DOI:
10.1039/D2PY00873D
(Paper)
Polym. Chem., 2022,
13, 5226-5233
Well-defined amine-reactive polymethacrylates through organocatalyzed controlled radical polymerization†
Received
5th July 2022
, Accepted 16th August 2022
First published on 17th August 2022
Abstract
A novel alkyl iodide bearing an azlactone group was synthesized and used as a reversible complex-mediated polymerization (RCMP) initiator for the organocatalyzed RCMP of methyl methacrylate (MMA) and poly(ethylene glycol) methyl ether methacrylate (mPEGMA). The efficiency of the new initiator was studied under different processes including thermal and photochemical activation. Well-defined α-azlactone-functionalized polymethacrylates (Đ < 1.30) with an absence of “free” toxic transition metals were obtained for the first-time. The amine-scavenging ability of these functional polymers was demonstrated by using benzylamine.
Introduction
Over the past decade, the azlactone functionality has become one of the most useful functional groups for organic chemistry and (bio)material synthesis.1–4 Due to its high reactivity towards amines, azlactone reacts readily with amine containing molecules without the need for a catalyst. Furthermore, the absence of side-product formation is a considerable advantage of the “azlactone-amine” reaction.5 Thanks to its high reactivity towards amines the azlactone group has been incorporated into polymer chains to obtain azlactone-based materials. These azlactone functionalized polymers were further used to immobilize amine-containing (bio)molecules such as peptide and protein for affinity chromatography,6 drug delivery7 and enzyme immobilization.8 Moreover, azlactone-containing polymers have also been used for preparing core–shell nanoparticles9 and nanostructured thin-films.10 Azlactone-functionalized polymers can be obtained either by introducing azlactone groups along the polymer backbone or by functionalization of the polymer chain-end. While polymers bearing multi-azlactone groups have been widely reported, there are few studies on the synthesis of azlactone-terminated polymers. In an early study, Fontaine and coworkers described post-polymerization modification (PPM) as a powerful method to obtain ω-azlactone-functionalized polymers using “click” reactions of thiol or alkyne-terminated polymers with azlactone molecules bearing vinyl or azido (–N3) groups, respectively.11,12 In these studies, the ω-azlactone-terminated polymers were successfully coupled to lysozyme to obtain the lysozyme-polymer conjugated product. However, the multi-step synthesis is a major drawback of chain-end PPM approach. Alternatively, the combination of reversible-deactivation radical polymerization13 (RDRP) with an azlactone-functionalized molecule as initiator or transfer agent has been shown to be a suitable method to access azlactone-terminated polymers.1 This approach is not only a directly synthetic route to obtain α-azlactone functionalized polymers with a well-defined structure, but also allows a quantitative azlactone functionalization unlike PPM. For example, Lewandowski et al.14 have reported the atom transfer radical polymerization (ATRP) of methyl methacrylate (MMA) using a bromo-azlactone molecule as an initiator and copper(I) chloride/bipyridine as a catalytic system to afford an α-azlactone functionalized poly(methyl methacrylate) (PMMA). However, the residual copper catalyst raises the question of the toxicity of ATRP-based polymers for biomedical applications.15 Similarly, different α-azlactone-terminated (co)polymers have also been obtained using an azlactone-functionalized alkoxyamine as a nitroxide-mediated polymerization (NMP) initiator, whereas the synthesis of α-azlactone-terminated polymethacrylates by NMP has not been reported.16 Additionally, the NMP approach suffers from the tedious synthesis of the starting nitroxide radical as well as potential residual traces of the copper used as a catalyst during the synthesis of the alkoxyamine initiator. To avoid the use of a copper catalyst, Ho et al.17 reported the synthesis of a series of α-azlactone-functionalized polystyrenes, poly(ethyl acrylate)s and poly(N-isopropyl acrylamide)s by reversible addition–fragmentation chain transfer (RAFT) polymerization using an azlactone-trithiocarbonate as a transfer agent. Nevertheless, the ability of azlactone-trithiocarbonate to control the RAFT polymerization of methacrylates remains challenging. Therefore, the copper-free synthesis of azlactone-terminated polymethacrylates has become a major challenge.
In this context, the development of metal-free RDRP techniques offered new opportunities to synthesize well-defined polymers. Among them, the reversible complexation-mediated polymerization (RCMP) has emerged as a powerful organocatalyzed technique for synthesizing polymethacrylates with a well-defined structure.18–21 RCMP is known as a simple and versatile process that can be performed under thermal or photo-irradiation without the addition of a radical initiator. In this context, this contribution focuses on the use of RCMP to synthesize well-defined α-azlactone-terminated polymethacrylates. In this study, we describe the synthesis of a new azlactone-functionalized iodide RCMP initiator to prepare well-defined α-azlactone terminated polymethacrylates. The efficiency of this new initiator in polymerizing methacrylates by RCMP was investigated for the first time, and then the reactivity of the resulting azlactone-terminated polymers towards amines was then demonstrated by a coupling reaction with benzylamine.
Experimental
Materials and characterization
See ESI.†
Synthesis of 2-(1-iodoethyl)-4,4-dimethyloxazol-5(4H)-one (iodo-azlactone, I-Azl).
In a round bottom flask equipped with a magnetic stirrer and a dropping funnel, sodium iodide (NaI, 3.00 g, 20.0 × 10−3 mol) and acetone (15.0 mL) were added and stirred at room temperature under argon. Then, a solution of 2-(1-bromoethyl)-4,4-dimethyloxazol-5(4H)-one17 (bromo-azlactone, 2.00 g, 9.09 × 10−3 mol) in acetone (5.0 mL) was added dropwise into the reaction mixture at room temperature, with a white solid forming during the addition. The reaction mixture was then stirred continuously at room temperature for 10 min and then at 40 °C for 2 h. The reaction mixture was filtered to remove the white salt and the filtrate was subsequently concentrated under reduced pressure at room temperature. The crude product was dissolved in diethyl ether, filtered and concentrated under vacuum to yield the final product as an orange oil. Yield: 75% (1.83 g). The product was stored under argon at −20 °C avoiding any contact with light and water. HR-MS analysis (C7H10NO2I): detected ion [M + H]+, calculated value m/zcalc = 267.9829 Da and experimental value m/zexp = 267.9828 Da. 1H NMR (300 MHz, CDCl3) δ (ppm): 4.73 (q, J = 7.1 Hz, I-CH(CH3)–), 2.08 (d, J = 7.1 Hz, I-CH(CH3)–), 1.41 and 1.40 (s, –C(CH3)2) (Fig. 1A). 13C NMR (75 MHz, CDCl3) δ (ppm): 180.23 (C
O)azlactone, 164.10 (C
N)azlactone, 66.18 (C(CH3)2), 23.89 and 23.70 (C(CH3)2), 23.18 (ICH(CH3)–), 8.17 (ICH(CH3)–) (see ESI Fig. S1†). FT-IR (cm−1): 1824 (ν(C
O)azlactone), 1657 (ν(C
N)azlactone) (see ESI Fig. S2†).
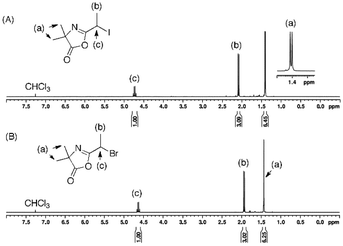 |
| Fig. 1
1H NMR (300 MHz) spectra of (A) I-Azl and (B) bromo-azlactone in CDCl3. | |
Typical thermal procedure for the reversible-mediated complex polymerization (RCMP)
RCMP of methyl methacrylate (MMA).
MMA (3.70 g, 0.037 mol), I-Azl (0.10 g, 3.75 × 10−4 mol), and tetrabutylammonium iodide (TBAI, 0.070 g, 1.90 × 10−4 mol) were sequentially added into a headspace vial equipped with a magnetic stir bar. The vial was then sealed using a headspace cap. The resulting mixture was deoxygenated by bubbling argon for 30 min under cooling in an iced bath. The mixture was then continuously stirred under argon for 10 min to reach room temperature. The vial was immersed in an oil bath thermostated at 80 °C to allow the polymerization to occur (initial reaction time, t = 0). Samples were removed periodically using a deoxygenated syringe to perform size exclusion chromatography (SEC) analysis and to monitor monomer conversion by 1H NMR spectroscopy. After 120 min, the polymerization was quenched by rapid cooling and exposure of the polymerization solution to air. The reaction mixture was afterwards dissolved in dichloromethane (DCM) and precipitated in methanol. The final polymer was filtered out and dried under vacuum at room temperature to obtain a white solid with DPn,NMR = 81, Mn,SEC = 9460 g mol−1, Đ = 1.19. 1H NMR (400 MHz, CDCl3) δ (ppm): 3.77 (–C(CH3)(I)COOCH3), 3.58 (–COOCH3), 2.06–1.65 (–CH2–C(CH3)–), 1.33–0.72 (–CH2–C(CH3)– and –CH3azlactone) (Fig. 3). FT-IR (cm−1): 1820 (ν(C
O)azlactone), 1725 (ν(C
O)ester) (see ESI Fig. S3†).
RCMP of mPEGMA.
Polymerization of mPEGMA was conducted according to the similar previous protocol for MMA at 80 °C using I-Azl (0.020 g, 7.49 × 10−5 mol), mPEGMA (Mn = 475 g mol−1, 3.55 g, 7.47 × 10−3 mol) and TBAI (33.4 mg, 9.05 × 10−5 mol). After 180 min, the mPEGMA conversion was at 7% with Mn,SEC = 7440 g mol−1 and Đ = 1.09.
Typical procedure for the photo-polymerization of mPEGMA by using I-Azl initiator with UV lamp operating at 365 nm
Using tri(n-butylamine) as a catalyst.
I-Azl (0.020 g, 7.49 × 10−5 mol), mPEGMA (Mn = 475 g mol−1, 1.42 g, 2.99 × 10−3 mol) and tri(n-butyl)amine (13.14 mg, 7.10 × 10−5 mol) were sequentially added into a headspace vial equipped with a magnetic stir bar. The vial was then sealed using a headspace cap. The mixture was then deoxygenated by bubbling argon for 30 min at room temperature. The deoxygenated vial was then irradiated inside the operating UV nail box (λ = 365 nm, 36 W) to allow the polymerization to occur (initial reaction time, t = 0). During polymerization, the temperature of the nail box was kept below 40 °C using computer fans. Samples were removed periodically using a deoxygenated syringe to perform SEC analysis and to monitor monomer conversion by 1H NMR spectroscopy. After 240 min, the polymerization was quenched by rapid cooling and exposure of the reaction mixture to air. The α-azlactone-functionalized poly(poly(ethylene glycol) methyl ether methacrylate) (poly(mPEGMA)) was precipitated in diethyl ether and dried under vacuum at room temperature to obtain a yellow gummy paste with Mn,SEC = 18
410 g mol−1, Đ = 1.18 (see ESI Table S1,† run 8). 1H NMR (400 MHz, CDCl3) δ (ppm): 4.07 (–COOCH2CH2O–), 3.76–3.57 (–CH2CH2O– of PEG unit). 3.37 (–OCH3), 2.05–1.47 (–CH2C(CH3)–), 1.44–0.74 (–CH2C(CH3)– and –CH3azlactone) (Fig. 6A). FT-IR (cm−1): 1820 (ν(C
O)azlactone), 1725 (ν(C
O)ester) (see ESI Fig. S4A†).
Using TBAI as a catalyst.
Photo-polymerization was carried out according to the previous procedure using I-Azl (0.020 g, 7.49 × 10−5 mol), mPEGMA (Mn = 475 g mol−1, 1.2 g, 2.53 × 10−3 mol) and TBAI (11.3 mg, 3.06 × 10−5 mol) (see ESI Table S2 and Fig. S4B†).
Coupling reaction of benzylamine with α-azlactone-functionalized polymethacrylates
α-Azlactone-functionalized PMMA (Mn,SEC = 9180 g mol−1, Đ = 1.22, 0.3 g, 3.27 × 10−5 mol) or poly(mPEGMA) (Mn,SEC = 20
770 g mol−1, Đ = 1.20, 0.3 g, 1.44 × 10−5 mol) (see ESI Table S3†) and tetrahydrofuran (THF, 2.00 mL) were charged into a glass scintillation flask equipped with a magnetic stir bar. Then, a solution of benzylamine (0.0243 g, 2.27 × 10−4 mol and 0.010 g, 9.35 × 10−5 mol for α-azlactone-functionalized PMMA and poly(mPEGMA), respectively) in THF (1.0 mL) was added into the reaction mixture and the resulting solution was stirred at 40 °C for 24 h under argon. The solution was then concentrated under rotary evaporator and precipitated in diethyl ether. The final polymer was filtered out and dried under vacuum at room temperature. SEC analysis: after coupling reaction of benzylamine with (1) α-azlactone-functionalized PMMA: Mn,SEC = 11
000 g mol−1, Đ = 1.13 and (2) α-azlactone-functionalized poly(mPEGMA): Mn,SEC = 20
500 g mol−1, Đ = 1.18.
Results and discussion
Organocatalyzed RCMP synthesis of well-defined α-azlactone-functionalized PMMA
The use of azlactone derivatives as an initiator or transfer agent in RDRP process has been rarely reported in the literature. We are only aware of three studies using azlactone derivatives as an ATRP initiator,14 NMP alkoxyamine16 or RAFT agent.17 Therefore, in this study a new azlactone-functionalized iodide was proposed and used as an initiator for the reversible complex-mediated polymerization (RCMP) of two methacrylate monomers. The 2-(1-iodoethyl)-4,4-dimethyloxazol-5(4H)-one (I-Azl) initiator was synthesized by the reaction between 2-(1-bromoethyl)-4,4-dimethyloxazol-5(4H)-one (bromo-azlactone) and sodium iodide (NaI) in acetone at 40 °C (Scheme 1).
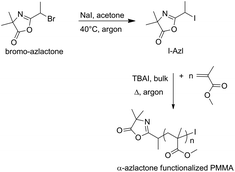 |
| Scheme 1 Synthesis of 2-(1-iodoethyl)-4,4-dimethyloxazol-5(4H)-one (I-Azl) and its mediated RCMP of MMA by using TBAI as a catalyst. | |
The successful bromine-iodine nucleophilic displacement reaction was confirmed by proton (1H) nuclear magnetic resonance (NMR) spectroscopy, with the signal –CH(CH3)X (labeled (c) in Fig. 1) shifting from 4.63 ppm to 4.73 ppm when bromine (X = Br) is replaced by a iodine (X = I) (Fig. 1A). I-Azl is expected to be a useful RCMP initiator for the synthesis of α-azlactone-functionalized polymers due to its secondary alkyl iodide structure adjacent to the strongly electron-withdrawing azlactone cycle acting as a stabilizing group.
Organic catalysts play an important role in the control and kinetic of RCMP as well as in the synthesis of high molar mass polymers.18–20 Due to its high reactivity, tetrabutylammonium iodide (TBAI) is widely chosen as catalyst for RCMP of methyl methacrylate (MMA).19,20 Typically, the polymerization temperature is strongly influenced by the initiator structure. For instance, previous studies showed that the RCMP initiators I-CH(CH3)– adjacent to an ester group (R: ethyl, phenyl) could only be initiated at 110 °C.22,23 To highlight the efficiency of the I-Azl where the I-CH(CH3)– part is adjacent to the azlactone group, the polymerization was carried out at 70 °C and 80 °C in bulk with the presence of 0.51 equiv. TBAI relative to I-Azl under argon (Scheme 1). Kinetic studies (Fig. 2A) showed linearity of pseudo-first order plots with a constant propagating radical concentration during polymerization at both temperatures. Fig. 2A also indicated an induction period. Such behavior could be due to the time required for complexation between the TBAI catalyst and the I-Azl initiator to trigger polymerization and/or to solubilize the TBAI in MMA. Furthermore, faster polymerization at 80 °C compared to 70 °C can be explained by an increase in the solubility of TBAI. Moreover, the low dispersities (Đ < 1.30) together with a linear evolution of the number-average molar masses determined by SEC analysis (Mn,SEC) against monomer conversion were observed, indicating that simultaneous polymer chain growth and no significant transfer reaction occurred during both polymerizations (Fig. 2B). The difference value between Mn,SEC and the theoretical number-average molar masses (Mn,th) could be attributed to the insufficient amount of deactivator (iodine/catalyst complex) accumulated in the early stage of polymerization. Indeed, the generated alkyl radicals from I-Azl or oligomers can combine quickly to terminate the polymerization reducing the initiator efficiency.
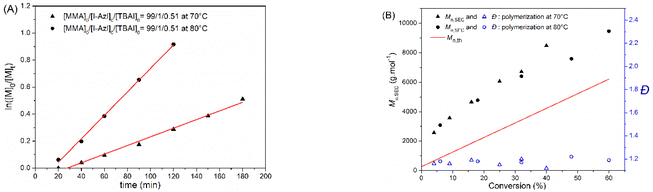 |
| Fig. 2 Polymerizations of MMA at 70 °C and 80 °C using I-Azl as the initiator and TBAI as the catalyst with: (A) kinetic plot versus time polymerization; (B) evolution of number-average molar mass and dispersities with conversion. | |
After precipitation, the presence of the azlactone group in the α-azlactone-terminated PMMA was confirmed by Fourier-Transform Infra-Red (FT-IR) spectroscopy with a characteristic band at 1820 cm−1 due to the carbonyl group of the azlactone ring (ν(C
O)azlactone) and a strong band at 1725 cm−1 due to the carbonyl ester (ν(C
O)ester) of the PMMA chain (ESI Fig. S3†).
In addition, the chemical structure of ω-iodide-terminated PMMA chain was confirmed by 1H NMR analysis with the presence of a small signal at 3.77 ppm assigned to the methyl ester group adjacent to the ω-iodide end chain19 (–C(CH3)(I)COOCH3, labeled (g) in Fig. 3); the large signal at 3.58 ppm being attributed to the –COOCH3 groups of the main chain (labeled (f) in Fig. 3). Finally, the number-average degree of polymerization (DPn,NMR) was determined by 1H NMR from the ratio of the integrations of these two signals and was estimated to be DPn,NMR = 81. This DPn,NMR value corresponds to a polymer chain having Mn,NMR = 8370 g mol−1 that correlates well with the Mn,SEC = 9460 g mol−1. These results show that the I-Azl is an efficient RCMP initiator to mediate the controlled RCMP of MMA to prepare a well-defined α-azlactone-functionalized PMMA.
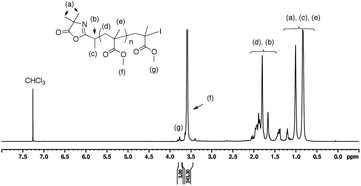 |
| Fig. 3
1H NMR spectrum (400 MHz) of a well-defined α-azlactone-functionalized PMMA (DPn,NMR = 81, Mn,SEC = 9460 g mol−1, Đ = 1.19) in CDCl3. | |
Impact of oxygen, catalyst amount and monomer structure on the efficiency of I-Azl
Due to the oxygen reaction with propagating radicals, the deoxygenation of a reaction mixture is a crucial step in radical polymerization. Therefore, the development of oxygen tolerant polymerization processes is still highly desired in polymer synthesis.24 Recently, Wang et al.25 have reported that the RCMP of MMA could be carried out without deoxygenation by using the iodine (I2) and N,N,N′,N′′,N′′-pentamethyldiethylenetriamine (PMDETA) as a catalyst. However, aside from this report, most RCMP experiments have been performed under inert atmosphere. Accordingly, we evaluated the oxygen tolerance of RCMP using TBAI catalyst and investigated the efficiency of the I-Azl initiator in performing a polymerization process under air. The I-Azl-mediated polymerization of MMA has been carried out with [MMA]0/[I-Azl]0/[TBAI]0 = 99/1/0.51 at 80 °C without the deoxygenation step. Fig. 4 confirmed the good control of the polymerization of MMA as indicated by the linear first-order kinetic plot of the polymerization and the low Đ values of the resulting polymers. This result clearly demonstrates the oxygen tolerance of RCMP technique as well as the efficiency of I-Azl in controlling polymerization of MMA even under air and without addition of I2. A longer inhibition period is observed for the polymerization performed under air (Fig. 4A and Table 1), oxygen being known to be a radical inhibitor, preventing the growth of macromolecular chains. Subsequently, deoxygenation was considered as a crucial step for further experiments to improve the efficiency of I-Azl. The influence of the [TBAI]0/[I-Azl]0 ratio was also studied during RCMP of MMA. Increasing [TBAI]0/[I-Azl]0 ratios from 0.51 to 1.21 resulted in a faster polymerization rate with higher monomer conversion after 90 min (entry 1 vs. entry 3, Table 1 and Fig. 4).
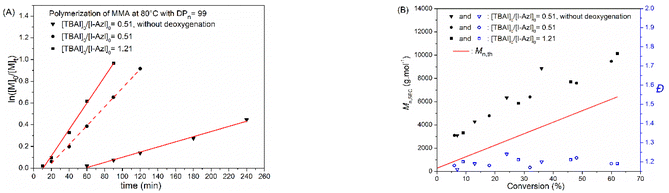 |
| Fig. 4 Polymerization of MMA at 80 °C using I-Azl as the initiator and TBAI as the catalyst with: (A) kinetic plot versus time polymerization; (B) evolution of number-average molar mass and dispersity values with monomer conversion. | |
Table 1 Summary of I-Azl mediated RCMP of MMA at 80 °C under different conditions
Entry |
[MMA]0 : [I-Azl]0 : [TBAI]0 |
Time (min) |
Conv.a (%) |
M
n,th
(g mol−1) |
M
n,SEC
(g mol−1) |
Đ
|
Determined by 1H NMR spectroscopy.
M
n,th = MI-Azl + (99 × Conv. × MMMA/100) with MMMA = 100 g mol−1.
Determined by size exclusion chromatography with THF as an eluent and PMMA standards used for the calibration curve.
Without deoxygenation step.
|
1 |
99 : 1 : 0.51 |
90 |
48 |
5020 |
7590 |
1.22 |
2 |
99 : 1 : 0.51d |
90 |
7 |
960 |
3090 |
1.16 |
3 |
99 : 1 : 1.21 |
90 |
62 |
6400 |
10 140 |
1.19 |
Among the methacrylic monomers, due to the biocompatible and ionic conductive properties of poly(ethylene oxide), mPEGMA has emerged as one of the leading monomers for the preparation of the biorelevant materials as well as solid polyelectrolyte for battery applications.26,27 To expand our research, the I-Azl initiator was used for the polymerization of mPEGMA with a [mPEGMA]0/[I-Azl]0/[TBAI]0 ratio of 100/1/1.21 at 80 °C under argon. After 180 min of polymerization, the monomer conversion was only 7% giving a polymer with Mn,SEC = 7440 g mol−1 and Đ = 1.09. According to the literature, RCMP in polar media at high temperatures higher than 55 °C can lead to the elimination of hydrogen iodide (HI) from the polymer chain-end.28 Therefore, the low conversion of mPEGMA can be attributed to the deactivation of the propagating radical by elimination of ω-iodide due to the hydrophilicity character of the mPEGMA monomer as a polar medium at 80 °C. To avoid the ω-iodide elimination, the RCMP process of mPEGMA could be improved by either using a thermal radical initiator and iodine (I2) at 60 °C or by photopolymerization.19,21,29 Nevertheless, the addition of co-initiators/I2 can lead to uncontrolled polymer chains and high polymer dispersity values. Therefore, the photopolymerization of mPEGMA using I-Azl as an initiator was considered in this study to synthesize well-defined α-azlactone-functionalized poly(mPEGMA).
I-Azl mediated RCMP of poly(ethylene glycol) methyl ether methacrylate under UV irradiation
The C–I bond of an alkyl iodide can be easily broken under exposure to visible or Ultra-violet (UV) radiation. So, the photo-irradiation has widely used to as alternative effective approach to initiate the RCMP process.29,30 Photo-trigged RCMP (photo-RCMP) was then carried out in bulk with tri(n-butyl)amine or TBAI as the catalyst (Scheme 2). In photo-RCMP, the best control of polymerization of mPEGMA was achieved with a [catalyst]0/[initiator]0 ratio equal to 0.95 when using tri(n-butyl)amine as a catalyst. This [catalyst]0/[initiator]0 ratio was adjusted to 0.41 when TBAI was used as a catalyst, in order to maintain a homogeneous solution due to the low solubility of TBAI in mPEGMA.
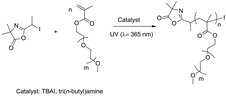 |
| Scheme 2 Photo-polymerization of mPEGMA at λ = 365 nm with I-Azl initiator and tri(n-butyl)amine or TBAI catalyst. | |
Fig. 5A and B show a pseudo-first order of kinetic plot and a linear evolution of Mn,SEC with monomer conversion together with low dispersity values (Đ < 1.2) for both catalysts. In addition, the unimodal SEC traces along with the progressive shift to shorter retention times illustrate the observed increase in molar mass throughout the polymerization, which is clearly indicative of a controlled radical polymerization (Fig. 5C and D). The structure of the obtained polymers was also verified by 1H NMR analysis showing the characteristics signals of the repeating poly(ethylene oxide) side chains at 3.76–3.57 (labeled (g) in Fig. 6) and 3.37 (labeled (h) in Fig. 6). Because of the low resolution of the signals of the two extremities in comparison with the other part of the polymer chain, it is difficult to determine the number-average degree of polymerization via NMR analysis. The presence of the azlactone group at the chain-end of poly(mPEGMA) was confirmed by FT-IR spectroscopy with the appearance of the distinguishable band ν(C
O)azlactone at 1820 cm−1 (see ESI Fig. S4†). All these results indicate that I-Azl is an efficient initiator to synthesize well-defined α-azlactone-functionalized poly(mPEGMA)s using a photo-RCMP process.
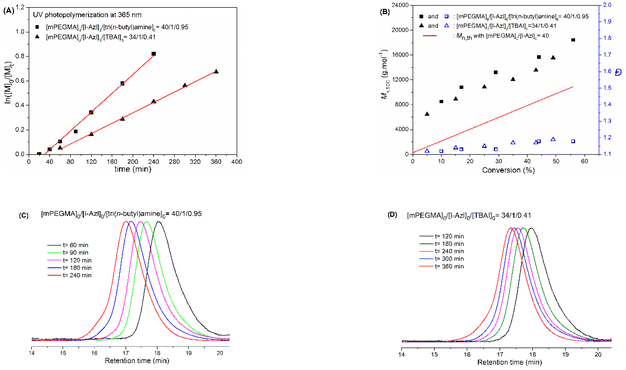 |
| Fig. 5 Photo-polymerization of mPEGMA at λ = 365 nm using tri(n-butyl)amine or TBAI as the catalyst: (A) kinetic plot versus time polymerization; (B) evolution of number-average molar mass and dispersity values with monomer conversion; (C) and (D) overlaid SEC traces of resulting polymer. | |
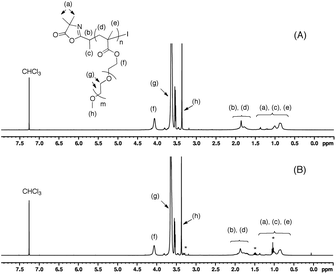 |
| Fig. 6
1H NMR spectra (400 MHz) of azlactone-functionalized poly(mPEGMA) obtained using (A) tri(n-butyl)amine and (B) TBAI catalyst in CDCl3 (* denoted the signal of residual TBAI catalyst). | |
Reactivity of azlactone-terminated polymers towards an amine
The azlactone ring has become an increasingly useful functionality for the rapid and modular design of functional materials thanks to its reactivity towards primary amines. To demonstrate the amine scavenging ability of the azlactone group installed at the polymer chain-end, α-azlactone-functionalized PMMA and α-azlactone-functionalized poly(mPEGMA) (ESI Table S3†) were reacted with an excess of benzylamine (6.9–6.5 equiv.) as a model amine (Scheme 3).
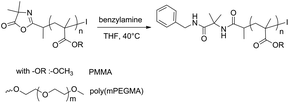 |
| Scheme 3 Coupling reaction between benzylamine and α-azlactone-functionalized PMMA and α-azlactone-functionalized poly(mPEGMA). | |
FT-IR analysis showed the disappearance of the characteristic carbonyl band of the azlactone group at 1820 cm−1 which confirmed the reaction between benzylamine and the azlactone chain-end (ESI Fig. S5 and 6†). The successful post-polymerization reaction of α-azlactone-terminated polymethacrylates was also confirmed by 1H NMR with the appearance of the aromatic signals of the benzyl group at 7.21–7.41 ppm (C6H5–, labeled (i) in Fig. 7) along with the characteristic signal of the polymer main chains. The benzylamide chain-end functionality of PMMA was calculated to be 72% by comparing the signal intensity of the phenyl group (C6H5–, labeled (i) in Fig. 7A) and the methyl ester of the MMA unit adjacent to the iodide end group (–COOCH3, labeled (g) in Fig. 7A).
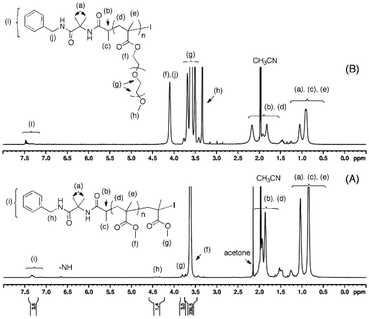 |
| Fig. 7
1H NMR spectra (400 MHz) of (A) benzylamine-functionalized PMMA (Mn,SEC = 11 000 g mol−1, Đ = 1.13) and (B) benzylamine-functionalized poly(mPEGMA, Mn,SEC = 20 500 g mol−1, Đ = 1.18) in CD3CN. | |
Conclusion
In conclusion, a novel azlactone-functionalized alkyl iodide (I-Azl) was successfully synthesized and used as an efficient initiator for the controlled polymerization of MMA under different experimental conditions to afford well-defined α-azlactone-functionalized PMMA by a metal-free process. The RCMP process with I-Azl as the initiator has proven to be tolerant to oxygen. Furthermore, I-Azl was demonstrated to be an excellent initiator for the photo-RCMP synthesis of α-azlactone-terminated poly(mPEGMA). The reactivity of the resulting α-azlactone-terminated polymers towards primary amines was demonstrated by their reaction with benzylamine as a model amine. These azlactone-terminated polymers based on a metal-free I-Azl mediated RCMP and having a highly amine-reactive terminal groups could be useful as a platform for new polymer materials and for bioconjugation applications.
Conflicts of interest
There are no conflicts to declare.
Acknowledgements
HTH thanks Christopher M. Plummer for assistance with editing the manuscript.
References
- H. T. Ho, M. E. Levere, D. Fournier, V. Montembault, S. Pascual and L. Fontaine, Aust. J. Chem., 2012, 65, 970–977 CrossRef CAS
.
- P. P. de Castro, A. G. Carpanez and G. W. Amarante, Chem. – Eur. J., 2016, 22, 10294–10318 CrossRef CAS PubMed
.
- S. M. Heilmann, J. K. Rasmussen and L. R. Krepski, J. Polym. Sci., Part A: Polym. Chem., 2001, 39, 3655–3677 CrossRef CAS
.
- H. T. Ho, V. Montembault, M. Rollet, S. Aboudou, K. Mabrouk, S. Pascual, L. Fontaine, D. Gigmes and T. N. T. Phan, Polym. Chem., 2020, 11, 4013–4021 RSC
.
- M. E. Buck and D. M. Lynn, Polym. Chem., 2012, 3, 66–80 RSC
.
- P. L. Coleman, M. M. Walker, D. S. Milbrath, D. M. Stauffer, J. K. Rasmussen, L. R. Krepski and S. M. Heilmann, J. Chromatogr. A, 1990, 512, 345–363 CrossRef CAS
.
- J. S. Kim, A. R. Sirois, A. J. Vazquez Cegla, E. Jumai'an, N. Murata, M. E. Buck and S. J. Moore, Bioconjugate Chem., 2019, 30, 1220–1231 CrossRef CAS PubMed
.
- S. P. Cullen, I. C. Mandel and P. Gopalan, Langmuir, 2008, 24, 13701–13709 CrossRef CAS PubMed
.
- M. E. Levere, H. T. Ho, S. Pascual and L. Fontaine, Polym. Chem., 2011, 2, 2878–2887 RSC
.
- J. W. Choi, M. C. D. Carter, W. Wei, C. Kanimozi, F. W. Speetjens, M. K. Mahanthappa, D. M. Lynn and P. Gopalan, Macromolecules, 2016, 49, 8177–8186 CrossRef CAS
.
- H. T. Ho, M. E. Levere, S. Pascual, V. Montembault, N. Casse, A. Caruso and L. Fontaine, Polym. Chem., 2013, 4, 675–685 RSC
.
- H. T. Ho, A. Bénard, G. Forcher, M. Le Bohec, V. Montembault, S. Pascual and L. Fontaine, Org. Biomol. Chem., 2018, 16, 7124–7128 RSC
.
- A. D. Jenkins, R. G. Jones and G. Moad, Pure Appl. Chem., 2010, 82, 483–491 CrossRef CAS
.
-
K. M. Lewandowski, D. D. Fansler, B. N. Gaddam, S. M. Heilmann, L. R. Krepski, S. B. Roscoe and M. S. Wendland, US6894133B2, 2005
.
- K. Matyjaszewski, Macromolecules, 2012, 45, 4015–4039 CrossRef CAS
.
- V. Delplace, S. Harrisson, H. T. Ho, A. Tardy, Y. Guillaneuf, S. Pascual, L. Fontaine and J. Nicolas, Macromolecules, 2015, 48, 2087–2097 CrossRef CAS
.
- H. T. Ho, F. Leroux, S. Pascual, V. Montembault and L. Fontaine, Macromol. Rapid Commun., 2012, 33, 1753–1758 CrossRef CAS PubMed
.
- A. Goto, T. Suzuki, H. Ohfuji, M. Tanishima, T. Fukuda, Y. Tsujii and H. Kaji, Macromolecules, 2011, 44, 8709–8715 CrossRef CAS
.
- A. Goto, A. Ohtsuki, H. Ohfuji, M. Tanishima and H. Kaji, J. Am. Chem. Soc., 2013, 135, 11131–11139 CrossRef CAS PubMed
.
- C.-G. Wang, A. M. L. Chong, H. M. Pan, J. Sarkar, X. T. Tay and A. Goto, Polym. Chem., 2020, 11, 5559–5571 RSC
.
- Z.-H. Chen, X.-Y. Wang and Y. Tang, Polym. Chem., 2022, 13, 2402–2419 RSC
.
- L. Lei, M. Tanishima, A. Goto, H. Kaji, Y. Yamaguchi, H. Komatsu, T. Jitsukawa and M. Miyamoto, Macromolecules, 2014, 47, 6610–6618 CrossRef CAS
.
- J. Zheng, C.-G. Wang, Y. Yamaguchi, M. Miyamoto and A. Goto, Angew. Chem., Int. Ed., 2018, 57, 1552–1556 CrossRef CAS PubMed
.
- J. Yeow, R. Chapman, A. J. Gormley and C. Boyer, Chem. Soc. Rev., 2018, 47, 4357–4387 RSC
.
- W. Wang, L. Bai, H. Chen, H. Xu, Y. Niu, Q. Tao and Z. Cheng, RSC Adv., 2016, 6, 97455–97462 RSC
.
- J.-F. Lutz, J. Polym. Sci., Part A: Polym. Chem., 2008, 46, 3459–3470 CrossRef CAS
.
- T. N. T. Phan, S. Issa and D. Gigmes, Polym. Int., 2019, 68, 7–13 CrossRef CAS
.
- C.-G. Wang, F. Hanindita and A. Goto, ACS Macro Lett., 2018, 7, 263–268 CrossRef CAS PubMed
.
- A. Ohtsuki, A. Goto and H. Kaji, Macromolecules, 2013, 46, 96–102 CrossRef CAS
.
- Y. Ni, L. Zhang, Z. Cheng and X. Zhu, Polym. Chem., 2019, 10, 2504–2515 RSC
.
|
This journal is © The Royal Society of Chemistry 2022 |