DOI:
10.1039/D2QO00184E
(Research Article)
Org. Chem. Front., 2022,
9, 2471-2476
The copper-catalyzed radical aminophosphinoylation of maleimides with anilines and diarylphosphine oxides†
Received
4th February 2022
, Accepted 18th March 2022
First published on 18th March 2022
Abstract
The copper-catalyzed radical multi-component coupling of maleimides, anilines, and diarylphosphine oxides has been realized, providing the rapid synthesis of a library of aminophosphinoylated maleimides, with the formation of C–N and C–P bonds. The remarkable feature of the current multi-component reaction was that five clinical drug molecules containing sulfonamide and arylamine functional groups underwent chemo-selective radical vinylphosphinoylation to access sterically congested products. Most impressively, this transformation worked on a wide range of substrates and showed good functional group tolerance.
Introduction
Recent studies have shown that β-phosphoryl enamines, owing to their unique structural characteristics, play an irreplaceable role in the fields of organic ligands, candidate pharmaceutical molecules and material chemistry.1 Thus, tremendous efforts have been devoted to designing valuable synthetic approaches for enriching the diversity and applicability of their derivatives.2 The initial studies involved Rh-catalyzed hydroaminovinylation of vinylphosphonate with alkyl amines,3 condensation of alkyl phosphonates with a Michael receptor4 and hydrophosphorylation of ynamides.5 However, these approaches require expensive metal catalysts and involve the use of harsh reaction conditions that would impede the discovery of new drugs. In recent years, direct C–H phosphorylation of enamides by way of various catalytic pathways has also attracted considerable attention (Scheme 1A).6 These described protocols rely on the preparation of substrates, a requirement of stoichiometric Mn or silver oxidant in some cases, and usually have the nitrogen atom of the starting material protected by electron-deficient groups under oxidative reaction conditions. These two-component reactions are key ways to prepare β-phosphoryl enamines, but three-component cascade reactions remain elusive. There is a considerable need to develop a clever complementary strategy for enriching the chemistry of β-phosphoryl enamines and achieving late-stage functionalization of polyfunctional drugs.
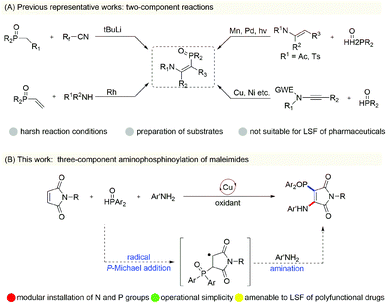 |
| Scheme 1 The synthetic strategy for the preparation of β-phosphoryl enamines. | |
Recently, we and other groups have demonstrated copper-catalyzed oxidative aminofunctionalizations of maleimides,7 and in these transformations have achieved in situ formation of enamine intermediates from the oxidative amination of maleimides with electron-rich secondary alkylamines, followed by copper-catalyzed oxidative cross-coupling with various nucleophilic reagents with high reaction efficiency. The success of these multi-component reactions lies in the secondary alkylamines being more nucleophilic than the other nucleophiles. It is difficult to get aromatic amines with low nucleophilicity to participate in the difunctionalization reactions of maleimides when using this strategy. In light of the significance of maleimide chemistry and the requirement of β-phosphoryl enamines, we wondered whether copper-catalyzed radical aminophosphinoylation of maleimides could be developed by performing radical addition of phosphinoyl with maleimide and then amination reactions (Scheme 1B). This protocol could overcome the intrinsic reactivity of anilines and provide a new strategy to afford the β-phosphoryl enamine motif. However, the preparation of aminophosphinoylated maleimides using the above-mentioned transformation is extremely challenging, mainly due to the well-developed and incidental copper-catalyzed oxidative dehydrogenation coupling of anilines to lead to aromatic azo compounds,8 hydrophosphinylation of H-phosphonates with electron-deficient alkenes,9 oxidative N–P cross-coupling between H-phosphonates and anilines,10 and homo-dehydrogenative P–P and P–O–P coupling of H-phosphonates.11
Results and discussion
We used inexpensive and readily available aniline 1a, N-phenyl maleimide 2a, and diphenylphosphine oxide 3a as a model substrate, and performed the radical multi-component aminophosphinoylation to examine our assumptions (Table 1, for details see ESI†). Based on our previous experience with maleimide chemistry, we focused on the inexpensive copper catalysts. After simple optimization of reaction conditions, we found that using DTBP as a free radical oxidant and CuCl as a catalyst, the desired product 4a was obtained with a yield of 11% (entry 1). Using other oxidants, namely 70% TBHP, K2S2O8, Mn(OAc)3 and TBPB, each led to lower yields than did using DTBP (entries 2–5). Some undesired byproduct from the P-Michael addition of maleimide was formed during reaction optimization. In other words, aniline did not rapidly capture the carbon free radical intermediate, thus reducing the efficiency of the conversion of the raw materials. Therefore, organic and inorganic bases commonly used in the laboratory were screened (entries 6–9); use of Li2CO3 as the base improved the yield of the target product, and the reaction became relatively clean. The choice of reaction solvent was found to be very important for accessing the target product. Replacing CH3CN with a CH3CN/toluene mixture increased the yield to 61% (entry 10). Subsequently, we re-screened a series of useful copper salts and found that CuCl2 showed the best catalytic performance (entry 11). Next, some additives or ligands were included in the catalytic system in an attempt to improve the yield of the target product. Surprisingly, introduction of the bidentate ligand 1,10-phen led to a sharp drop in yield (entry 12), which may have been due to the ligand having coordinated the copper ion and reducing its Lewis acidity. In addition, the current radical cascade reaction was found to be insensitive to the reaction atmosphere, and product in considerable yield was still obtained under oxygen conditions (entry 13).
Table 1 Reaction optimizationa
As illustrated in Scheme 2, the multi-component radical aminophosphinoylation reaction was found to provide a concise pathway to construct a comprehensive library of aminophosphinoylated maleimides. At first, N-phenyl maleimide and diphenylphosphine oxide were used as cross-coupling partners. A range of arylamines having different substituents with distinct electronic properties effectively participated in the current transformation. Specifically, a wide range of common functional groups, namely methyl (4b–4e), ester (4f), trifluoromethoxy (4h), trifluomethylthio (4i), methoxyl (4j), halogen (4k–4m), sulfonyl (4n), acyl (4o) and trifluoromethyl (4p) groups, were nicely accommodated in these reactions. However, ortho-substituted arylamine substrates provided the corresponding products in relatively low yields (4d–4g), which may have been due to steric repulsion. Most importantly, 4-iodoaniline delivered the anticipated vinylphosphinoylation product (4m) with high reaction efficiency, highlighting the powerful generality and appeal of the late-stage modification of the achieved products. Additionally, the practicability of this reaction was demonstrated on the 5 mmol reaction scale, with the target product 4a obtained in 70% yield. Alkyl amines did not act as efficient coupling partners, probably due to the oxidative dehydrogenation of alkyl amines having resulted in their decompositions into aldehyde byproducts under oxidizing conditions.10 Note that the current multi-component reaction was found to not be limited to N-aryl substituted maleimide, and N-alkyl maleimides proved to be entirely compatible with the reaction and afforded the corresponding products in good to excellent yields. We have prepared a series of N-benzyl substituted maleimide substrates (4s–4ac) containing important functional groups suitable for this reaction and yielding good reaction efficiency. The oxidative aminophosphinoylation could be performed using polychlorinated maleimide as an electrophile (4z). Furthermore, the aminophosphinoylation coupling of electron-rich heteroaryl-substituted maleimide (4ac) was also feasible when using the optimal reaction conditions and the anticipated product was provided with a useful yield. To broaden the significant applicability of this protocol, methyl (4ad, 4ag), methoxyl (4ae, 4af) and chlorine (4ah)-substituted diarylphosphine oxides were tested for the current reaction conditions, and found to be suitable—with the corresponding products isolated in good yields. We also conducted an orthogonal experiment. Using a combination of different arylamines and organic phosphines effectively enriched the molecular library of aminophosphinoylated maleimides (4ai).
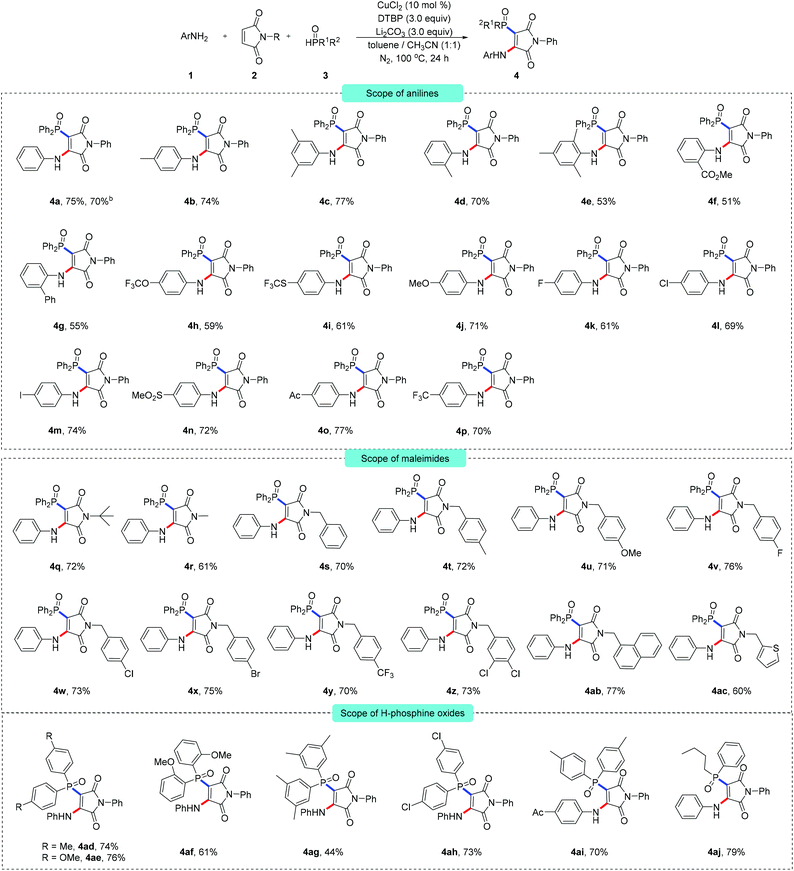 |
| Scheme 2 The radical aminophosphinoylation of maleimides, anilines, and diarylphosphine oxides. | |
Another aspect of the multi-component reaction that we noticed was that dialkylphosphites such as diethyl phosphite and diisopropyl phosphite were unsuitable for this transformation and did not give aminophosphonated products.
To highlight the practical application of the strategy involving radical aminophosphinoylation of maleimide, we sought to examine the late-stage modification of pharmaceuticals bearing sulfonamide and arylamine functional groups. As shown in Scheme 3, dapsone (5a), a sulfone drug used to treat acne vulgaris, was handily achieved using the above-mentioned protocol, which provided an efficient pathway for the discovery of a therapeutic agent for bacterial infection. Sulfanilamide (5b), an anti-vulvovaginal candidiasis drug, was readily converted into the desired product with unique chemo-selectivity by carrying out a radical vinylphosphinoylation. Sulfamethoxazole (5c), an oral sulfonamide antibiotic, was highly compatible with the reaction and gave the expected product in an acceptable yield. The sulfonamide antibiotic sulfapyridine (5d), commonly used to treat dermatitis herpetiformis, was also compatible and provided the vinylphosphinoylated product in good yield. Furthermore, the vinylphosphinoylated methyl sulfonamide clisetine was successfully obtained in 69% yield.
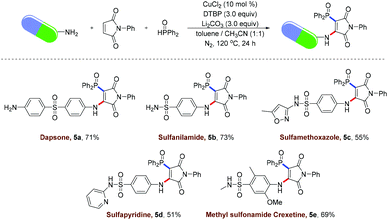 |
| Scheme 3 Synthetic applications. | |
To investigate whether the aminophosphinoylation of maleimide occurred through a free radical reaction process, as shown in Scheme 4, we selected BHT and 1,1-diphenyl ethylene as free radical scavengers and performed two control experiments (eqn (1) and (2)). In these cases, the desired conversion reaction was completely inhibited, and radical adduct 6a was isolated in 65% yield and radical adduct 6b was detected using GC-MS. Next, we studied the sequence of the multi-component reaction (eqn (3) and (4)). The reaction of N-phenyl maleimide with aniline or diphenylphosphine oxide under the standard reaction conditions showed hardly any nucleophilic addition and oxidative coupling products. Next, TBAB was added to the reaction between N-phenyl maleimide and diphenylphosphine oxide under the standard reaction conditions (eqn (5)); no bromophosphinoylated product 6g was generated. This experimental result excluded the formation of a carbocation intermediate.12 Finally, no reaction occurred between N,P,P-triphenylphosphinic amide and N-phenyl maleimide (eqn (6)). This result indicated the untenability of this transformation to progress by way of the insertion into the maleimide carbon–carbon double bond of an N–P bond. Based on the aforementioned experimental results, a tentative mechanism for aminophosphinoylation reaction was derived, as shown in Scheme 4C. According to this mechanism, single-electron oxidation of organophosphorus with DTBP generates phosphinoyl radicals in the presence of copper salt,13 followed by nucleophilic addition of maleimides with P-centered radical to form alkyl radical A.14 Then, the coordination between A and copper-aniline complex affords trivalent copper intermediate B,15 which undergoes reductive elimination to yield α,β-aminophosphinoylation product C. Finally according to the mechanism, copper-promoted oxidative dehydrogenation of C accesses the desired product.16
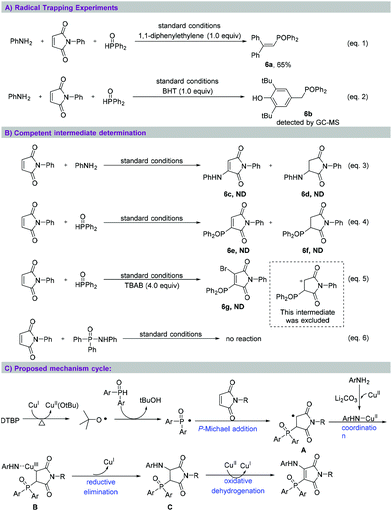 |
| Scheme 4 Mechanistic considerations and control experiments. | |
Conclusions
In summary, the concise and straightforward copper-catalyzed radical aminophosphinoylation of maleimides with anilines and diarylphosphine oxides was achieved, and a library of aminophosphinoylated maleimides with unique structures was efficiently constructed. Most impressively for this multi-component reaction was the ability to use it to effectively achieve chemo-selective radical vinylphosphinoylations of various drug molecules bearing multiple sulfonamide and arylamine functional groups, providing an available pathway for the discovery of new maleimide candidate drugs. Notably, this reaction was found to display a wide range of applications, excellent tolerance of functional groups, and good atom economy during synthesis.
Conflicts of interest
There are no conflicts to declare.
Acknowledgements
Financial support from the National Natural Science Foundation of China (21602158), Zhejiang Provincial Natural Science Foundation (LTQ20B010001), State Key Laboratory of Structural Chemistry (No. 20200008), and Public Welfare Science and Technology Project of Wenzhou (No. Y20210209) is greatly appreciated.
Notes and references
-
(a) J.-A. Ma, Catalytic Asymmetric Synthesis of α- and β-Amino Phosphonic Acid Derivatives, Chem. Soc. Rev., 2006, 35, 630–636 RSC
;
(b) H.-Q. Du and X.-P. Hu, Rh-Catalyzed Asymmetric Hydrogenation of (Z)-β-Phosphorylated Enamides: Highly Enantioselective Access to β-Aminophosphines, Org. Lett., 2019, 21, 8921–8924 CrossRef CAS PubMed
;
(c) M. Jin, S.-F. Yin and S.-D. Yang, Bismuth(III)-Catalyzed Sequential Enamine-Imine Tautomerism/2-Aza-Cope Rearrangement of Stable β-Enaminophosphonates: One-Pot Synthesis of β-Aminophosphonates, Org. Lett., 2020, 22, 2811–2815 CrossRef CAS PubMed
.
-
(a) T. Janecki, A. Albrecht, J. F. Koszuk, J. Modranka and D. Słowak, A Simple and Effective Synthesis of Activated Vinylphosphonates from 3-Methoxy-2-diethoxyphosphorylacrylate, Tetrahedron Lett., 2010, 51, 2274–2276 CrossRef CAS
;
(b) Y. Gao, G. Lu, P. Zhang, L. Zhang, G. Tang and Y. Zhao, A Cascade Phosphinoylation/Cyclization/Desulfonylation Process for the Synthesis of 3-Phosphinoylindoles, Org. Lett., 2016, 18, 1242–1245 CrossRef CAS PubMed
.
- Y.-S. Lin, B. E. Ali and H. Alper, A Novel One-Pot Reaction: Zwitterionic Rhodium Complex-Catalyzed Hydroaminovinylation of Vinyl Sulfones and a Vinylphosphonate, J. Am. Chem. Soc., 2001, 123, 7719–7720 CrossRef CAS PubMed
.
- F. Palacios, S. Pascual, J. Oyarzabal and A. M. Ochoa De Retana, Fluoroalkyl α, β-Unsaturated Imines. Valuable Synthetic Intermediates from Primary Fluorinated Enamine Phosphonates, Org. Lett., 2002, 4, 769–772 CrossRef CAS PubMed
.
-
(a) A. Fadel, F. Legrand, G. Evano and N. Rabasso, Highly Regio- and Stereoselective Nickel-Catalyzed Addition of Dialkyl Phosphites to Ynamides: an Efficient Synthesis of β-Aminovinylphosphonates, Adv. Synth. Catal., 2011, 353, 263–267 CrossRef CAS
;
(b) A. Kinbara, M. Sato, K. Yumita and T. Yamagishi, Copper-Catalyzed Hydrophosphinylation of Terminal Ynamides with H-Phosphinates, Tetrahedron, 2017, 73, 1705–1710 CrossRef CAS
;
(c) H. Huang, H. Zhu and J. Y. Kang, Regio- and Stereoselective Hydrophosphorylation of Ynamides for the Synthesis of β-Aminovinylphosphine Oxides, Org. Lett., 2018, 20, 2778–2781 CrossRef CAS PubMed
;
(d) Z. Peng, Y. Tu, X. Zeng, Z. Zhang and J. Zhao, Regio- and Stereoselective Hydrophosphorylation of Ynamides: A Facile Approach to (Z)-β-Phosphor-Enamides, Adv. Synth. Catal., 2019, 361, 4489–4494 CrossRef CAS
.
-
(a) Y. Liu, Z. Liu, Y. Zhang and C. Xiong, Manganese(III) Acetylacetonate-Mediated Phosphorylation of Enamides at Room Temperature, Adv. Synth. Catal., 2018, 360, 3492–3496 CrossRef CAS
;
(b) S. Pal, A.-C. Gaumont, S. Lakhdar and I. Gillaizeau, Diphenyliodonium Ion/Et3N Promoted Csp2-H Radical Phosphorylation of Enamides, Org. Lett., 2019, 21, 5621–5625 CrossRef CAS PubMed
;
(c) D.-L. Zhang, C.-K. Li, R.-S. Zeng, A. Shoberu and J.-P. Zou, Manganese(III)-mediated Selective Phosphorylation of Enamides: Direct Synthesis of β-Phosphoryl Enamides, Org. Chem. Front., 2019, 6, 236–240 RSC
;
(d) H.-Q. Cao, H.-N. Liu, Z.-Y. Liu, B.-K. Qiao, F.-G. Zhang and J.-A. Ma, Direct Enamido C(sp2)-H Diphosphorylation Enabled by a PCET-Triggered Double Radical Relay: Access to gem-Bisphosphonates, Chem. – Eur. J., 2020, 26, 5515–5521 CrossRef CAS PubMed
;
(e) T. Lei, G. Liang, Y.-Y. Cheng, B. Chen, C.-H. Tung and L.-Z. Wu, Cobaloxime Catalysis for Enamine Phosphorylation with Hydrogen Evolution, Org. Lett., 2020, 22, 5385–5389 CrossRef CAS PubMed
.
-
(a) Z.-H. Yang, H.-R. Tan, Y.-L. An, Y.-W. Zhao, H.-P. Lin and S.-Y. Zhao, Three-Component Coupling Reactions of Maleimides, Thiols, and Amines: One-Step Construction of 3,4-Heteroatom-functionalized Maleimides by Copper-Catalyzed C(sp2)-H Thioamination, Adv. Synth. Catal., 2018, 360, 173–179 CrossRef CAS
;
(b) S. Shi, Y. Ma, J. Zhou, J. Li, L. Chen and G. Wu, Copper-Catalyzed Oxidative Thioamination of Maleimides with Amines and Bunte Salts, Org. Lett., 2020, 22, 1863–1867 CrossRef CAS PubMed
;
(c) Y. Xu, J. Gao, C. Wang, Y. Ma, J. Zhou and G. Wu, Copper-Catalyzed Thioamination of Maleimides with Diethylphosphorodithioate and Amines, Org. Chem. Front., 2021, 8, 3457–3462 RSC
;
(d) W. Zhang, Y. Yao, Y. Xu, X. Zhou and G. Wu, Amine Hydrochloride Salts as Bifunctional Reagents for the Radical Aminochlorination of Maleimides, Org. Chem. Front., 2021, 8, 5766–5770 RSC
.
- C. Zhang and N. Jiao, Copper-Catalyzed Aerobic Oxidative Dehydrogenative Coupling of Anilines Leading to Aromatic Azo Compounds using Dioxygen as an Oxidant, Angew. Chem., Int. Ed., 2010, 49, 6174–6177 CrossRef CAS PubMed
.
-
(a) B. Xiong, R. Shen, M. Goto, S.-F. Yin and L.-B. Han, Highly Selective 1, 4- and 1, 6-Addition of P(O)-H Compounds to p-Quinones: A Divergent Method for the Synthesis of C- and O-Phosphoryl Hydroquinone Derivatives, Chem. – Eur. J., 2012, 18, 16902–16910 CrossRef CAS PubMed
;
(b) H. K. Lenker, M. E. Richard, K. P. Reese, A. F. Carter, J. D. Zawisky, E. F. Winter, T. W. Bergeron, K. S. Guydon and R. A. Stockland, Phospha-Michael Additions to Activated Internal Alkenes: Steric and Electronic Effects, J. Org. Chem., 2012, 77, 1378–1385 CrossRef CAS PubMed
;
(c) J. Han, J. Kim, J. Lee, Y. Kim and S. Y. Lee, Boron Lewis Acid-Catalyzed Hydrophosphinylation of N-Heteroaryl-Substituted Alkenes with Secondary Phosphine Oxides, J. Org. Chem., 2020, 85, 15476–15487 CrossRef CAS PubMed
.
-
(a) G. Wang, Q.-Y. Yu, S.-Y. Chen and X.-Q. Yu, Copper-Catalyzed Aerobic Oxidative Cross-Coupling of Arylamines and Dialkylphosphites Leading to N-Arylphosphoramidates, Tetrahedron Lett., 2013, 54, 6230–6232 CrossRef CAS
;
(b) J. Fraser, L. J. Wilson, R. K. Blundell and C. J. Hayes, Phosphoramidate Synthesis via Copper-Catalysed Aerobic Oxidative Coupling of Amines and H-Phosphonates, Chem. Commun., 2013, 49, 8919–8921 RSC
;
(c) Y. Zhou, J. Yang, T. Chen, S.-F. Yin, D. Han and L.-B. Han, Stereospecific Aerobic Oxidative Dehydrocoupling of P(O)H Bonds with Amines Catalyzed by Copper, Bull. Chem. Soc. Jpn., 2014, 87, 400–402 CrossRef CAS
;
(d) Y. Ou, Y. Huang, Z. He, G. Yu, Y. Huo, X. Li, Y. Gao and Q. Chen, A Phosphoryl Radical-Initiated Atherton-Todd-type Reaction under Open Air, Chem. Commun., 2020, 56, 1357–1360 RSC
.
- Y. Zhou, S. Yin, Y. Gao, Y. Zhao, M. Goto and L.-B. Han, Selective P-P and P-O-P Bond Formations through Copper-Catalyzed Aerobic Oxidative Dehydrogenative Couplings of H-Phosphonates, Angew. Chem., Int. Ed., 2010, 49, 6852–6855 CrossRef CAS PubMed
.
- P.-Z. Zhang, L. Zhang, J.-A. Li, A. Shoberu, J.-P. Zou and W. Zhang, Phosphinoyl Radical Initiated Vicinal Cyanophosphinoylation of Alkenes, Org. Lett., 2017, 19, 5537–5540 CrossRef CAS PubMed
.
-
(a) J. Gu and C. Cai, Cu(I)/Fe(III)-Catalyzed C-P Cross-Coupling of Styrenes with H-Phosphine Oxides: A Facile and Selective Synthesis of Alkenylphosphine Oxides and β-Ketophosphonates, Org. Biomol. Chem., 2017, 15, 4226–4230 RSC
;
(b) G. Zhang, L. Fu, P. Chen, J. Zou and G. Liu, Proton-Coupled Electron Transfer Enables Tandem Radical Relay for Asymmetric Copper-Catalyzed Phosphinoylcyanation of Styrenes, Org. Lett., 2019, 21, 5015–5020 CrossRef CAS PubMed
.
-
(a) Y.-M. Li, M. Sun, H.-L. Wang, Q.-P. Tian and S.-D. Yang, Direct Annulations toward Phosphorylated Oxindoles: Silver-Catalyzed Carbon-Phosphorus Functionalization of Alkenes, Angew. Chem.,
Int. Ed., 2013, 52, 3972–3976 CrossRef CAS PubMed
;
(b) Y. Gao, X. Li, W. Chen, G. Tang and Y. Zhao, Copper-Catalyzed Phosphonation-Annulation Approaches to the Synthesis of β-Phosphonotetrahydrofurans Involving C-P and C-O Bonds Formation, J. Org. Chem., 2015, 80, 11398–11406 CrossRef CAS PubMed
;
(c) W. Liang, K. Jiang, F. Du, J. Yang, L. Shuai, Q. Ouyang, Y.-C. Chen and Y. Wei, Iron-Catalyzed, Iminyl Radical-Triggered Cascade 1,5-Hydrogen Atom Transfer/(5+2) or (5+1) Annulation: Oxime as a Five-Atom Assembling Unit, Angew. Chem., Int. Ed., 2020, 59, 19222–19228 CrossRef CAS PubMed
.
- C. Jing, X. Chen, K. Sun, Y. Yang, T. Chen, Y. Liu, L. Qu, Y. Zhao and B. Yu, Copper-Catalyzed C4-H Regioselective Phosphorylation/Trifluoromethylation of Free 1-Naphthylamines, Org. Lett., 2019, 21, 486–489 CrossRef CAS PubMed
.
-
(a) C.-Y. Wang, R.-J. Song, W.-T. Wei, J.-H. Fan and J.-H. Li, Copper-Catalyzed Oxidative Coupling of Acids with Alkanes Involving Dehydrogenation: Facile Access to Allylic Esters and Alkylalkenes, Chem. Commun., 2015, 51, 2361–2363 RSC
;
(b) X. Tang, W. Wu, W. Zeng and H. Jiang, Copper-Catalyzed Oxidative Carbon-Carbon and/or Carbon-Heteroatom Bond Formation with O2 or Internal Oxidants, Acc. Chem. Res., 2018, 51, 1092–1105 CrossRef CAS PubMed
.
|
This journal is © the Partner Organisations 2022 |