DOI:
10.1039/D1QO01539G
(Highlight)
Org. Chem. Front., 2022,
9, 216-222
Decarbonylative Sonogashira cross-coupling: a fruitful marriage of alkynes with carboxylic acid electrophiles
Received
12th October 2021
, Accepted 17th November 2021
First published on 19th November 2021
Abstract
The Sonogashira cross-coupling is one of the most fundamental C–C bond-forming reactions, wherein the strategic value of an alkyne moiety has found widespread applications at the frontiers of organic chemistry, materials science and drug discovery as the cornerstone building block of chemical synthesis. Although traditional variants of Sonogashira cross-coupling involve aryl halides and pseudohalides as electrophiles, recently, tremendous advances have been made in the unconventional disconnection exploiting common carboxylic acids by a decarbonylation/transmetalation pathway. This manifold (1) permits one to take advantage of carboxylic acids as a ubiquitous class of substrates in organic synthesis that are derived from an orthogonal pool of precursors to aryl halides and pseudohalides and (2) combines the benefits of the palladium-catalyzed C(sp2)–C(sp) coupling of terminal alkynes with the inherent presence of the carboxylic acid moiety in pharmaceuticals, natural products and organic materials. In this highlight article, we summarize the recent progress in the decarbonylative Sonogashira cross-coupling of carboxylic acid electrophiles to produce arylalkynes and conjugated enynes as a novel avenue for chemical synthesis, whereby a large number of chemical reactions critically rely on transformations of alkynes.
Cross-coupling reactions represent the most powerful and most widely utilized class of organic reactions for the synthesis of C–C bonds.1,2 In this respect, the Nobel Prize-winning Suzuki cross-coupling and Heck olefination are predicated on the capacity of preformed organometallic reagents and alkenes as nucleophilic coupling partners (pKa of aliphatic or C(sp2)–H bonds of 43–50). In this avenue, the ability of terminal alkynes to form copper(I) and palladium(II) acetylides by direct deprotonation (pKa of terminal alkynes of 25, Fig. 1) has enabled broadly useful C(sp2)–C(sp) carbon–carbon bond formation3 in a mild, highly predictable and broadly useful manner since its first discovery in 1975 by the groups of Sonogashira, Heck and Cassar.4
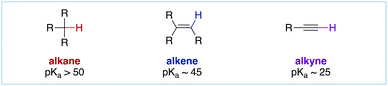 |
| Fig. 1 Acid dissociation constants of C–H bonds. | |
One of the major benefits of the Sonogashira cross-coupling is that alkynes are widely used as fundamental substrates to forge various organic molecules in a plethora of academic and industrial applications in the synthesis of pharmaceuticals, natural products, and functional materials.1–3,5 In addition, the Sonogashira cross-coupling generates substituted arylalkyne and conjugated enyne motifs that on their own represent synthetically important products.3
At present, the most common strategy for the synthesis of arylalkynes relies on transition-metal-catalyzed Sonogashira cross-coupling of aryl halides and pseudohalides as electrophiles.3 The established electrophiles include aryl iodides, bromides and chlorides as well as pseudohalides, such as triflates, tosylates, mesylates, diazonium salts, sulfonium salts, ammonium salts, iodonium salts, phosphonium salts, and carbamates and nitriles employing C–X, C–O, C–N, C–S and C–P cleavage by direct metal insertion into the electrophilic bond (Fig. 2A).6 The catalytic systems typically involve Pd(0)/Cu(I) catalysis and Pd(0) copper-free catalysis.1–6 The participation of copper can effectively accelerate the Sonogashira cross-coupling by forming Cu(I)-acetylides; however, at the same time, the addition of copper has its own shortcomings in that the in situ formed copper acetylides usually tend to lead to the formation of homo-coupling products of terminal alkynes. This side-reaction not only wastes raw starting materials but also creates separation problems in isolating arylalkyne products.
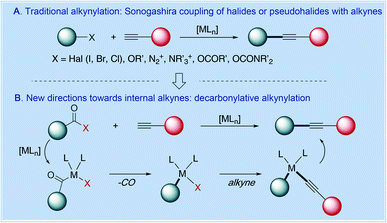 |
| Fig. 2 General methods for the synthesis of arylalkynes. | |
An alternative catalytic system for Sonogashira cross-coupling that has received increased attention is a copper-free variant.1–6 Recent studies suggest that this catalysis involves a tandem Pd/Pd cycle with rate-limiting transmetalation.7 However, these copper-free Sonogashira reactions typically require an excess of bases and tend to be more limited in scope than Cu-co-catalyzed variants, which leads to environmental impacts and reduces the applicability of these methods.
In consideration of the tremendous utility of alkynes as fundamental building blocks in organic synthesis, recent efforts have been focused on the development of new variants of Sonogashira cross-coupling.8 In particular, major advances have been made in the unconventional disconnection exploiting common carboxylic acids as electrophiles in Sonogashira cross-coupling by a decarbonylation/transmetalation pathway in the absence of external oxidants (Fig. 2B). This manifold (1) permits one to take advantage of carboxylic acids as a ubiquitous class of substrates in organic synthesis that are derived from an orthogonal pool of precursors to aryl halides and pseudohalides9,10 and (2) combines the benefits of the palladium-catalyzed C(sp2)–C(sp) coupling of terminal alkynes1–6 with the inherent presence of the carboxylic acid moiety in pharmaceuticals, natural products and organic materials. Furthermore, carboxylic acids as a class of substrates are stable, non-toxic, easy-to-handle solids, and, as needed, easy to be synthesized by various methods. Therefore, because of the above advantages in addition to their ubiquitous presence in late-stage intermediates and orthogonal reactivity, carboxylic acids are often regarded as ideal substrates for organic synthesis and catalysis.9,10
In this article, we summarize the recent progress in the decarbonylative Sonogashira cross-coupling of carboxylic acid electrophiles to produce arylalkynes and conjugated enynes as a novel avenue for chemical synthesis. These reactions employ readily-accessible carboxylic acid derivatives, such as anhydrides (R–C(O)–O2), esters (R–C(O)–OR′) and amides (R–C(O)–NR′R′′), including in situ protocols directly deploying carboxylic acids (R–C(O)–OH), enabling the mild, reagent and substrate controlled oxidative addition of the C(acyl)–X bond (X = OCOR, OR′, NR′R′′) to a transition metal, followed by decarbonylation under redox neutral conditions.
The pioneering study on the decarbonylative cross-coupling of carboxylic acid derivatives with π-systems was reported in 1998 by de Vries and co-workers,11 showing that this cross-coupling could be regarded as a precedent to the decarbonylative manifold of carboxylic acid derivatives (Fig. 3A).12 This Pd-catalyzed methodology achieved the coupling of aromatic carboxylic acid anhydrides with alkenes at 0.25% Pd loading to deliver the corresponding arylalkenes. In 2002, Goossen and co-workers followed up on the study by de Vries and developed a highly selective Pd-catalyzed decarbonylative olefination of aryl esters using a 4-nitro-activating group on the ester component to facilitate oxidative addition (Fig. 3B).13a The same group also reported the Pd-catalyzed decarbonylative olefination of enol esters (Fig. 3C).13b While these early studies set the stage for efficient decarbonylative C–C bond-forming methodologies from esters14 and amides,15 including reactions with π-systems,16 in contrast, the Sonogashira coupling for the synthesis of C(sp2)–C(sp) bonds from carboxylic acid derivatives represented an attractive goal. Compared with the traditional cross-coupling of aryl halides, the advantage of the decarbonylative cross-coupling manifold is that it adopts a well-established two-electron mechanism,17,18 while using ubiquitous carboxylic acids as coupling partners.
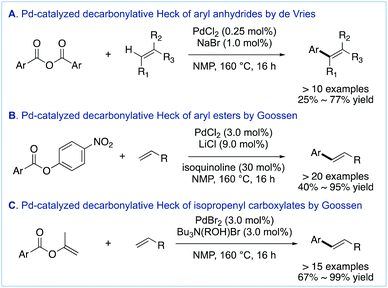 |
| Fig. 3 First examples of Pd-catalyzed decarbonylative cross-coupling of carboxylic acid derivatives with π-systems. | |
In 2017, Yamaguchi and co-workers reported the first study on the decarbonylative Sonogashira coupling of carboxylic acid derivatives using aromatic esters as electrophiles (Fig. 4).19 They established a catalytic system of Pd(acac)2 (5 mol%) and dcypt (10 mol%) as a bidentate thiophene-based phosphine ligand in the presence of CuI (10 mol%) as an effective catalyst. The proposed mechanism involves the oxidative addition of the C(acyl)–O bond to Pd(0), transmetalation with copper(I) acetylide, decarbonylation, and reductive elimination. Intriguingly, the thiophene-based phosphine proved to be significantly more effective than related dcype, indicating that a bite angle might play an important role in promoting decarbonylation in this process.18a Since carboxylic acids are widely present in pharmaceuticals and natural products, and orthogonal cross-couplings with conventional electrophiles are feasible, this first decarbonylative Sonogashira coupling set the stage for new vistas in alkyne synthesis.
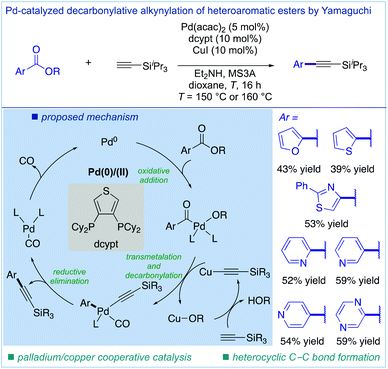 |
| Fig. 4 Pd/Cu-co-catalyzed decarbonylative Sonogashira cross-coupling of esters. | |
Subsequently, Rueping and co-workers developed the decarbonylative Sonogashira cross-coupling of amides using twisted N-acyl-glutarimides introduced by our group as highly effective substrates for this transformation (Fig. 5).20 The reactions were optimally accomplished using a Ni/Cu-co-catalytic system in the presence of Ni(cod)2 (20 mol%), dcype (40 mol%) and CuI (10 mol%). Importantly, the transmetalation step was proposed to occur prior to the decarbonylation step, which is a more established pathway for decarbonylative cross-couplings. Intriguingly, this system also permits the synthesis of conjugated enynes, thus demonstrating for the first time that decarbonylative Sonogashira cross-couplings could be used for the synthesis of more sensitive π-conjugated systems. The method represents a rare example of Ni-catalyzed Sonogashira cross-coupling pioneered by Hu and co-workers.21 The high stability and perpendicular N–C(O) twist of N-acyl-glutarimides22 clearly expedites this challenging coupling.
 |
| Fig. 5 Ni/Cu-catalyzed decarbonylative Sonogashira cross-coupling of amides. | |
In an independent report, Chen and co-workers disclosed a palladium-catalyzed copper-free decarbonylative Sonogashira cross-coupling of amides (Fig. 6).23 As an alternative to the Ni/Cu-catalyzed variant, this method also uses N-acyl-glutarimides as the privileged class of amide N–C(O) electrophiles for the pivotal cross-coupling. The reaction conditions are operationally simple and involve Pd(OAc)2 (3 mol%) and dppp (6 mol%) in the presence of Na2CO3 (1.0 equiv.). It is noteworthy that several other acyclic twisted amides, including N-acyl-glutarimides, N-acyl-succinimides and N-acyl-saccharins, are compatible with this method. Mechanistically, the decarbonylation step was proposed prior to the transmetalation step, and the complete reaction mechanism involves the oxidative addition of the C–N bond, decarbonylation, transmetalation and reductive elimination.
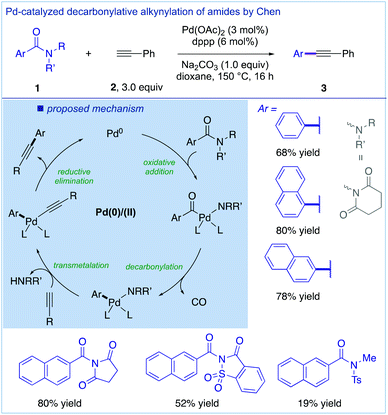 |
| Fig. 6 Pd-catalyzed decarbonylative Sonogashira cross-coupling of amides. | |
Most recently, two independent reports on the direct decarbonylative Sonogashira coupling of carboxylic acids by in situ conversion to the corresponding mixed anhydrides (cf. de Vries, Fig. 3) were published by the Chen group (Fig. 7)24 and our group (Fig. 8).25 These two catalytic systems are complementary, involving Pd2(dba)3 (2.5 mol%) and Xantphos (10 mol%) in the presence of an Ac2O activator (Fig. 7) and Pd(OAc)2 (5 mol%) and Xantphos (10 mol%) in the presence of a piv2O activator (Fig. 8). The most important feature of these complementary methods is that they directly use carboxylic acids as aryl electrophiles; thus prior conversion to the activated carboxylic acid derivatives is not required. This in turn enables one to perform direct synthesis of complex alkynes from pharmaceuticals and natural products. At the same time, the establishment of a copper-free system is intriguing and bodes well for future applications of these methods for the functionalization of complex APIs.
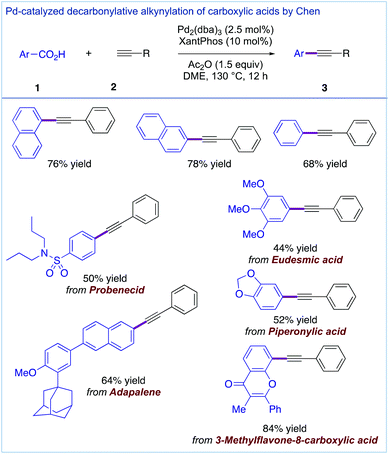 |
| Fig. 7 Decarbonylative Sonogashira cross-coupling of carboxylic acids by Chen. | |
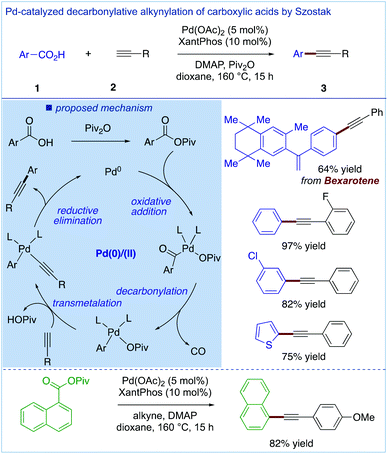 |
| Fig. 8 Pd-catalyzed decarbonylative Sonogashira coupling of carboxylic acids by Szostak. | |
In the realm of decarbonylative Sonogashira cross-coupling reactions, the first catalytic system for employing acid halides was reported by Nishihara and co-workers using aroyl fluorides as electrophiles (Fig. 9).26 The use of less stable acid halides (cf. esters, amides, and anhydrides, Fig. 4–8) requires alkynylsilanes as nucleophiles with PdCl2 (10 mol%), dppp (15 mol%) and CuI (5 mol%) catalytic systems.26a This study represents an important scope extension that is attainable from yet another complementary class of carboxylic acid derivatives. Recently, this aroyl fluoride decarbonylative Sonogashira methodology was advanced to the Ni-catalyzed/copper-free system of Ni(cod)2 (10 mol%) and dppp (15 mol%),26b which permitted the use of simple alkynes with a comparatively more limited scope (not shown).
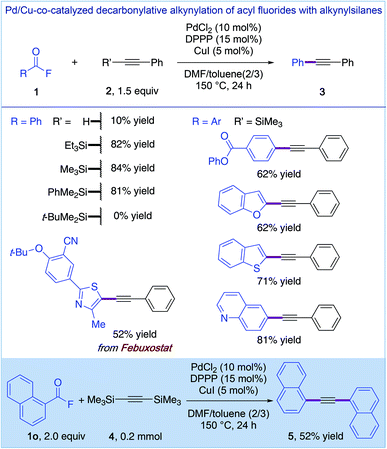 |
| Fig. 9 Pd/Cu-co-catalyzed decarbonylative alkynylation of acyl fluorides with alkynylsilanes by Nishihara. | |
Although the main topic of the present highlight is decarbonylative Sonogashira cross-coupling (loss of CO), a related method for the decarboxylative Sonogashira cross-coupling (loss of CO2) of carboxylic acids via Pd-catalyzed decarboxylative bromination was recently reported by the Su group (Fig. 10).27 The reaction mechanism involves the decarboxylative bromination of carboxylic acids to produce aryl bromides, which then react with terminal alkynes in a conventional Pd(0)/(II) cycle using Pd(PPh3)4 (10 mol%) and PCy3 (20 mol%) in the absence of copper to deliver a range of functionalized diaryl alkynes. This useful methodology bears all the hallmarks of decarboxylative processes,28 requiring ortho-substitution for decarboxylation. This contrasts with decarbonylative processes,12,14–18 which have no or very little limitations in terms of substrate scope.
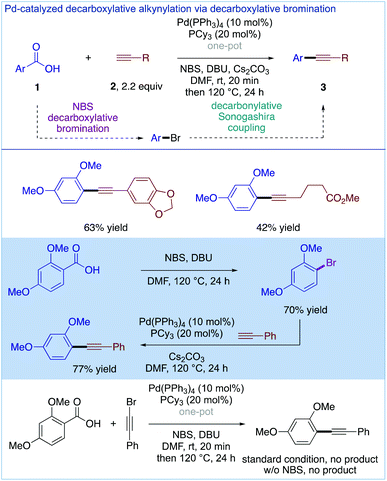 |
| Fig. 10 Pd-catalyzed decarboxylative alkynylation via decarboxylative bromination by Su. | |
Conclusions
In summary, decarbonylative Sonogashira cross-coupling of carboxylic acid derivatives as electrophiles has been accomplished through the oxidative addition of the C(acyl)–X bond and decarbonylation using a broad variety of substrates and catalytic systems. The most important advancement is that carboxylic acids as electrophiles enable the translation of the traditional Sonogashira cross-coupling manifold of aryl halides and pseudohalides to ubiquitous carboxylic acids that are inherently present in complex pharmaceuticals, natural products and organic materials. Thus, the development of decarbonylative Sonogashira cross-coupling merges the potential of diversity in alkyne functionalization with carboxylic acid substrates. Moreover, these studies address several previous major limitations, including (1) addition of acyl electrophiles across π-systems rather than cross-coupling and (2) alkyne dimerization (Glaser–Hay coupling), by inventing new highly reactive yet stable acyl precursors (phenolic esters, N-acyl-glutarimides, mixed anhydrides, and acyl fluorides) together with the precise tuning of catalytic systems (Pd/Cu, Ni/Cu, Pd, and Ni) that permit one to match the rate of oxidative addition and decarbonylation with the class of acyl precursors preventing side reactions. Considering the importance of alkynes and carboxylic acids as fundamental substrates in organic synthesis, many exciting developments are expected in this area.
Conflicts of interest
There are no conflicts to declare.
Acknowledgements
We thank Yantai Nanshan University, Nanjing University of Information Science and Technology, the NIH (1R35GM133326), the NSF (CAREER CHE-1650766), and Rutgers University for generous support.
Notes and references
-
(a)
A. de Meijere, S. Bräse and M. Oestreich, Metal-Catalyzed Cross-Coupling Reactions and More, Wiley, 1st edn, 2014 CrossRef;
(b)
G. A. Molander, J. P. Wolfe and M. Larhed, Science of Synthesis: Cross-Coupling and Heck-Type Reactions, Thieme, 1st edn, 2013 Search PubMed.
-
T. J. Colacot, New Trends in Cross-Coupling, The Royal Society of Chemistry, 1st edn, 2015 Search PubMed.
-
(a) R. Chinchilla and C. Najera, The Sonogashira Reaction: A Booming Methodology in Synthetic Organic Chemistry, Chem. Rev., 2007, 107, 874–922 CrossRef CAS PubMed;
(b) R. Chinchilla and C. Najera, Recent Advances in Sonogashira Reactions, Chem. Soc. Rev., 2011, 40, 5084–5121 RSC;
(c) R. Chinchilla and C. Najera, Chemicals from Alkynes with Palladium Catalysts, Chem. Rev., 2014, 114, 1783–1826 CrossRef CAS PubMed.
-
(a) L. Cassar, Synthesis of Aryl- and Vinyl-Substituted Acetylene Derivatives by the Use of Nickel and Palladium Complexes, J. Organomet. Chem., 1975, 93, 253–257 CrossRef CAS;
(b) H. A. Dieck and F. R. Heck, Palladium Catalyzed Synthesis of Aryl, Heterocyclic and Vinylic Acetylene Derivatives, J. Organomet. Chem., 1975, 93, 259–263 CrossRef CAS;
(c) K. Sonogashira, Y. Tohda and N. Hagihara, A Convenient Synthesis of Acetylenes: Catalytic Substitutions of Acetylenic Hydrogen with Bromoalkenes, Iodoarenes and Bromopyridines, Tetrahedron Lett., 1975, 16, 4467–4470 CrossRef.
-
(a) J. Magano and J. R. Dunetz, Large-Scale Applications of Transition Metal-Catalyzed Couplings for the Synthesis of Pharmaceuticals, Chem. Rev., 2011, 111, 2177–2250 CrossRef CAS;
(b) C. Torborg and M. Beller, Adv. Synth. Catal., 2009, 351, 3027 CrossRef CAS.
- For selected examples, see:
(a) Z. Y. Tian, S. M. Wang, S. J. Jia, H. X. Song and C. P. Zhang, Sonogashira Reaction Using Arylsulfonium Salts as Cross-Coupling Partners, Org. Lett., 2017, 19, 5454–5457 CrossRef CAS PubMed;
(b) X. Pu, H. Li and T. J. Colacot, Heck Alkynylation (Copper-Free Sonogashira Coupling) of Aryl and Heteroaryl Chlorides, Using Pd Complexes of t-Bu2(p-NMe2C6H4)P: Understanding the Structure–Activity Relationships and Copper Effects, J. Org. Chem., 2013, 78, 568–581 CrossRef CAS;
(c) G. Fabrizi, A. Goggiamani, A. Sferrazza and S. Cacchi, Sonogashira Cross-Coupling of Arenediazonium Salts, Angew. Chem., Int. Ed., 2010, 49, 4067–4070 CrossRef CAS PubMed.
- M. Gazvoda, M. Virant, B. Pinter and J. Košmrlj, Mechanism of copper-free Sonogashira reaction operates through palladium-palladium transmetalation, Nat. Commun., 2018, 9, 4814 CrossRef.
- For studies on C–H alkynylation and alkynylation through ligand design, see:
(a) J. He, M. Wasa, K. S. L. Chan and J. Q. Yu, Palladium(0)-Catalyzed Alkynylation of C(sp3)–H Bonds, J. Am. Chem. Soc., 2013, 135, 3387–3390 CrossRef CAS PubMed;
(b) M. Eckhardt and G. C. Fu, The First Applications of Carbene Ligands in Cross-Couplings of Alkyl Electrophiles: Sonogashira Reactions of Unactivated Alkyl Bromides and Iodides, J. Am. Chem. Soc., 2003, 125, 13642–13643 CrossRef CAS PubMed.
- For reviews on the use of carboxylic acids as substrates in catalysis, see:
(a) L. J. Gooßen, N. Rodriguez and K. Gooßen, Carboxylic Acids as Substrates in Homogeneous Catalysis, Angew. Chem., Int. Ed., 2008, 47, 3100–3120 CrossRef;
(b) N. Rodriguez and L. J. Gooßen, Decarboxylative Coupling Reactions: a Modern Strategy for C–C-Bond Formation, Chem. Soc. Rev., 2011, 40, 5030–5048 RSC.
-
(a) L. J. Gooßen, G. Deng and L. M. Levy, Synthesis of Biaryls via Catalytic Decarboxylative Coupling, Science, 2006, 313, 662–664 CrossRef PubMed;
(b) J. Twilton, C. C. Le, P. Zhang, M. H. Shaw, R. W. Evans and D. W. C. MacMillan, The merger of transition metal and photocatalysis, Nat. Rev. Chem., 2017, 1, 52 CrossRef CAS.
- M. S. Stephan, A. J. J. M. Teunissen, G. K. M. Verzijl and J. G. de Vries, Heck Reactions without Salt Formation: Aromatic Carboxylic Anhydrides as Arylating Agents, Angew. Chem., Int. Ed., 1998, 37, 662–664 CrossRef CAS PubMed.
- H. Lu, T. Y. Yu, P. F. Xu and H. Wei, Selective Decarbonylation via Transition-Metal-Catalyzed Carbon–Carbon Bond Cleavage, Chem. Rev., 2021, 121, 365–411 CrossRef CAS PubMed.
-
(a) L. J. Gooßen and J. Paetzold, Pd-Catalyzed Decarbonylative Olefination of Aryl Esters: Towards a Waste-Free Heck Reaction, Angew. Chem., Int. Ed., 2002, 41, 1237–1241 CrossRef;
(b) L. J. Gooßen and J. Paetzold, Decarbonylative Heck Olefination of Enol Esters: Salt-Free and Environmentally Friendly Access to Vinyl Arenes, Angew. Chem., Int. Ed., 2004, 43, 1095–1098 CrossRef.
- For reviews on C–O activation in esters, see:
(a) R. Takise, K. Muto and J. Yamaguchi, Cross-Coupling of Aromatic Esters and amides, Chem. Soc. Rev., 2017, 46, 5864–5888 RSC;
(b) L. Guo and M. Rueping, Decarbonylative Cross-Couplings: Nickel Catalyzed Functional Group Interconversion Strategies for the Construction of Complex Organic Molecules, Acc. Chem. Res., 2018, 51, 1185–1195 CrossRef CAS PubMed.
- For reviews on C–N activation in amides, see:
(a) C. Liu and M. Szostak, Twisted Amides: From Obscurity to Broadly Useful Transition-Metal-Catalyzed Reactions by N–C Amide Bond Activation, Chem. – Eur. J., 2017, 23, 7157–7173 CrossRef CAS PubMed;
(b) C. Liu and M. Szostak, Decarbonylative Cross-Coupling of Amides, Org. Biomol. Chem., 2018, 16, 7998–8010 RSC;
(c) G. Li, S. Ma and M. Szostak, Amide Bond Activation: The Power of Resonance, Trends Chem., 2020, 2, 914–928 CrossRef CAS.
-
(a) G. Meng and M. Szostak, General Olefin Synthesis by the Palladium-Catalyzed Heck Reaction of Amides: Sterically Controlled Chemoselective N–C Activation, Angew. Chem., Int. Ed., 2015, 54, 14518–14522 CrossRef CAS PubMed;
(b) C. Liu, G. Meng and M. Szostak, N-Acylsaccharins as Amide-Based Arylating Reagents via Chemoselective N–C Cleavage: Pd-Catalyzed Decarbonylative Heck Reaction, J. Org. Chem., 2016, 81, 12023–12030 CrossRef CAS PubMed.
- For representative decarbonylative cross-couplings of esters and amides, see:
(a) K. Muto, J. Yamaguchi, D. G. Musaev and K. Itami, Decarbonylative Organoboron Cross-Coupling of Esters by Nickel Catalysis, Nat. Commun., 2015, 6, 7508 CrossRef PubMed;
(b) L. Guo, A. Chatupheeraphat and M. Rueping, Decarbonylative Silylation of Esters by Combined Nickel and Copper Catalysis for the Synthesis of Arylsilanes and Heteroarylsilanes, Angew. Chem., Int. Ed., 2016, 55, 11810–11813 CrossRef CAS PubMed;
(c) H. Yue, L. Guo, S. C. Lee, X. Liu and M. Rueping, Selective Reductive Removal of Ester and Amide Groups from Arenes and Heteroarenes through Nickel-Catalyzed C–O and C–N Bond Activation, Angew. Chem., Int. Ed., 2017, 56, 3972–3976 CrossRef CAS PubMed;
(d) H. Yue, L. Guo, H. H. Liao, Y. Cai, C. Zhu and M. Rueping, Catalytic Ester and Amide to Amine Interconversion: Nickel-Catalyzed Decarbonylative Amination of Esters and Amides by C–O and C–C Bond Activation, Angew. Chem., Int. Ed., 2017, 56, 4282–4285 CrossRef CAS;
(e) R. Takise, R. Isshiki, K. Muto, K. Itami and J. Yamaguchi, Decarbonylative Diaryl Ether Synthesis by Pd and Ni Catalysis, J. Am. Chem. Soc., 2017, 139, 3340–3343 CrossRef CAS PubMed;
(f) C. A. Malapit, M. Borrell, M. W. Milbauer, C. E. Brigham and M. S. Sanford, Nickel-Catalyzed Decarbonylative Amination of Carboxylic Acid Esters, J. Am. Chem. Soc., 2020, 142, 5918–5923 CrossRef CAS PubMed;
(g) S. Shi, G. Meng and M. Szostak, Synthesis of Biaryls through Nickel-Catalyzed Suzuki–Miyaura Coupling of Amides by Carbon–Nitrogen Bond Cleavage, Angew. Chem., Int. Ed., 2016, 55, 6959–6963 CrossRef CAS PubMed;
(h) C. Liu and M. Szostak, Decarbonylative Phosphorylation of Amides by Palladium and Nickel Catalysis: The Hirao Cross-Coupling of Amide Derivatives, Angew. Chem., Int. Ed., 2017, 56, 12718–12722 CrossRef CAS PubMed.
- For representative computational studies on decarbonylative couplings, see:
(a) C. Liu, C. L. Ji, X. Hong and M. Szostak, Palladium-Catalyzed Decarbonylative Borylation of Carboxylic Acids: Tuning Reaction Selectivity by Computation, Angew. Chem., Int. Ed., 2018, 57, 16721–16726 CrossRef CAS PubMed;
(b) C. Liu, C. L. Ji, T. Zhou, X. Hong and M. Szostak, Bimetallic Cooperative Catalysis for Decarbonylative Heteroarylation of Carboxylic Acids via C-O/C-H Coupling, Angew. Chem., Int. Ed., 2021, 60, 10690–10699 CrossRef CAS PubMed;
(c) C. Liu, Z. X. Qin, C. L. Ji, X. Hong and M. Szostak, Highly-Chemoselective Step-Down Reduction of Carboxylic Acids to Aromatic Hydrocarbons via Palladium Catalysis, Chem. Sci., 2019, 10, 5736–5742 RSC.
- T. Okita, K. Kumazawa, R. Takise, K. Muto, K. Itami and J. Yamaguchi, Palladium-Catalyzed Decarbonylative Alkynylation of Aromatic Esters, Chem. Lett., 2017, 46, 218–220 CrossRef CAS.
- W. Srimontree, A. Chatupheeraphat, H. H. Liao and M. Rueping, Amide to Alkyne Interconversion via a Nickel/Copper-Catalyzed Deamidative Cross-Coupling of Aryl and Alkenyl Amides, Org. Lett., 2017, 19, 3091–3094 CrossRef CAS PubMed.
-
(a) O. Vechorkin, D. Barmaz, V. Proust and X. Hu, Ni-Catalyzed Sonogashira Coupling of Nonactivated Alkyl Halides: Orthogonal Functionalization of Alkyl Iodides, Bromides, and Chlorides, J. Am. Chem. Soc., 2009, 131, 12078–12079 CrossRef CAS PubMed;
(b) Q. Fan, H. Sun, S. Xie, Y. Dong, X. Li, O. Fuhr and D. Fenske, Nickel-Catalyzed Sonogashira Coupling Reactions of Nonactivated Alkyl Chlorides under Mild Conditions, Organometallics, 2021, 40, 2240–2245 CrossRef CAS.
-
(a) G. Meng, J. Zhang and M. Szostak, Acyclic Twisted Amides, Chem. Rev., 2021, 121, 12746–12783 CrossRef CAS PubMed;
(b) G. Meng and M. Szostak, N-Acyl-Glutarimides: Privileged Scaffolds in Amide N–C Cross-Coupling, Eur. J. Org. Chem., 2018, 2352–2365 CrossRef CAS.
- L. Liu, D. Zhou, M. Liu, Y. Zhou and T. Chen, Palladium-Catalyzed Decarbonylative Alkynylation of Amides, Org. Lett., 2018, 20, 2741–2744 CrossRef CAS PubMed.
- X. Li, L. Liu, T. Huang, Z. Tang, C. Li, W. Li, T. Zhang, Z. Li and T. Chen, Palladium-Catalyzed Decarbonylative Sonogashira Coupling of Terminal Alkynes with Carboxylic Acids, Org. Lett., 2021, 23, 3304–3309 CrossRef CAS PubMed.
- C. Liu and M. Szostak, Decarbonylative Sonogashira Cross-Coupling of Carboxylic Acids, Org. Lett., 2021, 23, 4726–4730 CrossRef CAS PubMed.
-
(a) Q. Chen, L. Fu and Y. Nishihara, Palladium/copper-cocatalyzed decarbonylative alkynylation of acyl fluorides with alkynylsilanes: synthesis of unsymmetrical diarylethynes, Chem. Commun., 2020, 56, 7977–7980 RSC;
(b) Q. Chen, L. Fu, J. You and Y. Nishihara, Nickel-Catalyzed Decarbonylative Alkynylation of Acyl Fluorides with Terminal Alkynes under Copper-Free Conditions, Synlett, 2021, 32, 1560–1564 CrossRef CAS.
- Q. Jiang, H. Li, X. Zhang, B. Xu and W. Su, Pd-Catalyzed Decarboxylative Sonogashira Reaction via Decarboxylative Bromination, Org. Lett., 2018, 20, 2424–2427 CrossRef CAS.
- Y. Wei, P. Hu, M. Zhang and W. Su, Metal-Catalyzed Decarboxylative C–H Functionalization, Chem. Rev., 2017, 117, 8864–8907 CrossRef CAS PubMed.
|
This journal is © the Partner Organisations 2022 |
Click here to see how this site uses Cookies. View our privacy policy here.