DOI:
10.1039/D2QM00542E
(Research Article)
Mater. Chem. Front., 2022,
6, 2491-2498
Naphthaldehyde-based Schiff base dyes: aggregation-induced emission and high-contrast reversible mechanochromic luminescence†
Received
7th June 2022
, Accepted 7th July 2022
First published on 8th July 2022
Abstract
Mechanochromic luminescent materials have attracted intense attention because of their excellent reversible optical behaviour, but the mechanism of the stimuli responsive behaviour remains ambiguous. Herein, a novel naphthaldehyde-based Schiff base, namely N2,N6-bis(2-(2-hydroxynaphthalen-1-yl)phenyl)pyridine-2,6-dicarboxamide (HNP) exhibiting both aggregation enhanced emission (AIEE) and excited state intramolecular proton transfer (ESIPT) characteristics has been synthesized. The mechanism of the reversible mechanochromism was investigated via experimental and theoretical procedures. Crystalline HNP exhibits a reversible mechanochromism from yellow to orange emission under external stimulus and back to yellow emission by fuming under a dichloromethane atmosphere. Single crystal X-ray diffraction analysis indicated that the compound HNP adopts two types of molecular conformations with different intramolecular torsion angles. Meanwhile, DFT and TD-DFT calculations demonstrated that the torsion angle change directly affects the electronic distribution, resulting in a discriminable energy gap. Clearly, the different molecular conformations play a crucial role in triggering the reversible mechanochromism upon grinding. Additionally, HNP is an excellent fluorescent probe for detecting Cu(II) ions in solution. Thus, this example not only provides clear evidence to clarify the mechanism of reversible mechanochromism, but also offers a new on-off chemosensor for Cu(II) ion detection.
1. Introduction
Mechanochromic luminescent (MCL) materials are a classical type of multifunctional material that can change emission colour reversibly under external stimulus, and these materials exhibit high efficiency toward diverse applications in sensors,1 information anti-counterfeiting,2 memory chips and optoelectronic devices.3 Organic materials with mechanochromic luminescence properties show unique advantages4 such as low cost, precise molecular structures with tunable optical properties. Moreover, molecular components with a twisted conformation have been utilized for constructing MCL materials, such as tetraphenylethylene,5 cyanostyrene,6 carbazole,7etc.
Aggregation-induced emission (AIE), is an abnormal fluorescence phenomenon, where a type of molecule is weakly fluorescent in solution but exhibits high fluorescence in the aggregated state.8 Organic luminescent materials with the AIE characteristic have shown great potential in organic light-emitting diodes,9 photodynamic therapy,10 fluorescent probes,11etc. Excited-state intramolecular proton transfer (ESIPT) is a classical fluorescence process, where the proton can transfer from a hydrogen bond donor (e.g. –OH and –NH2) to a hydrogen bond receptor (e.g. = N– and C
O)12via a tautomeric transformation under irradiation, resulting in a large red-shifted emission. Specifically, the combination of the AIE and ESIPT features can be used to overcome environmental sensitivity to avoid self-absorption.13
Schiff base compounds have wide versatility and exhibit good solubility in common solvents, in addition to being easily functionalized when needed for various applications. Previously, our group reported that the tetraphenylethylene functionalized salicylaldehyde Schiff-base with the aggregation-induced enhanced emission (AIEE) property is an excellent fluorescent probe for Cu(II) ion detection14 and we note that a naphthyl-based Schiff base zinc complex has been used for cellular uptake studies.15 Moreover, the coordination mode of a salicylaldehyde Schiff-base fluorescent probe toward Cu(II) was evaluated by single crystal X-ray diffraction.16 In recent years, many Schiff base MCL compounds have been reported17 and proved to be excellent fluorescence carriers with high-contrast reversible mechanochromic luminescence properties.18 Generally, photoluminescence spectra, scan/transmission electron microscopy, and powder X-ray-diffraction are the typical techniques employed for understanding the mechanochromic mechanism. However, understanding the intrinsic mechanism of MCL behaviour remains an enormous challenge.
In this work, a Schiff base compound HNP was synthesized via a facile one-step synthetic method, which exhibits typical AIEE plus ESIPT characteristics. Due to the presence of the flexible Schiff base skeleton, the AIEE-active molecule HNP adopts a twisted molecular conformation via several strong intra/inter-molecular interactions, which leads to different emissions in the crystalline state. More interestingly, this crystal displays high-contrast reversible mechanochromic luminescence properties via orange and yellow emission, and this result is ascribed to the different molecular conformations in the crystal packing. The relationship between the molecular packing and the emission properties is discussed to understand in greater depth the reversible mechanochromic mechanism, via single crystal X-ray diffraction, SEM, and photoluminescence spectroscopy, as well as theoretical calculations. Moreover, Schiff base HNP can act as a ‘turn-off’ fluorescence sensor for potential applications in Cu2+ detection.
2. Results and discussion
2.1 Synthesis
The synthetic route to Schiff base N2,N6-bis(2-(2-hydroxynaphthalen-1-yl)phenyl)pyridine-2,6-dicarboxamide (HNP) is presented in Scheme 1. The intermediate N,N′-(2-aminophenyl)-2,6-dicarboxylimide pyridine (1) was synthesized according to a previous report.14 Treatment of 1 with 2-hydroxy-1-naphthaldehyde dissolved in methanol afforded the target red compound HNP in 78% yield. Interestingly, a yellow powder was obtained when more than 0.3 equivalents of H2SO4 catalyst was added to the reaction, which shows the same characterization data for the 1H/13C NMR spectroscopy (Fig. S1 and S2, ESI†) and high-resolution mass spectrometry (HRMS) (Fig. S3, ESI†) as compound HNP.
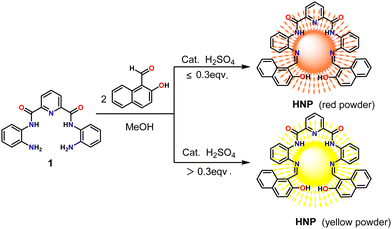 |
| Scheme 1 Synthesis of compound HNP. | |
2.2 Photophysical properties
The UV-vis absorption spectra and photoluminescence (PL) spectra of compound HNP were measured in seven solutions (CH3OH, EtOH, CHCl3, THF, DCM, DMSO and DMF) and are shown in Fig. S4 (ESI†). The absorption band at λ = 319 nm represents the electronic transition of the Schiff base unit,19 and the absorption peak at λ = 396 nm is tentatively assigned to the electron–electron transition from the Schiff base to the amide–pyridine ring, which is greatly affected by the polarity of the solvent.20 Meanwhile, the wide absorption wavelength range from 419 nm to 475 nm is due to the formation of intramolecular hydrogen bonds.21 In addition, the compound HNP exhibits weak fluorescence in all solvents, but the fluorescence intensity is stronger in weak polarity solvents (such as CHCl3, THF and DCM) than in strong polarity solvents (CH3OH, EtOH, DMF and DMSO). Moreover, the compound exhibits a red-shifted emission in the protic solvents methanol and ethanol as compared to DMSO, and this may be ascribed to the presence of the —OH group which can stabilize the enol conformation and lead to the adoption of a twisted intramolecular charge transfer (TICT) process.22
2.3 Aggregation-induced emission properties
To understand the effect of water on the emission process, the UV-vis spectra and photoluminescence (PL) spectra characteristics were studied in a mixture of THF/H2O with different water fractions (fw). As shown in Fig. S5, (ESI†) the peak at 419–475 nm gradually decreases, which may be attributed to the blocking of the ESIPT by the intermolecular H-bonding between the HNP and H2O.23 On the other hand, as shown in Fig. 1, a dual emission in THF was observed under excitation (λex = 350 nm), the short-wavelength emission originating from enol emission and the latter from keto emission.22 Moreover, the shorter-wavelength emission decreased gradually with the increase of water content, and eventually disappeared when the water fraction (fw) increased to more than 80%. This is attributed to molecular aggregation, resulting in strengthened intramolecular H-bonding, which is a key factor for an efficient ESIPT process.12 Furthermore, HNP emits weak orange emission with a maximum emission peak at 588 nm, as the water fraction (fw) increased from 0% and 70%, and the emission intensity gradually decreased with the increase of water content. This is consistent with the UV-vis spectra, which show that the fluorescence intensity is reduced. As the water fraction (fw) increased from 70% to 95%, the emission intensity was enhanced ca. 3.5-fold, indicating that HNP exhibits a clear aggregation-induced enhanced emission (AIEE). To further investigate the aggregation-induced emission (AIE) properties of HNP, the emission spectra were recorded with a different excitation (λex = 440 nm) and higher concentration (5.00 × 10−5 mol L−1) in THF solution using different THF/water mixtures. The results revealed almost the same trend, where the overall fluorescence intensity was enhanced (Fig. S6, ESI†). Moreover, the stability of the probe HNP at various pH values was examined in the THF/water (VTHF/Vwater = 1/9, Tris-HCl buffer) system (Fig. S7, ESI†). With the changing pH, the absorbance of HNP gradually decreases as the pH value increases from 1.00 to 3.00, while the absorbance exhibits a slight change over the pH range from 5.00 to 12.00. On the other hand, the fluorescence intensity remains unchanged in the pH range from 1.00 to 12.00. This result indicated that the Schiff-based compound HNP was stable at various pH values from 2.00 to 12.00.
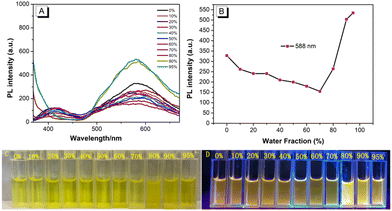 |
| Fig. 1 (A) Fluorescence spectra of the fluorescent probe (2.00 × 10−5 mol L−1) at different water contents (λex = 350 nm) in THF. (B) The effect of water volume fraction on the fluorescence probe at 588 nm. (C) Photographs of the fluorescent probes in THF solution containing different water components under natural light. (D) Photographs of the fluorescent probes in THF solution containing different water components under 365 nm UV light. | |
2.4 Mechanochromism properties
As we know, most AIE luminogens exhibit excellent reversible mechanochromism properties under external stimulation. Considering the flexibility of the molecular structure of HNP, the reversible mechanochromism behaviour was studied. The yellow compound HNP displays a bright orange emission with a maximum emission peak at λem = 540 nm, (Φf = 5.44%). Once the yellow compound HNP was ground, the powder emitted a large, red-shifted emission with λmaxem = 662 nm (Φf = 1.55%), and the fluorescence lifetime (τ) changed from 5.35 ns (HNP-y) to 1.81 ns. Moreover, the red emission powder could recover the yellow emission under a CH2Cl2 atmosphere, indicating that HNP displays reversible mechanical stimuli-responsive properties. Furthermore, the samples of HNP before and after grinding were evaluated by powder X-ray diffraction. As shown in Fig. 2, the crystal sample HNP-y exhibits sharp intensity peaks, indicative of a good microcrystalline structure, while powdered HNP-r displays weak and broad diffraction peaks, and the peaks at 2θ = 12° and 16° almost disappeared after grinding, indicating that the molecular arrangement displays a large change with a red-shifted emission. Moreover, the diffraction peak of HNP-r can return back to a sharp peak via CH2Cl2 fuming, and the emission colour changes from red to orange, and this process agrees with their optical behaviour. Additionally, the morphology of the samples HNP-y and HNP-r was also investigated by scanning electron microscopy (SEM). As shown in Fig. 2c, crystalline HNP-y exhibits a block 3D aggregate structure, while powdered HNP-r shows a 2D fibrous-like structure along the same direction (Fig. 2d), indicating that HNP show different morphology before and after grinding, which was further characterized by the UV-Vis absorption spectra and is illustrated in Fig. S8 (ESI†). After fuming with CH2Cl2, the UV-vis absorption of the samples is almost overlapped with the crystalline HNP-y.
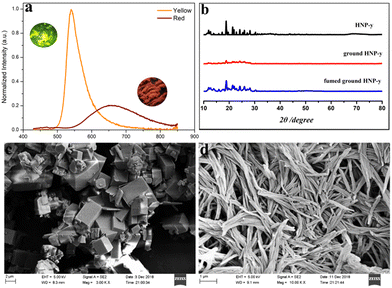 |
| Fig. 2 (a) Fluorescence spectra of powdered HNP-y (orange line) and powdered HNP-r (red line). (b) PXRD patterns of crystals of HNP-y and HNP-r and when ground and after fuming with CH2Cl2. SEM image of HNP powder under different fluorescent emissions (c) yellow and (d) red. | |
2.5 Single crystal structure analysis
Single crystal X-ray diffraction is an efficient tool to visualize the molecular conformation and for understanding the relationship between the molecular packing and the optical behaviour. Thus, we attempted to cultivate crystals of HNP by slow solvent evaporation at room temperature. Firstly, pure HNP (20 mg) was dissolved in a chloroform/ethanol mixture to obtain block red crystals (HNP-r); yield 12 mg. Then, the mother liquor was collected to afford yellow needle crystals (HNP-y) (yield 6 mg). Both molecular structures of the crystals HNP-y and HNP-r were confirmed by single crystal X-ray diffraction analysis, and the key crystal data are summarized in Table S1 (ESI†). The HNP-y crystal belongs to the triclinic crystal system with the space group P
, and the asymmetric unit cell of HNP-y contains two molecules. Meanwhile, the crystal HNP-r crystallises in the higher symmetrical monoclinic system with the C2/c space group, and each asymmetric unit contains four molecules. The ORTEP diagrams of HNP-y and HNP-r are shown in Fig. S9 (ESI†).
As shown in Fig. 3, the two terminal naphthyl rings in the crystal HNP-y adopt an intersecting type conformation, whereas the situation observed in HMP-r is almost coplanar. The difference is that one side of the planar group is almost perpendicular to another side plane with a dihedral angle of 84.78° in crystal HNP-y. Two pairs of intramolecular hydrogen bond interactions (O2–H2B⋯N3 and O4–H4B⋯N5) are formed between the hydroxyl and imino groups at distances of 1.776 Å and 1.794 Å, respectively, which are shorter than our previous reported tetraphenylethylene functionalised salicylaldehyde Schiff-base (1.882 Å and 1.858 Å),14 and other intramolecular hydrogen bonds (N–H⋯O and N–H⋯O), e.g. 2.051 Å and 2.086 Å, respectively,16 which indicated that the intramolecular hydrogen bond is stronger here. For crystal HNP-r, the two planar groups are almost parallel, and the dihedral angle is 4.86°, and the the distance between the centres of the terminal naphthyl rings is in the range 4.488–4.894 Å, suggesting that HNP-r prefers to form π–π stacking. Also, intramolecular hydrogen bonding (O–H⋯N) is observed at a distance of 1.822 Å. The intramolecular hydrogen bonds (N2–H2A⋯O2′) are both 2.498 Å long.
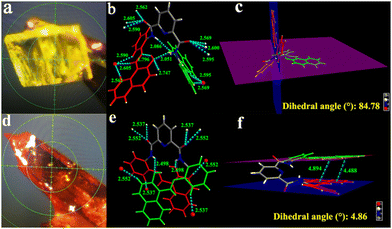 |
| Fig. 3 (a) Photographs of HNP-y. (b) Hydrogen bonding in HNP-y. (c) Dihedral angle of the HNP-y crystal. (d) Photographs of HNP-r. (e) Hydrogen bonding in HNP-r. (f) Dihedral angle of the HNP-r crystal. | |
In the packing of the structure, the HNP-y molecules are connected by intermolecular π–π interactions and multiple C–H⋯O hydrogen bonds between adjacent molecules. Furthermore, intermolecular π⋯π interactions are observed between two naphthyl rings and two adjacent molecules at a distance of 3.35 Å and 3.36 Å. Multiple intermolecular bonds C–H⋯O interactions were formed between the carbonyl oxygen atoms with the phenyl ring or the naphthyl ring of the adjacent molecules. These intermolecular forces enable the HNP-y molecules to adopt a parallel arrangement forming columns overlapping along the a-axis (Fig. 4a), and the adjacent molecules in the adjacent columns overlap with each other with anti-parallel π-overlap (Fig. 4b).
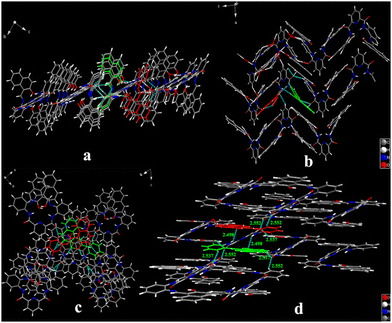 |
| Fig. 4 (a) Molecular packing structures, intermolecular interactions in the ordered structure of the HNP-y crystal. (b) Two adjacent dimers and intermolecular interactions of the HNP-y crystal. (c) Molecular packing structures, intermolecular interactions in the ordered structure of the HNP-r crystal. (d) Two adjacent dimers and intermolecular interactions of the HNP-r crystal. The blue dotted lines indicate intermolecular interactions. | |
On the other hand, for the HNP-r crystals, the adjacent molecules are connected by π⋯π stacking between the phenyl and pyridine rings (≈ 3.38 Å), thereby forming a three-dimensional structure (Fig. 4c). Each of the adjacent molecules are arranged in an anti-parallel direction along the c-axis (Fig. 4d). Infinite stacking was also observed in each column, but compared with HNP-y crystals, intermolecular overlap was smaller and the arrangement was less orderly.
Moreover, the simulated X-ray diffraction (XRD) patterns of HNP-y and HNP-r crystals totally coincided with that of HNP-y and HNP-r crystalline powders, respectively, suggesting the same molecular packing modes in them. However, compared to the powder X-ray diffraction data, the HNP-y crystals and HNP-r crystals show different diffraction peaks, indicated that both crystals HNP-y and HNP-r arrange in different stacking modes (Fig. S10, ESI†). Indeed, the crystals HNP-y and HNP-r emit a yellow and red emission with maximum emission peak at 540 nm and 662 nm, respectively. This result is also consistent with the mechanochromism behaviour.
2.6 Theoretical calculations
In order to explore the electronic structures of the HNP-y and HNP-r molecules, density functional theory (DFT) and time-dependent DFT (TD-DFT) calculations were performed using the B3LYP/6-31G(d) basis set. As shown in Fig. 5, the HOMO and LUMO levels of HNP-y are almost spread over the whole molecular framework, while the HOMO level of HNP-r is mainly localized on the phenyl and naphthyl parts, while the LUMO level is distributed on the naphthyl fragments. Moreover, the energy gap (Eg) of HNP-r (3.59 eV) is higher than HNP-r (3.44 eV). TD-DFT calculations indicating that the main absorption for HNP-r is assigned to the S1–S0 transition from HOMO to LUMO, in which the HOMO → LUMO contribution in HNP-r is 91.1%, and the HOMO → LUMO contribution in HNP-y is only 60.0%.24 Thus, the molecular conformation has a significant influence on the excited state properties and on the oscillator strength (ƒ) of the S1–S0 transition.22 Based on the results of the calculations and the molecular structure, the dihedral angle of the two naphthyl groups decreases, and the ƒ value reduces from 0.1499 to 0.1273, suggesting that a decreased twist of the dihedral angle results in a red-shifted emission with weaker emission. Therefore, it would be a useful strategy to change the ability of the electron donor or electron acceptor to regulate the charge transfer efficiency in order to achieve a high fluorescence yield in this type of luminescence material.
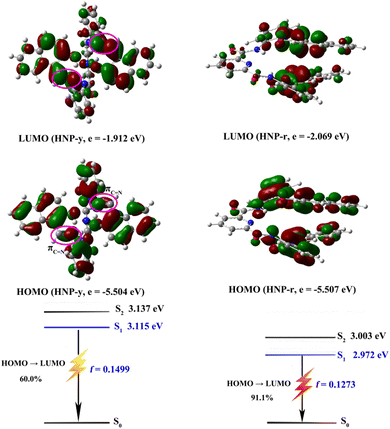 |
| Fig. 5 The frontier orbitals and theoretically calculated vertical excitation energies, transition natures of the excited states and oscillator strengths for HNP-y and HNP-r. | |
2.7 Fluorogenic Cu2+ sensing
In order to evaluate the sensing properties of HNP, its sensing properties in THF with several metal cations (solutions of Hg+, Cr3+ and Mg2+ and Zn2+ were prepared from their chloride salts; solutions of Cu2+, Cd2+, Pb2+, Ca2+, Co2+, Ba2+, Ag+, Ni2+, Li+, K+, Na+, Al3+, Zn2+ and Fe3+ were prepared from their nitrate salts) were investigated by UV-vis absorption and fluorescence measurements (Fig. 6). The absorption spectra of HNP showed that the absorption peak at 396 nm remained unchanged upon addition of several kinds of metal ions except in the case of Cu2+ and Co2+. Upon addition of Cu2+, the absorbance located at 396 nm was red shifted to 443 nm. Except for Co2+, other metal ions had little effect on the absorption. The fluorescence emission at 588 nm upon excitation by 440 nm was quenched upon addition of Cu2+, while other metal cations had little effect on the fluorescence intensity of the probe. Moreover, competition experiments were conducted, and as shown in Fig. S11 (ESI†), other metal ions did not interfere with the recognition of HNP toward Cu2+, with the exception of Co2+ which had a slight effect on the probe detection of Cu2+, indicating that the probe had strong anti-interference properties. The results of UV-Vis absorption spectra and fluorescence spectra show that the probe has high specificity toward copper ions. Moreover, fluorescence titration experiments of Cu2+ were carried out in THF. As shown in Fig. S12 (ESI†), the absorbance of the probe at 396 nm gradually decreased with the increase of Cu2+ concentration; meanwhile, a new absorbance at 443 nm gradually increased. Moreover, the fluorescence intensity of the probe decreased gradually at 588 nm, and the fluorescence quenching rate reached 95.35% when two equivalents of Cu2+ were added. Additionally, the combination ratio between them was further determined by the equal mole continuous transformation method (Job's plot). As shown in Fig. S13 (ESI†), through the fluorescence spectrum, it can be seen that probe/probe + Cu2+ has an inflection point at 0.33, indicating that the mixing ratio between probe and Cu2+ is 1
:
2.
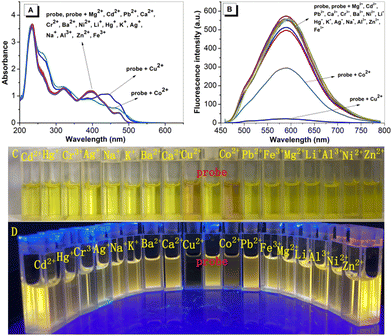 |
| Fig. 6 (A) UV-vis absorption spectra and (B) fluorescence spectra of fluorescent probes (5.00 × 10−5 mol L−1) interacting with different metal ions (1.00 × 10−4 mol L−1) in THF solution (λex = 440 nm); (C) photographs of the probes interacting with metal ions under natural light; (D) photographs of the probes interacting with metal ions under 365 nm UV illumination. | |
Furthermore, the detection limit of probe HNP for Cu2+ was calculated to be 1.8 × 10−7 mol L−1 from the fluorescence titration experiments following the IUPAC method (Fig. S14, ESI†).25 On the basis of the 2
:
1 stoichiometry, the association constant between HNP and Cu2+ was estimated to be 5.70 × 103 mol L−1 by using the Benesi–Hildebrand equation (Fig. S15, ESI†).26
The crystal structure is important for us to understand the coordination mode and molecular packing. Fortunately, single crystals of complex HNP-Cu were obtained by slow evaporation from solutions in ethanol at room temperature. The crystal data are summarized in Table S1 (ESI†). The complex HNP-Cu crystallizes in the orthorhombic system and each unit cell contains two complex molecules. Moreover, as shown in Fig. S16a (ESI†), one HNP molecule coordinates with two Cu2+ ions, which is consistent with the titration experiments. Each Cu atom is four-coordinate with four atoms (oxygen and nitrogen atoms), which results in removal of the hydrogen ions of the hydroxyl groups and thus the process of ESIPT was intercepted, so that it shows fluorescence quenching. The two planes of the terminal aromatic rings of HNP are parallel; therefore, a 1D chain was formed by countless helices in the same direction via intermolecular π⋯π interactions (d ≈ 3.30 Å) and intramolecular hydrogen bond interactions (Fig. S16b, ESI†). HNP is a highly selective and sensitive chemosensor for Cu2+ ions.
3. Conclusions
In summary, we report here a naphthaldehyde based Schiff base material with both excited state intramolecular proton transfer and AIEgen fluorescence emission which can undergo a polymorphic switch on changing the solvent. Crystals of both HNP-y and HNP-r, with different emission colours were obtained by slow evaporation in chloroform/ethanol mixtures. Single crystal X-ray diffraction data and DFT calculations show that the HNP-r molecule is formed in a more compact stacking mode due to the smaller dihedral angle of the terminal aromatic ring planes and stronger intermolecular interactions, resulting in a red-shifted emission and weaker fluorescence emission. The multiple stimuli-responsive behaviour of HNP results in mechanochromic luminescence and its ability to act as a sensitive chemosensor for the Cu2+ ion. Our work provides a strategy for further understanding the relationship between the molecular structure and the emission mechanism at the molecular level and for the design of multi-stimuli responsive materials and can contribute to the understanding and the future design of new mechanochromic luminescent materials. It will stimulate a new approach for the realization of mechanochromic luminescent materials and provide a new understanding of the nature of mechanochromic behaviour.
Author contributions
Lingyi Shen carried out the experiments, wrote the paper and analyzed the X-ray structure. Chang-Jin Yu, carried out the experiments and wrote the paper. Hai-Fang Xie and Na Xu carried out the experiments. Hong Xu analyzed the experimental data. Ya-Li Huang provided experimental guidance. Xing Feng provided experimental guidance and design. Carl Redshaw analyzed the experimental data and revised the paper. Qi-Long Zhang provided research ideas, designed the experiments and modified the paper.
Conflicts of interest
There are no conflicts to declare.
Acknowledgements
This work was supported by the National Natural Science Foundation of China (22065009 and 22066007), Guizhou Provincial Natural Science Foundation ZK[2021]076, Guizhou Provincial Department of Education Foundation KY[2022]229 and the Guizhou Province College Students Innovation and Entrepreneurship Project (S202110660004). C. R. thanks the EPSRC for an Overseas Travel Grant (EP/R23816/1).
Notes and references
-
(a) S. Mo, Q. Meng, S. Wan, Z. Su, H. Yan, B. Z. Tang and M. Yin, Tunable mechanoresponsive self-assembly of an amide-linked dyad with dual sensitivity of photochromism and mechanochromism, Adv. Funct. Mater., 2017, 27, 1701210 CrossRef;
(b) W. Li, Q. Huang, Z. Mao, Q. Li, L. Jiang, Z. Xie, R. Xu, Z. Yang, J. Zhao, T. Yu, Y. Zhang, M. P. Aldred and Z. Chi, Alkylchain introduction: in situ solar-renewable colorful organic mechanoluminescence materials, Angew. Chem., Int. Ed., 2018, 57, 12727–12732 CrossRef CAS PubMed;
(c) Y. Li, S. Liu, T. Han, H. Zhang, C. Chuah, R. T. K. Kwok, J. W. Y. Lam and B. Z. Tang, Sparks fly when AIE meets with polymers, Mater. Chem. Front., 2019, 3, 2207–2220 RSC;
(d) M. A. C. Stuart, W. T. S. Huck, J. Genzer, M. Müller, C. Ober, M. Stamm, G. B. Sukhorukov, I. Szleifer, V. V. Tsukruk, M. Urban, F. Winnik, S. Zauscher, I. Luzinov and S. Minko, Emerging applications of stimuli-responsive polymer materials, Nat. Mater., 2010, 9, 101–113 CrossRef PubMed;
(e) Y. Sagara, S. Yamane, M. Mitani, C. Weder and T. Kato, Mechanoresponsive luminescent molecular assemblies: an emerging class of materials, Adv. Mater., 2016, 28, 1073–1095 CrossRef CAS PubMed.
-
(a) Z. Yang, Z. Chi, Z. Mao, Y. Zhang, S. Liu, J. Zhao, M. P. Aldred and Z. Chi, Recent advances in mechanoresponsive luminescence of tetraphenylethylene derivatives with aggregation-induced emission properties, Mater. Chem. Front., 2018, 2, 861–890 RSC;
(b) L. Tan, S. Mo, B. Fang, W. Cheng and M. Yin, Dual fluorescence switching of a Rhodamine 6G-naphthalimide conjugate with high contrast in the solid state, J. Mater. Chem. C, 2018, 6, 10270–10275 RSC.
-
(a) X. Guo, D. Zhang and D. Zhu, Logic control of the fluorescence of a new dyad, spiropyran-perylene diimide-spiropyran, with light, ferric ion, and proton: construction of a new three-input “AND” logic gate, Adv. Mater., 2004, 16, 125–130 CrossRef CAS;
(b) F. M. Raymo, R. J. Alvarado, S. Giordani and M. A. Cejas, Memory effects based on intermolecular photoinduced proton transfer, J. Am. Chem. Soc., 2003, 125, 2361–2364 CrossRef CAS PubMed;
(c) L. Zhu, M.-Q. Zhu, J. K. Hurst and A. D. Q. Li, Light-controlled molecular switches modulate nanocrystal fluorescence, J. Am. Chem. Soc., 2005, 127, 8968–8970 CrossRef CAS PubMed.
- Z. Wang, F. Yu, W. Chen, J. Wang, J. Liu, C. Yao, J. Zhao, H. Dong, W. Hu and Q. Zhang, Rational control of charge transfer excitons toward high-contrast reversible mechanoresponsive luminescent switching, Angew. Chem., Int. Ed., 2020, 59, 17580–17586 CrossRef CAS PubMed.
-
(a) G. Huang, Y. Jiang, S. Yang, B. S. Li and B. Z. Tang, Multistimuli response and polymorphism of a novel tetraphenylethylene derivative, Adv. Funct. Mater., 2019, 29, 1900516 CrossRef;
(b) Z. Lu, S. Yang, X. Liu, Y. Qin, S. Lu, Y. Liu, R. Zhao, L. Zheng and H. Zhang, Facile synthesis and separation of E/Z isomers of aromatic-substituted tetraphenylethylene for investigating their fluorescent properties via single crystal analysis, J. Mater. Chem. C, 2019, 7, 4155–4163 RSC.
-
(a) X. Feng, J. Zhang, Z. Hu, Q. Wang, M. M. Islam, J.-S. Ni, M. R. J. Elsegood, J. W. Y. Lam, E. Zhou and B. Z. Tang, Pyrene-based aggregation-induced emission luminogens (AIEgen): structure correlated with particle size distribution and mechanochromism, J. Mater. Chem. C, 2019, 7, 6932–6940 RSC;
(b) J. Zeng, X. Wang, X. Song, Y. Liu, B. Liao, J. Bai, C. Redshaw, Q. Chen and X. Feng, Steric influences on the photophysical properties of pyrene-based derivatives; mechanochromism and their pH-responsive ability, Dyes Pigm., 2022, 200, 110123 CrossRef CAS.
- B. Huang, Z. Li, H. Yang, D. Hu, W. Wu, Y. Feng, Y. Sun, B. Lin and W. Jiang, Bicolour electroluminescence of 2-(carbazol-9-yl)anthraquinone based on a solution process, J. Mater. Chem. C, 2017, 5, 12031–12034 RSC.
-
(a) J. Luo, Z. Xie, J. W. Y. Lam, L. Cheng, H. Chen, C. Qiu, H. S. Kwok, X. Zhan, Y. Liu, D. Zhu and B. Z. Tang, Aggregation-induced emission of 1-methyl-1,2,3,4,5-pentaphenylsilole, Chem. Commun., 2001, 1740–1741 RSC;
(b) Y. Hong, J. W. Y. Lam and B. Z. Tang, Aggregation-induced emission, Chem. Soc. Rev., 2011, 40, 5361–5388 RSC;
(c) J. Huang, N. Sun, J. Yang, R. Tang, Q. Li, D. Ma and Z. Li, Blue aggregation induced emission luminogens: high external quantum efficiencies up to 3.99% in LED device, and restriction of the conjugation length through rational molecular design, Adv. Funct. Mater., 2014, 24, 7645–7654 CrossRef CAS;
(d) H. Wu, X.-C. Fan, H. Wang, F. Huang, X. Xiong, Y.-Z. Shi, K. Wang, J. Yu and X.-H. Zhang, Conformational isomerization: A novel mechanism to realize the AIE-TADF behaviors, Aggregate, 2022 DOI:10.1002/agt2.243.
-
(a) V. S. Padalkar and S. Seki, Excited-state intramolecular proton-transfer (ESIPT)-inspired solid state emitters, Chem. Soc. Rev., 2016, 45, 169–202 RSC;
(b) G. Yang, Y. Ran, Y. Wu, M. Chen, Z. Bin and J. You, Endowing imidazole derivatives with thermally activated delayed fluorescence and aggregation-induced emission properties for highly efficient non-doped organic light-emitting diodes, Aggregate, 2021, 3, e127, DOI:10.1002/agt2.127;
(c) T. Zhou, T. Jia, B. Kang, F. Li, M. Fahlman and Y. Wang, Nitrile-substituted QA derivatives: new acceptor materials for solution-processable organic bulk heterojunction solar cells, Adv. Energy Mater., 2011, 1, 431–439 CrossRef CAS;
(d) C. Wang, K. Wang, Q. Fu, J. Zhang, D. Ma and Y. Wang, Pentaphenylphenyl substituted quinacridone exhibiting intensive emission in both solution and solid state, J. Mater. Chem. C, 2013, 1, 410–413 RSC.
- Y. Li, R. Zhang, Q. Wan, R. Hu, Y. Ma, Z. Wang, J. Hou, W. Zhang and B. Z. Tang, Trojan horse-like nano-AIE aggregates based on homologous targeting strategy and their photodynamic therapy in anticancer application, Adv. Sci., 2021, 8, 2102561 CrossRef CAS PubMed.
-
(a) J. Zhao, S. Ji, Y. Chen, H. Guo and P. Yang, Excited state intramolecular proton transfer (ESIPT): from principal photophysics to the development of new chromophores and applications in fluorescent molecular probes and luminescent materials, Chem. Chem. Phys., 2012, 14, 8803–8817 RSC;
(b) M. K. Bera, C. Chakraborty, P. K. Singh, C. Sahu, K. Sen, S. Maji, A. K. Das and S. Malik, Fluorene-based chemodosimeter for “turn-on”
sensing of cyanide by hampering ESIPT and live cell imaging, J. Mater. Chem. B, 2014, 2, 4733–4739 RSC.
-
(a) K. Sakai, T. Ishikawa and T. Akutagawa, A blue-white-yellow color-tunable excited state intramolecular proton transfer (ESIPT) fluorophore: sensitivity to polar–nonpolar solvent ratios, J. Mater. Chem. C, 2013, 1, 7866–7871 RSC;
(b) Y. Hong, J. W. Y. Lam and B. Z. Tang, Aggregation-induced emission, Chem. Soc. Rev., 2011, 40, 5361 RSC.
- Z. Hu, H. Zhang, Y. Chen, Q. Wang, M. R. J. Elsegood, S. J. Teat, X. Feng, M. M. Islam, F. Wu and B. Z. Tang, Tetraphenylethylene-based color-tunable AIE-ESIPT chromophores, Dyes Pigm., 2020, 175, 108175 CrossRef CAS.
- H.-F. Xie, C.-J. Yu, Y.-L. Huang, H. Xu, Q.-L. Zhang, X.-H. Sun, X. Feng and C. Redshaw, A turn-off fluorescent probe for the detection of Cu2+ based on a tetraphenylethylene-functionalized salicylaldehyde Schiff-base, Mater. Chem. Front., 2020, 4, 1500–1506 RSC.
- C. Redshaw, M. R. J. Elsegood, J. W. A. Frese, S. Ashby, Y. Chao and A. Mueller, Cellular uptake studies of two hexanuclear, carboxylate bridged, Zinc ring structures using fluorescence microscopy, Chem. Commun., 2012, 48, 6627–6629 RSC.
- X. Zhang, S.-T. Wu, X.-J. Yang, L.-Y. Shen, Y.-L. Huang, H. Xu, Q.-L. Zhang, T. Sun, C. Redshaw and X. Feng, Dynamic Coordination between a Triphenylamine-Functionalized Salicylaldehyde Schiff Base and a Copper(II) Ion, Inorg. Chem., 2021, 60, 8581–8591 CrossRef CAS PubMed.
-
(a) F. Han, R. Zhang, Z. Zhang, J. Sua and Z. Ni, A new TICT and AIE-active tetraphenylethene-based Schiff base with reversible piezofluorochromism, RSC Adv., 2016, 6, 68178–68184 RSC;
(b) P. Yadav, A. K. Singh, C. Upadhyay and V. P. Singh, Photoluminescence behaviour of a stimuli responsive Schiff base: Aggregation induced emission and piezochromism, Dyes Pigm., 2019, 160, 731–739 CrossRef CAS;
(c) I. Majumder, P. Chakraborty, S. Dasgupta, C. Massera, D. Escudero and D. Das, A Deep Insight into the photoluminescence properties of Schiff base CdII and ZnII complexes, Inorg. Chem., 2017, 56, 12893–12901 CrossRef CAS PubMed.
- Y. Nie, H. Zhang, J. Miao, X. Zhao, Y. Li and G. Sun, Synthesis, aggregation-induced emission and mechanochromism of a new carborane-tetraphenylethylene hybrid, J. Organomet. Chem., 2018, 865, 200–205 CrossRef CAS.
-
(a) A. Klein, A.-K. Schmieder, N. Hurkes, C. Hamacher, A. O. Schüren, M. P. Feth and H. Bertagnolli, Mono- and Binuclear Arylnickel Complexes of the α-Diimine Bridging Ligand 2,2′-Bipyrimidine (bpym), Eur. J. Inorg. Chem., 2010, 934–941 CrossRef CAS;
(b) L. Shen, Y. Zhao, Q. Luo, Q.-S. Li, B. Liu, C. Redshaw, B. Wu and X.-J. Yang, Cyclotrimerization of alkynes catalyzed by a self-supported cyclic tri-nuclear nickel(0) complex with α-diimine ligands, Dalton Trans., 2019, 48, 4643 RSC.
-
(a) E. Ragnoni, D. M. Di, A. Iagatti, A. Lapini and R. Righini, Mechanism of the intramolecular iharge transfer state formation in all-trans-β-Apo-8′-carotenal: influence of solvent polarity and polarizability, J. Phys. Chem. B, 2015, 119, 420–432 CrossRef CAS PubMed;
(b) Y. J. Zhang, K. Wang, G. L. Zhuang, Z. Q. Xie, C. Zhang, F. Cao, G. X. Pan, H. F. Chen, B. Zou and Y. G. Ma, Multicolored-fluorescence switching of ICT-type organic solids with clear color difference:
mechanically controlled excited state, Chem. – Eur. J., 2015, 21, 2474–2479 CrossRef CAS PubMed;
(c) V. A. Galievsky, S. I. Druzhinin, A. Demeter, P. Mayer, S. A. Kovalenko, T. A. Senyushkina and K. A. Zachariasse, Ultrafast intramolecular charge transfer with N-(4-cyanophenyl)carbazole. evidence for a LE precursor and dual LE + ICT fluorescence, J. Phys. Chem. A, 2010, 114, 12622–12638 CrossRef CAS PubMed.
- Q. Yu, X. Zhang, S.-T. Wu, H. Chen, Q.-L. Zhang, H. Xu, Y.-L. Huang, B.-X. Zhu and X.-L. Ni, Twisted Schiff-base macrocycle showing excited-state intramolecular proton-transfer (ESIPT): assembly and sensing properties, Chem. Commun., 2020, 56, 2304–2307 RSC.
- M. Das, M. Brahma and G. Krishnamoorthy, Light-driven switching between intramolecular proton-transfer and charge-transfer states, J. Phys. Chem. B, 2021, 125, 2339–2350 CrossRef CAS PubMed.
- J. E. Kwon and S. Y. Park, Advanced organic optoelectronic materials: Harnessing excited-state intramolecular proton transfer (ESIPT) process, Adv. Mater., 2011, 23, 3615–3642 CrossRef CAS PubMed.
- H.-W. Zheng, S. Li, M. Wu, Y. Kang, J.-B. Li, Q.-F. Liang, X.-J. Zheng, D.-C. Fang and L.-P. Jin, Multi-stimuli responsive behaviors of two TPE-based tautomers in the solid state and in solution, J. Mater. Chem. C, 2020, 8, 4246–4252 RSC.
- G. L. Long and J. D. Winefordner, Limit of detection. A closer look at the IUPAC definition, Anal. Chem., 1983, 55, 712A–724A CrossRef CAS.
- P. Thordarson, Determining association constants from titration experiments in supramolecular chemistry, Chem. Soc. Rev., 2011, 40, 1305–1323 RSC.
Footnotes |
† Electronic supplementary information (ESI) available: Detailed experimental description, characterization and physical measurements (PDF). X-ray crystallographic data for the three compounds (CIF). CCDC 2174338–2174340. For ESI and crystallographic data in CIF or other electronic format see DOI: https://doi.org/10.1039/d2qm00542e |
‡ L. Shen and C.-J. Yu contributed equally to this work. |
|
This journal is © the Partner Organisations 2022 |