Asymmetric transfer hydrogenation of boronic acid pinacol ester (Bpin)-containing acetophenones†
Received
25th March 2022
, Accepted 12th April 2022
First published on 12th April 2022
Abstract
A series of Bpin-containing acetophenone derivatives were reduced by asymmetric transfer hydrogenation (ATH), using Noyori–Ikariya catalysts, with formic acid/triethylamine, to alcohols in high ee when the Bpin is in the para- or meta-position. Substrates containing ortho-Bpin groups were reduced in lower ee, with formation of a cyclic boron-containing group. The products were converted to substituted derivatives using Pd-catalysed coupling reactions. The results represent the first examples of ATH of Bpin-containing ketones.
Introduction
The asymmetric transfer hydrogenation (ATH) of ketones, to give enantiomerically-enriched alcohols, using Noyori–Ikariya catalysts [(arene)Ru(TsDPEN)Cl] such as 1 and derivatives such as 2–5 (Fig. 1),1 has been successfully applied extensively to a wide range of ketone substrates. Excellent results have been obtained for the ATH of acetophenone derivatives,2 acetylenic ketones,3 fluorinated derivatives,4 as well as dynamic kinetic resolutions of a number of substrate classes.5 Functional groups which are known to be compatible with Noyori–Ikariya catalysts include oxygenated, nitrogen-containing, carboxy groups, and halides.1–5
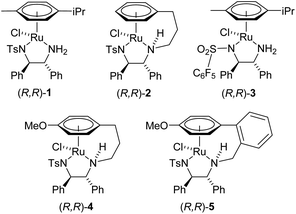 |
| Fig. 1 Catalysts commonly used in asymmetric transfer hydrogenation (ATH) of ketones, and in this study. | |
The ATH of acetophenone derivatives containing halides, particularly bromide and iodide, offers the possibility to functionalise the alcohol product through a number of processes, for example Pd-catalysed coupling reactions (Fig. 2a).6 In these cases, the coupling partners typically contain a boronic acid, or Bpin group.7 However, the requisite boron-containing aryl or vinyl reagent might not be readily available for the couplings and in these cases it would be advantageous for the ATH product to contain a boronic acid or Bpin since this would allow couplings to be carried out using the corresponding aryl or vinylic halides (Fig. 2b). To our surprise, a search of the literature indicated that the Ru-catalysed ATH of Bpin ketones has not previously been reported. The enzyme-catalysed resolution of Bpin-containing 1-arylethanols,8a and their kinetic resolution through oxidation,8b have been reported, as has their synthesis through the use of alcohol dehydrogenases (ADHs) for the asymmetric reduction of Bpin-containing ketones.8c,d Given the lack of data on ATH of boron-containing substrates, we investigated the ATH of a range of Bpin-containing acetophenone derivatives.
 |
| Fig. 2 (a) Asymmetric transfer hydrogenation (ATH) of substituted acetophenone substrates and subsequent coupling reactions. (b) ATH of Bpin-containing substrates followed by Pd-catalysed couplings. | |
Results and discussion
In our initial tests, we investigated the ATH of the known 4-Bpin substituted acetophenone 6, which was prepared via palladium-catalysed coupling reaction of 4-bromophenone with B2pin2.9 Despite the lack of literature precedents, the reduction proceeded cleanly, using formic acid/triethylamine 5
:
2 azeotrope (FA/TEA) as the reductant, 1 mol% of catalyst and DCM as a co-solvent, to give the corresponding alcohol 7 in full conversion and in 98% ee, using a range of ATH catalysts (Table 1). The 3C-tethered catalyst 2 gave the product in full conversion in 24 h, therefore this was selected for solvent screening. Alternative solvents, however, and a reaction without solvent, gave no improvement (Table 1).
Table 1 ATH of p-Bpin-acetophenonea
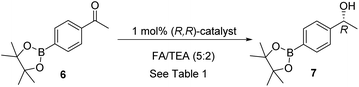
|
Entry |
Catalyst |
Co-solvent |
Time/h |
Yield/% |
ee/% |
Full conversion was observed in each case.
|
1 |
(R,R)-1 |
DCM |
72 |
56 |
98 |
2 |
(R,R)-2 |
DCM |
24 |
90 |
98 |
3 |
(R,R)-3 |
DCM |
72 |
52 |
98 |
4 |
(R,R)-4 |
DCM |
24 |
53 |
98 |
5 |
(R,R)-5 |
DCM |
24 |
53 |
97 |
6 |
(R,R)-2 |
None |
24 |
72 |
98 |
7 |
(R,R)-2 |
MeOH |
24 |
50 |
98 |
8 |
(R,R)-2 |
MeCN |
24 |
58 |
98 |
Alcohol product 7 has been reported in asymmetric form,8 and the R-configuration was confirmed by comparison of the optical rotation and the HPLC retention time data to the published values. Product 7 was converted to the known 4-Ph derivative 8 using a palladium-catalysed coupling with bromobenzene (Fig. 3), underlining the utility of the Bpin-containing products.8a
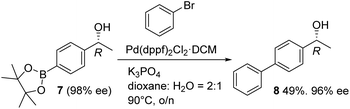 |
| Fig. 3 Conversion of Bpin-containing ATH product to the 4-phenyl derivative. | |
This was extended to a range of further Bpin-containing substrates, (Fig. 4). para- and meta-Bpin substituted acetophenones were reduced in full conversion and high ee to products 9–15 (Fig. 4a). The meta-substituted Bpin product 9 has been reported in asymmetric form, and a comparison of optical rotation and HPLC data served to confirm its R-configuration.8a Substituents at the α-position of the ketone, including Me, Ph, phenoxy and morpholine, were all tolerated.
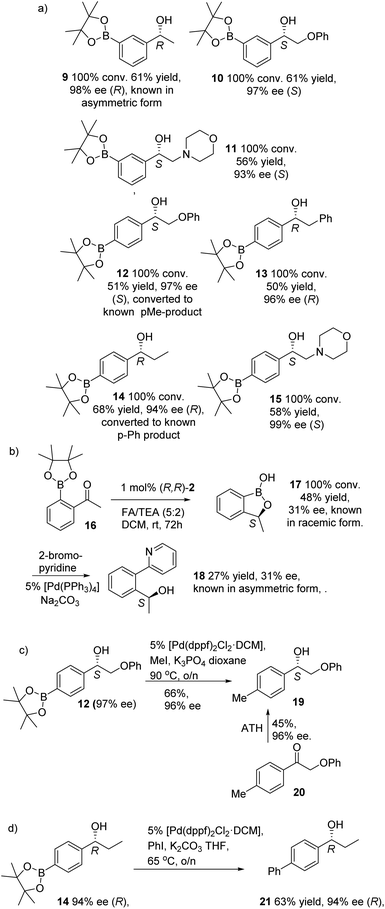 |
| Fig. 4 ATH products of Bpin-containing acetophenones; (a) para- and meta-substituted products. Conditions; 1 mol% catalyst (R,R)-2, FA/TEA, DCM, rt, 72 h (except for 9 and 14 which require 24 h). (b) ATH of ortho-Bpin acetophenone. (c) Introduction of a para-Me group to 12 gives the same product enantiomer as formed by direct ATH of 20 under identical conditions. (d) Pd-Catalysed conversion of (R)-14 to known (R)-21 to establish its configuration and ee. Products are novel unless otherwise indicated. | |
In contrast, an ortho-Bpin-substituted substrate 16 gave a cyclic benzoboroxole 17, which has previously been reported in racemic form,10 in much lower ee (Fig. 4b). The ee and configuration of the ortho Bpin product was established as S by comparison with the known product 18 of its Pd-catalysed coupling with 2-bromopyridine (Fig. 4b).11 It is not clear why the configuration of 17 is reversed relative to the para- and meta-substituted derivatives. The low ee reflects a marginal energy difference between reduction modes in this case.
In the case of 12 (97% ee), a palladium-catalysed reaction was used to substitute Bpin with Me, giving the previously-reported alcohol (S)-19 in 96% ee. The direct reduction of 20 with the same ATH catalyst enantiomer also resulted in the formation of (S)-19, in 96% ee (Fig. 4c).12 In this case, the configuration of the product generated through either pathway, with catalyst (R,R)-2, was identical, indicating that similar directing effects were operating in both cases. In addition, the R-configuration and ee of the propiophenone reduction product 14 (which could not be directly established by chiral HPLC) was confirmed by conversion to the known derivative 1-([1,1′-biphenyl]-4-yl)propan-1-ol 21 (Fig. 4d) through the [Pd(dppf)2Cl2·DCM]-catalysed reaction with PhBr (63% yield, 94% ee).13
A further series of substrates, containing aromatic rings opposing a para-Bpin-containing aryl group, were reduced, to products 22–24 (Fig. 5). Although ATH was successful, the product ee's were much lower than the earlier series. An almost racemic product 22 was formed from the ketone containing an unsubstituted phenyl ring,14 although this could be improved to 55% (product 23) through the introduction of an ortho-methoxy group, possibly the result of increase steric hindrance forcing the OMe ring into a less hindered position, as has been reported for similar catalysts.15 A para-methoxy substituted ring gave a product 24 of intermediate ee (20%).
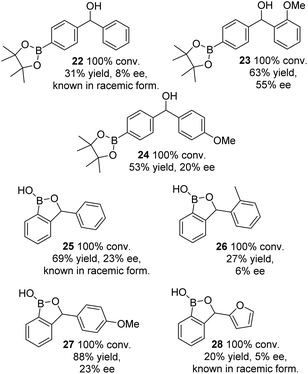 |
| Fig. 5 ATH products of Bpin-containing ketones containing aromatic rings. Conditions; 1 mol% catalyst (R,R)-2, FA/TEA, DCM, 72 h, rt. The product configurations were not confirmed. Products are novel unless otherwise indicated. | |
A series of ortho-Bpin-containing substrates were also tested, again containing a range of aromatic groups. The ee's of the products 25–28 were again low in all cases, indicating that the catalyst cannot readily distinguish between the aromatic rings flanking the ketone in each case.10 Due to the low ee values of the products, the absolute configurations of the products in Fig. 5 could not be confirmed.
To underline the value of the products, further transformations using Pd-catalysed couplings were investigated. Products 29–32, of couplings of Bpin derivatives with 2-Br styrene and 2-bromofuran were prepared (Fig. 6) without significant loss of ee in the products. Compound 29 has been prepared through the ATH of a halogenated ketone linked to a Pd-catalysed coupling with styryl boronic acid, i.e. complementary to our strategy.6b
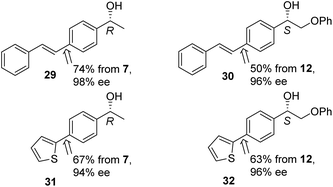 |
| Fig. 6 Coupling products of 4-Bpin reduction products; arrow indicates position of new bond. Conditions: ArBr or ArCH = CHBr, [Pd(dppf)2Cl2·DCM], dioxane, water, K3PO4, 90 °C, o/n. Starting material 7 was of 98% ee and 12 was of 97% ee. | |
Conclusion
In conclusion, we report the first application of asymmetric transfer hydrogenation, using highly practical Noyori–Ikariya catalysts, to the enantioselective reduction of ketones containing Bpin functional groups. para- and meta-Bpin-substituted acetophenones give products of very high ee whilst ortho-Bpin-containing substrates are reduced in low ee, with the formation of a cyclic product. The products can be converted to a number of products using Pd-catalysed cross coupling reactions with aryl and vinyl halides, providing a valuable complementary strategy to the use of Pd-catalysed couplings of halide-containing ATH products.
Data sharing statement
The research data (and/or materials) supporting this publication can be accessed at https://wrap.warwick.ac.uk/
Author contributions
YZ planned the investigations, completed the synthetic chemistry, analysed the data and contributed to writing the paper. MW provided supervision, planned the investigations, analysed the data and contributed to writing the paper.
Conflicts of interest
There are no conflicts to declare.
Acknowledgements
We thank Warwick University for financial support and Matthew W. M. Earl for helpful advice on the Pd-catalysed coupling reactions.
Notes and references
-
(a) D. Wang and D. Astruc, Chem. Rev., 2015, 115, 6621–6686 CrossRef CAS PubMed;
(b) A. E. Cotman, Chem. – Eur. J., 2021, 27, 39–53 CrossRef CAS PubMed;
(c) A. A. Mishra and B. M. Bhanage, Chirality, 2021, 33, 337–378 CrossRef CAS PubMed;
(d) R. Noyori and S. Hashiguchi, Acc. Chem. Res., 1997, 30, 97–102 CrossRef CAS;
(e) H. G. Nedden, A. Zanotti-Gerosa and M. Wills, Chem. Rec., 2016, 16, 2623–2643 CrossRef PubMed.
-
(a) A. Fujii, S. Hashiguchi, N. Uematsu, T. Ikariya and R. Noyori, J. Am. Chem. Soc., 1996, 118, 2521–2522 CrossRef CAS;
(b) S. Hashiguchi, A. Fujii, J. Takehara, T. Ikariya and R. Noyori, J. Am. Chem. Soc., 1995, 117, 7562–7563 CrossRef CAS;
(c) A. Kišić, M. Stephan and B. Mohar, Org. Lett., 2013, 15, 1614–1617 CrossRef PubMed;
(d) A. A. Mishra and B. M. Bhanage, ChemistrySelect, 2019, 4, 14032–14035 CrossRef CAS;
(e) J. Li, Z. Lin, Q. Huang, Q. Wang, L. Tang, J. Zhu and J. Deng, Green Chem., 2017, 19, 5367–5370 RSC;
(f) A. M. R. Hall, D. B. G. Berry, J. N. Crossley, A. Codina, I. Clegg, J. P. Lowe, A. Buchard and U. Hintermair, ACS Catal., 2021, 11, 13649–13659 CrossRef CAS PubMed;
(g) D. Zhang, T. Cheng, Q. Zhao, J. Xu and G. Liu, Org. Lett., 2014, 16, 5764–5767 CrossRef CAS PubMed;
(h) A. Kišić, M. Stephan and B. Mohar, Adv. Synth. Catal., 2015, 357, 2540–2546 CrossRef;
(i) S. Lan, H. Zhang, Z. Chen, S. Yang and X. Fang, Adv. Synth. Catal., 2021, 363, 2071–2077 CrossRef CAS.
-
(a) K. Matsumura, S. Hashiguchi, T. Ikariya and R. Noyori, J. Am. Chem. Soc., 1997, 119, 8738–8739 CrossRef CAS;
(b) V. K. Vyas, R. C. Knighton, B. M. Bhanage and M. Wills, Org. Lett., 2018, 20, 975–978 CrossRef CAS PubMed;
(c) D. Brandt, A. Dittoo, V. Bellosta and J. Cossy, Org. Lett., 2015, 17, 816–819 CrossRef CAS PubMed;
(d) Z. Fang and M. Wills, Org. Lett., 2014, 16, 374–377 CrossRef CAS PubMed;
(e) K. Siva Nagi Reddy and G. Sabitha, Tetrahedron Lett., 2017, 58, 1198–1201 CrossRef CAS;
(f) K. Takahashi, Y. Arai and T. Honda, Tetrahedron Lett., 2017, 58, 4048–4050 CrossRef CAS;
(g) N. Arai, H. Satoh, N. Utsumi, K. Murata, K. Tsutsumi and T. Ohkuma, Org. Lett., 2013, 15, 3030–3033 CrossRef CAS PubMed;
(h) G. Kumaraswamy, V. Narayanarao, P. Shanigaram and G. Balakishan, Tetrahedron, 2015, 71, 8960–8964 CrossRef CAS.
-
(a) D. Šterk, M. Stephan and B. Mohar, Org. Lett., 2006, 8, 5935–5938 CrossRef PubMed;
(b) B. Mohar, M. Stephan and U. Urleb, Tetrahedron, 2010, 66, 4144–4149 CrossRef CAS;
(c) A. E. Cotman, D. Cahard and B. Mohar, Angew. Chem., Int. Ed., 2016, 55, 5294–5298 CrossRef CAS PubMed;
(d) L.-S. Zheng, P. Phansavath and V. Ratovelomanana-Vidal, Org. Lett., 2018, 20, 5107–5111 CrossRef CAS PubMed;
(e) M. Wu, T. Cheng, M. Ji and G. Liu, J. Org. Chem., 2015, 80, 3708–3713 CrossRef CAS PubMed;
(f) T. Wang, E. M. Phillips, S. M. Dalby, E. Sirota, S. Axnanda, C. S. Shultz, P. Patel, J. H. Waldman, E. Alwedi, X. Wang, K. Zawatzky, M. Chow, N. Padivitage, M. Weisel, M. Whittington, J. Duan and T. Lu, Org. Process Res. Dev., 2022, 26, 543–550 CrossRef CAS;
(g) R. M. Betancourt, P. Phansavath and V. Ratovelomanana-Vidal, J. Org. Chem., 2021, 86, 12054–12063 CrossRef CAS PubMed;
(h) T. Touge, H. Nara, M. Kida, K. Matsumura and Y. Kayaki, Org. Lett., 2021, 23, 3070–3075 CrossRef CAS PubMed.
-
(a) P.-G. Echeverria, T. Ayad, P. Phansavath and V. Ratovelomanana-Vidal, Synthesis, 2016, 2523–2539 CAS;
(b) C. G. Goodman, D. T. Do and J. S. Johnson, Org. Lett., 2013, 15, 2446–2449 CrossRef CAS PubMed;
(c) B. Mohar, A. Valleix, J.-R. Desmurs, M. Felemez, A. Wagner and C. Mioskowski, Chem. Commun., 2001, 2572–2573 RSC;
(d) V. K. Vyas and B. M. Bhanage, Org. Lett., 2016, 18, 6436–6439 CrossRef CAS PubMed;
(e) Z.-Q. Hu, X. Li, L.-X. Liu, C.-B. Yu and Y.-G. Zhou, J. Org. Chem., 2021, 86, 17453–17461 CrossRef PubMed;
(f) G. S. Caleffi, J. d. O. C. Brum, A. T. Costa, J. L. O. Domingos and P. R. R. Costa, J. Org. Chem., 2021, 86, 4849–4858 CrossRef CAS PubMed;
(g) G. V. More, P. V. Malekar, R. G. Kalshetti, M. H. Shinde and C. V. Ramana, Tetrahedron Lett., 2021, 66, 152831 CrossRef CAS;
(h) P. Ciesielski and P. Metz, Nat. Commun., 2020, 11, 3091 CrossRef CAS PubMed;
(i) Y.-M. Zhang, Q.-Y. Zhang, D.-C. Wang, M.-S. Xie, G.-R. Qu and H.-M. Guo, Org. Lett., 2019, 21, 2998–3002 CrossRef CAS PubMed.
-
(a) L. C. Rocha, I. G. Rosset, R. F. Luiz, C. Raminelli and A. L. M. Porto, Tetrahedron: Asymmetry, 2010, 21, 926–929 CrossRef CAS;
(b) X. Shu, R. Jin, Z. Zhao, T. Cheng and G. Liu, Chem. Commun., 2018, 54, 13244–13247 RSC;
(c) M. Planellas, R. Pleixats and A. Shafir, Adv. Synth. Catal., 2012, 354, 651–662 CrossRef CAS;
(d) S. Ganesamoorthy, K. Shanmugasundaram and R. Karvembu, J. Mol. Catal. A: Chem., 2013, 371, 118–124 CrossRef CAS;
(e) M. Cubinak, V. Eigner and T. Tobrman, Adv. Synth. Catal., 2018, 360, 4604–4614 CrossRef CAS;
(f) S.-C. A. Lin, Y.-H. Liu, S.-M. Peng and S.-T. Liu, Organometallics, 2020, 39, 123–131 CrossRef CAS.
- G. Dilauro, F. Messa, F. Bona, S. Perrone and A. Salomone, Chem. Commun., 2021, 57, 10564–10567 RSC.
-
(a) L. H. Andrade and T. Barcellos, Org. Lett., 2009, 11(14), 3052–3055 CrossRef CAS PubMed;
(b) D. J. Palmeira, L. S. Araujo, J. C. Abreu and L. H. Andrade, J. Mol. Catal. B: Enzym., 2014, 110, 117–125 CrossRef CAS;
(c) T. Barcellos, K. Tauber, W. Kroutil and L. H. Andrade, Tetrahedron: Asymmetry, 2011, 22, 1772–1777 CrossRef CAS;
(d) K. S. Madden, P. M. T. Todd, K. Urata, A. J. Russell, K. A. Vincent and H. A. Reeve, ChemRxiv, 2020, 1–64, DOI:10.26434/chemrxiv.12532301.v1.
- J. Ratniyom, N. Dechnarong, S. Yotphan and S. Kiatisevi, Eur. J. Org. Chem., 2014, 1381–1385 CrossRef CAS.
-
(a) D. S. Gunasekera, D. J. Gerold, N. S. Aalderks, J. S. Chandra, C. A. Maanu, P. Kiprof, V. V. Zhdankin and M. V. Ram Reddy, Tetrahedron, 2007, 63, 9401–9405 CrossRef CAS;
(b) J. Zhu, Y. Wei, D. Lin, C. Ou, L. Xie, Yu Zhao and W. Huang, Org. Biomol. Chem., 2015, 13, 11362–11368 RSC;
(c) Y.-H. Yan, Z.-F. Li, X.-L. Ning, J. Deng, J.-L. Yu, Y. Luo, Z. Wang, G. Li, G.-B. Li and Y.-C. Xiao, Bioorg. Med. Chem. Lett., 2021, 41, 127956 CrossRef CAS PubMed.
- E. Liardo, N. Rios-Lombardia, F. Moris, J. Gonzalez-Sabin and F. Rebolledo, Eur. J. Org. Chem., 2018, 3031–3035 CrossRef CAS.
- K. Huang, M. Ortiz-Marciales, W. Correa, E. Pomales and X. Y. López, J. Org. Chem., 2009, 74, 4195–4202 CrossRef CAS PubMed.
- Q. Wang, S. Li, C.-J. Hou, T.-T. Chu and X.-P. Hu, Appl. Organomet. Chem., 2019, 33, e5108 Search PubMed.
- Y. Ashikari, T. Kawaguchi, K. Mandai, Y. Aizawa and A. Nagaki, J. Am. Chem. Soc., 2020, 142, 17039–17047 CrossRef CAS PubMed.
-
(a) Y. Zheng, J. Martinez-Acosta, M. Khimji, L. C. Barbosa, G. J. Clarkson and M. Wills, ChemCatChem, 2021, 13, 4384–4391 CrossRef CAS;
(b) T. Touge, H. Nara, M. Fujiwhara, Y. Kayaki and T. Ikariya, J. Am. Chem. Soc., 2016, 138, 10084–10087 CrossRef CAS PubMed;
(c) B. Wang, H. Zhou, G. Lu, Q. Liu and X. Jiang, Org. Lett., 2017, 19, 2094–2097 CrossRef CAS PubMed;
(d) Q. Liu, C. Wang, H. Zhou, B. Wang, J. Lv, L. Cao and Y. Fu, Org. Lett., 2018, 20, 971–974 CrossRef CAS PubMed;
(e) Y. Zheng, G. J. Clarkson and M. Wills, Org. Lett., 2020, 22, 3717–3721 CrossRef CAS PubMed.
Footnote |
† Electronic supplementary information (ESI) available: General experimental details, experimental data for the preparation and ATH of all substrates, NMR and chiral HPLC spectra. See DOI: https://doi.org/10.1039/d2ob00569g |
|
This journal is © The Royal Society of Chemistry 2022 |
Click here to see how this site uses Cookies. View our privacy policy here.