol L−1, 40.0 mL), 1.0 mol% PANQA-C4F-Ag(0)-98 : 1, 25 °C. The yield was obtained by UV-visible spectrophotometer. | |
2.2.5 Ag(0) nanoparticles stabilized by functionalized fiber. Preventing agglomeration of Ag(0) nanoparticles is a main factor in improving fiber-supported Ag(0) catalyst activity. As is mentioned above, the catalytic activity of quaternary ammonium fiber with butyl group immobilized Ag(0) nanoparticles catalyst PANQA-C4F-Ag(0) are better than that of PANTAF-Ag(0), because the steric and electrostatic effects of the quaternary ammonium salts in fibers are more conducive to stabilizing the nanoparticles. As shown in Scheme 2, the halogen ions can stabilize the Ag(0) nanoparticles through chelation, and the butyl chains in the quaternary ammonium salt group can disperse the Ag(0) nanoparticles through steric effect.
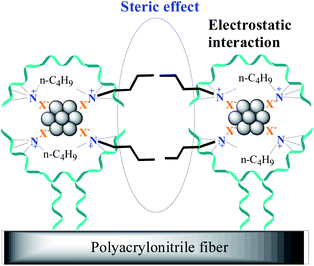 |
| Scheme 2 The stabilization of Ag(0) through steric effect and electrostatic of quaternary ammonium salts functionalized fiber. | |
2.2.6 The possible mechanisms of fiber-catalyzed 4-NP reduction. The possible mechanisms are proposed and shown in Scheme 3. In the first step, 4-NP can be easily enriched into cationic microenvironment on the surface of the fibers due to the electrostatic attraction. However, the PANQA-C4F-Ag(0) with low Ag(0) nanoparticle loading and too high quaternary ammonium salt functionality has lower catalytic activity, which can be explained by that the 4-NP is bound to the surface of fiber and cannot move freely, and the low-density silver catalytic sites cannot catalyze the conversion of more reactants. Therefore, compared with the PANQA-C4F-Ag(0)-61
:
1, PANQA-C4F-Ag(0)-193
:
1 and PANQA-C4F-Ag(0)-296
:
1, the PANQA-C4F-Ag(0)-98
:
1 has best catalytic activity. In the second step, 4-aminophenol (4-AP) is reduced to Ag (0) nanoparticles and corresponding anions return to the surface of fiber. The proposal mechanism diagram proves that the cationic microenvironment on the surface of the fiber can promote the reaction.
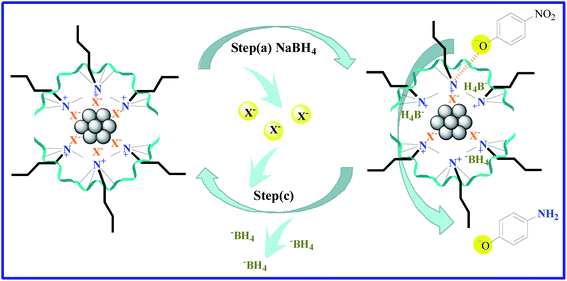 |
| Scheme 3 PANQA-C4F-Ag(0) catalyzed reduction of 4-NP. | |
2.3 Comparison of PANQA-C4F-Ag(0) with other catalytic systems
Compared with other catalysts for 4-NP reduction, fiber-supported Ag(0) nanoparticle catalyst PANQA-C4F-Ag(0) shows moderate performance. The result was shown in Table S1.† The 4-NP reduction catalyzed by PANQA-C4F-Ag(0) has the advantages of high conversion rate and good cycle performance.
3. Conclusion
Different Ag(0) nanoparticle functionalized fiber catalysts were successfully prepared. Screened by 4-NP reduction, the quaternary ammonium fiber with butyl group immobilized Ag(0) nanoparticles catalyst PANQA-C4F-Ag(0) was chosen as the optimal catalyst for subsequent experiments. PANQA-C4F-Ag(0) can efficiently catalyze the reduction of 4-NP under room temperature with high conversion rate of 99.6%, which proves that PANQA-C4F-Ag(0) has application value in the nitrophenol wastewater treatment. Additionally, possible catalytic mechanisms based on the fiber microenvironment was proposed. Furthermore, the catalytic activity of PANQA-C4F-Ag(0) for the reduction of 4-NP decreased little after 20 times of use, which show its good reusability and practical application potential (Fig. 9).
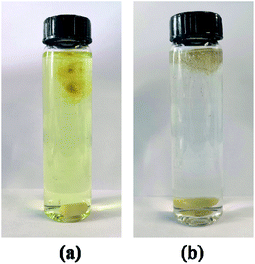 |
| Fig. 9 The picture of reaction system before and after reaction. (a) Before reaction, (b) after reaction. | |
Conflicts of interest
There are no conflicts to declare.
Acknowledgements
This work was financially supported by the National Science Foundation of Hebei province, China (no. H2020209288).
References
- R. Gao, L. Pan, H. Wang, X. Zhang, L. Wang and J. Zou, Ultradispersed Nickel Phosphide on Phosphorus-Doped Carbon with Tailored d-Band Center for Efficient and Chemoselective Hydrogenation of Nitroarenes, ACS Catal., 2018, 8, 8420–8429 CrossRef CAS.
- R. Gao, L. Pan, Z. Li, X. Zhang, L. Wang and J. Zou, Cobalt nanoparticles encapsulated in nitrogen-doped carbon for room-temperature selective hydrogenation of nitroarenes, Chin. J. Catal., 2018, 39, 664–672 CrossRef CAS.
- M. Yaseen, B. Manu, N. Kudri and H. S. Govardhanaswamy, Use of redox mediators for the enhanced degradation of selected nitrophenols, Appl. Water Sci., 2019, 9, 194 CrossRef CAS.
- R. Fatima, M. N. Afridi, V. Kumar, J. Lee, I. Ali, K. Kim and J. Kim, Photocatalytic degradation performance of various types of modified TiO2 against nitrophenols in aqueous systems, J. Cleaner Prod., 2019, 231, 899–912 CrossRef CAS.
- X. Liu, L. Zhao, H. Lai, S. Li and Z. Yi, Efficient photocatalytic degradation of 4-nitrophenol over graphene modified TiO2, J. Chem. Technol. Biotechnol., 2017, 92, 2417–2424 CrossRef CAS.
- S. Varshney, R. Bar Ziv and T. Zidki, On the Remarkable Performance of Silver-based Alloy Nanoparticles in 4-Nitrophenol Catalytic Reduction, ChemCatChem, 2020, 12, 4680–4688 CrossRef CAS.
- S. R. Thawarkar, B. Thombare, B. S. Munde and N. D. Khupse, Kinetic investigation for the catalytic reduction of nitrophenol using ionic liquid stabilized gold nanoparticles, RSC Adv., 2018, 8, 38384–38390 RSC.
- S. Gopi, A. G. Ramu, S. Sakthivel, G. Maia, C. Jang, D. Choi and K. Yun, Cobalt-modified 2D porous organic polymer for highly efficient electrocatalytic removal of toxic urea and nitrophenol, Chemosphere, 2021, 265, 129052 CrossRef CAS PubMed.
- N. Hu, J. Y. Yin and Q. Tang, Comparative study of amphiphilic hyperbranched and linear polymer stabilized organo-soluble gold nanoparticles as efficient recyclable catalysts in the biphasic reduction of 4-nitrophenol, J. Polym. Sci., Part A: Polym. Chem., 2011, 49, 3826–3834 CrossRef CAS.
- Y. Liu, Y. Fan and Y. Yuan, Amphiphilic hyperbranched copolymers bearing a hyperbranched core and a dendritic shell as novel stabilizers rendering gold nanoparticles with an unprecedentedly long lifetime in the catalytic reduction of 4-nitrophenol, J. Mater. Chem., 2012, 22, 21173–21182 RSC.
- N. Hu, J. Y. Yin and Q. Tang, Comparative study of amphiphilic hyperbranched and linear polymer stabilized organo-soluble gold nanoparticles as efficient recyclable catalysts in the biphasic reduction of 4-nitrophenol, J. Polym. Sci., Part A: Polym. Chem., 2011, 49, 3826–3834 CrossRef CAS.
- Y. Y. Chu, Y. Qian, W. J. Wang and X. L. Deng, A dual-cathode electro-Fenton oxidation coupled with anodic oxidation system used for 4-nitrophenol degradation, J. Hazard. Mater., 2012, 199–200, 179–185 CrossRef CAS PubMed.
- K. Chakraborty, S. Chakrabarty, T. Pal and S. Ghosh, Synergistic effect of zinc selenide-reduced graphene oxide towards enhanced solar light-responsive photocurrent generation and photocatalytic 4-nitrophenol degradation, New J. Chem., 2017, 41, 4662–4671 RSC.
- Q. Yang, E. Guo, H. Liu and Q. Lu, Engineering of Z-scheme 2D/3D architectures with Bi2MoO6 on TiO2 nanosphere for enhanced photocatalytic 4-nitrophenol degradation, J. Taiwan Inst. Chem. Eng., 2019, 105, 65–74 CrossRef CAS.
- N. L. McCombs, J. D Antonio, D. A. Barrios, L. M. Carey and R. A. Ghiladi, Nonmicrobial Nitrophenol Degradation via Peroxygenase Activity of Dehaloperoxidase-Hemoglobin fromAmphitrite ornata, Biochemistry, 2016, 55, 2465–2478 CrossRef CAS PubMed.
- J. Rong, H. Chen, F. Qiu, T. Zhang, Y. Zhu, D. Yang, J. Xu, X. Rong, Q. Guo and X. Peng, In-situ immobilization and pyrolysis of metal-organic framework supported on biomorphic layered double hydroxides as highly active and stable heterogeneous catalyst, J. Taiwan Inst. Chem. Eng., 2018, 88, 78–88 CrossRef CAS.
- S. Lu, J. Yu, Y. Cheng, Q. Wang, A. Barras, W. Xu, S. Szunerits, D. Cornu and R. Boukherroub, Preparation of silver nanoparticles/polydopamine functionalized polyacrylonitrile fiber paper and its catalytic activity for the reduction 4-nitrophenol, Appl. Surf. Sci., 2017, 411, 163–169 CrossRef CAS.
- J. Wang, Y. Li, H. Li, Z. Cui, Y. Hou, W. Shi, K. Jiang, L. Qu and Y. Zhang, A novel synthesis of oleophylic Fe2O3/polystyrene fibers by γ-Ray irradiation for the enhanced photocatalysis of 4-chlorophenol and 4-nitrophenol degradation, J. Hazard. Mater., 2019, 379, 120806 CrossRef CAS PubMed.
- G. Qiao, Q. Xu, A. Wang, D. Zhou and J. Z. Yin, Efficient synthesis of sub-5 nm Ag nanoparticles by the desorption effect of supercritical CO2 in SBA-15, Nanotechnology, 2020, 31, 375603 CrossRef CAS PubMed.
- Z. Wang, F. Li, L. Zhang, J. Z. Qian and S. K. Cao, Phase-transfer-assisted synthesis of cysteine-Ag nanoparticles/graphene oxide nanocomposite and its enhanced performance in antibiosis and biosensing, Nanotechnology, 2020, 31, 455603 CrossRef CAS PubMed.
- S. Chen, G. Wang, W. Sui, A. M. Parvezc and C. L. Si, Synthesis of lignin-functionalized phenolic nanosphere supported Ag nanoparticles with excellent dispersion stability and catalytic performance, Green Chem., 2020, 22, 2879–2888 RSC.
- C. Xie, J. Song, H. Wu, Y. Hu, H. Z. Liu, Y. D. Yang, Z. R. Zhang, B. F. Chen and B. X. Han, Naturally occurring gallic acid derived multifunctional porous polymers for highly efficient CO2 conversion and I2 capture, Green Chem., 2018, 20, 4655–4661 RSC.
- R. V. Sales, H. O. M. A. Moura, A. B. F. Câmara, E. Rodríguez-Castellón, J. A. B. Silva, S. B. C. Pergher, L. M. A. Campos, M. M. Urbina, T. C. Bicudo and L. S. de Carvalho, Assessment of Ag Nanoparticles Interaction over Low-Cost Mesoporous Silica in Deep Desulfurization of Diesel, Catalysts, 2019, 9, 651 CrossRef CAS.
- Z. Chen and R. L. Luck, Oxidation of olefins using atmospheric oxygen atoms initiated by tert-butylhydroperoxide or hydrogen peroxide with silver nanoparticles deposited on MCM-41 as catalysts, Green Chem., 2016, 18, 3354–3359 RSC.
- X. Zhang, B. Ren, X. Li, Y. Xu, B. Liu, P. Yu, Y. Sun and D. Mei, Efficiently enhanced visible-light photocatalytic activity by in situ deposition of Ag@AgBr on g-C3N4/Fe3O4 magnetic heterogeneous materials, Sep. Purif. Technol., 2021, 254, 117596 CrossRef CAS.
- S. Chang, C. Liu, Y. Sun, Z. Yan, X. Zhang, X. Hu and H. Zhang, Fe3O4 Nanoparticles Coated with Ag-Nanoparticle-Embedded Metal-Organic Framework MIL-100(Fe) for the Catalytic Reduction of 4-Nitrophenol, ACS Appl. Nano Mater., 2020, 3, 2302–2309 CrossRef CAS.
- J. Huang, X. Han, X. Zhao and C. Meng, Facile preparation of core-shell Ag@SiO2 nanoparticles and their application in spectrally splitting PV/T systems, Energy, 2021, 215, 119111 CrossRef CAS.
- H. Li, W. Xu, L. Qiu, Y. Wang, L. Xiao, F. Ouyang, L. Lin and X. Chen, Effect of Silver Doping on F-TiO2/SiO2 Nano-Powder Catalysts for Photocatalytic Degradation of Acrylonitrile Wastewater, J. Nanoelectron. Optoelectron., 2019, 14, 1043–1047 CrossRef CAS.
- Y. Yang, X. Wu, C. He, J. Huang, S. Yin, M. Zhou, L. Ma, W. Zhao, L. Qiu, C. Cheng and C. Zhao, Metal-Organic Framework/Ag-Based Hybrid Nanoagents for Rapid and Synergistic Bacterial Eradication, ACS Appl. Mater. Interfaces, 2020, 12, 13698–13708 CrossRef CAS PubMed.
- J. Shi, L. Zhang, N. Sun, D. Hu, Q. Shen, F. Mao, Q. Gao and W. Wei, Facile and Rapid Preparation of Ag@ZIF-8 for Carboxylation of Terminal Alkynes with CO2 in Mild Conditions, ACS Appl. Mater. Interfaces, 2019, 11, 28858–28867 CrossRef CAS PubMed.
- F. Xu, Q. Zhang and Z. Gao, Simple one-step synthesis of gold nanoparticles with controlled size using cationic Gemini surfactants as ligands: Effect of the variations in concentrations and tail lengths, Colloids Surf., A, 2013, 417, 201–210 CrossRef CAS.
- Y. Xu, Y. Zhao, L. Chen, X. Wang, J. Sun, H. Wu, F. Bao, J. Fan and Q. Zhang, Large-scale, low-cost synthesis of monodispersed gold nanorods using a gemini surfactant, Nanoscale, 2015, 7, 6790–6797 RSC.
- D. Li, W. Fang, Y. Feng, Q. Geng and M. Song, Stability properties of water-based gold and silver nanofluids stabilized by cationic gemini surfactants, J. Taiwan Inst. Chem. Eng., 2019, 97, 458–465 CrossRef CAS.
- D. Astruc, F. Lu and J. R. Aranzaes, Nanoparticles as Recyclable Catalysts: The Frontier between Homogeneous and Heterogeneous Catalysis, Angew. Chem., Int. Ed., 2005, 44, 7852–7872 CrossRef CAS PubMed.
- T. Jain, A. R. Tehrani-Bagha, H. Shekhar, R. Crawford, E. Johnson, K. Nørgaard, K. Holmberg, P. Erhart and K. Moth-Poulsen, Anisotropic growth of gold nanoparticles using cationic gemini surfactants: effects of structure variations in head and tail groups, J. Mater. Chem. C, 2014, 2, 994–1003 RSC.
- S. He, H. Chen, Z. Guo, B. Wang, C. Tang and Y. Feng, High-concentration silver colloid stabilized by a cationic gemini surfactant, Colloids Surf., A, 2013, 429, 98–105 CrossRef CAS.
- J. Xu, X. Han, H. Liu and Y. Hu, Synthesis and optical properties of silver nanoparticles stabilized by gemini surfactant, Colloids Surf., A, 2006, 273, 179–183 CrossRef CAS.
- S. Bhattacharya and J. Biswas, Role of spacer lengths of gemini surfactants in the synthesis of silver nanorods in micellar media, Nanoscale, 2011, 3, 2924 RSC.
- S. Datta, J. Biswas and S. Bhattacharya, How does spacer length of imidazolium gemini surfactants control the fabrication of 2D-Langmuir films of silver-nanoparticles at the air-water interface?, J. Colloid Interface Sci., 2014, 430, 85–92 CrossRef CAS PubMed.
- D. Li, W. Fang, Y. Zhang, X. Wang, M. Guo and X. Qin, Stability and Thermal Conductivity Enhancement of Silver Nanofluids with Gemini Surfactants, Ind. Eng. Chem. Res., 2017, 56, 12369–12375 CrossRef CAS.
- G. Xu, J. Cao, Y. Zhao, L. S. Zheng, M. L. Tao and W. Q. Zhang, Phosphorylated Polyacrylonitrile Fiber as an Efficient and Greener Acetalization Catalyst, Chem.–Asian J., 2017, 12, 2565–2575 CrossRef CAS PubMed.
Footnote |
† Electronic supplementary information (ESI) available. See DOI: 10.1039/d1ra07321d |
|
This journal is © The Royal Society of Chemistry 2022 |