DOI:
10.1039/D2QO00400C
(Research Article)
Org. Chem. Front., 2022,
9, 2949-2954
Synthesis of morpholino nucleosides starting from enantiopure glycidol†
Received
11th March 2022
, Accepted 12th April 2022
First published on 13th April 2022
Abstract
The synthesis of modified morpholino monomers was performed in a few steps through the condensation between 6-hydroxymethyl-morpholine acetal and nucleobases under Lewis acid conditions. The key common precursor of the targets – 6-hydroxymethyl-morpholine acetal – is easily synthesised via oxirane ring opening of optically pure glycidol using N-nosyl aminoacetaldehyde as a nucleophile, followed by an O-benzoylation/ring-closure tandem reaction sequence. Using readily available building blocks, this strategy allows access to diversified optically pure morpholino monomers in good yields and anomeric ratios.
Introduction
During the past few decades, the development of “oligonucleotide therapies” has been revealed as a crucial strategy for the treatment of mutagenic diseases, leading to repercussions throughout the synthetic planning in drug discovery. Synthetic oligonucleotides regulate gene expression by interacting selectively with the RNA target. As a consequence, the transfer of genetic information and, in turn, the production of degenerate proteins are blocked.1 Depending on the modes of action, different synthetic oligos were realized.2 Among these analogues, the antisense3 oligos phosphorodiamidate morpholinos (PMOs) have gained substantial interest, offering realistic prospects for the treatment of diseases involved in gene expression.4 The synthesis of the monomers was disclosed by the Summerton research group in 1997 (Fig. 1, path a).5 Following this procedure, several modifications were realised on the morpholino subunit to enhance or modify the PMO properties. Modifications have been realised at the phosphoramidate linkage,6 either dendrimers or peptides rich in arginine residues at the terminal 3′-nitrogen atom were introduced.7 In this way, two PMO drugs have been approved for the treatment of Duchenne muscular dystrophy.8 Modifications at the nucleobase level have also been explored by functionalization of the canonical morpholino monomer.9
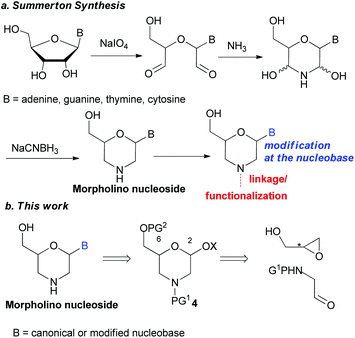 |
| Fig. 1 Chemical structures of the morpholino unit and functionalization sites. | |
Beyond the interest in the oligonucleotide field, 2,6-substituted morpholines are widely embedded in compounds with a range of biological activities.10 For example, this class of heterocycles has found wide application in peptide synthesis,11 and has been used as HIV protease inhibitors,12 antimicrobial agents,13 or therapeutics in obesity and diabetes,14 tumors,15 sexual dysfunction,16 and pain experience.17 Therefore, various synthetic strategies have been reported in the literature to access the 2,6-substituted morpholine skeleton.18 To the best of our knowledge any of them describes the direct morpholine functionalization with a nucleic base.
On these bases and given our ongoing interest in the synthesis of functionalised nitrogen containing heterocycles,19 here we reported our efforts toward the development of a synthetic protocol for nucleobase-modified morpholino monomers (Fig. 1, path b). The key step of the process involves the condensation of 4, which is the common precursor of target nucleoside analogues, with model nucleic bases as nucleophiles under Lewis acid-catalysed glycosylation reaction conditions. The proposed strategy gives access to optically pure compounds whose stereochemistry is strictly related to the geometry of the starting epoxide sources.
Results and discussion
The protocol for the synthesis of morpholinos begins with the nucleophilic ring-opening of optically pure (R)-glycidol using N-nosyl 2,2-dimethoxyethanamine (1). The ortho-nosyl (Nso) protective group was selected for its easy cleavage under mild reaction conditions. This reaction was carried out under solid–liquid phase transfer catalysis (SL-PTC) conditions (Scheme 1, step a), which did not affect the carbon stereocenter20 and the corresponding enantiopure tertiary amide 2 was isolated in very good yield. The key intermediate 4 was then prepared through a tandem protecting/ring-closing sequence by reacting equimolar amounts of diol 2, benzoyl chloride and pyridine (Scheme 1, step b).21 Benzoylation of the primary hydroxy function generated the intermediate 3 that, through in situ cyclization promoted by pyridinium chloride, finally gave the desired acetal 4 in 92% yield. The ring-closing reaction to 4 formed a glycosyl-like hemiacetal bond, with the consequent formation of the anomeric C-2 carbon atom (25
:
75-α/β anomeric ratio), which is the reactive site for the nucleobase attack to the morpholine ring.
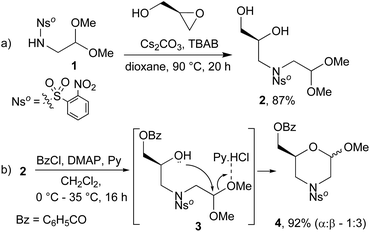 |
| Scheme 1 Synthesis of the key precursor (4) of morpholino nucleosides. Reaction conditions: (a) 1 (2.2 mmol), (R)-glycidol (2.0 mmol), dioxane (4.0 mL), Cs2CO3 (0.2 mmol), TBAB (0.2 mmol); (b) 2 (1.0 mmol), BzCl (1.1 mmol), Py (1.0 mmol), DMAP (0.1 mmol), CH2Cl2 (3.0 mL). | |
To study the nucleobase insertion reaction conditions, 4 was coupled with adenine (A) (Table 1). Preliminary runs, in the presence of SnCl4, evidenced that the use of MeCN as a solvent and the addition of a Lewis acid at 0 °C followed by the raising of the reaction temperature to 25 °C are the essential requisites for the reaction progress (entry 1). Under these conditions, 5α was isolated as the major anomer (47%) together with 5β (22%). This 68
:
32-α/β ratio remained the same when pure 4α or 4β anomer was made to react instead of using the 4α,β mixture. Since the anomeric configuration of 4 does not influence the product stereochemistry, we suppose that the reaction most likely proceeds via an intermediate oxocarbenium ion ox-4a. Moreover, since N-glycosylation of monosaccharides, except in the case of 2-deoxysugar,22 provided complete β-selectivity,23 we can assume that the ratio of the 5α/5β mixture depends on the absence of coordinating substituents at the C-3 position of 4. Further experiments indicated that the use of a higher or lower reaction temperature was detrimental (entries 2 and 3).
Table 1 Lewis acid activation of 4: study of the reaction conditionsa
Using DMF, CH2Cl2, or p-xylene as the solvent instead of -MeCN (entries 4–6), a complex reaction mixture was formed. In the presence of softer Lewis acids (such as indium trichloride, ytterbium chloride, zirconium tetrachloride or titanium isopropoxide), morpholine 4 was recovered unchanged (entries 7–10), while titanium tetrachloride furnished a mixture of anomers 5α,β (entry 11). Conversely, using trimethylsilyl (trifluoromethane)sulfonate (TMSOTf, entry 12), together with a good overall yield, a reversed 5α/5β ratio was found, 5β being the major anomer.
To evaluate the scope of this procedure, the best reaction conditions using TMSOTf were applied to the condensation of acetal 4 with modified nucleobases 6-chloropurine (CHP, Fig. 2), silylated hypoxanthine (HPX), 2,6-diaminopurine (Z), and silylated 5-fluorouracil (5-FU). To our delight, nucleobases NB-H (Scheme 2) gave the corresponding N,O-protected monomers 6–9 in good yields with the prevalence of the β-anomer. The condensation of 4 with the adenine derivatives, CHP and silylated HPX, gave analogous results to that obtained with adenine itself, and the corresponding analogues 6 and 7 were isolated in 69% and 73% yield, respectively, in a 30
:
70-α/β anomeric ratio. In particular, the reaction conditions promoted the silylether cleavage in HPX providing the corresponding O-deprotected analogues 7. The reaction on Z furnished the corresponding nucleoside 8β in 42% yield. It is worth noting that 8α has not been isolated, even though the NMR spectra of the crude showed a set of signals consistent with this anomer. Finally, the reaction of silylated 5-FU gave both better yield and selectivity of the corresponding O,O-deprotected products 9 (85% yield; 25
:
75-α/β anomeric ratio).
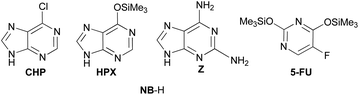 |
| Fig. 2 Nucleobase NB-H employed in the synthesis of morpholinos. | |
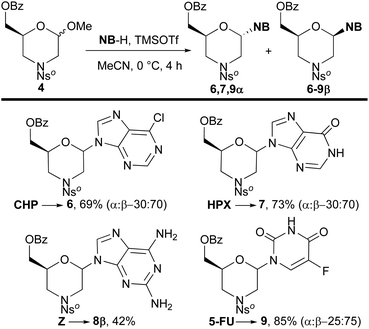 |
| Scheme 2 Scope of the reaction: synthesis of protected morpholino derivatives 6–9. Reaction conditions: 4 (0.5 mmol), NB-H (1.0 mmol), TMSOTf (1.5 mmol), MeCN (1.0 mL). | |
To investigate the chemoselective N- and O-deprotection reactions of these compounds, 9β was chosen as a model.
Firstly, the N-(2-nitrobenzene)sulfonyl group was efficiently removed by reaction with p-thiocresol and the corresponding morpholine 10β was isolated in good yields (Scheme 3, step a). In the next step, the benzoate function was removed from 10β by trans-esterification with sodium methoxide in refluxing methanol, and the corresponding 5-fluorouridine analogue 11β was isolated in good yields (Scheme 3, step b).
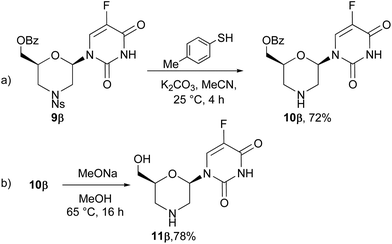 |
| Scheme 3
N- and O-deprotection of the 5-fluorouridine analogue 9β. Reaction conditions: (a) 9β (0.3 mmol), 4-methylbenzenethiol (0.4 mmol), K2CO3 (1.0 mmol), MeCN (3.0 mL); (b) 10β (0.23 mmol), MeONa (0.5 mmol), MeCN (1.0 mmol), MeOH (2.0 mL). | |
To by-pass the O-protection/deprotection steps and to better understand the cyclization mechanism, the same runs were carried out (Scheme 4). Initially, one-pot O-protection/cyclization/NB-H nucleophilic attack was performed on diol 2 in the presence of BzCl and 5-FU (path a), without adding a base (i.e.pyridine; Scheme 1, step b). Under these conditions, the target compound 9 was isolated in only 14% yield together with a large amount of bicyclic 1,3-dioxolane 12 (path a). A successive attempt at the tandem cyclization/NB-H coupling reaction under similar conditions, without benzoyl chloride (path b), gave 12 as the sole product. The hypothesized mechanism for the formation of 12 implicates the formation of a zwitterionic species ox-4b that, in turn, was evolved by intramolecular attack of the oxanion, the strongest nucleophile, on the carbocation. Besides, compound 12 resulted to be stable when made to react with a large excess (4.0 mol equiv.) of 5-FU, whereas when BzCl was used together with 5-FU, a small amount of 9 was formed (path c). The latter result proves that, under these conditions, an equilibrium between dioxolane 12 and the oxonium species ox-4b is effective. Actually, by O-benzoylation of ox-4b, the oxonium chloride ox-4c forms and then it can evolve to 9.
 |
| Scheme 4 Reactivity of diol 2. Reaction conditions: (a) 2 (0.5 mmol), 5-FU (1.0 mmol), BzCl (0.7 mmol), TMSOTf (1.5 mmol), MeCN (2.0 mL); (b) 2 (0.5 mmol), 5-FU (4.0 mmol), TMSOTf (1.5 mmol), MeCN (2.0 mL); (c) 12 (0. mmol), 5-FU (1.5 mmol), BzCl (0.7 mmol), TMSOTf (1.5 mmol), MeCN (2.0 mL). | |
NMR experiments corroborate the stereochemistry assigned to protected morpholino anomers 5,6,7, and9α and 5–9β. As shown for compounds 5α,β and 9α,β (Fig. 3), the NOESY analysis of the β-anomers showed H2–H6 spatial proximity indicating, in agreement with the J values, that the substituents on C2 and C6 are both equatorial. In fact, this interaction is absent in the α-anomers. Furthermore, in the 5α anomer, the H′2–H6 correlation was found, revealing their proximity. On the other hand, derivative 5β presented a weak H3eq–H′8 correlation. The NH2 residue of purine residues in 5α,β did not show spatial proximity to any other proton. As regard the pyrimidine derivative 9α, a correlation between H6–H′6 occurs, while the nucleobase in 9β did not reveal any spatial interaction.
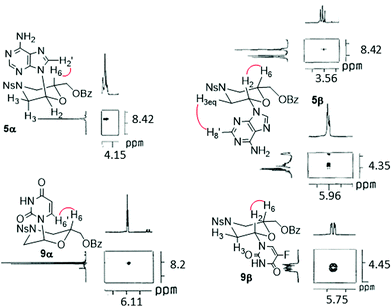 |
| Fig. 3 Selected NOE correlations for morpholino 5–9. | |
Conclusions
In summary, we have described a rapid synthesis of morpholino monomers from readily available building blocks. The 2-methoxymorpholine 4 is the key intermediate for the direct installation of the nucleobase. The proposed methodology furnished the biologically relevant β-morpholino analogues as the major anomers, when (trimethyl)silyltriflate was used to promote the condensation reaction. Conversely, when SnCl4 was used as a Lewis acid, the main reaction products were the α-anomers, which have never been synthesised before. Pseudo α- and β-morpholino nucleosides, having R-configuration on C6, could be constructed using (S)-glycidol in the synthesis of 2. Further work will also be done to study and extend the activation of morpholine 4 toward different nucleophiles.
Conflicts of interest
There are no conflicts to declare.
References
-
(a)
A. Aartsma-Rus, A. L. Jackson and A. A. Levin, Mechanisms of oligonucleotide actions, in Oligonucleotide-Based Drugs and Therapeutics, ed. N. Ferrari and R. Seguin, John Wiley & Sons, 2018, p. 1 Search PubMed;
(b) W. Yin and M. Rogge, Targeting RNA: A transformative therapeutic strategy, Clin. Transl. Sci., 2019, 12, 98 CrossRef CAS PubMed.
- For a review about oligonucleotides, see: C. I. E. Smith and R. Zain, Therapeutic Oligonucleotides: State of the Art, Annu. Rev. Pharmacol. Toxicol., 2019, 59, 605 CrossRef CAS PubMed and references therein.
- For a review about antisense oligos, see:
R. Kole, A. R. Krainer and S. Altman, RNA therapeutics: beyond RNA interference and antisense oligonucleotides, Nat. Rev. Drug Discovery, 2012, 11, 125 Search PubMed and references therein.
- For a review about morpholinos’ modes of action and applications, see: J. D. Moulton, Guide for Morpholino Users: Toward Therapeutics, J. Drug Discovery Dev. and Delivery, 2016, 3 Search PubMed , 1021 and references therein.
- J. Summerton and D. Weller, Morpholino antisense oligomers: design, preparation and properties, Antisense Nucleic Acid Drug Dev., 1997, 7, 187 CrossRef CAS PubMed.
-
(a) H. K. Langner, K. Jastrzebska and M. H. Carruthers, Synthesis and Characterization of Thiophosphoramidate Morpholino Oligonucleotides and Chimeras, J. Am. Chem. Soc., 2020, 142, 16240 CrossRef CAS PubMed;
(b)
M. Carruthers and P. Sibasish, Synthesis of backbone modified morpholino oligonucleotides and chimeras using phosphoramidite chemistry, U.S. Patent ApplicationUS16/359776, 2019 Search PubMed;
(c)
P. P. Seth and M. Ostergaard, Morpholino modified oligomeric compounds, WO2018/165564A1, 2018;
(d) M. J. Palframan, R. D. Alharthy, P. K. Powalowska and C. J. Hayes, Synthesis of triazole-linked morpholino oligonucleotides via Cu(I) catalysed cycloaddition, Org. Biomol. Chem., 2016, 14, 3112 RSC;
(e) N. Zhang, C. Tan, P. Cai, Y. Jiang, P. Zhang and Y. Zhao, Synthesis and properties of morpholino chimeric oligonucleotides, Tetrahedron Lett., 2008, 49, 3570 CrossRef CAS;
(f) N. Zhang, C. Tan, P. Cai, P. Zhang, Y. Zhao and Y. Jiang, RNA interference in mammalian cells by siRNAs modified with morpholino nucleoside analogues, Bioorg. Med. Chem., 2009, 17, 2441 CrossRef CAS PubMed.
-
(a) H. M. Moulton, In vivo delivery of morpholino oligos by cell-penetrating peptides, Curr. Pharm. Des., 2013, 19, 2963 CrossRef CAS PubMed;
(b) P. A. Morcos, Y. Li and S. Jiang, Vivo-Morpholinos: A non-peptide transporter delivers Morpholinos into a wide array of mouse tissues, BioTechniques, 2008, 45, 616 CrossRef PubMed.
-
(a) K. R. Q. Lim, R. Maruyama and T. Yokota, Eteplirsen in the treatment of Duchenne muscular dystrophy, Drug Des., Dev. Ther., 2017, 11, 533 CrossRef CAS PubMed;
(b) Y.-A. Heo, Golodirsen: First Approval, Drugs, 2020, 80, 329 CrossRef PubMed.
-
(a)
B. Nandi, S. Pattanayak, S. Paul, J. Kundu and S. Sinha, Synthesis of Nucleobase-Functionalized Morpholino Monomers, in Non-Natural Nucleic Acids. Methods in Molecular Biology, ed. N. Shank, Humana, New York, 2019, p. 107 Search PubMed;
(b) I. P. Vohtancev, Y. V. Sherstyuk, V. N. Silnikov and T. V. Abramova, Effective Synthesis of 5-Iodo Derivatives of Pyrimidine Morpholino Nucleosides, Org. Prep. Proced. Int., 2018, 50, 332 CrossRef CAS.
- For recent reviews about morpholine containing bioactive molecules, see:
(a) A. Tzara, D. Xanthopoulos and A. P. Kourounakis, Morpholine as a Scaffold in Medicinal Chemistry: An Update on Synthetic Strategies, ChemMedChem, 2020, 15, 392 CrossRef CAS PubMed;
(b) A. P. Kourounakis, D. Xanthopoulos and A. Tzara, Morpholine as a privileged structure: A review on the medicinal chemistry and pharmacologicalactivity of morpholine containing bioactive molecules, Med. Res. Rev., 2020, 40, 709 CrossRef CAS PubMed and references therein.
-
(a) For a review about peptide synthesis and interaction, see: R. Bucci, F. Foschi, C. Loro, E. Erba, M. L. Gelmi and S. Pellegrino, Fishing in the Toolbox of Cyclic Turn Mimics: a Literature Overview of the Last Decade, Eur. J. Org. Chem., 2021, 2887 CrossRef CAS and references therein;
(b) C. R. Kinzie, A. D. Steele, S. M. Pasciolla and W. M. Wuest, Synthesis of cyclic dimeric methyl morpholinoside – a common synthetic precursor to cyclic dinucleotide analogs, Tetrahedron Lett., 2014, 55, 4966 CrossRef CAS;
(c) N. Debreczeni, M. Bege, M. Herczeg, I. Bereczki, G. Batta, P. Herczegh and A. Borbás, Tightly linked morpholino-nucleoside chimeras: new, compact cationic oligonucleotide analogues, Org. Biomol. Chem., 2021, 19, 8711 RSC;
(d) F. Vaghi, R. Bucci, F. Clerici, A. Contini and M. L. Gelmi, Non-natural 3-Arylmorpholino-β-amino Acid as a PPII Helix Inducer, Org. Lett., 2020, 22, 6197 CrossRef CAS PubMed;
(e) R. Bucci, A. Contini, F. Clerici, S. Pellegrino and M. L. Gelmi, From glucose to enantiopure morpholino β-amino acid: A new tool for stabilizing γ-turns in peptides, Org. Chem. Front., 2019, 6, 972 RSC;
(f) V. V. Kapoerchan, E. Spalburg, A. J. De Neeling, R. H. Mars-Groenendijk, D. Noort, J. M. Otero, P. Ferraces-Casais, A. L. Llamas-Saiz, M. J. Van Raaij, J. Van Doorn, G. A. Van Der Marel, H. S. Overkleeft and M. Overhand, Gramicidin S Derivatives Containing cis– and trans–Morpholine Amino Acids (MAAs) as Turn Mimetics, Chem. – Eur. J., 2010, 16, 4259 CrossRef CAS PubMed;
(g) F. Sladojevich, A. Guarna and A. Trabocchi, Evaluation of stereochemically dense morpholine-based scaffolds as proline surrogates in β-turn peptides, Org. Biomol. Chem., 2010, 8, 916 RSC.
-
J. A. Mccauley, C. Beaulieu, D. J. Bennett, C. J. Bungard, S. Crane, T. J. Greshock, M. K. Holloway, S. Lessard, D. Mckay, C. Molinaro, O. Moradei, M. V. Sivalenka, V. Linh Truong, S. Tummanapalli and P. D. Williams, Preparation of 2,6-substituted morpholine derivatives as HIV protease inhibitors, PCT Int. ApplWO2015134366A1, 2015 Search PubMed.
-
E. Carlson, H. Hansen, L. Hawkins, S. Ishizaka, M. Mackey, S. Schiller, C. Ogawa and H. Davis, Selectively substituted quinoline compounds as TLR7 and TLR8 antagonists or inhibitors and their preparation, U.S. Pat. Appl. PublUS20150105370A1, 2015 Search PubMed.
-
(a) Y. Tamura, N. Omori, N. Kouyama, Y. Nishiura, K. Hayashi, K. K. Watanabe, Y. Tanaka;, T. Chiba, H. Yukioka, H. Sato and T. Okuno, Identification of a novel and orally available benzimidazole derivative as an NPY Y5 receptor antagonist with in vivo efficacy, Bioorg. Med. Chem. Lett., 2012, 22, 6554 CrossRef CAS PubMed;
(b)
N. Kouyama, A. Yukimasa, Y. Nishiura and Y. Tamura, Preparation of heterocyclic compounds as NPY Y5 receptor antagonists, PCT Int. ApplWO2012020725A1, 2012 Search PubMed.
- For a review about the anticancer use of morpholines, see: F. Arshad, M. F. Khana, W. Akhtar, M. M. Alam, L. M. Nainwal, S. K. Kaushika, M. Akhter, S. Parvez, S. M. Hasan and M. Shaquiquzzaman, Revealing quinquennial anticancer journey of morpholine: A SAR based review, Eur. J. Med. Chem., 2019, 167, 324 CrossRef CAS PubMed and references therein.
-
C. M. N. Allerton, A. D. Baxter, A. S. Cook, D. Hepworth and K.-F. Wong, Preparation of 2-phenylmorpholines and related compounds as dopamine agonists in the treatment of sexual dysfunction, PCT Int. ApplWO2004052372A1, 2004 Search PubMed.
-
M. A. Ackley, Preparation of N-propylmorpholine derivatives as dopamine agonists
for the treatment of pain, PCT Int. ApplWO2008087512A1, 2008 Search PubMed.
- For a review about morpholine synthesis, see:
(a) V. A. Palchykov and V. A. Chebanov, Recent progress in the synthesis of morpholines, Chem. Heterocycl. Compd., 2019, 55, 324 CrossRef CAS and references therein;
(b) D. Yang, C. Wang, Y. Wang, G. Liu, T. Cheng and R. Liu, One-pot enantioselective construction of 3,4-dihydro-2H-1,4-oxazines over Ru/Au relay catalysis and its mechanistic serendipity, Org. Chem. Front., 2021, 9, 117 Search PubMed.
-
(a) C. Loro, L. Molteni, M. Papis, E. M. Beccalli, D. Nava, L. Lo Presti, S. Brenna, G. Colombo, F. Foschi and G. Broggini, Direct Synthesis of Fluorescent Oxazolo-phenoxazines by Copper-Catalyzed/Hypervalent Iodine(III)-Mediated Dimerization/Cyclization of 2-Benzylamino-phenols, J. Org. Chem., 2022, 87, 1032 CrossRef CAS PubMed;
(b) L. Pinzi, F. Foschi, M. S. Christodoulou, D. Passarella and G. Rastelli, Design and Synthesis of Hsp90 Inhibitors with B-Raf and PDHK1 Multi-Target Activity, ChemistryOpen, 2021, 10, 1177 CrossRef CAS PubMed;
(c) E. Bonandi, F. Foschi, C. Marucci, M. Luzzani and D. Passarella, Vincamine by synthesis and semi-synthesis, Phytochem. Rev., 2021, 20, 343 CrossRef CAS;
(d) M. S. Christodoulou, S. Giofrè, E. M. Beccalli, F. Foschi and G. Broggini, Divergent Conversion of 4-Naphthoquinone-substituted 4 H-Isoxazolones to Different Benzo-fused Indole Derivatives, Org. Lett., 2020, 22, 2735 CrossRef CAS PubMed;
(e) S. Giofrè, E. M. Beccalli, F. Foschi, C. La Rosa, L. Lo Presti and M. Christodoulou, Chemo- and Regioselective Palladium(II)-Catalyzed Aminoarylation of N-Allylureas Providing 4-Arylmethyl Imidazolidinones, Synthesis, 2019, 51, 1 CrossRef;
(f) F. Foschi, C. Loro, R. Sala, J. Oble, L. Lo Presti, E. M. Beccalli, G. Poli and G. Broggini, ‘Intramolecular Aminoazidation of Unactivated Terminal Alkenes by Palladium-Catalyzed Reactions with Hydrogen Peroxide as the Oxidant, Org. Lett., 2020, 22, 1402 CrossRef CAS PubMed;
(g) S. Gazzola, E. M. Beccalli, T. Borelli, C. Castellano, D. Diamante and G. Broggini, Selective 7-endo-Cyclization of 3-Aza-5-alkenols through Oxidative Pd(II)-Catalyzed Olefin Oxyarylation, Synlett, 2018, 29, 503 CrossRef CAS;
(h) F. Foschi, D. Albanese, I. Pecnikaj, A. Tagliabue and M. Penso, Regioselective O-Sulfonylation of N,N-bis(2-Hydroxyalkyl)-tosylamides as a Synthetic Key-Step to Enantiopure Morpholines, Org. Lett., 2017, 19, 70 CrossRef CAS PubMed;
(i) D. Albanese, F. Foschi and M. Penso, A Practical Approach to the Synthesis of Enantiomerically Pure 2,6-Disubstituted Morpholines under Phase Transfer Catalysis Conditions, Catal. Today, 2009, 140, 100 CrossRef CAS;
(j) M. Penso, F. Foschi, S. Pellegrino, A. Testa and M. L. Gelmi, Diastereoselective protocols for the synthesis of 2,3-trans- and 2,3 cis-6-methoxy-morpholine-2-carboxylic acid derivatives, J. Org. Chem., 2012, 77, 3454 CrossRef CAS PubMed.
-
(a) G. Pozzi, V. Mihali, F. Foschi, M. Penso, S. Quici and R. H. Fish, 3,5-Bis(n-perfluorooctyl)benzyltriethyl ammonium Bromide (F-TEBA): An Efficient, Easily Recoverable Fluorous Catalyst for Solid-Liquid PTC Reactions, Adv. Synth. Catal., 2009, 351, 3072 CrossRef CAS;
(b) see ref. 19c–f.
- For references on the employment of N-protected 2,2-dimethoxyethanamine as the electrophile, see:
(a) A. S. Gazizov, A. R. Burilov, M. A. Pudovik and O. G. Sinyashin, Reactions of nitrogen-containing acetals with aromatic nucleophiles, Russ. Chem. Rev., 2017, 86, 75 CrossRef CAS;
(b) R. Bogacki, D. M. Gill, W. J. Kerr, S. Lamont, J. A. Parkinson and L. C. Paterson, Flexible access to conformationally-locked
bicyclic morpholines, Chem. Commun., 2013, 49, 8931 RSC;
(c) A. Agrebi, F. Allouche, F. Chabchoub and L. El Kaïm, Cyclization Cascade of Hydrazono Ugi Adducts towards Pyrazoles, Eur. J. Org. Chem., 2013, 5805 CrossRef CAS;
(d) D.-Y. Liu, S.-M. Zhu, R.-Q. Li, J.-S. Tian and T.-P. Loh, α-Amino Acetal: A Synthetic Intermediate for the Construction of Aza-Polycycles, Org. Lett., 2019, 21, 6357 CrossRef CAS PubMed;
(e) M. Righi, F. Topi, S. Bartolucci, A. Bedini, G. Piersanti and G. Spadoni, Synthesis of Tryptamine Derivatives via a Direct, One-Pot Reductive Alkylation of Indoles, J. Org. Chem., 2012, 77, 6351 CrossRef CAS PubMed.
- C. Fossey, H. Landelle, D. Laduree and M. Robba, Synthesis of N-1β-D-Arabinofuranosyl and N-1-2′-Deoxy-β-D-erythropentofuranosyl Thieno [3,2-d] Pyrimidine Nucleosides, Nucleosides, Nucleotides Nucleic Acids, 1994, 13, 925 CrossRef CAS.
-
(a) U. Niedballa and H. Vorbruggen, A General Synthesis of N-Glycosides. I., Synthesis of Pyrimidine Nucleosides, J. Org. Chem., 1974, 39, 3654 CrossRef CAS PubMed;
(b) Y. Tor, S. Del Valle, D. Jaramillo, S. G. Srivatsan, A. Rios and H. Weizman, Designing new isomorphic fluorescent nucleobase analogues: the thieno[3,2-d]pyrimidine core, Tetrahedron, 2007, 63, 3608 CrossRef CAS.
|
This journal is © the Partner Organisations 2022 |