DOI:
10.1039/D2QO01399A
(Research Article)
Org. Chem. Front., 2022,
9, 6295-6304
Synthesis, structure–property relationships and absorbance modulation of highly asymmetric photochromes with variable oxidation and substitution patterns†
Received
5th September 2022
, Accepted 3rd October 2022
First published on 4th October 2022
Abstract
Asymmetric diarylethenes with benzo[b]thiophen-3-yl and 2-thienyl residues having variable oxidation degrees (S and/or SO2) remained unexplored. These photochromes provide reversibly photoswitchable absorbance and multicolor emission modulation. Here we report 18 photochromic 1,2-diarylperfluorocyclopentenes with oxidized and non-oxidized 2-methylbenzo[b]thiophen-3-yl, as well as 5-aryl-3-methylthiophen-2-yl groups. The structure–property relationships were studied for three groups of compounds: non-oxidized, mono-oxidized (to SO2 in the benzothiophene part), and fully-oxidized (to 2 × SO2). The quantum chemistry calculations helped to interpret the substituents’ effects in each group and predict the photophysical properties of yet unavailable photochromes. The photochromic systems with absorbance modulation introduced in this work were designed for the use in diffraction-unlimited writing and reading with light, nanopattering and optical lithography.
Introduction
Photochromism is the ability of a compound to reversibly switch between two molecular states upon irradiation with light.1 In most cases, the two states represent isomeric structures, and new chemical bonds are formed and broken intramolecularly in the course of a photochromic reaction. Illumination with light of two distinct wavelengths is required for interconversion (Scheme 1), and the colour change arises from differences in the absorption spectra of the two states (isomers).2 Diarylethenes3–5 having benzo[b]thiophen-3-yl or 3-thienyl residues attached to the double bond of perfluorocyclopentene,6 are promising candidates for practical applications. These photochromes feature high photo-reaction efficiency,7 strong fatigue resistance (large number of switching cycles),6,8,9 thermal stability of the states,10 and well separated absorption spectra of the isomers. Due to their unique properties, diarylethenes became attractive molecular tools in various research fields, from material to life science.11–18 In particular, some diarylethenes present fluorescence emission only in one isomer,19–26 enabling the most sensitive readout method.
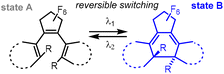 |
| Scheme 1 Under irradiation with light, photochromic 1,2-diarylperfluorocyclopentenes undergo reversible transitions between two stable states. | |
The photo-physical properties of photochromic compounds are strongly influenced by the core structure. So far, a variety of structural modifications have been reported, such as introduction of aryl or other (functional) groups to C-6 of benzo[b]thiophene27,28 or C-5 of thiophene,29,30 the replacement of these heteroarenes with other heterocycles (e.g., nitrogen-31,32 or oxygen-containing ones33,34), variation of the alkyl group(s) at the “central” reactive carbon atoms (C-2 and C-2′),35 and the replacement of perfluorocyclopentene by non-fluorinated 5- or 6-membered rings with double bonds.6,36,37 The syntheses of diarylethenes and their applications in material and life sciences have been studied and reviewed over the last decades.38–40
Oxidation of a thiophene/benzo[b]thiophene residue to 1,1-dioxide,41–43 or change from a “normal type” 3-(benzo)thienyl to an “inverse-type” 2-(benzo)thenyl substitution pattern44–46 are known to drastically alter the conjugation path, optical spectra and the switching parameters of diarylethenes. For example, 1,2-bis(2-alkyl-1-benzothiophene-1,1-dioxide-3-yl)perfluorocyclopentenes47,48 exhibit high fluorescent quantum yields (up to 0.9) in their closed-form (CF).43 This property makes them useful in many photonic applications: optical memories,49,50 bioimaging,51 and super-resolution microscopy.52,53 On the other hand, the 2-thienyl substituted “inverse type” diarylethenes undergo ring-closure reactions under irradiation in the short wavelength region, and some of them are known to be fluorescent in their open forms (OFs).54 While these chemical modifications are key to customize the photophysical properties, the studies of sulfone (SO2) and “inverse type” diarylethenes were focused on highly symmetric structures.
Recently, asymmetric “sulfide-sulfone”55 and “half-inversed sulfone” diarylethenes56,57 with unique photo-physical properties were reported (Fig. 1, upper part). The former showed ratiometric fluorescence switching (both isomers were fluorescent) and endured more than 2 × 104 switching cycles.55 The latter switched the emission of an adjacent perylene dye on and off via photo-induced electron transfer (PET).56,57 However, in the previous reports, asymmetric oxidation and “inversed” linkage of the thiophene or benzo[b]thiophene units (via C-2) were assessed separately, and not combined in one structure. In this work, we used an asymmetric diarylethene, which was applied as a successful modulator in a PET system,56 as a lead (Fig. 1, DAE-PDI). We varied the substituents (electron donors and acceptors) in both parts (left and right) of the molecule and changed the oxidation state of S-atoms to produce highly asymmetric structures.
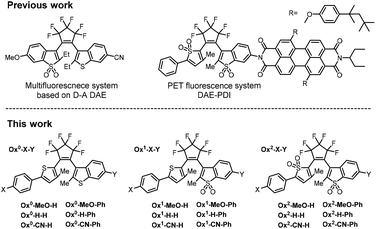 |
| Fig. 1 Upper part: previously reported asymmetric diarylethenes with a sulfone fragment. Lower part: asymmetric structures Ox0, Ox1 and Ox2 of the present work; X and Y denote functional groups. | |
In general, the absorption and emission properties of diarylethenes depend on (1) oxidation state of benzo[b]thiophene or thiophene core, (2) the presence of donor and/or acceptor groups in the “active” positions of the conjugated system, and (3) the presence and nature of an aryl group at C-6 of benzo[b]thiophene. To get insights into the trends of structure–spectra relationships, we designed and prepared 18 photochromic 1,2-diarylperfluorocyclopentenes with 2-methyl-benzo[b]thiophen-3-yl and 5-aryl-3-methylthiophen-2-yl parts (Fig. 1, lower part). We categorized them into three groups: non-oxidized (Ox0-X-Y), mono-sulfones (Ox1-X-Y with oxidized benzo[b]thiohene units), and bis-sulfones (Ox2-X-Y). In each group, the substituent X at the para-position of the phenyl group is either hydrogen, or electron-acceptor (CN), or electron-donor (MeO). In the right part of the molecule, the benzo[b]thiophene unit is either non-oxidized or oxidized, and the substituent Y = H or phenyl (Ph).
Results and discussion
Synthesis
The synthesis routes to the designed 18 compounds and intermediates A(Y), C(Y) with variation points Y and Br, respectively, are shown in Scheme 2. The starting materials, A(H) and A(Ph) were prepared in 3–4 steps from commercially available materials (see ESI†). The residue Y may be hydrogen or any (het)aryl group compatible with the reagents and conditions in Scheme 2. The freshly prepared A(H) and A(Ph) were reacted with 2-lithio-3-methyl-5-(trimethylsilyl)thiophen to give intermediates B(H) and B(Ph), respectively. Treating with bromine in DCM transformed the trimethylsilyl (TMS) group in B(Y) to bromides C(Y). The six non-oxidized diarylethenes Ox0-X-Y (see Fig. 1 and Scheme 2) were obtained by a Suzuki–Miyaura reaction of bromides C(H) and C(Ph) with boronic acids (or esters of boronic acids; see ESI†): p-methoxyphenyl, phenyl, and p-cyanophenyl. The oxidation of sulfur atoms in both thiophene and benzothiophene fragments to sulfone residues was carried out by treating with excess of m-chloroperoxybenzoic acid (mCPBA), yielding fully oxidized compounds (Ox2-X-Y). On the other hand, oxidization of compounds C(Y) with aqueous H2O2 in acetic acid (30 min under reflux) gave sulfones D(Y), in which only benzothiophene units were selectively oxidized. The position of the oxidation site was determined by the comparison of the 1H NMR spectra (characteristic proton shift in the aromatic region), as shown in Fig. S3a and S5a.† Finally, a Suzuki–Miyaura coupling reaction of D(Y) with corresponding boronic acids gave asymmetrically oxidized diarylethene series (Ox1-X-Y). The detailed synthetic procedures and NMR spectra (Fig. S1–S23†) of all new compounds are given in ESI.†
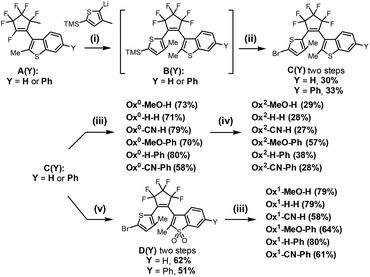 |
| Scheme 2 Synthesis of the diarylethenes: (i) THF, −70 °C; (ii) DCM, Br2, 0 °C to r.t.; (iii) boronic esters, Pd(PPh3)4, THF/Na2CO3 aq. reflux, 1.5–3.0 h; (iv) mCPBA, DCM, r.t., 1–3d; (v) H2O2, AcOH, reflux, 30 min. | |
Photophysical properties of Ox0-X-Y and Ox1-X-Y series
The photoisomerization experiments, measurements of the spectra and photophysical parameters were performed in the home-built optical setup described previously.58 The photo-cyclization (ΦO→C) and photo-cycloreversion (ΦC→O) quantum yields of both series Ox0-X-Y and Ox1-X-Y were determined by using 365 nm and 470 nm light, respectively. The relative fluorescence quantum yields (ΦF) were determined with quinine sulfate (ΦF = 0.55 in 0.5 M aq. H2SO4), as the reference material. The main photophysical properties of 12 non-oxidized and mono-oxidized diarylethenes are given in Table 1.
Table 1 Photophysical properties of the series Ox0-X-Y and Ox1-X-Y measured in 1,4-dioxane
Compounds |
Φ
O→C
|
Φ
C→O
|
α
365 nm)
|
Abs
λ
max/nm (εOF × 10−3) d |
Abs
λ
max/nm (εCF × 10−3) e |
Fl
λ
max/nm (ΦF)f |
Photo-cyclization quantum yields measured at 365 nm.
Photo-cycloreversion quantum yields measured at 470 nm.
Photo-conversion degree (αλ = [CF]/([CF] + [OF])) at the photostationary state by using 365 nm light.
Absorption maxima of OFs (molar absorptivity; M−1 cm−1).
Absorption maxima of CFs; nm (molar absorptivity; M−1 cm−1).
Emission maxima; nm (relative fluorescence quantum yields) of OFs; standard: quinine sulfate in 0.5M H2SO4 (ΦF = 0.55).59
|
Ox0-H-H/Ox0-H-Ph
|
0.18/0.22 |
0.35/0.33 |
0.61/0.6 |
349(15.6)/347(13.5) |
480(8.4)/489(10.5) |
442 (0.02)/449(0.01) |
Ox1-H-H/Ox1-H-Ph
|
0.18/0.14 |
0.31/0.29 |
0.77/0.5 |
363(14.4)/350(16.6) |
450(11.2)/463(15.3) |
490(0.04)/488(0.05) |
Ox0-MeO-H/Ox0-MeO-Ph
|
0.17/0.17 |
0.33/0.36 |
0.75/0.53 |
366(17.4)/366(17.2) |
480(8.0)/490(11.3) |
472(0.04)/472(0.03) |
Ox1-MeO-H/Ox1-MeO-Ph
|
0.12/0.13 |
0.26/0.36 |
0.39/0.30 |
384(15.4)/379(13.2) |
452(10.9)/462(12.9) |
543(0.09)/534(0.07) |
Ox0-CN-H/Ox0-CN-Ph
|
0.15/0.19 |
0.34/0.41 |
0.58/0.54 |
346(20.8)/343(20.6) |
478(8.7)/489(12.1) |
450(0.02)/467(0.01) |
Ox1-CN-H/Ox1-CN-Ph
|
0.20/0.18 |
0.31/0.30 |
0.82/0.68 |
357(18.8)/350(23.1) |
446(11.8)/460(16.7) |
467(0.04)/469(0.05) |
In the present study, the photochromic reactions of all asymmetric diarylethenes occurred by irradiation below 560 nm; the “normal” diarylethenes undergo ring-opening reactions at longer wavelengths. For the non-oxidized Ox0-X-Y and mono-sulfone Ox1-X-Y series, the OFs showed intense absorption bands in the UV region (320 nm to 420 nm), while the CFs’ absorption bands appear between 400 nm and 560 nm. Both the cyclization and cycloreversion quantum yields ΦO→C and ΦC→O are high (0.12–0.35). Interestingly, compounds from the Ox0-X-Y and Ox1-X-Y series emit only in their OFs, so that their photochromic reactions are accompanied by the reversible “turn-off” type of the fluorescence switch. In other words, upon irradiation with UV light (365 nm), their emission vanishes along with the formation of the CFs. Thus, conversions in the photostationary state (PSS) were calculated from the fluorescence modulation (αPSS (365 nm) = IFPSS/IF0, where IF is the fluorescence intensity at the PSS or before the irradiation, where the entire compound is in the OF). Then, the absorption spectrum of the CF (AbsCF (λ)) was calculated from the absorption spectra measured of the OF (AbsOF (λ)) and at the PSS (AbsPSS (λ)), according to the following expression AbsCF (λ) = (AbsPSS (λ) − (1 − αPSS) × AbsOF (λ))/αPSS. The irradiation of CFs with visible light triggers the ring-opening reactions, with full recovery of the initial fluorescence intensity.
In order to reveal the influence of the substituent X (X = CN, H, or MeO), the absorption spectra in the series Ox0-X-Y were compared, while Y was fixed as H (Fig. 2a–c). The group X influences the position of the absorption maximum (Absλmax) in the OFs, but not in the CFs. For example, compounds Ox0-CN-H, Ox0-H-H, Ox0-MeO-H in their OFs (in 1.4-dioxane) exhibited clear bathochromic shift of the Absλmax values from 346 nm to 349 nm and 366 nm, respectively; while the Absλmax value of the CFs remained almost unchanged (479 nm ± 1 nm, Table 1). The similar trend is observed in the series Ox0-X-Ph and Ox1-X-Y (Y = H, Ph). For detailed comparison, the absorption spectra of compounds Ox0-X-Ph are shown in Fig. 2d–f; see also Table 1. The influence of the substituents X and Y on the Absλmax values were further assessed by using quantum chemical calculations and compared with the features observed for the double oxidized analogs Ox2-X-Y in the following sections. In what concerns emission intensity, all ΦF values of Ox1-X-Y in their OFs (measured in 1.4-dioxane) are approximately 2–4 times higher than these of Ox0-X-Y (Table 1). The nature of the Y substituent attached to benzothiophene (or benzothiophene sulfone) has almost no influence on fluorescence, but the residue X influences the ΦF values and the position of emission maxima (Flλmax; see Table 1).
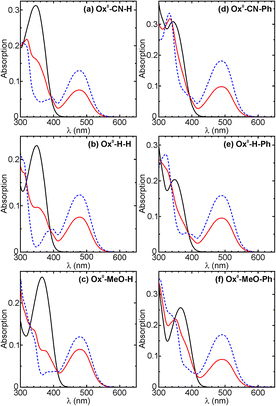 |
| Fig. 2 Absorption spectra of compounds (a) Ox0-CN-H, (b) Ox0-H-H, (c) Ox0-MeO-H, (d) Ox0-CN-Ph, (e) Ox0-H-Ph, and (f) Ox0-MeO-Ph measured in 1,4-dioxane. Black, red and blue (dashed) lines represent the OF, the PSS by 365 nm excitation, and the calculated (from emission modulation) CF spectra. | |
To explore the substituent effects in the Ox1-X-Y series, we considered three compounds Ox1-X-H (X = CN, H, and MeO). Their photo-switching and emission in solvents of various polarity (cyclohexane, ethyl acetate, and acetonitrile) were studied. The fluorescence spectra and the photophysical data are given in Fig. 3a–c and Table 2, respectively. We found that both Ox1-CN-H and Ox1-H-H switch reversibly between fluorescent and non-fluorescent states, regardless of the solvent polarity (Fig. 3a and b). These two compounds showed similar emission efficiencies and photoreaction quantum yields (ΦO→C and ΦC→O) in all solvents we used. On the other hand, the fluorescent properties of Ox1-MeO-H strongly depends on the solvent polarity. Compared to Ox1-CN-H and Ox1-H-H, the ΦF value and the photoswitching quantum yields of Ox1-MeO-H were found to be 5 times lower in acetonitrile (Table 2). It was also found that the emission intensity of Ox1-MeO-H depends on the solvent polarity much stronger than these of Ox1-CN-H and Ox1-H-H (Fig. 3c). The solvatochromism of Ox1-MeO-H is a result of the strong intramolecular charge-transfer (CT) in the excited state of the OF, induced by the conjugation between the methoxy (donor) and the benzothiophene sulfone (acceptor) groups. To quantitatively illustrate the solvatochromic fluorescence of compounds Ox1-X-H, their emission maxima are plotted in the CIE 1931 color space diagram as shown in the Fig. 3d.
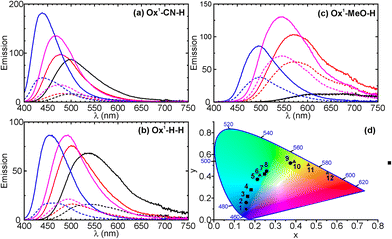 |
| Fig. 3 Emission spectra of (a) Ox1-CN-H, (b) Ox1-H-H and (c) Ox1-MeO-H in the OFs (solid lines) and in the photostationary state (α365 nm; dashed lines), in cyclohexane (blue lines), dioxane (purple lines), ethyl acetate (red lines) and acetonitrile (black lines). (d) CIE 1931 color space with emission colors of three compounds, in squares for Ox1-CN-H (i.e., 1, 3, 4, and 7 represent color of Ox1-CN-H in cyclohexane (cHex), dioxane (Diox), ethyl acetate (EtAc), and acetonitrile (MeCN), respectively), circles for Ox1-H-H (2, 5, 8, and 9 represent colors in cHex, Diox, EtAc, and MeCN, respectively) and triangles for Ox1-MeO-H (6, 10, 11, and 12 represent colors in cHex, Diox, EtAc, and MeCN, respectively). | |
Table 2 Photo-switching and fluorescent properties of Ox1-X-Y in various solvents
|
Solvent a |
Φ
O→C
(
Φ
C→O
)
|
α
(365 nm)
|
Fl
λ
max/nm(EffF) d |
cHex, Diox, EtAc, and MeCN are cyclohexane, 1,4-dioxane, ethyl acetate, and acetonitrile respectively.
Photo-cyclization and photo-cycloreversion quantum yields measured by using 365 nm and 470 nm light sources, respectively.
The photo-conversion degrees at the photo-stationary state (PSS) by using 365 nm light were calculated from the degrees of the fluorescence quench of OFs.
The emission maxima (relative emission efficiencies referenced to the value measured in 1,4-dioxane; the absolute values are in Table 1).
|
Ox1-CN-H
|
cHex |
0.13 (0.27) |
0.73 |
438 (1.15) |
Diox |
0.20 (0.31) |
0.82 |
467 (1.00) |
EtAc |
0.12 (0.23) |
0.82 |
476 (0.78) |
MeCN |
0.12(0.20) |
0.81 |
500 (0.81) |
Ox1-H-H
|
cHex |
0.14 (0.28) |
0.80 |
454 (0.84) |
Diox |
0.18 (0.31) |
0.77 |
490 (1.00) |
EtAc |
0.12 (0.23) |
0.78 |
502 (0.96) |
MeCN |
0.11 (0.22) |
0.76 |
537 (1.13) |
Ox1-MeO-H
|
cHex |
0.14 (0.25) |
0.56 |
495 (0.49) |
Diox |
0.12 (0.26) |
0.39 |
543 (1.00) |
EtAc |
0.08 (0.20) |
0.40 |
572 (0.88) |
MeCN |
0.02 (0.24) |
0.09 |
616 (0.13) |
Photophysical properties of Ox2-X-Y
Unlike compounds in the series Ox0-X-Y and Ox1-X-Y, the fully oxidized Ox2-X-Y analogs were non-fluorescent both in their OFs and CFs and exhibited either low photoreaction quantum yields (ΦO→C and ΦC→O) or irreversible photoreactions. We found that only Ox2-MeO-H and Ox2-MeO-Ph can undergo reversible photochromic transformations. The unique feature is that these two compounds exhibited “inversed” photochromism: the absorption bands of the OFs were found at longer wavelengths than these of the CFs. The photochromism of Ox2-MeO-H, Ox2-MeO-Ph and their photophysical parameters are given in Fig. 4 and Table 3, respectively. The absorption bands of the open and closed forms overlapped, and in order to achieve the highest possible photo-conversion degree, the irradiation wavelengths had to be carefully selected. We found that 455 nm and 365 nm light sources are optimal for photo-cyclization and cycloreversion reactions, respectively. In the case of Ox2-MeO-H, irradiation with 455 nm light of the solution in 1,4-dioxane led to the decrease of the absorption band of the OF (Absλmax = 406 nm), while the new absorption band peaking at 355 nm emerged. Then the solution of Ox2-MeO-H was analyzed by means of HPLC and HRMS, and we confirmed that the new absorption band (Absλmax = 355 nm) is due to the formation of the CF of Ox2-MeO-H (Fig. 4a). Moreover, HPLC analysis revealed that the photo-conversion by 455 nm light is almost quantitative (α(455nm) > 0.98), due to the well-separated absorption bands between OF and CF of Ox2-MeO-H (i.e.CF has almost no absorption at 455 nm). As a control, when 405 nm light was employed, the conversion became incomplete (α405 nm = 0.74). Further irradiation with 365 nm light regenerated the OF (Fig. 4a), and the reverse photo-conversion degree (α′(365nm) = 1 − α(365nm) = [OF]/([CF] + [OF])) was determined to be 0.81 by HPLC analysis. The enhancement in the absorbance at 355 nm achieved between the two photo-stationary states is ×2.2. The photophysical properties of Ox2-MeO-Ph were also studied under the same irradiation condition. In comparison to Ox2-MeO-H, a lower photo-conversion degree to the CF was observed (α455 nm = 0.39). This is a result of a smaller shift in the absorption maxima between the OF and the CF, i.e. due to considerable absorption of the CF at 455 nm (Fig. 4b). The phenyl substituent (Y) attached to benzothiophene sulfone induced the bathochromic shift of the CF's absorption band, resulting in a larger overlap with the absorption band of the OF. From the measurements of the whole Ox2-X-Y series, we found that introduction of an electron donating (e.g., methoxy) group (as X) and the absence of aryl groups at C-6 of the oxidized benzothiophene part, are key factors in the design of the “inverse type” photochromic diarylethenes. The factors reduce the undesired overlap of the absorption bands of the OF and CF.
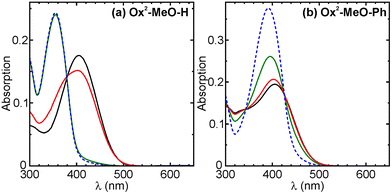 |
| Fig. 4 The absorption spectra of (a) Ox2-MeO-H and (b) Ox2-MeO-Ph, where black, red, green lines and blue-dashed line correspond to the OFs, PSS under irradiation with 365 nm light, PSS under irradiation with 455 nm light, and calculated CFs, respectively. | |
Table 3 The key photophysical data of Ox2-MeO-H and Ox2-MeO-Ph
|
Φ
O→C
|
Φ
C→O
|
α
(455 nm)
|
α′(365 nm)d |
Photo-cyclization quantum yields measured with 455 nm light.
Photo-cycloreversion quantum yields measured with 365 nm light.
The photo-conversion degrees at the photostationary states (PSS) by using 455 nm light (αλ = [CF]/([CF] + [OF])) was measured by HPLC.
The photo-conversion degrees at the photostationary states (PSS) by using 365 nm light (α′λ = [OF]/([CF] + [OF])) was measured by HPLC.
|
Ox2-MeO-H
|
9.7 × 10–3 |
1.3 × 10–2 |
0.98 |
0.81 |
Ox2-MeO-Ph
|
1.1 × 10–2 |
6.4 × 10–2 |
0.39 |
0.91 |
Quantum chemical calculations
To gain a better understanding of the structure–property relationships of the new asymmetric photochromes, we performed the density functional theory (DFT) calculations by using Gaussian 09 program package.60 The geometries of compounds were optimized using B3LYP exchange–correlation hybrid functional together with the 6-31++G(d,p) basis set in vacuum of both OFs (anti-parallel conformer) and CFs (anti-parallel conformer). We have calculated energies and obtained the isodensity surface plots of the frontier molecular orbitals (FMO). In the series of CFs of Ox0-X-Y, both HOMO and LUMO are localized on the whole molecule excluding substituent X and the neighbouring phenyl ring (Fig. 5a). This results in closely similar energy values of the HOMO–LUMO gaps for the whole series. In the case where Y = phenyl, the localization of FMO's is extended on this phenyl ring (attached to the benzothiophene), but does not involve the phenyl substituent attached to the thiophene ring (Fig. 5a). Usually, the lowest energy transition from the ground state is between HOMO and LUMO. This nicely explains, why substituent X does not affect the absorption maxima of the CF and why switching from Y = H to Y = Ph results in a bathochromic shift. In the OFs of Ox0-X-Y series, HOMO is localized on all molecular π-system, including the phenyl substituent; it also applies, if Y = Ph (Fig. 5b). Yet, the LUMO localization partially excludes the benzothiophene residue and the substituent Y, which explains the observation that the absorption maxima of OFs are mainly affected by substituent X but not Y. Similar regularities are also valid for the Ox1-X-Y series of compounds (Fig. S24†).
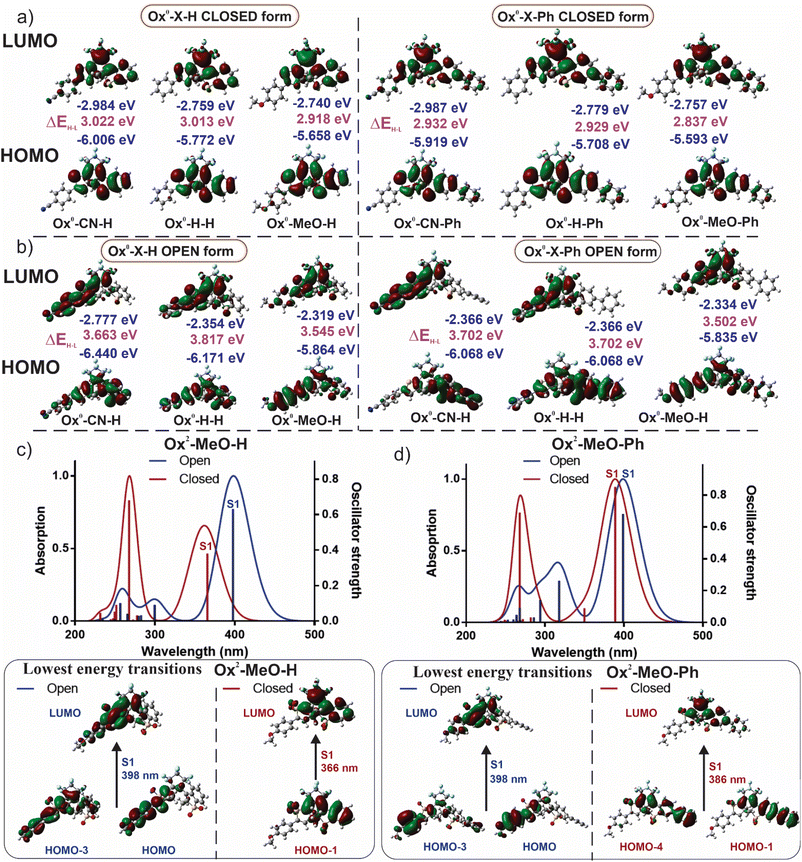 |
| Fig. 5 The DFT calculated ground state isodensity surface plots of the FMOs in (a) CFs and (b) OFs of OX0-X-Y series. TD-DFT simulated vertical excitation energies including 1,4-dioxane as solvent with IEFPCM model and fitted absorption spectra on excitation bands and representation of molecular orbitals responsible for the lowest energy transitions for (c) Ox2-MeO-H and (d) Ox2-MeO-Ph in OFs and CFs. | |
The localization of FMOs in the series Ox2-X-Y indicates a strong charge transfer character of the excited state and was not sufficient to explain the demonstrated “inversed photochromism” of Ox2-MeO-H(Ph) compounds (Fig. S24–S28†). In order to get better understanding of the nature of the absorption bands, we included 1,4-dioxane as solvent with IEFPCM solvation model and calculated the vertical excitation energies based on TD-DFT with CAM-B3LYP/6-31++G(d,p) hybrid exchange–correlation functional. The latter includes the long-range corrections and performs well with charge transfer transitions. The significant similarity between experimental and theoretical absorption spectra were observed (Fig. 5c and d). For the CF of Ox2-MeO-H, the lowest energy transition S0 → S1 is not a usual HOMO–LUMO transition (which becomes forbidden, due to a significantly small orbital overlap), but it is a HOMO−1 → LUMO transition instead. This results in a higher energy difference, and therefore, the absorption band of the CF is observed at the unusually short wavelengths (Fig. 5c). In the OF of Ox2-MeO-H, the S0 → S1 excitation arises from a mix of HOMO → LUMO and HOMO-3 → LUMO events. For the CF of Ox2-MeO-Ph, the S0 → S1 transition involves similar type of mixed orbitals (Fig. 5d). The lowest energy band of the CF of Ox2-MeO-Ph again excluded the HOMO orbital and relied on a mixed (HOMO−1 + HOMO-4) to LUMO transition. We hypothesize that the exclusion of HOMO in the series of Ox2-MeO-Y (OFs) is due to a spatially very small overlap of FMO's at the sulfone oxygen atoms (Fig. S24–S28†), which forbids a HOMO → LUMO transition and results in inversed absorption bands of CFs and OFs. The calculations reasonably predicted the positions and trends in the experimentally observed lowest energy absorption bands, including rapprochement of the CF and OF of Ox2-MeO-Ph; thus giving a better insight into the nature and control of “inverse photochromism” (Fig. 5 and S25–S28†). The experimental data and quantum chemical calculations introduced in this paper will serve for the design and optimization of “inversed” diarylethene photoswhitches with well-separated bands of the OF and CF.
Absorbance modulation
As easily processable substances, organic photoswitchable dyes are of high interest for new optical recording systems. In the far-field photolithography, the optical resolution is limited by diffraction to a half of the wavelength of the irradiation source.61 The reversible optical switching between two stable molecular states was recognized as a new physical concept enabling to “break the diffraction barrier” and applicable in lens-based optical imaging, as well as writing at the nanoscale.62 Improving the optical resolution in recording media through the use of photochromic layers was mainly developed by Rajesh Menon under the name “Absorbance Modulation Optical Lithography (AMOL)”.63 The photochromic dyes are appealing as main materials for writing at the nanoscale, because they feature transitions between two stable ground states (Scheme 1). Thus, light of low intensities may be applied.62 It preserves the photochromic layer from degradation, and the performance (contrast ratio and optical resolution) of the recording system is improved. Unfortunately, none of the photochromic diarylethenes reported so far possesses the absorption modulation properties required for the lens-based writing at the nanoscale: strong change of absorption at the certain wavelength, high degree of conversion in a photostationary state, photostability (high number of switching cycles), and the controllable switching kinetics. The symmetric diarylethene based on the two 5,5′-dimethyl-2,2′-bithiophene units attached via positions 4 to the double bond (C-1 and C-2) of the perfluorocyclopentane bridge has been reported as benchmark material for AMOL.63 It requires the use of 325 nm light for performing the ring-closure reaction. The ring-opening step (irradiation at 633 nm) is very slow, with quantum efficiency ca. 10−5–10−6, and this reduces the number of achievable switching cycles. Surprisingly, other diarylethenes have not yet been applied in AMOL.63,64 Therefore, one of our goals was to offer a general synthetic route to photochromic diarylethenes capable of efficient and rapid absorbance modulation with focusable light.
The aberration corrected lenses with large numerical apertures (1.35–1.45) are available only for wavelengths greater than 360 nm. In line with this, compact and affordable diode lasers emitting at 375 nm provide one of the shortest wavelengths available for optical switching and lens-based writing at the nanoscale. As an example, in Fig. 6 we present the absorption modulation of compound Ox0-MeO-H dissolved in 1,4-dioxane. Table 1 contains the data on photophysical properties. The absorption modulation at 375 nm is about 2.5 fold. In other organic solvents (ethyl acetate, acetonitrile and cyclohexane), similar properties were observed. These organic solvents are compatible with polymer matrixes in which the photochromic compounds are applied for nanolithography.
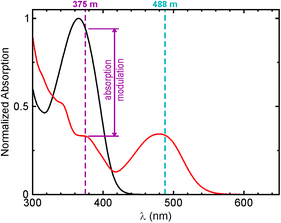 |
| Fig. 6 Absorption modulation of compound Ox0-MeO-H in 1,4-dioxane solution; for structure, see Fig. 1; for photophysical properties, see Table 1. | |
For compound in Fig. 6, the degree of conversion in the photostationary state (transition to the closed-ring isomer in Scheme 1) is relatively high (75% under irradiation with 365 nm light), but the cyclization is not complete. However, incomplete conversion does not preclude secure writing based just on discerning between exposed and non-exposed areas. In AMOL, a thin film of photochromic material is placed on top of a conventional photoresist and illuminated simultaneously by a focused light of wavelength λ1 and another irradiation pattern of wavelength λ2 featuring the local minimum or minima (e.g., a doughnut-shaped beam).62–65 The λ1 beam transforms the photochromic material to a more transparent (at λ1) state, and the underlying photoresist may be exposed. However, the λ2 irradiation reverses the transformation in all areas, except the local minimum (minima), so that the photoresist remains exposed only in these subdiffractionally “squeezed” areas. As a result, higher photolithographic resolution and information density may be achieved. This is expected to be the case for compound Ox0-MeO-H (Fig. 6), as well as diarylethenes Ox1-CN-H and Ox0-CN-Ph (Table 1), if we apply 375 nm and 488 nm lasers as λ1 and λ2 irradiation sources, respectively. The main advantages over the benchmark compound63 are that the use of 325 nm light is avoided, and the cycloreversion quantum yield increased over several orders of magnitude. We consider the structure–spectra relationships found in the series of asymmetric diarylethenes with variable oxidation patterns as guidelines for the design of absorbance modulators applicable for writing and reading on nanoscale with visible light.
Conclusions
In this work, the structure–properties relationships of highly asymmetric photochromic diaryethenes were studied. Eighteen new compounds were designed, and their syntheses performed. The three groups – non-oxidized Ox0-X-Y, mono-oxidized Ox1-X-Y, and fully-oxidized Ox2-X-Y − were compared, in which the substituents X = CN, H, or MeO and Y = H or Ph. It was found that the OFs of Ox0-X-Y and Ox1-X-Y are weakly fluorescent. Among them, compound Ox1-MeO-H with an electron donating methoxy group showed the highest value of ΦF and the strongest solvatochromism (in respect of emission color), due to the CT effect in the excited state. Interestingly, the fully oxidized diarylethene reported by Fukaminato et al.56 also showed reverse-type photochromism. However, this phenomenon is rare. In the present study, only Ox2-MeO-H and Ox2-MeO-Ph having methoxy groups underwent the reverse-type photochromic reaction. Our study revealed that the absorption bands of OF and CF of Ox2-MeO-H are fully separated, enabling the full conversion to CF by irradiation of 455 nm. We explained the observed substituents’ effect and the nature of inverse photochromism by using DFT calculations. We found that the large hypsochromic shifts observed in CFs of Ox2-MeO-H and Ox2-MeO-Ph are ascribed to the “forbidden” and hybrid HOMO–LUMO transitions.
We believe that the findings reported here will contribute to the development of photochromic compounds applicable as highly efficient molecular switches in material and life sciences. We plan to design and test the photoswitching performance of absorbance modulators in organic solvents and polymer matrices.
Conflicts of interest
There are no conflicts to declare.
Acknowledgements
We thank J. Bienert (MPI NAT), Dr H. Frauendorf, and Dr M. John and co-workers (Institut für Organische und Biomolekulare Chemie, Georg-August-Universitat, Göttingen, Germany) for recording NMR and high resolution mass spectra. Open Access funding provided by the Max Planck Society.
References
- H. Bouas-Laurent and H. Dürr, Organic photochromism (IUPAC Technical Report), Pure Appl. Chem., 2001, 73, 639–665 CrossRef CAS.
-
K. Nakatani, J. Piard, P. Yu and R. Métivier, Photochromic Materials, ed. H. Tian and J. Zhang, Wiley, Weinheim, 2016, pp. 1–45 Search PubMed.
-
M. Irie, Y. Yokoyama and T. Seki, New Frontiers in Photochromism, Springer, Tokyo, 2013, pp. 3–116 Search PubMed.
- H. Tian and S. Yang, Recent progresses on diarylethene based photochromic switches, Chem. Soc. Rev., 2004, 33, 85–97 RSC.
- M. Irie, T. Fukaminato, K. Matsuda and S. Kobatake, Photochromism of Diarylethene Molecules and Crystals: Memories, Switches, and Actuators, Chem. Rev., 2014, 114, 12174–12277 CrossRef CAS PubMed.
- M. Hanazawa, R. Sumiya, Y. Horikawa and M. Irie, Thermally irreversible photochromic systems. Reversible photocyclization of 1,2-bis (2-methylbenzo[b]thiophen-3-yl)perfluorocyclocoalkene derivatives, J. Chem. Soc., Chem. Commun., 1992, 206–207 RSC.
- S. Fukumoto, T. Nakashima and T. Kawai, Photon-Quantitative Reaction of a Dithiazolylarylene in Solution, Angew. Chem., Int. Ed., 2011, 50, 1565–1568 CrossRef CAS PubMed.
- Y.-C. Jeong, D. G. Park, E. Kim, K.-H. Ahn and S. I. Yang, Fatigue-resistant photochromic dithienylethenes by controlling the oxidation state, Chem. Commun., 2006, 1881–1883 RSC.
- M. Herder, B. M. Schmidt, L. Grubert, M. Pätzel, J. Schwarz and S. Hecht, Improving the Fatigue Resistance of Diarylethene Switches, J. Am. Chem. Soc., 2015, 137, 2738–2747 CrossRef CAS PubMed.
- M. Irie, T. Lifka, S. Kobatake and N. Kato, Photochromism of 1,2-Bis(2-methyl-5-phenyl-3-thienyl)perfluorocyclopentene in a Single-Crystalline Phase, J. Am. Chem. Soc., 2000, 122, 4871–4876 CrossRef CAS.
- K. Higashiguchi, G. Taira, J. Kitai, T. Hirose and K. Matsuda, Photoinduced Macroscopic Morphological Transformation of an Amphiphilic Diarylethene Assembly: Reversible Dynamic Motion, J. Am. Chem. Soc., 2015, 137, 2722–2729 CrossRef CAS PubMed.
- J. Okuda, Y. Tanaka, R. Kodama, K. Sumaru, K. Morishita, T. Kanamori, S. Yamazoe, K. Hyodo, S. Yamazaki, T. Miyatake, S. Yokojima, S. Nakamura and K. Uchida, Photoinduced cytotoxicity of a photochromic diarylethene via caspase cascade activation, Chem. Commun., 2015, 51, 10957–10960 RSC.
- H. Sato, T. Matsui, Z. Chen, J. Pirillo, Y. Hijikata and T. Aida, Photochemically Crushable and Regenerative Metal–Organic Framework, J. Am. Chem. Soc., 2020, 142, 14069–14073 CrossRef CAS PubMed.
- T. Fukushima, K. Tamaki, A. Isobe, T. Hirose, N. Shimizu, H. Takagi, R. Haruki, S. Adachi, M. J. Hollamby and S. Yagai, Diarylethene-Powered Light-Induced Folding of Supramolecular Polymers, J. Am. Chem. Soc., 2021, 143, 5845–5854 CrossRef CAS PubMed.
- F. Terao, M. Morimoto and M. Irie, Light-Driven Molecular-Crystal Actuators: Rapid and Reversible Bending of Rodlike Mixed Crystals of Diarylethene Derivatives, Angew. Chem., Int. Ed., 2012, 51, 901–904 CrossRef CAS PubMed.
- R. Nishimura, K. Hyodo, H. Sawaguchi, Y. Yamamoto, Y. Nonomura, H. Mayama, S. Yokojima, S. Nakamura and K. Uchida, Fractal Surfaces of Molecular Crystals Mimicking Lotus Leaf with Phototunable Double Roughness Structures, J. Am. Chem. Soc., 2016, 138, 10299–10303 CrossRef CAS PubMed.
- M. Berberich, A.-M. Krause, M. Orlandi, F. Scandola and F. Würthner, Toward Fluorescent Memories with Nondestructive Readout: Photoswitching of Fluorescence by Intramolecular Electron Transfer in a Diaryl Ethene-Perylene Bisimide Photochromic System, Angew. Chem., Int. Ed., 2008, 47, 6616–6619 CrossRef CAS PubMed.
- M. Berberich and F. Würthner, Terrylene bisimide-diarylethene photochromic switch, Chem. Sci., 2012, 3, 2771–2777 RSC.
- G. Naren, S. Li and J. Andréasson, A simplicity-guided cocktail approach toward multicolor fluorescent systems, Chem. Commun., 2020, 56, 3377–3380 RSC.
- M. Takeshita and M. Irie, Reversible Fluorescence Intensity Change of a Diarylethene, Chem. Lett., 1998, 1123 CrossRef CAS.
- K. Yagi and M. Irie, Fluorescence Property of Photochromic Diarylethenes with Indole Groups, Bull. Chem. Soc. Jpn., 2003, 76, 1625 CrossRef CAS.
- F. Hu, L. Jiang, M. Cao, Z. Xu, J. Huang, D. Wu, W. Yang, S. H. Liu and J. Yin, Cyanine-based dithienylethenes: synthesis, characterization, photochromism and biological imaging in living cells, RSC Adv., 2015, 5, 5982–5987 RSC.
- H. Liu and Y. Chen, The Photochromism and Fluorescence of Diarylethenes with a Imidazole Bridge Unit: A Strategy for the Design of Turn-on Fluorescent Diarylethene System, J. Phys. Chem. A, 2009, 113, 5550–5553 CrossRef CAS PubMed.
- S. C. Pang, H. Hyun, S. Lee, D. Jang, M. J. Lee, S. H. Kang and K. H. Ahn, Photoswitchable fluorescent diarylethene in a turn-on mode for live cell imaging, Chem. Commun., 2012, 48, 3745–3747 RSC.
- T. Nakagawa, Y. Miyasaka and Y. Yokoyama, Photochromism of a spiro-functionalized diarylethene derivative: multi-colour fluorescence modulation with a photon-quantitative photocyclization reactivity, Chem. Commun., 2018, 54, 3207–3210 RSC.
- Z. Xu, Q. T. Liu, X. Wang, Q. Liu, D. Hean, K. C. Chou and M. O. Wolf, Quinoline-containing diarylethenes: bridging between turn-on fluorescence, RGB switching and room temperature phosphorescence, Chem. Sci., 2020, 11, 2729–2734 RSC.
- K. Matsuda and M. Irie, Photochromism of Diarylethenes with Two Nitronyl Nitroxides: Photoswitching of an Intramolecular Magnetic Interaction, Chem. – Eur. J., 2001, 7, 3466–3473 CrossRef CAS.
- J. Lee, T. Kwon and E. Kim, Electropolymerization of an EDOT-modified diarylethene, Tetrahedron Lett., 2007, 48, 249 CrossRef CAS.
- M. Bossi, V. Belov, S. Polyakova and S. W. Hell, Reversible Red Fluorescent Molecular Switches, Angew. Chem., Int. Ed., 2006, 45, 7462–7465 CrossRef CAS PubMed.
- F. Hu, M. Cao, X. Ma, S. H. Liu and J. Yin, Visible-Light-Dependent Photocyclization: Design, Synthesis, and Properties of a Cyanine-Based Dithienylethene, J. Org. Chem., 2015, 80, 7830–7835 CrossRef CAS PubMed.
- H. B. Cheng, Y. D. Huang, L. Zhao, X. Li and H. C. Wu, A prominent bathochromic shift effect of indole-containing diarylethene derivatives, Org. Biomol. Chem., 2015, 13, 3470–3475 RSC.
- F. Ortica, P. Smimmo, U. Mazzucato, G. Favaro, A. Heynderickx, C. Moustrou and A. Samat, Photoinduced Processes in Dipyrrolyl-Perfluoro-Cyclopentenes, Photochem. Photobiol., 2006, 82, 1326–1333 CrossRef CAS PubMed.
- T. Yamaguchi, W. Taniguchi, T. Ozeki, S. Irie and M. Irie, Photochromism of diarylethene oxazole derivatives in a single-crystalline phase, J. Photochem. Photobiol., A, 2009, 207, 282 CrossRef CAS.
- S. Pu, H. Li, G. Liu, W. Liu, S. Cui and C. Fan, Synthesis and the effects of substitution upon photochromic diarylethenes bearing an isoxazole moiety, Tetrahedron, 2011, 67, 1438–1447 CrossRef CAS.
- T. Yamaguchi and M. Irie, Photochromism of bis(2-alkyl-1-benzothiophen-3-yl)perfluorocyclopentene derivatives, J. Photochem. Photobiol.,
A, 2006, 178, 162 CrossRef CAS.
- S. Chen, Y. Yang, Y. Wu, H. Tian and W. Zhu, Multi-addressable photochromic terarylene containing benzo[b]thiophene-1,1-dioxide unit as ethene bridge: multifunctional molecular logic gates on unimolecular platform, J. Mater. Chem., 2012, 22, 5486–5494 RSC.
- D. Kitagawa, T. Nakahama, Y. Nakai and S. Kobatake, 1,2-Diarylbenzene as fast T-type photochromic switch, J. Mater. Chem. C, 2019, 7, 2865–2870 RSC.
- J. Zhang and H. Tian, The Endeavor of Diarylethenes: New Structures, High Performance, and Bright Future, Adv. Opt. Mater., 2018, 6, 1701278 CrossRef.
-
K. Uno, V. N. Belov and M. L. Bossi, Photoswitchable Fluorophores for Super-Resolution Optical Microscopy, in Molecular Photoswitches, ed. Z. L. Pianowski, Wiley VCH, 2022, vol. 2, pp. 605–626 Search PubMed.
- D. Kim and S. Y. Park, Multicolor Fluorescence Photoswitching: Color-Correlated versus Color-Specific Switching, Adv. Opt. Mater., 2018, 6, 1800678 CrossRef.
- Y.-C. Jeong, S. I. Yang, K.-H. Ahn and E. Kim, Highly fluorescent photochromic diarylethene in the closed-ring form, Chem. Commun., 2005, 2503–2505 RSC.
- M. Taguchi, T. Nakagawa, T. Nakashima and T. Kawai, Photochromic and fluorescence switching properties of oxidized triangle terarylenes in solution and in amorphous solid states, J. Mater. Chem., 2011, 21, 17425–17432 RSC.
- K. Uno, H. Niikura, M. Morimoto, Y. Ishibashi, H. Miyasaka and M. Irie, In Situ Preparation of Highly Fluorescent Dyes upon Photoirradiation, J. Am. Chem. Soc., 2011, 133, 13558–13564 CrossRef CAS PubMed.
- T. Fukaminato, M. Tanaka, L. Kuroki and M. Irie, Invisible photochromism of diarylethene derivatives, Chem. Commun., 2008, 3924–3926 RSC.
- S. Aloïse, R. Yibin, I. Hamdi, G. Buntinx, A. Perrier, F. Maurel, D. Jacquemin and M. Takeshita, The photochemistry of inverse dithienylethene switches understood, Phys. Chem. Chem. Phys., 2014, 16, 26762–26768 RSC.
- K. Uchida and M. Irie, A Photochromic Dithienylethene That Turns Yellow by UV Irradiation, Chem. Lett., 1995, 969–970 CrossRef CAS.
- M. Irie and M. Morimoto, Photoswitchable Turn-on Mode Fluorescent Diarylethenes: Strategies for Controlling the Switching Response, Bull. Chem. Soc. Jpn., 2018, 91, 237–250 CrossRef CAS.
- Y. Takagi, T. Kunishi, T. Katayama, Y. Ishibashi, H. Miyasaka, M. Morimoto and M. Irie, Photoswitchable fluorescent diarylethene derivatives with short alkyl chain substituents, Photochem. Photobiol. Sci., 2012, 11, 1661–1665 CrossRef CAS PubMed.
- D. Kim, J. E. Kwon and S. Y. Park, Fully Reversible Multistate Fluorescence Switching: Organogel System Consisting of Luminescent Cyanostilbene and Turn-On Diarylethene, Adv. Funct. Mater., 2018, 28, 1706213 CrossRef.
- D. Okada, Z. Lin, J. Huang, O. Oki, O. Morimoto, X. Liu, T. Minari, S. Ishii, T. Nagao, M. Irie and Y. Yamamoto, Optical microresonator arrays of fluorescence-switchable diarylethenes with unreplicable spectral fingerprints, Mater. Horiz., 2020, 7, 1801–1808 RSC.
- D. Kim, K. Jeong, J. E. Kwon, H. Park, S. Lee, S. Kim and S. Y. Park, Dual-color fluorescent nanoparticles showing perfect color-specific photoswitching for bioimaging and super-resolution microscopy, Nat. Commun., 2019, 10, 3089 CrossRef PubMed.
- B. Roubinet, M. L. Bossi, P. Alt, M. Leutenegger, H. Shojaei, S. Schnorrenberg, S. Nizamov, M. Irie, V. N. Belov and S. W. Hell, Carboxylated Photoswitchable Diarylethenes
for Biolabeling and Super-Resolution RESOLFT Microscopy, Angew. Chem., Int. Ed., 2016, 55, 15429–15433 CrossRef CAS PubMed.
- K. Uno, A. Aktalay, M. L. Bossi, M. Irie, V. N. Belov and S. W. Hell, Turn-on mode diarylethenes for bioconjugation and fluorescence microscopy of cellular structures, Proc. Natl. Acad. Sci. U. S. A., 2021, 118, e2100165118 CrossRef CAS PubMed.
- H. Sotome, D. Kitagawa, T. Nakahama, S. Ito, S. Kobatake, M. Irie and H. Miyasaka, Cyclization reaction dynamics of an inverse type diarylethene derivative as revealed by time-resolved absorption and fluorescence spectroscopies, Phys. Chem. Chem. Phys., 2019, 21, 8623–8632 RSC.
- K. Uno, M. L. Bossi, V. N. Belov, M. Irie and S. W. Hell, Multicolour fluorescent “sulfide–sulfone” diarylethenes with high photo-fatigue resistance, Chem. Commun., 2020, 56, 2198–2201 RSC.
- T. Fukaminato, T. Doi, N. Tamaoki, K. Okuno, Y. Ishibashi, H. M. Miyasaka and M. Irie, Single-Molecule Fluorescence Photoswitching of a Diarylethene−Perylenebisimide Dyad: Non-destructive Fluorescence Readout, J. Am. Chem. Soc., 2011, 133, 4984–4990 CrossRef CAS PubMed.
- M. Berberich, M. Natali, P. Spenst, C. Chiorboli, F. Scandola and F. Würthner, Nondestructive Photoluminescence Read-Out by Intramolecular Electron Transfer in a Perylene Bisimide-Diarylethene Dyad, Chem. – Eur. J., 2012, 18, 13651–13664 CrossRef CAS PubMed.
- K. Uno, M. L. Bossi, T. Konen, V. N. Belov, M. Irie and S. W. Hell, Asymmetric Diarylethenes with Oxidized 2-Alkylbenzothiophen-3-yl Units: Chemistry, Fluorescence, and Photoswitching, Adv. Opt. Mater., 2019, 7, 1801746 CrossRef.
- A. M. Brouwer, Standards for photoluminescence quantum yield measurements in solution (IUPAC Technical Report), Pure Appl. Chem., 2011, 83, 2213–2228 CrossRef CAS.
-
M. J. Frisch, G. W. Trucks, H. B. Schlegel, G. E. Scuseria, M. A. Robb, J. R. Cheeseman, G. Scalmani, V. Barone, B. Mennucci, G. A. Petersson, H. Nakatsuji, M. Caricato, X. Li, H. P. Hratchian, A. F. Izmaylov, J. Bloino, G. Zheng, J. L. Sonnenberg, M. Hada, M. Ehara, K. Toyota, R. Fukuda, J. Hasegawa, M. Ishida, T. Nakajima, Y. Honda, O. Kitao, H. Nakai, T. Vreven, J. A. Montgomery Jr., J. E. Peralta, F. Ogliaro, M. Bearpark, J. J. Heyd, E. Brothers, K. N. Kudin, V. N. Staroverov, R. Kobayashi, J. Normand, K. Raghavachari, A. Rendell, J. C. Burant, S. S. Iyengar, J. Tomasi, M. Cossi, N. Rega, J. M. Millam, M. Klene, J. E. Knox, J. B. Cross, V. Bakken, C. Adamo, J. Jaramillo, R. Gomperts, R. E. Stratmann, O. Yazyev, A. J. Austin, R. Cammi, C. Pomelli, J. W. Ochterski, R. L. Martin, K. Morokuma, V. G. Zakrzewski, G. A. Voth, P. Salvador, J. J. Dannenberg, S. Dapprich, A. D. Daniels, Ö. Farkas, J. B. Foresman, J. V. Ortiz, J. Cioslowski and D. J. Fox, Gaussian 09 Revision D.01, Gaussian Inc., Wallingford, CT, 2009 Search PubMed.
- P. Rodgers, What is a diffraction limit?, Nat. Nanotechnol., 2009 DOI:10.1038/nnano.2009.115.
- S. W. Hell, S. Jakobs and L. Kastrup, Imaging and writing at the nanoscale with focused visible light through saturable optical transitions, Appl. Phys. A, 2003, 77, 859–860 CrossRef CAS.
- T. L. Andrew, H. Y. Tsai and R. Menon, Confining light to deep subwavelength dimensions to enable optical nanopatterning, Science, 2009, 324, 917–921, DOI:10.1126/science.1167704.
- For the use of spyropyranes (a) and azobenzenes (b, c), see:
(a) H. Vijayamohanan, E. F. Palermo and C. K. Ullal, Spirothiopyran-Based Reversibly Saturable Photoresist, Chem. Mater., 2017, 29, 4754–4760 CrossRef CAS;
(b) Y. Cao, Z. Gan, B. Jia, R. A. Evans and M. Gu, High-photosensitive resin for super-resolution direct-laser-writing based on photoinhibited polymerization, Opt. Express, 2011, 19, 19486–19494 CrossRef CAS PubMed;
(c) H.-Y. Tsai, S. W. Thomas and R. Menon, Parallel scanning-optical nanoscopy with optically confined probes, Opt. Express, 2010, 18, 16014–16024 CrossRef CAS PubMed.
- R. Menon and H. I. Smith, Absorbance-modulation optical lithography, J. Opt. Soc. Am. A, 2006, 23, 2290–2294 CrossRef PubMed.
|
This journal is © the Partner Organisations 2022 |
Click here to see how this site uses Cookies. View our privacy policy here.